A transcriptional constraint mechanism limits the homeostatic response to activity deprivation in mammalian neocortex
Figures
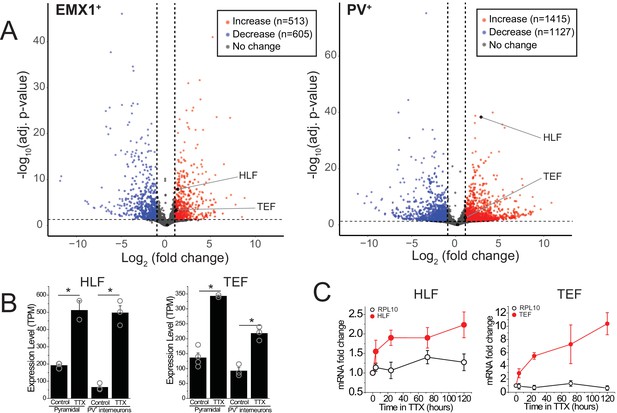
RNA-sequencing identifies transcripts affected by activity deprivation including the PARbZIP family of transcription factors.
(A) Volcano plots of bulk RNA-seq from sorted fluorescently labeled pyramidal cells (left) and PV+ interneurons (right). Dashed lines indicate a fold change of 2 and adjusted p value of 0.05. Differential expression analysis revealed that TTX resulted in upregulation (513 in pyramidal, 1415 in PV+ interneurons) as well as downregulation (605 in pyramidal, 1127 in PV+ interneurons) of genes, among which Hlf and Tef were identified as upregulated with activity block in both excitatory and inhibitory neurons. Statistical analysis (Wald test followed by Benjamini-Hochberg correction) reveals that Hlf and Tef are significantly upregulated in both pyramidal cells and PV+ interneurons, while Dbp and Nfil3 are not significantly altered in either cell type. (B) Bar graphs displaying transcript per million (TPM) values of Hlf (left panel) and Tef (right panel) in pyramidal cells (left) and interneurons (right) in control and 5-day TTX treated slices. Bars are mean values +/- SEM, open symbols are individual experiments. (C). Quantitative real-time PCR analysis of Hlf and Tef expression in whole cortex lysates following a time course of activity deprivation (n = 3–4 slices per time point; error bars are SEM). Two-way ANOVA reveals significant differences between Hlf/Tef and RPL10 (p<0.05).
-
Figure 1—source data 1
qPCR Data.
- https://cdn.elifesciences.org/articles/74899/elife-74899-fig1-data1-v2.xlsx
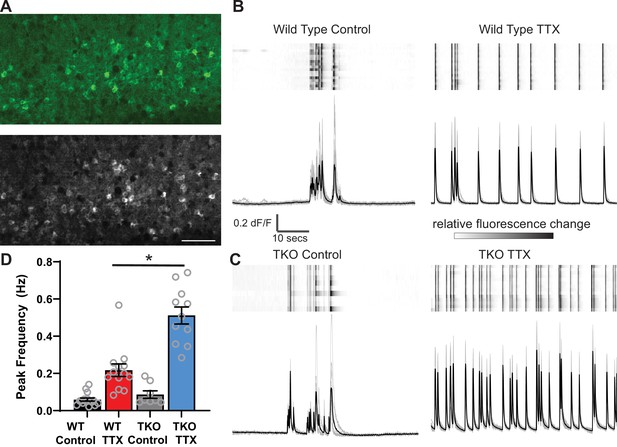
Calcium imaging reveals exaggerated response to activity deprivation in Hlf−/−/Dbp−/−/Tef−/− triple knockout (TKO) slices.
