Fungi: Sex and self defense
When walking through the woods we often look up, focusing on the birds and the rustling leaves in the canopy above us, but on the ground a drama is unfolding: the fungi are under attack. Looking down you may see a slug grazing on mold that has established itself on an old log, or a mushroom swarmed by fruit flies on the forest floor. So how do fungi protect themselves from these attacks if they cannot physically escape?
Previous research has shown that fungi defend themselves using secondary metabolites, chemical compounds which are not essential for growth but involved in ecological interactions (Janzen, 1977; Rohlfs and Churchill, 2011). These compounds can be toxic to animals and/or drive them away from the fungus. As predators can appear without warning, fungi must be ready with the metabolites at short notice, either by making them ahead of time, or by rapidly creating them in response to a threat (Drott et al., 2017). However, even in the best-studied fungi, it is unclear exactly where and when these defensive chemicals are created, making it difficult to fully understand their ecological purpose.
Now, in eLife, researchers from the University of Göttingen – including Li Liu and corresponding authors Jennifer Gerke and Gerhard H Braus – report that a set of previously identified secondary metabolites called xanthones (Sanchez et al., 2011) are produced during certain life stages of the soil-dwelling fungus Aspergillus nidulans (Liu et al., 2021). Xanthones are synthesized through a series of chemical reactions controlled by a group of genes known as the mdp/xpt cluster. The proteins encoded by the mdp genes make the ‘backbone’ of the metabolite, which is then progressively modified by proteins produced from the xpt genes until the final compound is formed.
To narrow down where xanthones are synthesized in the fungus, Liu et al. added a fluorescent tag to the protein responsible for the final chemical reaction, as this represents the complete synthesis of the secondary metabolites produced by the mdp/xpt pathway. This revealed that xanthones are created in Hülle cells which support the development of cleistothecia, fruiting bodies that allow the fungus to sexually reproduce and last through the winter (Troppens et al., 2020). This suggests that xanthones are not produced throughout the life of the fungus, but are only generated during the stages of the fungus’ sexual lifecycle when cleistothecia form.
Next, Liu et al. set out to determine the role of other genes in the mdp/xpt cluster by creating a set of mutant fungi that are missing one of these genes. They found that each gene plays a specific role in the sequence of chemical reactions that synthesize the xanthones used by the fungi. As a result, none of the mutant strains were able to produce the final xanthones, and instead accumulated intermediate chemical structures that are generated during this pathway. Like xanthones, these intermediates only appeared at times when the fungus was forming cleistothecia.
It is clear from these findings that A. nidulans likely uses xanthones during sexual development; but what role do these secondary metabolites play in ecology? To investigate this, Liu et al. grew fungal colonies and cleistothecia from mutated and non-mutated (or wild-type) strains of A. nidulans and exposed them to arthropods (invertebrates with exoskeletons such as insects and arachnids) that eat fungi (Figure 1). Wild-type colonies – which can produce all of the xanthones – were damaged less heavily by the arthropods than the mutant colonies. Further experiments showed that, in addition to mitigating damage from arthropods, some of the intermediates formed during synthesis can suppress fungal growth when added to other fungi in the laboratory. However, these intermediates did not accumulate to high levels in the wild-type strain and also suppressed the development of A. nidulans, raising doubts about their potential benefit to the fungus when competing with other fungi in nature.
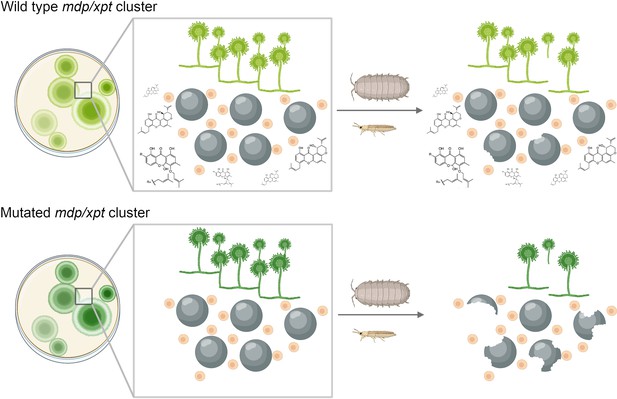
Chemical products of the mdp/xpt gene cluster protect A. nidulans from predators.
A. nidulans produces secondary metabolites called xanthones using a set of genes known as the mdp/xpt cluster. (Top) In the wild-type fungus, xanthones (shown as chemical structures) are produced by Hülle cells (small beige circles) and then accumulate in sexual fruiting bodies called cleistothecia (large black circles). When arthropods attack the wild-type fungus, the xanthones deter these predators and stop them from destroying the cleistothecia. (Bottom) Fungi with lab-induced mutations in the mdp/xpt genes are unable to produce xanthones, making them more susceptible to fungus-eating arthropods.
Image credit: Milton T Drott; Figure created using BioRender.com.
