Cell and circuit origins of fast network oscillations in the mammalian main olfactory bulb
Figures
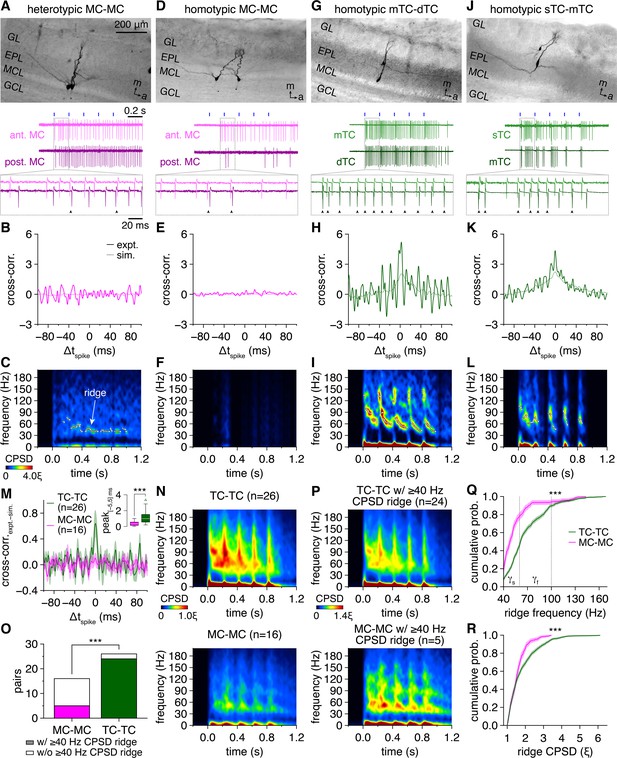
Multiglomerular activation evokes widespread synchronization of tufted cell (TC) firing across fast- and slow-gamma frequencies and limited synchronization of mitral cell (MC) firing across slow-gamma frequencies.
(A) Example cell-attached recording of a heterotypic MC pair during photostimulation. Morphology (upper) and representative trial (lower; blue rectangles: 10 ms light pulses of the 5 Hz photostimulation protocol; arrowheads: synchronous spikes, |Δtspike| ≤ 5 ms). GL: glomerular layer; EPL: external plexiform layer; MCL: mitral cell layer; GCL: granule cell layer; m: medial; a/ant.: anterior; post.: posterior. (B) Trial-averaged cross-correlogram of spike times recorded throughout the photostimulation protocol in the MC pair in (A) (‘expt.’), compared to the cross-correlogram of spike times simulated from rate-matched independent Poisson processes (‘sim.’). (C) Trial-averaged spike-time cross-power spectral density (CPSD) spectrogram from the MC pair in (A) following photostimulation onset at 0.0 s. Continuous epochs (ΔHz/ms < 150) of high CPSD reflecting robust periodic synchrony are defined as ‘ridges’ and demarcated with white circles. Color is scaled by multiples of the ridge threshold (ξ). (D–L) Same as (A–C) for a homotypic MC pair (D–F), a homotypic middle TC (mTC) and deep TC (dTC) pair (G–I), and a homotypic superficial TC (sTC) and mTC pair (J–L). Scaling in (F, I, L) is the same as in (C). (M) Mean spike-time cross-correlograms (with slow-timescale firing rate correlations removed via subtraction of the simulated spike-time cross-correlograms) of TC pairs and MC pairs. Inset: cross-correlogram peaks within |Δtspike| ≤ 5 ms were higher among TCs than MCs (Wilcoxon rank-sum test: p=3.0 × 10–4). (N) Spike-time CPSD spectrograms averaged across all TC pairs (upper) and MC pairs (lower). (O) More TC than MC pairs exhibited spike-time CPSD ridges (chi-squared test: p=3.2 × 10–5, χ2 = 17.3). (P) Spike-time CPSD spectrograms averaged across all TC pairs (upper) and MC pairs (lower) exhibiting CPSD ridges. (Q, R) Cumulative distributions of frequencies (Q) and CPSD (R) across all spike-time CPSD ridges. TCs exhibited faster (Q) (two-sample Kolmogorov–Smirnov test: p=1.7 × 10–44) and more precise (R) (two-sample Kolmogorov–Smirnov test: p=3.2 × 10–11) gamma-frequency synchrony than MCs. Shading denotes 95%confidence intervals. γs: slow-gamma frequencies, 40–60 Hz; γf: fast-gamma frequencies, 60–100 Hz.
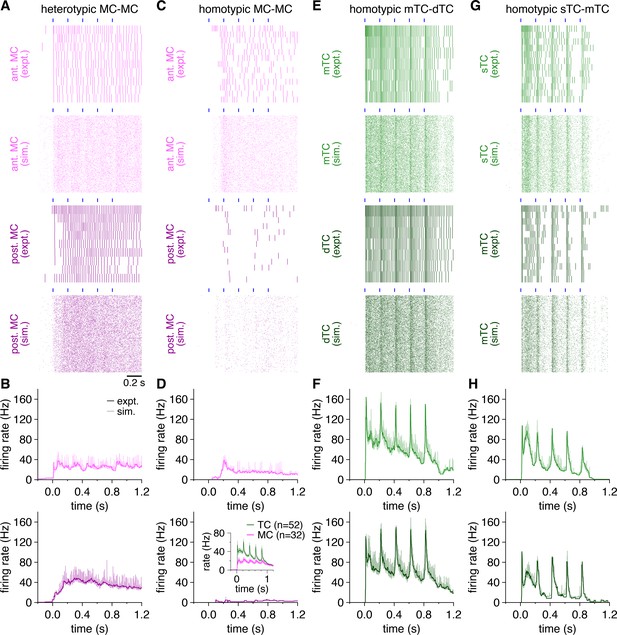
Multiglomerular activation evokes higher firing rates and more phasic firing patterns among tufted cells (TCs) than mitral cells (MCs).
(A) Raster plots of spike times recorded during photostimulation across all trials in the MCs in Figure 1A (‘expt.’), as well as across 500 trials of spike times simulated from rate-matched independent Poisson processes (‘sim.’). Blue rectangles: 10 ms light pulses of the 5 Hz photostimulation protocol. (B) Trial-averaged experimental and simulated firing rates of the MCs in Figure 1A following photostimulation onset at 0.0 s. (C–H) Same as (A, B) for the MCs and TCs in Figure 1D, G and J. Inset: average firing rates across all MCs and TCs.
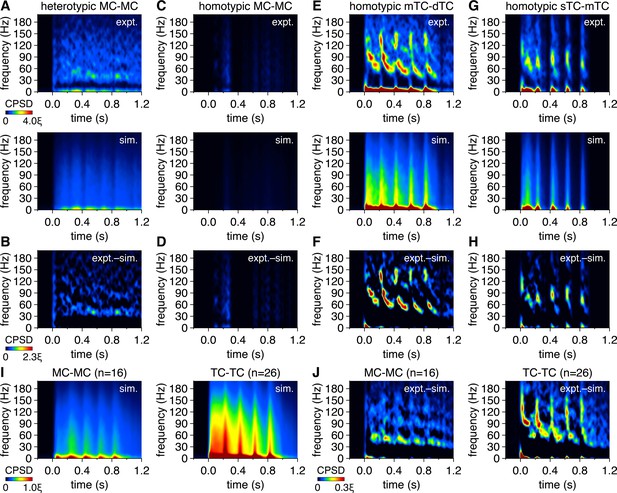
Mitral cell (MC) pairs and tufted cell (TC) pairs exhibit greater gamma-frequency spike-time synchrony than spike times simulated from rate-matched independent Poisson processes.