(A). A confocal image of GCaMP6f fluorescence during an upstate in wild-type (WT) control slice (top panel) and a standard deviation projection of the same field of view of calcium signal during 1 min of recording (bottom panel). Scale bar = 50 µm. ROIs were manually selected around active cells identified by high standard deviation values. (B, C) GCaMP6f fluorescence heat map of selected ROIs (each row is one cell) during 1 min of recording (top panels) also shown as overlapping traces (gray, bottom panels) and an average fluorescence trace (black, bottom panels) in WT (B) and TKO slices (C) from control (left) and TTX-treated conditions (right). Traces show absolute dF/F, heatmap scale is normalized for each cell so that the full intensity range corresponds to the range of dF/F. (D) Quantification of peak frequency in GCaMP6f fluorescence traces. N = 16 slices for WT Control, N = 13 WT TTX, N = 8 TKO Control, and N = 11 TKO TTX. Error bars indicate SEM. TTX treatment significantly increases peak frequency in WT slices with significant (p<0.0001) interaction between treatment and genotype. The increase is more dramatic in the TKO slices. Two-way ANOVA with Tukey’s correction for multiple comparisons to test statistical significance between conditions *p≤0.0001.
-
Figure 2—source data 1
Calcium imaging analysis summary, 2D TTX; WT, TKO.
- https://cdn.elifesciences.org/articles/74899/elife-74899-fig2-data1-v2.xlsx
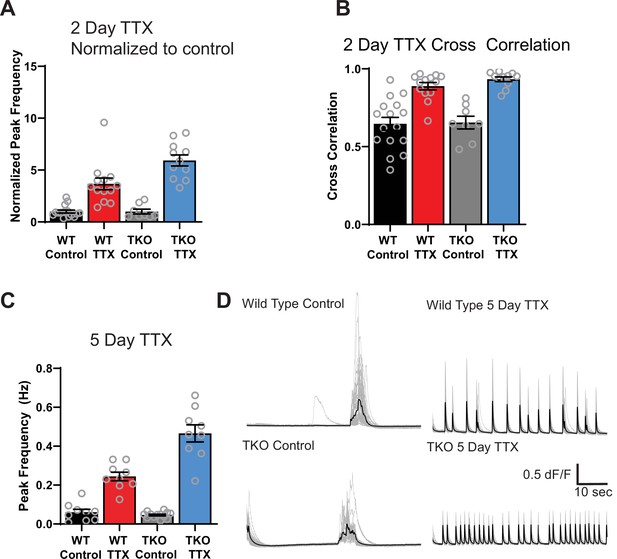
Ca2+ activity in mutant slices is similarly exaggerated after 2 and 5 days of activity withdrawal.
(A) Quantification of peak frequency (from Figure 1D) normalized to control in WT and TKO slices after 2 days of activity deprivation. (B) Quantification of peak synchrony values in each of the conditions in (A). Open symbols are individual slice cultures, filled bars are mean ± SEM. Synchrony values in WT condition are close to 1 and do not show further increase in the TKO slides. (C) Quantification of peak frequency after 5 days of activity deprivation and traces from representative slices (D) of the genotypes in (C). Increasing TTX incubation time to 5 days of TTX does not change the TKO phenotype. Peak frequency increased in WT (from 0.06 ± 0.01 to 0.24 ± 0.02) and this response is exaggerated in the TKO (from 0.05 ± 0.005 to 0.47 ± 0.04; a 2.5-fold greater increase than in WT). As at 2 days, two-way ANOVA revealed significant main effects for TTX (p < 0.0001) and genotype (p < 0.0001) and a significant interaction between treatment and genotype (p < 0.0001) and post hoc test (Tukey) revealed a significant difference between WT TTX and TKO TTX (p < 0.0001), but no difference between WT and TKO control activity (p = 0.97). Also, as at 2 days, synchrony increased dramatically in the presence of TTX in both genotypes (to 0.96 and 0.93) but this was not significantly different and there was also no significant difference in the values at baseline in the two genotypes (0.54 and 0.58). N = 9 for WT control, N = 9 for WT TTX, N = 13 for TKO control, and N = 9 for TKO TTX. TKO, triple knockout; WT, wild-type.
-
Figure 2—figure supplement 1—source data 1
Calcium imaging analysis summary, 5D TTX; WT, TKO.
