Connexins evolved after early chordates lost innexin diversity
Figures
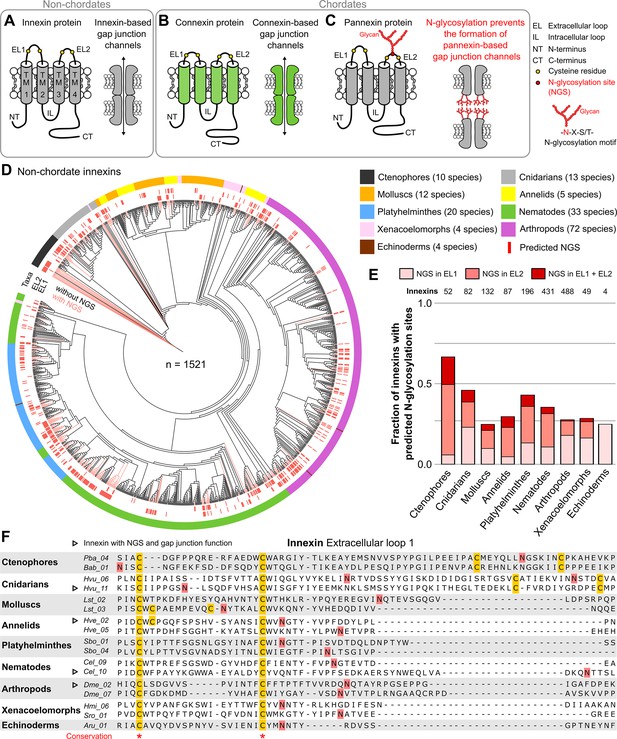
Innexins with N-linked glycosylation sites are widespread among non-chordate animals.
(A) Innexins and (B) connexins are integral membrane proteins that share a common membrane topology with four hydrophobic transmembrane domains (TM) linked by one intracellular (IL) and two extracellular loops (ELs). Connexin and innexin proteins can assemble to form a hemichannel. The hemichannels of two neighboring cells can form intercellular gap junction channels that are stabilized by disulfide bonds between cysteine residues in their ELs (Dahl et al., 1991) (yellow circles). (C) The innexin homologs of vertebrates, called pannexins, are glycoproteins that contain N-linked glycosylation consensus sites (NGS) within their ELs. The attachment of glycans to NGSs block the disulfide bond formation and hence prevents the proper docking of the hemichannels and the formation of pannexin-based gap junctions (Ruan et al., 2020). (D) Maximum likelihood phylogeny of innexin proteins from 174 non-chordate species (n=1521 innexins). Black and red branches represent innexins without and with NGSs in the ELs, respectively. The red bars in the inner circle indicate whether EL1 or EL2 contains the NGS. The colors of the outer circle represent the phylum classification of the innexins. (E) Fraction of innexins with NGSs in their ELs in the taxonomic groups shown in (D). (F) Representative multiple sequence alignment of the EL1 of innexins. The alignment contains selected innexin sequences of representative species of each taxonomic group. All identified non-chordate innexins as well as the complete alignment are provided as a supplementary files (Figure 1—source data 2 and Figure 1—source data 4). Conserved residues are highlighted (*, absolutely conserved). The cysteine residues (yellow) and the NGSs (red) in each EL1 are colored (note that some innexins have more than two cysteines). The identified NGSs in EL1 and EL2 of the innexins of all other non-chordate species are shown in Figure 1—source data 1. Arrowheads mark innexins with NGSs that have been shown to form functional gap junction channels (Figure 1—source data 3).
-
Figure 1—source data 1
A list of the identified N-glycosylation sites (NGSs) in the extracellular loops of innexins in non-chordate species.
- https://cdn.elifesciences.org/articles/74422/elife-74422-fig1-data1-v1.zip
-
Figure 1—source data 2
Multiple sequence alignment of all non-chordate innexins.
- https://cdn.elifesciences.org/articles/74422/elife-74422-fig1-data2-v1.zip
-
Figure 1—source data 3
Extracellular N-glycosylation sites (NGSs) in non-chordate innexins with confirmed gap junction function.
The identified NGSs have a high potential score (see Materials and methods) and the amino acid distribution around the N-glycosylation motif shows no indications for an unoccupancy of the predicted NGSs (Petrescu, 2003).