(A) Trial-averaged experimental spike-time cross-power spectral density (CPSD) spectrogram from the MC pair in Figure 1A following photostimulation onset at 0.0 s (upper), and equivalent trial-averaged CPSD spectrogram of spike times simulated across 500 trials from rate-matched independent Poisson processes (lower). Scaling is identical to Figure 1A. (B) Subtraction of simulated spike-time CPSD spectrogram from experimental spike-time CPSD spectrogram. Color is scaled from 0 to multiples of ξ to visualize periodic synchrony within experimental spike times exceeding that within simulated spike times. (C–H) Same as (A, B) for the MC pair and TC pairs in Figure 1D, G and J. Scaling in (C, E, G) and (D, F, H) is the same as in (A) and (B), respectively. (I) Simulated spike-time CPSD spectrograms averaged across all MC pairs (left) and TC pairs (right). Color is scaled identical to Figure 1N. (J) Subtraction of simulated spike-time CPSD spectrograms from experimental spike-time CPSD spectrograms, averaged across all MC pairs (left) and TC pairs (right), revealing greater gamma-frequency CPSD among experimental than simulated spike times.
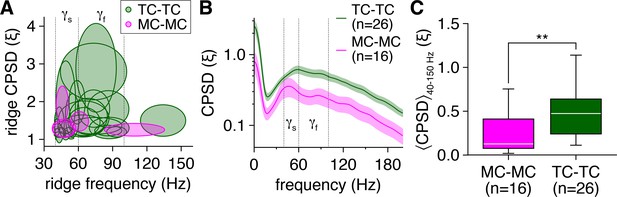
Tufted cells (TCs) exhibit greater gamma-frequency spike-time synchrony than mitral cells (MCs) on both a per-pair basis and independent of ridge-based analyses.
(A) Distribution of ridge cross-power spectral density (CPSD) vs. frequency for the 5/16 MC pairs and 24/26 TC pairs exhibiting spike-time CPSD ridges. Ellipses denote mean ± standard deviation of CPSD and frequencies across all ridges detected in each pair. (B) Time-independent spike-time CPSD of MC pairs and TC pairs recorded throughout the photostimulation protocol. (C) TC pairs exhibited greater spike-time CPSD averaged across 40–150 Hz (corresponding to the range of essentially all detected spike-time CPSD ridges) and throughout the photostimulation protocol than MC pairs (Wilcoxon rank-sum test: p=2.8 × 10–3).
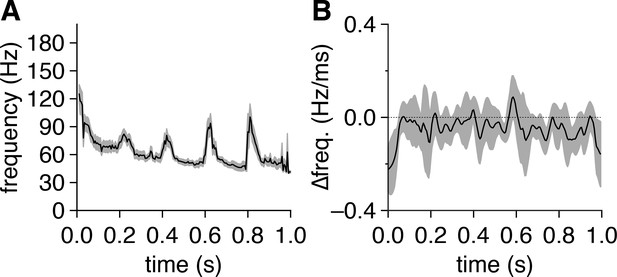
Fast-gamma-frequency spike-time synchrony emerges early in each 5 Hz photostimulation cycle and decelerates toward slow-gamma frequencies.
(A) Mean spike-time cross-power spectral density (CPSD) ridge frequency across all pairs following photostimulation onset at 0.0 s. (B) Mean derivative of spike-time CPSD ridge frequency across all pairs (with 50-ms-long sliding window average applied).
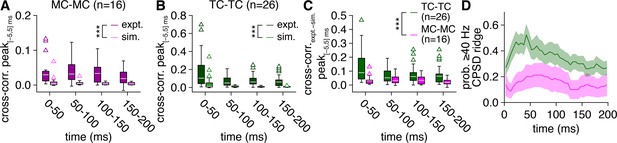
Greater synchronization of tufted cell (TC) than mitral cell (MC) firing persists throughout the average 5 Hz photostimulation cycle.
(A) Experimental spike times recorded across MC pairs (‘expt.’) within consecutive 50 ms windows of the 5 Hz photostimulation cycle exhibited consistently higher cross-correlogram peaks (within |Δtspike| ≤ 5 ms) than spike times simulated from rate-matched independent Poisson processes (‘sim.’) (two-way ANOVA on ranks, expt./sim. × 50 ms window: significant main effect of expt./sim., p=1.2 × 10–9, F1,120 = 43.6; no significant main effect of 50 ms window, p=0.34, F3,120 = 1.1; no significant interaction, p=0.99, F3,120 = 0.03). (B) Experimental spike times recorded across TC pairs (‘expt.’) likewise exhibited consistently higher cross-correlogram peaks than spike times simulated from rate-matched independent Poisson processes (‘sim.’) (two-way ANOVA on ranks, expt./sim. × 50 ms window: significant main effect of expt./sim., p=1.2 × 10–19, F1,200 = 102.1; no significant main effect of 50 ms window, p=0.34, F3,200 = 1.1; no significant interaction, p=0.62, F3,200 = 0.6). (C) Spike-time cross-correlograms (with slow-timescale firing rate correlations removed via subtraction of simulated spike-time cross-correlograms) within consecutive 50 ms windows of the 5 Hz photostimulation cycle exhibited consistently higher peaks (within |Δtspike| ≤ 5 ms) among TC than MC pairs (two-way ANOVA on ranks, cell type × 50 ms window: significant main effect of cell type, p=4.6 × 10–4, F1,160 = 12.8; no significant main effect of 50 ms window, p=0.23, F3,160 = 1.5; no significant interaction, p=0.90, F3,160 = 0.2). (D) The probability of robust periodic spike-time synchrony reflected in spike-time cross-power spectral density (CPSD) ridges was consistently higher among TC than MC pairs throughout the average photostimulation cycle.
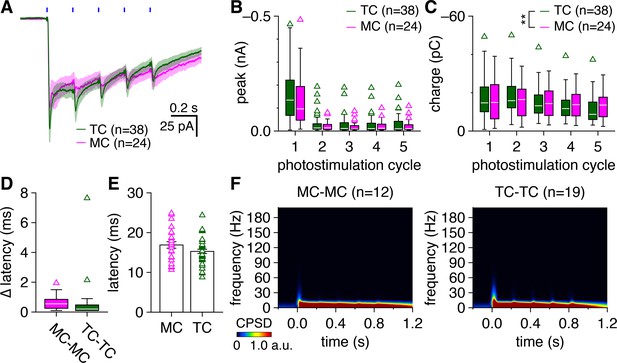
Full-field photostimulation evokes comparable excitatory currents in tufted cells (TCs) and mitral cells (MCs).
(A) Trial-averaged excitatory currents recorded in TCs and MCs during photostimulation. Blue rectangles: 10 ms light pulses of the 5 Hz photostimulation protocol. (B) TCs and MCs exhibited comparable peak excitatory current amplitudes (relative to currents immediately preceding each photostimulation light pulse) (two-way ANOVA on ranks, cell type × photostimulation cycle: no significant main effect of cell type, p=0.76, F1,300 = 0.1; no significant main effect of photostimulation cycle, p=0.74, F4,300 = 0.5; no significant interaction, p=0.25, F4,300 = 1.4). (C) MCs exhibited greater excitatory charge transferred (i.e., integrated current) throughout each photostimulation cycle than TCs (two-way ANOVA on ranks, cell type × photostimulation cycle: significant main effect of cell type, p=2.7 × 10–3, F1,300 = 9.1; no significant main effect of photostimulation cycle, p=0.70, F4,300 = 0.6; no significant interaction, p=0.93, F4,300 = 0.21). (D) MCs and TCs exhibited comparable within-pair cell-to-cell differences in excitatory input latency following photostimulation onset (Wilcoxon rank-sum test: p= 0.12). (E) MCs and TCs exhibited comparable absolute latencies to excitatory input following photostimulation onset (two-sample t-test: p = 0.092, t60 = 1.7). (F) Cross-power spectral density (CPSD) spectrograms of excitatory currents averaged across all MC pairs (left) and TC pairs (right). Color is scaled to saturate theta-frequency components, which failed to reveal any gamma-frequency synchronous patterning.