- https://cdn.elifesciences.org/articles/74899/elife-74899-fig2-figsupp1-data1-v2.xlsx
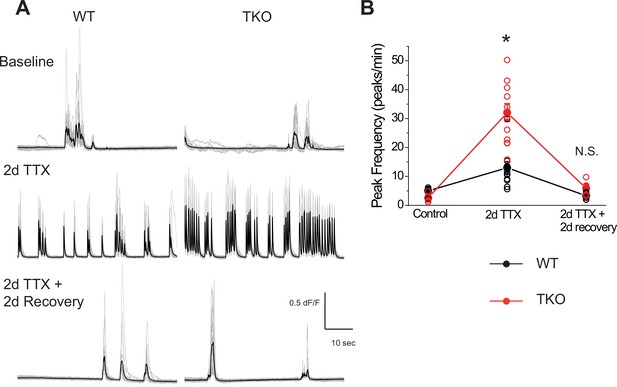
Ca2+ activity recovers to baseline levels following washout of TTX in both wild-type and mutant slices.
(A) Representative GCaMP6f fluorescence intensity over 1 min of recording in WT (left panels) and TKO slices (right panels) during control (top row), immediately after TTX washout (middle row) and after 2 days of recovery following TTX washout (bottom row). (B) Quantification of peak frequency in each of the conditions in (A). Open symbols are individual slice cultures, filled symbols are mean ± SEM. Two-way ANOVA revealed significant main effect for drug treatment (p < 0.0001) and genotype (p = 0.0009) and a significant interaction between treatment and genotype (p = 0.017). Although TTX incubation has a much stronger effect on spontaneous Ca2+ activity immediately after washout in mutant slices (p < 0.0001, post hoc Tukey test), the activity returns to baseline in both genotypes (p = 0.99, post hoc Tukey test). N = 5 for WT age-matched control, N = 3 for TKO age-matched control, N = 11 for WT TTX, N = 11 for TKO TTX, N = 5 for WT recovery, and N = 4 for TTX recovery. TKO, triple knockout; WT, wild-type.
-
Figure 2—figure supplement 2—source data 1
Calcium imaging analysis summary, recovery.
- https://cdn.elifesciences.org/articles/74899/elife-74899-fig2-figsupp2-data1-v2.xlsx
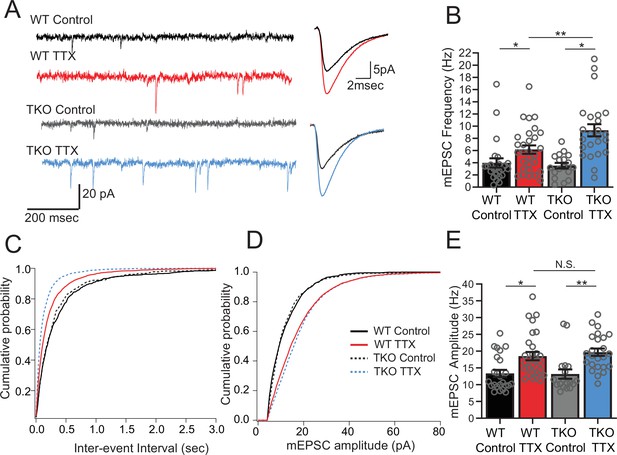
Frequency but not amplitude of excitatory synaptic currents is disproportionally upregulated in TTX-treated TKO slices.
(A) Representative traces of mEPSC recordings from WT control (black), WT TTX-treated (red), TKO control (gray), and TKO TTX-treated (blue) slices. Right panel, average mEPSC waveforms for the same conditions. Both the frequency and the amplitude is increased in TTX-treated slices; however, the increase in frequency is more dramatic in TKO slices. (B) Quantification of mEPSC frequency for each condition. Colored bars with error bars are mean ± SEM, open circles are individual cells; N = 24 for WT control, N = 29 for WT TTX, N = 18 for TKO control, and N = 24 for TKO TTX. Two-way ANOVA revealed significant main effect of TTX treatment (p < 0.0001), genotype alone (p=0.030) and interaction between treatment and genotype (p = 0.041). Post hoc Tukey test revealed enhanced effect of TTX on mEPSC frequency in TKO cells compared to WT (p = 0.009, **). (C) Cumulative probability histogram for mEPSC inter-event intervals in each condition. (D) Cumulative probability histogram for mEPSC amplitudes in each condition. (E) mEPSC amplitudes for each condition. Two-way ANOVA revealed significant main effect for drug treatment (p < 0.0001) but not genotype (p = 0.74) or interaction between treatment and genotype (p = 0.55). TTX treatment enhanced mEPSC amplitude in both WT (p = 0.012, Tukey post hoc test, *) and TKO (p = 0.0035, Tukey post hoc test, **) to the same extent (p = 0.89, WT TTX compared to TKO TTX, Tukey post hoc test, N.S.). TKO, triple knockout; WT, wild-type.