Hülle cells are found in other fungi (Dyer and O'Gorman, 2012), and genes resembling the mdp/xpt cluster occur in other species where no sexual cycle has been observed to date (de Vries et al., 2017). It remains to be seen how secondary metabolites that appear at specific life stages – like the ones in this study – translate into these other species. Furthermore, it is unclear how these chemical compounds relate to previous observations that other secondary metabolites with unknown functions are only produced under certain conditions (Georgianna et al., 2010). The findings of Liu et al. emphasize the complicated interplay between fungi and their environment, and spark further questions about about how the fungus' investment in protecting its sexual offspring has impacted its evolution.
References
-
Balancing selection for aflatoxin in Aspergillus flavus is maintained through interference competition with, and fungivory by insectsProceedings of the Royal Society B: Biological Sciences 284:20172408.https://doi.org/10.1098/rspb.2017.2408
-
Sexual development and cryptic sexuality in fungi: insights from Aspergillus speciesFEMS Microbiology Reviews 36:165–192.https://doi.org/10.1111/j.1574-6976.2011.00308.x
-
Why fruits rot, seeds mold, and meat spoilsThe American Naturalist 111:691–713.https://doi.org/10.1086/283200
-
Fungal secondary metabolites as modulators of interactions with insects and other arthropodsFungal Genetics and Biology 48:23–34.https://doi.org/10.1016/j.fgb.2010.08.008
-
Genome-based deletion analysis reveals the prenyl xanthone biosynthesis pathway in Aspergillus nidulansJournal of the American Chemical Society 133:4010–4017.https://doi.org/10.1021/ja1096682
Article and author information
Author details
Publication history
Copyright
© 2021, Drott
This article is distributed under the terms of the Creative Commons Attribution License, which permits unrestricted use and redistribution provided that the original author and source are credited.
Metrics
-
- 1,366
- views
-
- 101
- downloads
-
- 2
- citations
Views, downloads and citations are aggregated across all versions of this paper published by eLife.
Download links
Downloads (link to download the article as PDF)
Open citations (links to open the citations from this article in various online reference manager services)
Cite this article (links to download the citations from this article in formats compatible with various reference manager tools)
Further reading
-
- Biochemistry and Chemical Biology
- Genetics and Genomics
Yerba mate (YM, Ilex paraguariensis) is an economically important crop marketed for the elaboration of mate, the third-most widely consumed caffeine-containing infusion worldwide. Here, we report the first genome assembly of this species, which has a total length of 1.06 Gb and contains 53,390 protein-coding genes. Comparative analyses revealed that the large YM genome size is partly due to a whole-genome duplication (Ip-α) during the early evolutionary history of Ilex, in addition to the hexaploidization event (γ) shared by core eudicots. Characterization of the genome allowed us to clone the genes encoding methyltransferase enzymes that catalyse multiple reactions required for caffeine production. To our surprise, this species has converged upon a different biochemical pathway compared to that of coffee and tea. In order to gain insight into the structural basis for the convergent enzyme activities, we obtained a crystal structure for the terminal enzyme in the pathway that forms caffeine. The structure reveals that convergent solutions have evolved for substrate positioning because different amino acid residues facilitate a different substrate orientation such that efficient methylation occurs in the independently evolved enzymes in YM and coffee. While our results show phylogenomic constraint limits the genes coopted for convergence of caffeine biosynthesis, the X-ray diffraction data suggest structural constraints are minimal for the convergent evolution of individual reactions.
-
- Biochemistry and Chemical Biology
- Structural Biology and Molecular Biophysics
The SARS-CoV-2 main protease (Mpro or Nsp5) is critical for production of viral proteins during infection and, like many viral proteases, also targets host proteins to subvert their cellular functions. Here, we show that the human tRNA methyltransferase TRMT1 is recognized and cleaved by SARS-CoV-2 Mpro. TRMT1 installs the N2,N2-dimethylguanosine (m2,2G) modification on mammalian tRNAs, which promotes cellular protein synthesis and redox homeostasis. We find that Mpro can cleave endogenous TRMT1 in human cell lysate, resulting in removal of the TRMT1 zinc finger domain. Evolutionary analysis shows the TRMT1 cleavage site is highly conserved in mammals, except in Muroidea, where TRMT1 is likely resistant to cleavage. TRMT1 proteolysis results in reduced tRNA binding and elimination of tRNA methyltransferase activity. We also determined the structure of an Mpro-TRMT1 peptide complex that shows how TRMT1 engages the Mpro active site in an uncommon substrate binding conformation. Finally, enzymology and molecular dynamics simulations indicate that kinetic discrimination occurs during a later step of Mpro-mediated proteolysis following substrate binding. Together, these data provide new insights into substrate recognition by SARS-CoV-2 Mpro that could help guide future antiviral therapeutic development and show how proteolysis of TRMT1 during SARS-CoV-2 infection impairs both TRMT1 tRNA binding and tRNA modification activity to disrupt host translation and potentially impact COVID-19 pathogenesis or phenotypes.