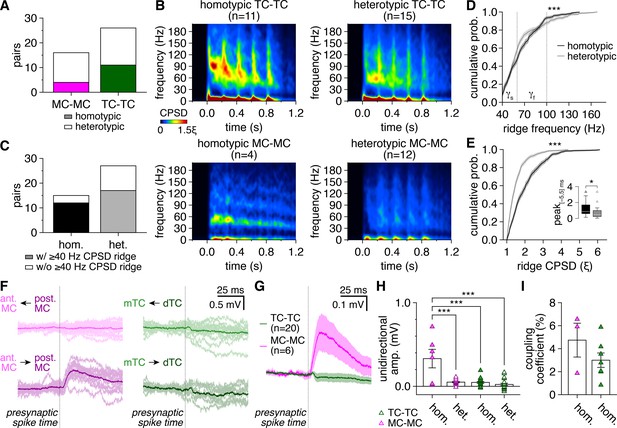
Greater synchronization of tufted cell (TC) than mitral cell (MC) firing extends across distinct patterns of glomerular organization and is not driven by intraglomerular lateral excitation.
(A) MCs and TCs included comparable proportions of homotypic and heterotypic pairs (chi-squared test: p=0.26, χ2 = 1.3). (B) Spike-time cross-power spectral density (CPSD) spectrograms averaged across all homotypic (left) and heterotypic (right) TC pairs (upper) and MC pairs (lower) following photostimulation onset at 0.0 s. (C) Pairs with spike-time CPSD ridges were detected among both homotypic pairs (‘hom.’) and heterotypic pairs (‘het.’) at comparable rates (chi-squared test: p=0.25, χ2 = 1.3). (D, E) Cumulative distributions of frequencies (D) and CPSD (E) across all spike-time CPSD ridges for homotypic and heterotypic pairs. Epochs of periodic synchrony were distributed across significantly different, though largely overlapping, frequencies among homotypic and heterotypic pairs (D) (two-sample Kolmogorov–Smirnov test: p=3.8 × 10–7), while periodic synchrony was substantially more precise among homotypic than heterotypic pairs (E) (two-sample Kolmogorov–Smirnov test: p=1.6 × 10–51). Shading denotes 95%confidence intervals. Inset: consistent with more precise synchrony, spike-time cross-correlogram peaks within |Δtspike| ≤ 5 ms (as in Figure 1M) were higher among homotypic than heterotypic pairs (Wilcoxon rank-sum test: p=0.033). (F) Unitary postsynaptic responses to single presynaptic spikes in the MC pair (left) and TC pair (right) in Figure 1D and G, revealing reliable asymmetric lateral excitation selectively between the homotypic MCs. Arrows: direction of transmission tested. Light traces: individual trials; dark traces: average. (G) Mean unitary postsynaptic response to single presynaptic spikes across homotypic MC pairs and homotypic TC pairs, revealing consistent intraglomerular lateral excitation among MCs and no visible excitation among TCs. (H) Lateral excitation (typically asymmetric within pairs) was exclusively detected among homotypic MCs, as revealed by stronger unitary postsynaptic response amplitudes across homotypic MC pairs than across either TC pairs or heterotypic MC pairs (two-way ANOVA, cell type × glomerular organization: significant main effect of cell type, p=1.4 × 10–7, F1,60 = 35.6; significant main effect of glomerular organization, p=2.4 × 10–7, F1,60 = 33.9; significant interaction, p=7.4 × 10–6, F1,60 = 24.1; post-hoc Tukey–Kramer test: homotypic MC-MC vs. heterotypic MC-MC, p=1.7 × 10–7; homotypic MC-MC vs. homotypic TC-TC, p=6.2 × 10–8; homotypic MC-MC vs. heterotypic TC-TC, p=8.5 × 10–9; heterotypic MC-MC vs. homotypic TC-TC, p=1.0; heterotypic MC-MC vs. heterotypic TC-TC, p=0.80; homotypic TC-TC vs. heterotypic TC-TC, p=0.83). (I) Homotypic MC pairs and homotypic TC pairs exhibited comparable electrical coupling coefficients (two-sample t-test: p=0.22, t8 = 1.3).
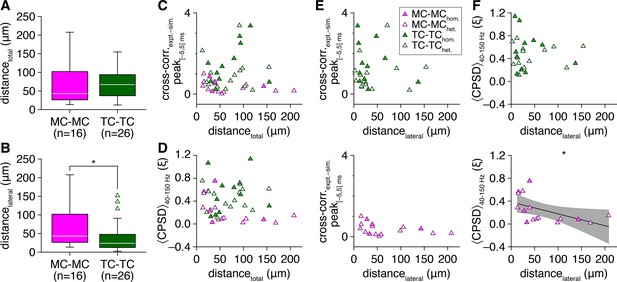
Profound cell-type differences in spike-time synchrony cannot be explained by differences in within-pair intersomatic distance.
(A, B) Mitral cells (MCs) and tufted cells (TCs) exhibited comparable within-pair total intersomatic distance (A) (Wilcoxon rank-sum test: p=0.51), while TCs exhibited shorter within-pair lateral intersomatic distance (i.e., distance parallel to the mitral cell layer) than MCs (B) (Wilcoxon rank-sum test: p=0.035). (C, D) Within-pair total intersomatic distance across both cell types did not correlate with spike-time cross-correlogram peaks within |Δtspike| ≤ 5 ms (as in Figure 1M) (C) (linear regression, slope not significantly different from 0: p=0.64, t40 = 0.48) or with spike-time cross-power spectral density (CPSD) averaged throughout the photostimulation protocol and across 40–150 Hz (D) (linear regression, slope not significantly different from 0: p=0.44, t40=–0.78). (E) Within-pair lateral intersomatic distance did not correlate with spike-time cross-correlogram peaks across TC pairs (upper) (linear regression, slope not significantly different from 0: p=0.89, t24=–0.14) or MC pairs (lower) (linear regression, slope not significantly different from 0: p=0.14, t14=–1.6). (F) Within-pair lateral intersomatic distance did not correlate with spike-time CPSD averaged throughout the photostimulation protocol and across 40–150 Hz across TC pairs (upper) (linear regression, slope not significantly different from 0: p=0.83, t24=–0.21) but did across MC pairs, exhibiting a negative relationship (linear regression, slope significantly different from 0: p=0.038, t14 = –2.3; R2 = 0.27).
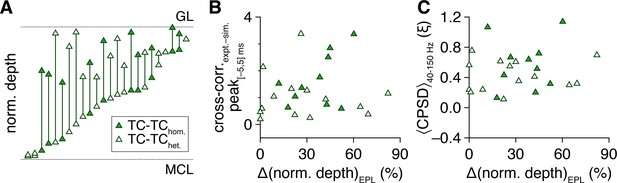
Tufted cell (TC) spike-time synchrony does not correlate with within-pair intersomatic differences in external plexiform layer (EPL) depth.