-
Figure 3—source data 1
mEPSC amplitude and frequency.
- https://cdn.elifesciences.org/articles/74899/elife-74899-fig3-data1-v2.xlsx
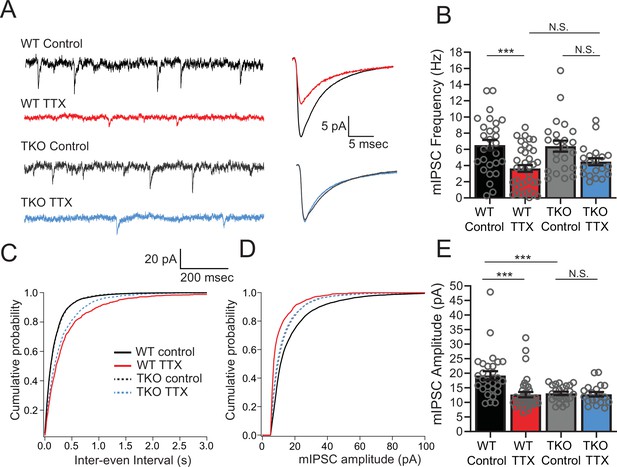
Inhibitory synaptic currents are not affected by the Hlf−/−/Dbp−/−/Tef−/− mutation in the TTX condition.
(A) Representative traces of mIPSC recordings from WT control (black), WT TTX-treated (red), TKO control (gray), and TKO TTX-treated (blue) slices. Right panel, average mIPSC waveforms for the same conditions. TTX treatment results in a diminished effect on mIPSC frequency in TKO and does not further decrease the amplitude of mIPSCs in TKO slices. (B) Quantification of mIPSC frequency. Colored bars with error bars are mean ± SEM, open circles are individual cells. Two-way ANOVA revealed a significant main effect of TTX treatment (p < 0.0001) but not genotype (p = 0.54). There was no significant interaction between treatment and genotype (p = 0.40), N = 28 for WT control, N = 37 for WT TTX, N = 23 for TKO control, and N = 20 for TKO TTX. TTX treatment decreased mIPSC frequency in WT (p = 0.0007, post hoc Tukey test) but not in TKO cells (p = 0.14, post hoc Tukey test). mIPSC frequency in TTX-treated cells is not different in TKO slices compared to WT (p = 0.72). The normalized change in frequency (TTX normalized to Control) was 0.55 in WT and 0.70 in TKO. We performed a power analysis that revealed that for the observed effect size and variance a sample of 68 neurons per condition would be required for significance. In (B), (C), and (E), cumulative probability histogram for mIPSC inter-event intervals in each condition. (D) Cumulative probability histogram for mIPSC amplitudes in each condition. (E) Quantification of mIPSC amplitudes for the same conditions as in (B). Two-way ANOVA revealed significant main effect of TTX (p = 0.002), and genotype (p = 0.0067), and a significant interaction between treatment and genotype (p = 0.005). TTX treatment decreased mIPSC amplitude in WT (p < 0.0001, Tukey post hoc test, ***), but not in TKO cells (p = 0.997, Tukey post hoc test, N.S.) presumably due to significantly lower baseline mIPSC amplitude in TKO slices compared to WT (p = 0008, post hoc Tukey test, ***). TKO, triple knockout; WT, wild-type.
-
Figure 4—source data 1
mIPSC amplitude and frequency.