- https://cdn.elifesciences.org/articles/74422/elife-74422-fig1-data3-v1.zip
-
Figure 1—source data 4
Protein sequences of the 1521 non-chordate innexins identified in this study.
- https://cdn.elifesciences.org/articles/74422/elife-74422-fig1-data4-v1.zip
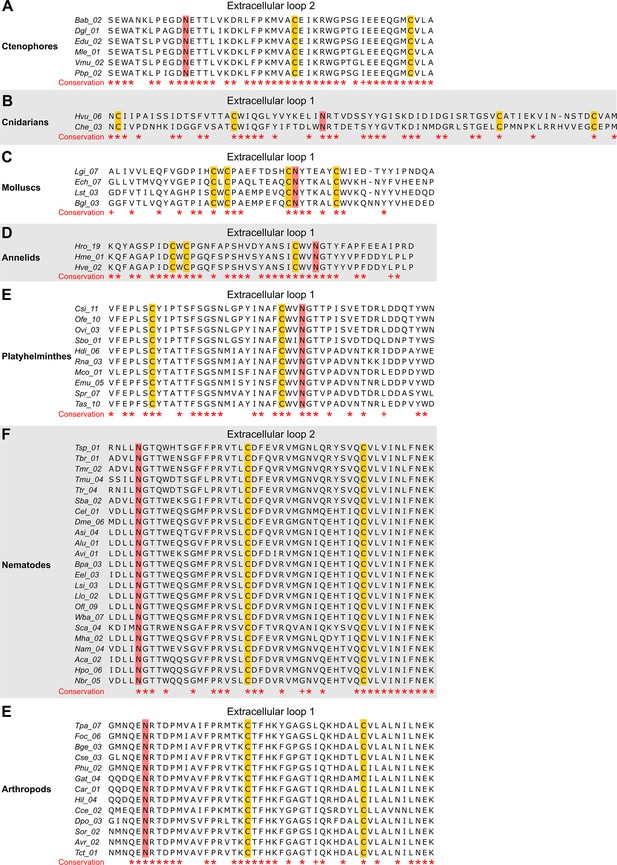
The N-linked glycosylation sites (NGSs) in the extracellular loops (ELs) of innexins are conserved in some orthologs.
The multiple sequence alignments show innexin orthologs in (A) ctenophores, (B) cnidarians, (C) molluscs, (D) annelids, (E) platyhelminthes, (F) nematodes, and (E) arthropods that have highly conserved NGSs in their EL1 or EL2. Note that we did not find any extracellular NGS that was conserved in all species within a phylum. Conserved residues are highlighted (*, absolutely conserved; +, physicochemical properties are conserved). The cysteine residues (yellow) and the NGSs (red) in each EL are colored. The identified NGSs in EL1 and EL2 of the innexins of all other non-chordate species are shown in Figure 1—source data 1.
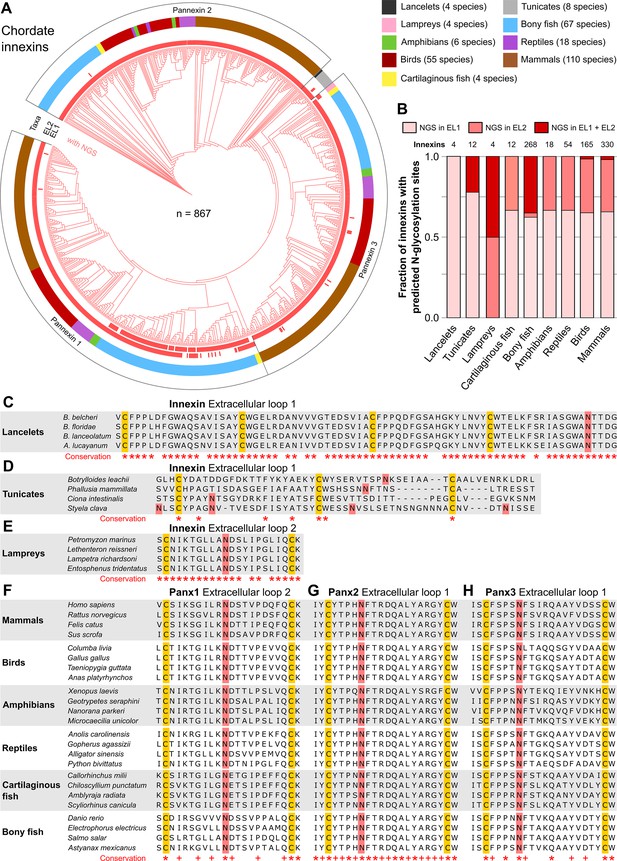
N-linked glycosylation of innexins is highly conserved in chordate animals.