(A) Normalized depth of TC somata within the EPL; lines connect recorded pairs. (B, C) Within-pair intersomatic differences in EPL depth did not correlate with spike-time cross-correlogram peaks within |Δtspike| ≤ 5 ms (as in Figure 1M) (B) (linear regression, slope not significantly different from 0: P = 0.47, t22 = 0.73) or with spike-time cross-power spectral density (CPSD) averaged throughout the photostimulation protocol and across 40–150 Hz (C) (linear regression, slope not significantly different from 0: p=0.52, t22 = 0.66).
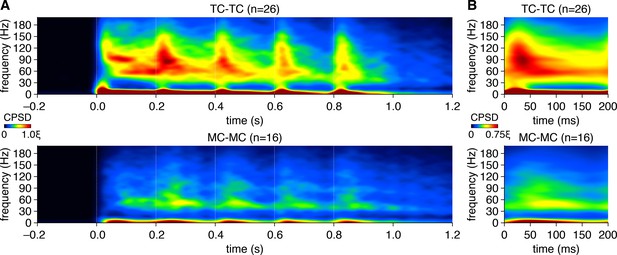
Mitral cells (MCs) and tufted cells (TCs) exhibit distinct patterns of gamma-frequency spike-time synchrony across the 5 Hz photostimulation cycle.
(A) Spike-time cross-power spectral density (CPSD) spectrograms averaged across all TC pairs (upper) and MC pairs (lower) expanded in time across consecutive photostimulation cycles (dashed lines). Scaling is identical to Figure 1N. (B) Same as (A), averaged across all photostimulation cycles.

Tufted cells (TCs) exhibit greater spike-time synchrony across theta frequencies than mitral cells (MCs).
(A) Expanded-timescale cross-correlogram of spike times recorded in the MC pair in Figure 1A, with 50-ms-long sliding window average applied to highlight temporal patterning at frequencies < 20 Hz. (B) Spike-time cross-power spectral density (CPSD) spectrogram of spike times recorded in the MC pair in Figure 1A, rescaled to theta frequencies. (C–H) Same as (A, B) for the MC pair and TC pairs in Figure 1D, G and J. (I) Mean expanded-timescale cross-correlograms of TC pairs and MC pairs. (J) Spike-time CPSD spectrograms averaged across all MC pairs (left) and TC pairs (right), rescaled to theta frequencies. (K) TC pairs exhibited greater spike-time CPSD averaged throughout the photostimulation protocol and across 2–12 Hz than MC pairs (Wilcoxon rank-sum test: p=1.1 × 10–3).
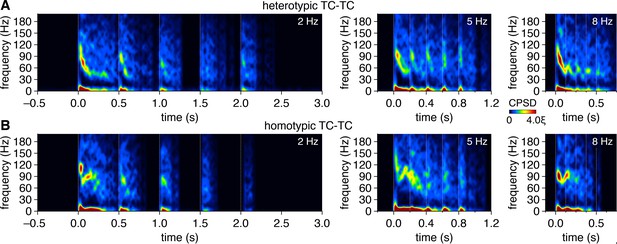
Multiglomerular activation at different theta frequencies evokes qualitatively similar patterns of periodic spike-time synchrony.
(A, B) Trial-averaged cross-power spectral density (CPSD) spectrograms of spike times evoked in a heterotypic tufted cell (TC) pair (A) and homotypic TC pair (B) following 2 Hz, 5 Hz, and 8 Hz photostimulation.
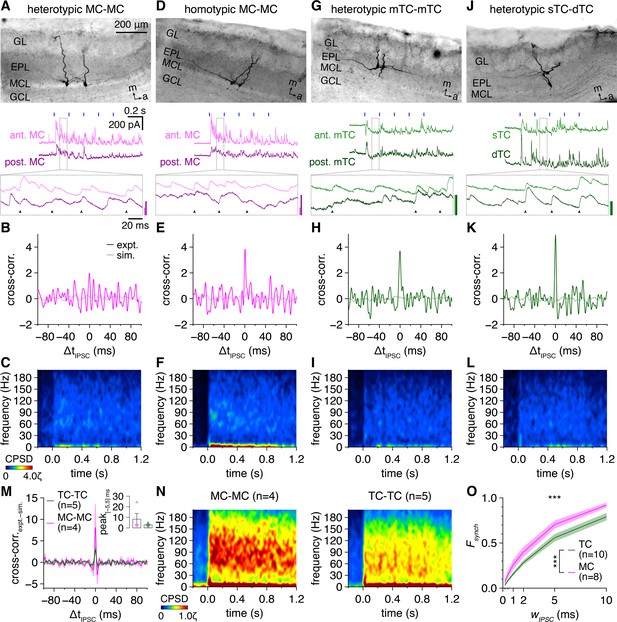
Multiglomerular activation evokes weak synchronization of inhibitory postsynaptic current (IPSC) times across gamma frequencies among both mitral cells (MCs) and tufted cells (TCs).
(A) Example voltage-clamp recording of a heterotypic MC pair during photostimulation. Morphology (upper) and representative trial (lower; blue rectangles: 10 ms light pulses of the 5 Hz photostimulation protocol; arrowheads: synchronous IPSCs, |ΔtIPSC| ≤ 1 ms). Inset scale bars: 100 pA. (B) Trial-averaged cross-correlogram of IPSC times recorded throughout the photostimulation protocol in the MC pair in (A) (‘expt.’) compared to the cross-correlogram of IPSC times simulated from rate-matched independent Poisson processes (‘sim.’). (C) Trial-averaged IPSC-time cross-power spectral density (CPSD) spectrogram from the MC pair in (A) following photostimulation onset at 0.0 s. Color is scaled by multiples of the 95th percentile of 40–200 Hz CPSD values observed throughout the photostimulation protocol in all pairs (ζ). No ridge analysis was applied given comparable levels of experimental and simulated IPSC-time CPSD across gamma frequencies (Figure 6—figure supplement 4). (D–L) Same as (A–C) for a homotypic MC pair (D–F), a heterotypic mTC pair (G–I), and a heterotypic sTC-dTC pair (J–L). Scaling in (F, I ,L) is the same as in (C). (M) Mean IPSC-time cross-correlograms (following subtraction of simulated IPSC-time cross-correlograms) of TC pairs and MC pairs. Inset: cross-correlogram peaks within |ΔtIPSC| ≤ 5 ms tended to be higher across MC than TC pairs (two-sample t-test: p=0.30, t7 = 1.1). (N) IPSC-time CPSD spectrograms averaged across all MC pairs (left) and TC pairs (right). (O) MCs exhibited a consistently greater fraction of IPSCs occurring synchronously (‘Fsynch’) within a specific time window (‘wIPSC’) than TCs (two-way ANOVA, cell type × wIPSC; significant main effect of cell type, p=1.7 × 10–7, F1,96 = 31.9; significant main effect of wIPSC, p=5.9 × 10–50, F5,96 = 208.9; no significant interaction, p=0.50, F5,96 = 0.87).
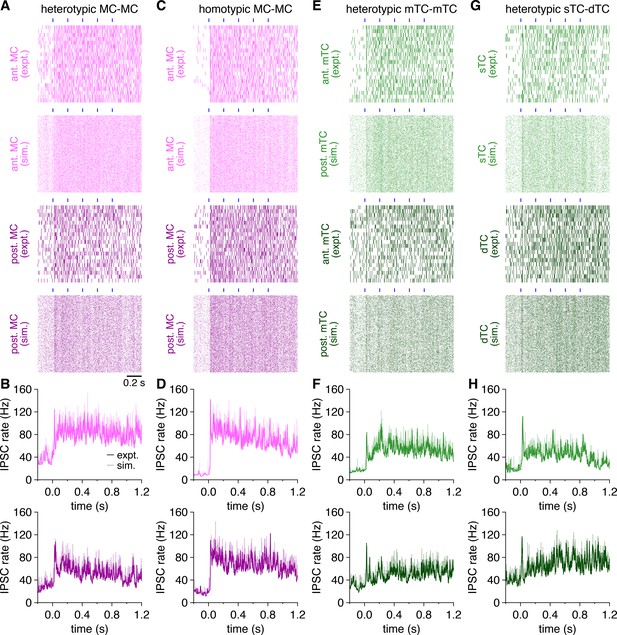
Multiglomerular activation evokes a tonic increase in inhibitory postsynaptic current (IPSC) rates among both mitral cells (MCs) and tufted cells (TCs).