- https://cdn.elifesciences.org/articles/74899/elife-74899-fig4-data1-v2.xlsx
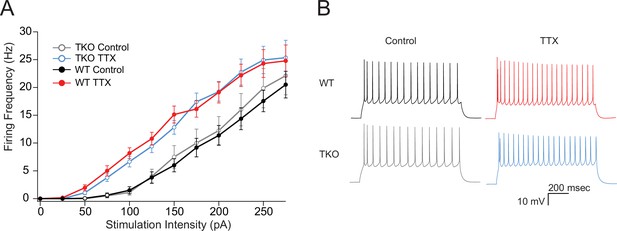
The effects of TTX on intrinsic excitability are not altered in TKO pyramidal neurons.
(A) Comparisons of frequency-current relationships for layer five pyramidal neurons in control (black, closed circles) and TTX-treated (red, closed circles) WT slices, and in control (gray, open circles), and TTX-treated (blue, open circles) TKO slices, N = 27 cells for WT TTX, N = 16 for WT control, N = 8 for TKO control, and N = 17 for TKO TTX; error bars are SEM. Two-day TTX treatment increases intrinsic excitability in both WT and TKO slice cultures to a similar extent. A three-way mixed ANOVA with between subjects factors of treatment and genotype, and a within subjects factor of current level, revealed significant main effects for TTX treatment (F(1,60) = 18.1, p = 7.3e−05) and current level (F(11,660) = 2.3e−190) but not for genotype (F(1,60) = 0.06, p = 0.94). There was a significant interaction between treatment and current level (p = 0.06) but not for interactions of genotype with current level (p = 0.93) or with both current level and treatment (p = 0.99). (B) Example traces of a train of action potentials from a pyramidal neuron in response to a 175 pA 0.5 s depolarizing current injection in control (left), TTX-treated (right), WT (top), or TKO (bottom) slices. TKO, triple knockout; WT, wild-type.
-
Figure 5—source data 1
Frequency-Current curves.
- https://cdn.elifesciences.org/articles/74899/elife-74899-fig5-data1-v2.xlsx
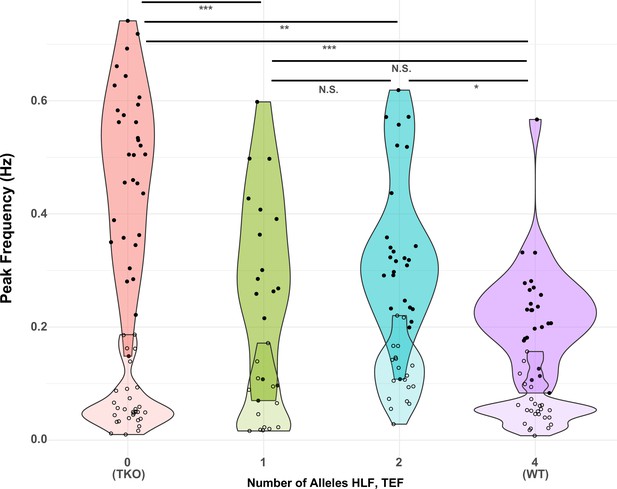
Presence of one allele of Hlf or Tef largely restores the WT response to activity deprivation.
Violin plots show the distribution of peak frequencies measured from TTX-treated (upper, darker colors, filled symbols) and control (lower, lighter colors, open symbols) slice cultures made from animals carrying 0, 1, 2, or 4 alleles of the PARbZIP TFs Hlf and Tef. Data are those used in Table 1 with the X-axis corresponding to models 6 (TTX) and 12 (Control). Horizontal positions of individual data points are jittered to prevent overplotting. Horizontal lines at top indicate results of post hoc t tests between levels for the TTX data with Tukey correction for multiple comparisons. *adj. p < 0.05, **adj. p < 0.01, ***adj. p < 0.001, N.S. – not significant, adj. p > 0.05. No post hoc testing was performed for the Control data since the fit of model 12 was not significant. WT, wild-type.
-
Figure 6—source data 1
Calcium imaging analysis summary, 2D, 5D TTX; all genotypes.
- https://cdn.elifesciences.org/articles/74899/elife-74899-fig6-data1-v2.xlsx
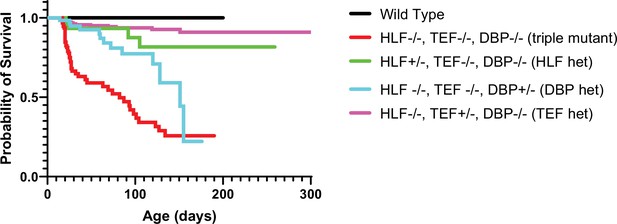
Presence of one allele of a PARbZIP TF improves survival relative to TKO animals.
