Air pollution particles hijack peroxidasin to disrupt immunosurveillance and promote lung cancer
Figures
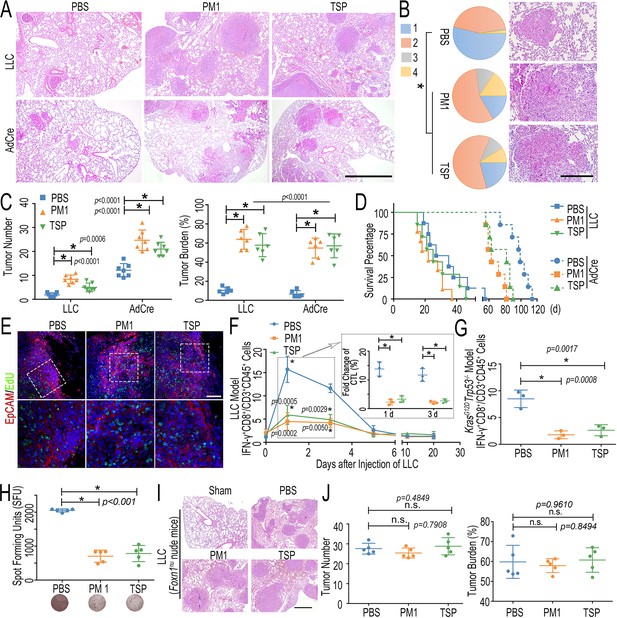
Fine particulate matter (FPM) accelerated lung tumorigenesis by inhibiting cytotoxic T cell lymphocyte (CTL) infiltration.
(A) Representative hematoxylin and eosin (H&E) staining images of different model 20 or 50 days after FPM-exposed mice were stimulated with LLC or Cre-inducible adenovirus (AdCre). Scale bar = 100 μm. (B) Tumor stage (stages 1–4) of lungs in KrasG12DTrp53-/- model (left) and representative tumors H&E staining images with higher magnification (right). p-Values are for comparisons of the percentage of stage 3 and 4 tumors in FPM-treated and control mice. n = 5. (C) Quantitative analysis of tumor number and tumor burden in lung tissue of different models treated as for panel (A). n = 5. (D) Survival analysis of mice exposed to FPM and subsequently stimulated with LLC or AdCre. n = 7. (E) Representative EdU staining images to analyze the proliferation rate of tumor site in lung tissue of LLC model 20 days after tumor initiation (DAPI, blue; EdU-positive cells, green). Scale bar = 100 μm. (F) The statistical analysis of CTLs (IFN-γ+CD8+/CD45+CD3+) in lung tissue based on flow cytometry at indicated day (0, 1, 3, 5, 10, and 20 days) after intravenous injection of LLC. n = 3. The inserted results in the dashed boxes indicate the fold change of CTLs in lung tissue 1 and 3 days after the LLC stimulation, relative to that under the physiological condition. (G) The statistical analysis of CTLs (IFN-γ+CD8+/CD45+CD3+) in lung tissue of KrasG12DTrp53-/- mice based on flow cytometry 4 weeks after tumor initiation with the intratracheal injection of AdCre. n = 3. (H) IFN-γ enzyme-linked immunospot assay (ELISpot) in the lung tissue of OT-1 TCR transgenic mice 1 day after ovalbumin-Lewis lung carcinoma (OVA-LLC) stimulation (upper) and representative immunospot images (lower). n = 5. (I) Representative H&E staining images of lung tissue in Foxn1nu nude mice exposed to FPM 20 days after intravenous injection of LLC. Scale bar = 100 μm. (J) Quantitative analysis of tumor number and tumor burden in lung tissue of Foxn1nu nude mice treated as for panel (I). n = 5. Images are representative of three independent experiments. Results are shown as mean ± SD. *p<0.05 after ANOVA with Dunnett’s tests.
-
Figure 1—source data 1
Physicochemical characteristic of fine particulate matter collected from airborne pollution in seven different locations.
- https://cdn.elifesciences.org/articles/75345/elife-75345-fig1-data1-v2.xlsx
-
Figure 1—source data 2
Excel spreadsheet source file for Figure 1C, D, F, G, H and J.
- https://cdn.elifesciences.org/articles/75345/elife-75345-fig1-data2-v2.xlsx

Morphology characterization of fine particulate matter collected from airborne pollution in different locations.