(A) Raster plots of IPSC times recorded during photostimulation across all trials in the MCs in Figure 6A (‘expt.’), as well as across 500 trials of spike times simulated from rate-matched independent Poisson processes (‘sim.’). Blue rectangles: 10 ms light pulses of the 5 Hz photostimulation protocol. (B) Trial-averaged experimental and simulated IPSC rates of the MCs in Figure 6A following photostimulation onset at 0.0 s. (C–H) Same as (A, B) for the MCs and TCs in Figure 6D, G and J.

Spontaneous and evoked inhibitory postsynaptic current (IPSC) properties are similar among mitral cells (MCs) and tufted cells (TCs).
(A, B) Trial-averaged IPSC rates (A) and amplitudes (B; averaged within 20 ms time bins) across all TCs and MCs during photostimulation. Blue rectangles: 10 ms light pulses of the 5 Hz photostimulation protocol. (C) Median IPSC waveforms recorded during the 5 s preceding photostimulation onset (‘spont.’) and throughout the photostimulation protocol (‘evoked’) in the MCs and TCs in Figure 6A, D, G and J. (D) Photostimulation markedly increased IPSC rates in both MCs and TCs, with a greater rate of evoked IPSCs in MCs than TCs (two-way ANOVA, cell type × IPSC type: significant main effect of cell type, p=4.1 × 10–3, F1,32 = 9.5; significant main effect of IPSC type, p=2.2 × 10–13, F1,32 = 144.2; no significant interaction, p=0.16, F1,32 = 2.0; post-hoc Tukey–Kramer test: MC spont. vs. MC evoked, p=5.4 × 10–9; TC spont. vs. TC evoked, p=3.1 × 10–8; MC evoked vs. TC spont., p=3.8 × 10–9; MC spont. vs. TC evoked, p=2.6 × 10–6; MC evoked vs. TC evoked, p=0.016). (E) Evoked IPSCs exhibited greater median amplitudes than spontaneous IPSCs in both MCs and TCs (two-way ANOVA, cell type × IPSC type: no significant main effect of cell type, p=0.10, F1,32 = 2.9; significant main effect of IPSC type, p=1.5 × 10–3, F1,32 = 12.1; no significant interaction, p=0.33, F1,32 = 0.96). (F) Evoked and spontaneous IPSCs exhibited comparable median rise times in both MCs and TCs (two-way ANOVA, cell type × IPSC type: no significant main effect of cell type, p=0.34, F1,32 = 0.95; no significant main effect of IPSC type, p=0.19, F1,32 = 1.8; no significant interaction, p=0.72, F1,32 = 0.13). (G) Median evoked IPSCs exhibited faster decay constants than median spontaneous IPSCs in both MCs and TCs (two-way ANOVA, cell type × IPSC type: no significant main effect of cell type, p=0.87, F1,32 = 0.026; significant main effect of IPSC type, p=1.5 × 10–5, F1,32 = 26.0; no significant interaction, p=0.13, F1,32 = 2.5).
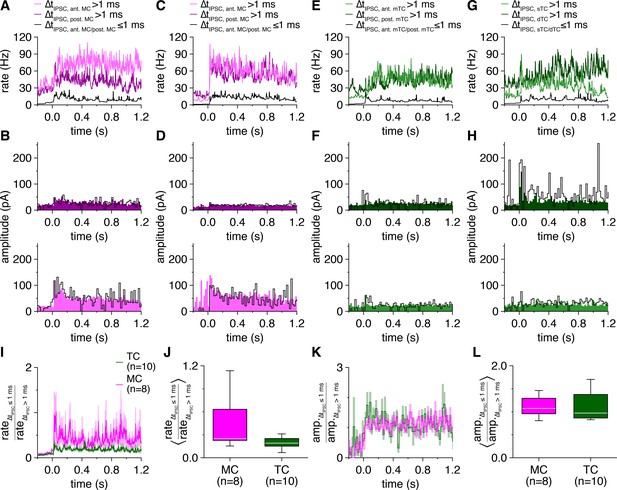
Mitral cells (MCs) and tufted cells (TCs) exhibit comparable proportions of evoked synchronous to asynchronous inhibitory postsynaptic current (IPSC) rates and amplitudes.
(A) Trial-averaged rates of IPSCs occurring synchronously (|ΔtIPSC| ≤ 1 ms) and asynchronously (|ΔtIPSC| > 1 ms) between MCs of the pair in Figure 6A following photostimulation onset at 0.0 s. Synchronous IPSC rates, nearly identical across cells by definition, are plotted as an average. (B) Amplitudes of IPSCs occurring synchronously and asynchronously between MCs of the pair in Figure 6A, averaged across trials within 20 ms bins. (C–H) Same as (A, B) for the MC pair and TC pairs in Figure 6D, G and J. (I) Mean ratio of synchronous to asynchronous IPSC rates among MCs and TCs. (J) The ratio of synchronous to asynchronous IPSC rates averaged throughout the photostimulation protocol tended to be higher among MCs than TCs (Wilcoxon rank-sum test: p=0.068). (K) Mean ratio of synchronous to asynchronous IPSC amplitudes among MCs and TCs. (L) MCs and TCs exhibited comparable ratios of synchronous to asynchronous IPSC amplitudes averaged throughout the photostimulation protocol (Wilcoxon rank-sum test: p=0.83).
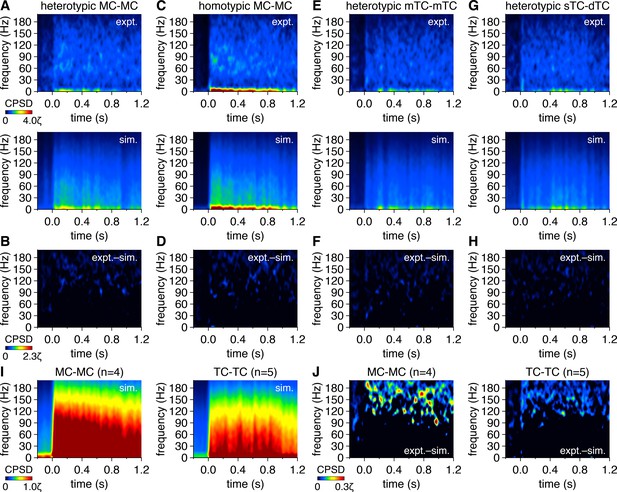
Mitral cell (MC) pairs and tufted cell (TC) pairs do not exhibit greater gamma-frequency inhibitory postsynaptic current (IPSC)-time synchrony than IPSC times simulated from rate-matched independent Poisson processes.