TKO mice have dramatically decreased survival (red line) compared to wild-type (WT) mice (black line). Presence of one copy of either Hlf (green line) or Tef (purple line) prevents premature death and the survival curves are not statistically different from WT, p = 0.12 and p = 0.56, respectively, Log-rank test with Bonferroni correction for multiple comparisons. DBP heterozygous mice (blue line) also have improved survival over TKO mice (p = 0.003) but are still statistically more likely to die prematurely than WT mice (p = 0.0003). N = 59 for DBP heterozygous, N = 96 for TKO, N = 36 for HLF heterozygous, N = 23 for WT, and N = 179 for TEF heterozygous. TKO, triple knockout.
-
Figure 7—source data 1
Survival data by genotype.
- https://cdn.elifesciences.org/articles/74899/elife-74899-fig7-data1-v2.csv
-
Figure 7—source data 2
Statistical analysis of survival data.
- https://cdn.elifesciences.org/articles/74899/elife-74899-fig7-data2-v2.pdf
Tables
Multivariate linear models of the dependence of calcium peak frequency (PF) on genotype.
Listed linear models were fit to peak frequencies of calcium transients measured from 87 control and 98 TTX-treated slices each from separate animals that differed in their genotype (null, heterozygote or wild-type) for each of the Hlf, Tef, and Dbp genes. The data (see attached file) includes the 88 experiments shown in Figure 2 and Figure 2—figure supplement 1 (WT and TKO) as well as 97 additional experiments (intermediate genotypes). Regressions were performed using the lm function in R where the variables Hlf, Tef, and Dbp represent the numbers of alleles of each gene (0, 1, or 2) and the variable (Hlf + Tef) represented the sum of the number of Hlf and Tef alleles. The column p > F reports the significance of each model assessed from an F test. For models that accounted for >10% of the variance in the data (adj R2 > 0.1; bold values), the intercept (b0) and coefficients (b1…) are listed, along with the 790 probability that the coefficient is nonzero (t test).
Model (TTX) | Adj R2 | Pr > F | b0 (Pr > t) | b1 (Pr > t) | b2 (Pr > t) | b3 (Pr > t) | |
---|---|---|---|---|---|---|---|
1 | PF = b0 + b1(Hlf) + b2(Tef) + b3(Dbp) | 0.284 | 2E−07 | 0.457 (<2E−16) | –0.055 (0.003) | –0.068 (4E−4) | 0.006 (0.71) |
2 | PF = b0 + b1(Hlf) | 0.198 | 4E−06 | 0.423 (<2E−16) | –0.081 (4E−6) | ||
3 | PF = b0 + b1(Tef) | 0.229 | 4E−07 | 0.437 (<2E−16) | –0.088 (4E−7) | ||
4 | PF = b0 + b1(Dbp) | 0.073 | 0.007 | ||||
5 | PF = b0+b1(Hlf) + b2(Tef) | 0.291 | 3E−08 | 0.458 (<2E−16) | –0.052 (0.003) | –0.066 (2E−4) | |
6 | PF = b0 + b1(Hlf + Tef) | 0.296 | 4E−09 | 0.485 (<2E−16) | –0.059 (4E−9) | ||
Model (Ctrl) | |||||||
7 | PF = b0 + b1(Hlf) + b2(Tef) + b3(Dbp) | 0.044 | 0.08 | ||||
8 | PF = b0 + b1(Hlf) | –0.01 | 0.84 | ||||
9 | PF = b0 + b1(Tef) | 0.005 | 0.52 | ||||
10 | PF = b0 + b1(Dbp) | 0.063 | 0.05 | ||||
11 | PF = b0 + b1(Hlf) + b2(Tef) | –0.01 | 0.62 | ||||
12 | PF = b0 + b1(Hlf + Tef) | –0.01 | 0.80 |
Reagent type (species) or resource | Designation | Source or reference | Identifiers | Additional information |