(A) Maximum likelihood phylogeny of innexin proteins from 276 non-chordate species (n=867 innexins). Red branches represent innexins with N-glycosylation consensus sites (NGS) in the extracellular loops (ELs), respectively. Note that each innexin in every chordate species has at least one NGS. The red bars in the inner circle indicate whether extracellular loop 1 or 2 (EL1 and EL2) contains the NGS. The colors of the outer circle represent the phylum classification of the innexins. (B) Fraction of innexins with NGSs in their ELs in the taxonomic groups shown in (A). Multiple sequence alignments of the EL1 of innexins in (C) lancelets and (D) tunicates. Note that lancelets and tunicates have more than two cysteine residues in EL1, like cnidarians and ctenophores. (E) Multiple sequence alignments of the EL2 of innexins in lampreys. Representative multiple sequence alignments of the highly conserved EL2 of (F) Pannexin 1 and the EL1 of (G) Pannexin 2 and (H) Pannexin 3 in vertebrates. Residues that are conserved in all innexins in each alignment are highlighted (*, absolutely conserved; +, physicochemical properties are conserved). Note that the pannexin alignments only contain the sequences of some representative species of each taxonomic group. All identified chordate innexins as well as the complete alignment are provided as supplementary files (Figure 2—source data 2 and Figure 2—source data 3). The cysteine residues (yellow) and the NGSs (red) in each EL are colored. The identified NGSs in EL1 and EL2 of the innexins of all other chordate species are shown in Figure 2—source data 1.
-
Figure 2—source data 1
A list of the identified N-glycosylation sites (NGS) in the extracellular loops of innexins in chordate species.
- https://cdn.elifesciences.org/articles/74422/elife-74422-fig2-data1-v1.zip
-
Figure 2—source data 2
Multiple sequence alignment of all chordate innexins.
- https://cdn.elifesciences.org/articles/74422/elife-74422-fig2-data2-v1.zip
-
Figure 2—source data 3
Protein sequences of the 867 chordate innexins identified in this study.
- https://cdn.elifesciences.org/articles/74422/elife-74422-fig2-data3-v1.zip
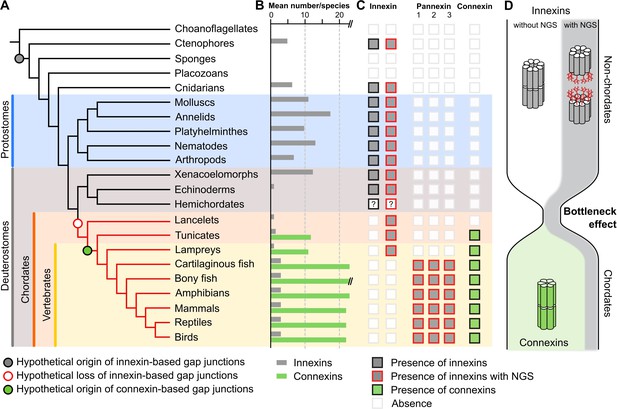
A new evolutionary scenario to explain the origin and exclusive use of connexin-based gap junctions in vertebrates.
(A) Simplified phylogenetic tree to visualize the hypothetical loss of innexin-based gap junctions and the origin of connexin-based gap junctions. Lineages that only possess innexins with extracellular NGSs are indicated by a red branch color. (B) Mean number of different innexins and connexins per species in each of the different phyla. The Y-axis was cut for clarity (bony fish have 39–46 connexins due to genome duplications; Mikalsen et al., 2021). In hemichordates, we only found fragmented innexin-like sequences. (C) Occurrence of innexins and connexins in different metazoans. Innexins with an N-glycosylation consensus site (NGS) in their extracellular domains are present in chordates and non-chordates. Note that lancelets only possess one highly conserved innexin with one NGS and lack connexins. (D) A hypothetical evolutionary scenario in which the connexin-based gap junctions evolved after innexin diversity was lost during a bottleneck event in the early chordate evolution.