(A) Trial-averaged experimental IPSC-time cross-power spectral density (CPSD) spectrogram from the MC pair in Figure 6A following photostimulation onset at 0.0 s (upper), and equivalent trial-averaged CPSD spectrogram of IPSC times simulated across 500 trials from rate-matched independent Poisson processes (lower). Scaling is identical to Figure 6A. (B) Subtraction of simulated IPSC-time CPSD spectrogram from experimental IPSC-time CPSD spectrogram. Color is scaled from 0 to multiples of ζ to visualize periodic synchrony within experimental IPSC times exceeding that within simulated IPSC times. (C–H) Same as (A, B) for the MC pair and TC pairs in Figure 6D, G and J. Scaling in (C, E, G) and (D, F, H) is the same as in (A) and (B), respectively. (I) Simulated IPSC-time CPSD spectrograms averaged across all MC pairs (left) and TC pairs (right). Color is scaled identical to Figure 6N. (J) Subtraction of simulated IPSC-time CPSD spectrograms from experimental IPSC-time CPSD spectrograms, averaged across all MC pairs (left) and TC pairs (right), revealing that gamma-frequency CPSD among experimental IPSC times is no greater than chance levels observed among independent Poisson processes.
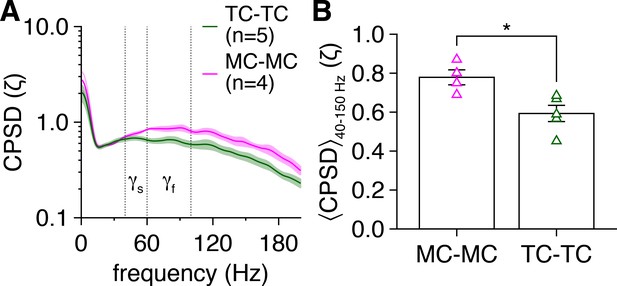
Tufted cells (TCs) do not exhibit greater gamma-frequency inhibitory postsynaptic current (IPSC)-time synchrony.
(A) Time-independent IPSC-time cross-power spectral density (CPSD) of mitral cell (MC) pairs and TC pairs recorded throughout the photostimulation protocol. (B) MC pairs exhibited greater IPSC-time CPSD averaged across 40–150 Hz and throughout the photostimulation protocol than TC pairs (two-sample t-test: p=0.015, t7 = 3.2).

Inhibitory postsynaptic current (IPSC)-time synchrony does not correlate with within-pair intersomatic distance.
(A, B) Mitral cells (MCs) and tufted cells (TCs) exhibited comparable within-pair total intersomatic distance (A) (two-sample t-test: p=0.93, t7 = 0.089) and lateral intersomatic distance (B) (two-sample t-test: p=0.28, t7 = 1.2). (C, D) Within-pair total intersomatic distance across both cell types did not correlate with IPSC-time cross-correlogram peaks within |ΔtIPSC| ≤ 5 ms (as in Figure 6M) (C) (linear regression, slope not significantly different from 0: p=0.58, t7=–0.58) or with IPSC-time cross-power spectral density (CPSD) averaged throughout the photostimulation protocol and across 40–150 Hz (D) (linear regression, slope not significantly different from 0: p=0.35, t7 = –0.99). (E, F) Within-pair lateral intersomatic distance across both cell types likewise did not correlate with IPSC-time cross-correlogram peaks (E) (linear regression, slope not significantly different from 0: p=0.93, t7 = –0.087) or with IPSC-time CPSD averaged throughout the photostimulation protocol and across 40–150 Hz (F) (linear regression, slope not significantly different from 0: p=0.69, t7 = 0.41).
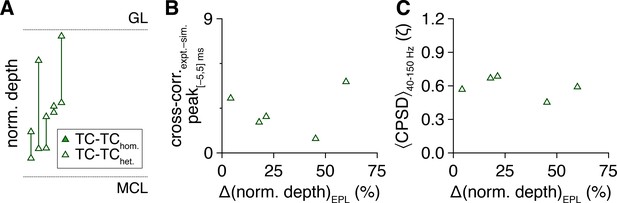
Tufted cell (TC) inhibitory postsynaptic current (IPSC)-time synchrony does not correlate with within-pair intersomatic differences in external plexiform layer (EPL) depth.
(A) Normalized depth of TC somata within the EPL; lines connect recorded pairs. (B, C) Within-pair intersomatic differences in EPL depth did not correlate with IPSC-time cross-correlogram peaks within |ΔtIPSC| ≤ 5 ms (as in Figure 6M) (B) (linear regression, slope not significantly different from 0: p=0.80, t3 = 0.27) or with IPSC-time cross-power spectral density (CPSD) averaged throughout the photostimulation protocol and across 40–150 Hz (C) (linear regression, slope not significantly different from 0: p=0.51, t3 = –0.74).
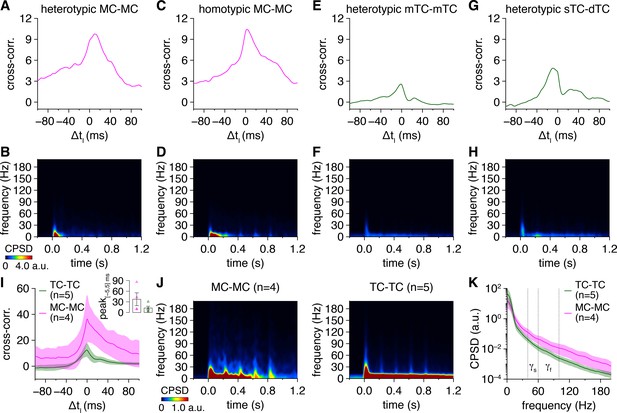
Gamma-frequency synchronization of inhibitory currents is weak among both mitral cells (MCs) and tufted cells (TCs).
(A) Trial-averaged cross-correlogram of inhibitory currents recorded throughout the photostimulation protocol in the MC pair in Figure 6A. (B) Trial-averaged cross-power spectral density (CPSD) spectrogram of inhibitory currents in the MC pair in Figure 6A following photostimulation onset at 0.0 s. (C–H) Same as (A, B) for the MC pair and TC pairs in Figure 6D, G and J. (I) Mean inhibitory current cross-correlograms of TC pairs and MC pairs. Inset: cross-correlogram peaks within |ΔtI| ≤ 5 ms tended to be higher across MC than TC pairs (two-sample t-test: p=0.19, t7 = 1.5). (J) CPSD spectrograms of inhibitory currents averaged across all MC pairs (left) and TC pairs (right). Color is scaled to saturate theta-frequency components, which failed to reveal any gamma-frequency synchronous patterning. Color is differentially scaled between (B, D, F, H, J) similar to the differential scaling between individual CPSD spectrograms and population-average CPSD spectrograms in other figures. (K) Time-independent CPSD of inhibitory currents recorded in MC pairs and TC pairs throughout the photostimulation protocol, revealing no obvious gamma-frequency synchrony.
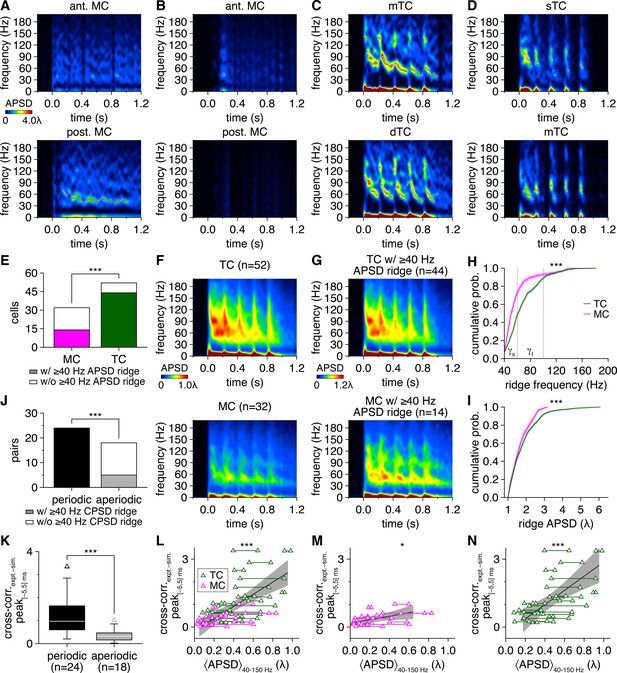
Greater oscillatory behavior among tufted cells (TCs) than mitral cells (MCs) promotes gamma-frequency spike-time synchrony.