---|---|---|---|---|
Gene (Mus musculus) | Hlf | MGI | MGI:MGI:96108 | |
Gene (Mm) | Tef | MGI | MGI:MGI:98663 | |
Gene (Mm) | Dbp | MGI | MGI:MGI:94866 | |
Strain, strain background (Mm) | Emx1-ires-Cre | Jackson Labs | JAX:005628 | |
Strain, strain background (Mm) | Pvalb-ires-Cre | Jackson Labs | JAX:017320 | |
Strain, strain background (Mm) | triple knockout (TKO) Hlf−/−/Dbp−/−/Tef−/−; TKO | Jackson Labs | EM:02489 | |
Strain, strain background (Mm) | Ai9 | Jackson Labs | JAX:007909 | |
Cell line (include species here) | ||||
Transfected construct (include species here) | ||||
Biological sample (include species here) | ||||
Antibody | (Include host species and clonality) | (Include dilution) | ||
Recombinant DNA reagent | AAV-GCaMP6f | Addgene | pAAV.Syn.GCaMP6f.WPRE.SV40 (AAV9 capsid serotype) 100837 | |
Sequence-based reagent | RPL10 Forward | This paper | PCR primers | 5′-CACGGCAGAAACGAGACTTT-3′ |
Sequence-based reagent | RPL10 Reverse | This paper | PCR primers | 5′-CACGGACGATCCTATTGTCA-3′ |
Sequence-based reagent | GAPDH (forward) | This paper | PCR primers | 5′-TCAATGAAGGGGTCGTTGAT-3′ |
Sequence-based reagent | GAPDH (reverse) | This paper | PCR primers | 5′-CGTCCCGTAGACAAAATGGT-3′ |
Sequence-based reagent | HLF (forward) | This paper | PCR primers | 5′-CGGTCATGGATCTCAGCAG-3′ |
Sequence-based reagent | HLF (reverse) | This paper | PCR primers | 5′-GTACCTGGATGGTGTCAGGG-3′ |
Sequence-based reagent | TEF (forward) | This paper | PCR primers | 5′-GAGCATTCTTTGCCTTGGTC-3′ |
Sequence-based reagent | TEF (reverse) | This paper | PCR primers | 5′-GGATGGTCTTGTCCCAGATG-3′ |
Peptide, recombinant protein | ||||
Commercial assay or kit | Ovation RNA-seq system | Nugen | ||
Commercial assay or kit | Illumina library quantification kit | KAPA biosystems | ||
Commercial assay or kit | Picopure RNA isolation kit | Life Technologies | ||
Commercial assay or kit | iScript cDNA Synthesis Kit | Bio-Rad | #1708891 | |
Commercial assay or kit | RNA Clean and Concentrator kit | Zymo Research | R1014 | |
Commercial assay or kit | SYBR Green Supermix | Bio-Rad | 1725270 | |
Chemical compound, drug | Tetrototoxin (TTX) | Abcam | ab120055 | |
Chemical compound, drug | DNQX | Sigma-Aldrich | D0540 | |
Chemical compound, drug | APV | R&D Systems | 0106 | |
Chemical compound, drug | Picrotoxin (PTX) | R&D Systems | 1128 | |
Software, algorithm | Calcium Imaging Analysis Code | https://github.com/VH-Lab/vhlab-TwoPhoton-matlab; copy archived at Van Hooser, 2023 | ||
Software, algorithm | MatLab | Mathworks https://www.mathworks.com/products/matlab.html | RRID:SCR_001622 | |
Software, algorithm | IgorPro | WaveMetrics https://www.wavemetrics.com/ | RRID:SCR_000325 | |
Software, algorithm | GraphPad PRISM | GraphPad Software https://www.graphpad.com/ | RRID:SCR_002798 | |
Software, algorithm | STAR | https://github.com/alexdobin/STAR; Dobin, 2023 | ||
Software, algorithm | featureCounts (Rsubread package) | https://bioconductor.org/packages/release/bioc/html/Rsubread.html | ||
Software, algorithm | DESeq2 | https://bioconductor.org/packages/release/bioc/html/DESeq2.html |