(A) Trial-averaged spike-time auto-power spectral density (APSD) spectrogram from the MCs in Figure 1A. Continuous epochs (ΔHz/ms < 150) of high APSD reflecting robust periodic firing are defined as ridges and demarcated with white circles. Color is scaled by multiples of the ridge threshold (λ). (B–D) Same as (A) for the MCs and TCs in Figure 1D, G and J. (E) More TCs than MCs exhibited spike-time APSD ridges (chi-squared test: p=8.3 × 10–5, χ2 = 15.5). (F) Spike-time APSD spectrograms averaged across all TCs (upper) and MCs (lower). (G) Spike-time APSD spectrograms averaged across all TCs (upper) and MCs (lower) exhibiting APSD ridges. (H, I) Cumulative distributions of frequencies (H) and APSD (I) across all spike-time APSD ridges. TCs exhibited faster (H) (two-sample Kolmogorov–Smirnov test: p=2.9 × 10–49) and more precise (N) (two-sample Kolmogorov–Smirnov test: p=2.5 × 10–9) gamma-frequency periodic firing than MCs. Shading denotes 95%confidence intervals. (J) Periodically firing pairs (comprised of cells with ≥1 APSD ridge) were substantially more likely to exhibit periodic spike-time synchrony (i.e., ≥ 1 spike-time cross-power spectral density [CPSD] ridge) than aperiodically firing pairs (chi-squared test: p=5.4 × 10–7, χ2 = 25.1). (K) Spike-time cross-correlogram peaks within |Δtspike| ≤ 5 ms were higher among periodically firing than aperiodically firing pairs (Wilcoxon rank-sum test: p=7.0 × 10–5). (L–N) Periodicity in firing (i.e., spike-time APSD averaged across 40–150 Hz and throughout the photostimulation protocol), averaged across cells of each pair, positively correlated with spike-time synchrony independent of periodicity (i.e., spike-time cross-correlogram central peak heights) among all MC pairs and TC pairs combined (L) (linear regression, slope significantly different from 0: p=6.4 × 10–7, t40 = 5.9; R2 = 0.47), among MC pairs alone (M) (linear regression, slope significantly different from 0: p=0.040, t14 = 2.3; R2 = 0.27), and among TC pairs alone (N) (linear regression, slope significantly different from 0: p=1.7 × 10–4, t24 = 4.5; R2 = 0.45). Shading denotes 95%confidence interval.
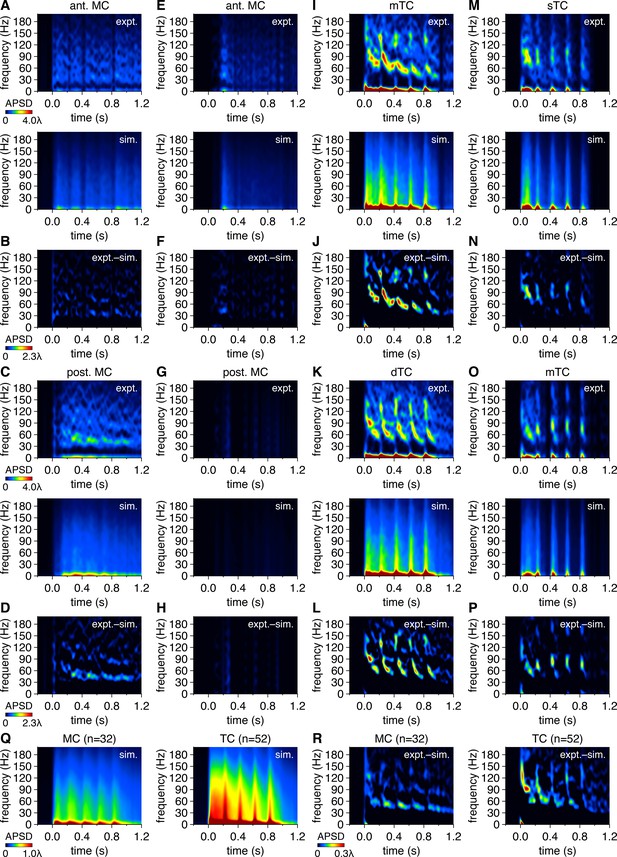
Mitral cells (MCs) and tufted cells (TCs) exhibit greater gamma-frequency spike-time periodicity than spike times simulated from rate-matched independent Poisson processes.
(A) Trial-averaged experimental spike-time auto-power spectral density (APSD) spectrogram from the anterior MC in Figure 1A following photostimulation onset at 0.0 s (upper), and equivalent trial-averaged APSD spectrogram of spike times simulated across 500 trials from a rate-matched Poisson process (lower). Scaling is identical to Figure 7A. (B) Subtraction of simulated spike-time APSD spectrogram from experimental spike-time APSD spectrogram. Color is scaled from 0 to multiples of λ to visualize periodicity within experimental spike-times exceeding that within simulated spike times. (C, D) Same as (A, B) for the posterior MC in Figure 1A. (E–P) Same as (A–D) for the MCs and TCs in Figure 1D, G and J. (Q) Simulated spike-time APSD spectrograms averaged across all MCs (left) and TCs (right). Color is scaled identical to Figure 7F. (R) Subtraction of simulated spike-time APSD spectrograms from experimental spike-time APSD spectrograms, averaged across all MCs (left) and TCs (right), revealing greater gamma-frequency APSD among experimental than simulated spike times.
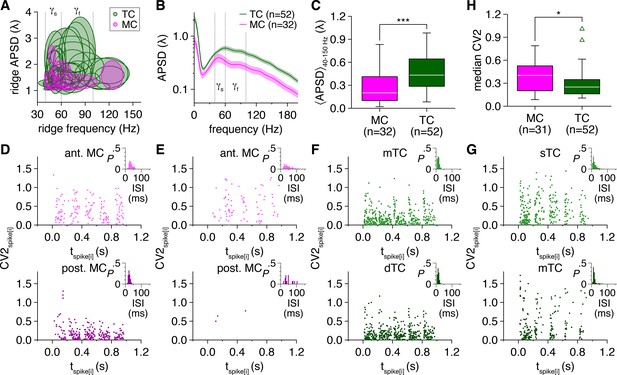
Tufted cells (TCs) exhibit greater oscillatory behavior than mitral cells (MCs) on both a per-cell basis and independent of ridge-based or spectral analyses.
(A) Cellwise distribution of ridge auto-power spectral density (APSD) vs. frequency. Ellipses denote mean ± standard deviation of APSD and frequencies across all ridges detected in each cell. (B) Time-independent spike-time APSD of MCs and TCs recorded throughout the photostimulation protocol. (C) TCs exhibited greater spike-time APSD averaged across 40–150 Hz and throughout the photostimulation protocol than MCs (Wilcoxon rank-sum test: p=2.5 × 10–4). (D) Normalized interspike interval (ISI) variability (spanning spike-times [i–1,i+1]) (i.e., CV2) recorded within each photostimulation cycle in the MCs in Figure 1A. Insets: corresponding ISI probability distributions. (E–G) Same as (D) for the MCs and TCs in Figure 1D, G and J. (H) TC firing exhibited lower median CV2 values throughout the photostimulation protocol than MC firing (Wilcoxon rank-sum test: p=0.021).
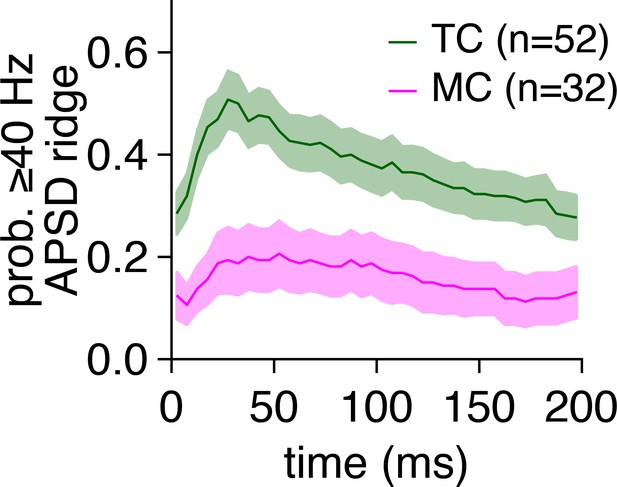
Greater periodicity of tufted cell (TC) than mitral cell (MC) firing persists throughout the average 5 Hz photostimulation cycle.
The probability of robust spike-time periodicity reflected in spike-time auto-power spectral density (APSD) ridges was consistently higher among TCs than MCs throughout the average photostimulation cycle.
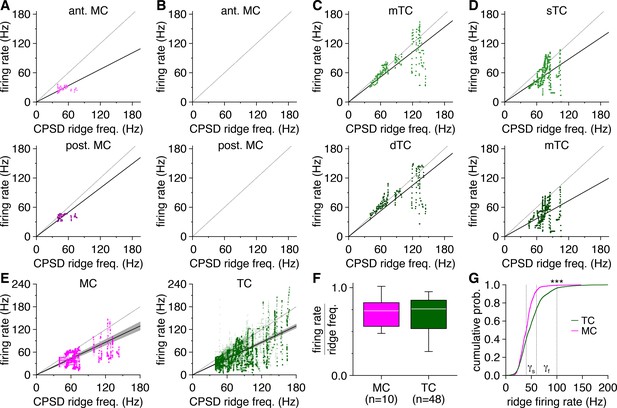
Mitral cell (MC) pairs and tufted cell (TC) pairs exhibit gamma-frequency spike-time synchrony specifically when firing at gamma frequencies.
(A) Instantaneous firing rate of the MCs in Figure 1A plotted against the simultaneous frequency of periodic spike-time synchrony during each spike-time cross-power spectral density (CPSD) ridge. Dashed line: unity. Solid line: mean firing rate to CPSD ridge frequency ratio. (B–D) Same as (A) for the MCs and TCs in Figure 1D, G and J. (E) Instantaneous firing rate plotted against the simultaneous frequency of periodic spike-time synchrony across all MCs (left) and TCs (right). Darker coloring denotes overlapping data points. Solid lines: mean firing rate to CPSD ridge frequency ratio, averaged across cells. (F) Instantaneous firing rates relative to the simultaneous frequency of periodic spike-time synchrony were comparable among MCs and TCs (Wilcoxon rank-sum test: p=0.89) and approached unity in both cells, consistent with synchronization of periodic firing across the majority of spikes fired during an epoch. (G) Cumulative distribution of instantaneous TC and MC firing rates recorded during spike-time CPSD ridges. TCs exhibiting gamma-frequency spike-time synchrony fired at higher rates than MCs exhibiting gamma-frequency spike-time synchrony (two-sample Kolmogorov–Smirnov test: p=0).
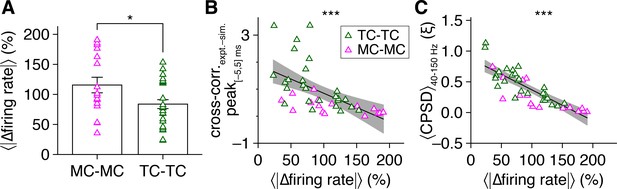
Firing rate differences attenuate periodic spike-time synchrony.
(A) Tufted cell (TC) pairs exhibited smaller mean firing rate differences, averaged throughout the photostimulation protocol and relative to the mean firing rate of the pair, than mitral cell (MC) pairs (two-sample t-test: p=0.027, t40 = 2.3). (B, C) Mean firing rate difference, averaged throughout the photostimulation protocol and relative to the mean firing rate of the pair, negatively correlated with spike-time cross-correlogram peaks within |Δtspike| ≤ 5 ms (as in Figure 1M) (B) (linear regression, slope significantly different from 0: p=1.1 × 10–4, t40 = –4.3; R2 = 0.31) and with spike-time cross-power spectral density (CPSD) averaged throughout the photostimulation protocol and across 40–150 Hz (C) (linear regression, slope significantly different from 0: p=2.5 × 10–11, t40 = –9.1; R2 = 0.68).

Intrinsic resonance is more widespread and better entrains firing among tufted cells (TCs) than mitral cells (MCs).
(A, B) Example recording of a resonant MC. Reconstructed morphology (A) and representative response to depolarizing step current injection (B) (light trace: original membrane potential; dark trace: subthreshold membrane potential; gray line: least squares estimate-sinusoid fit to subthreshold oscillation [STO]). (C) Spectrogram showing continuous Morlet wavelet transform (CWT) of subthreshold membrane potential from the MC response in (B). CWT ridges are confirmed as STOs following post-hoc visual inspection of the underlying membrane potential and demarcated with white circles. Colored circles: ridge maxima, defining frequency of confirmed STO. (D) Post-STO spike phases for the MC in (A), showing a non-uniform distribution at phases just prior to the STO peak (Rayleigh’s test: pBH = 2.0 × 10–8). Dark-colored bars: spike phases from the example response in (B). Arrow: median spike phase. (E) Instantaneous firing rates were consistently slower than the preceding STO frequency in the MC in (A). Dashed line: unity. Solid line: mean firing rate to STO frequency ratio. Filled symbols: STOs detected in the example response in (B). (F–J) Same as (A–E) for a non-resonant MC. (K–O) Same as (A–E) for a resonant mTC. Post-STO spike phases were non-uniformly distributed at phases just prior to the STO peak (N) (Rayleigh’s test: pBH = 1.0 × 10–6). (P–T) Same as (A–E) for a second resonant mTC. Post-STO spike phases were non-uniformly distributed at phases just prior to the STO peak (S) (Rayleigh’s test: pBH = 3.2 × 10–5). (U) More TCs than MCs were resonant (chi-squared test: p=0.029, χ2 = 4.8). (V) Post-STO spikes were significantly phase-locked (i.e., non-uniformly distributed) among comparable proportions of resonant MCs and TCs (chi-squared test: p=0.54, χ2 = 0.37), encompassing the vast majority of resonant cells. Inset: resonant MCs and TCs with phase-locked firing exhibited comparable median spike phases (Watson–Williams test: p=0.87). (W) Instantaneous firing rate vs. preceding STO frequency across all resonant MCs (left) and TCs (right). Solid lines: mean firing rate to STO frequency ratio, averaged across cells. Inset: TCs exhibited closer entrainment of firing rate to preceding STO frequency than MCs (two-sample t-test: p=0.020, t14 = 2.6).
Additional files
-
Transparent reporting form
- https://cdn.elifesciences.org/articles/74213/elife-74213-transrepform1-v2.pdf
-
Source data 1
Spreadsheet of source data for all figures.
- https://cdn.elifesciences.org/articles/74213/elife-74213-supp1-v2.xlsx