(A) Representative scanning electronic microscope (SEM) for particles detained on the fiber filter membrane, which were collected from airborne pollution in seven different locations, including Nanjing City (Qixia, Jiangning, Pukou, Gulou, and Gaochun) in Jiangsu Province, Suzhou City in Anhui Province, and Tieling City in Liaoning Province (named as QX, JN, PK, GL, GC, SZ, and TL) and tobacco smoke particle (TSP). Scale bar = 5 μm. (B) Representative transmission electron microscopy (TEM) images for detached particles from filter membrane in panel (A). Scale bar = 100 nm. Images are representative of three independent experiments.
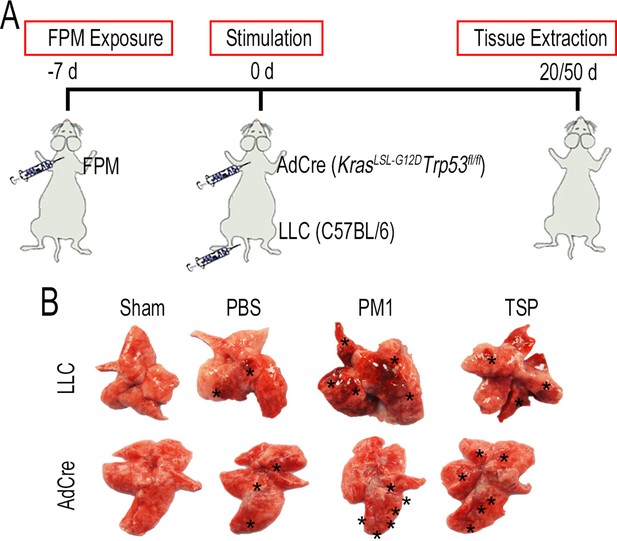
The effect of fine particulate matter on tumorgenesis in two lung cancer models.
(A) Schematic diagram of Lewis lung carcinoma (LLC)-stimulated or KrasG12DTrp53-/--transgenic lung cancer model with fine particulate matter (FPM) exposure. (B) Gross lung tissue images in the FPM-exposed mice of LLC model or KrasG12DTrp53-/-- model 20 or 50 days after mice were stimulated with LLC or Cre-inducible adevirus (AdCre). The tumor sites are labeled with black asterisk.
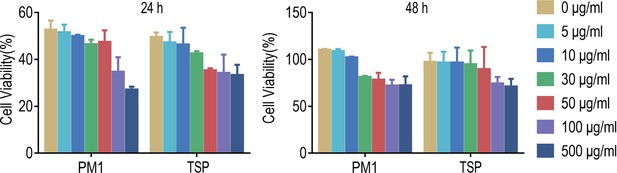
Cell cytotoxic analysis of Lewis lung carcinoma (LLC) cells stimulated with a serial concentration of fine particulate matter (FPM) (0, 5, 10, 30, 50, 100, and 500 μg/mL) for 24 and 48 hr.
n = 3. Results are shown as mean ± SD.
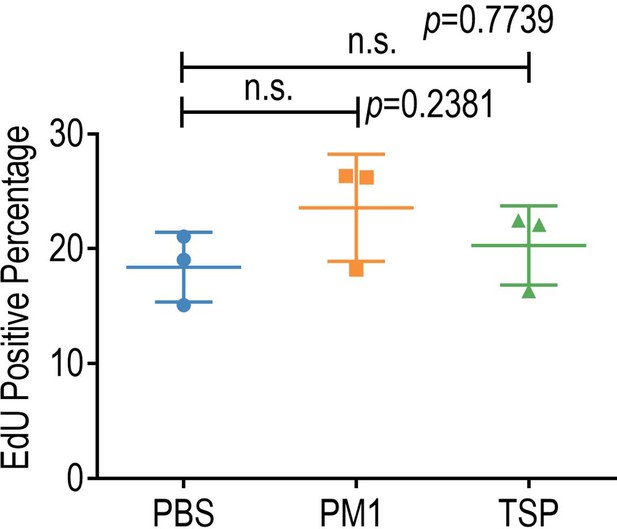
The quantified analysis of EdU-positive cells in tumor site of lung tissue of Lewis lung carcinoma (LLC) model 20 days after tumor initiation.
n = 3. Results are shown as mean ± SD. n.s., no statistical significance.

Flow cytometry analysis of CTLs in FPM-exposed lung tissue of mice after the LLC stimulation for indicated days.
(A) Gating strategy for the quantification of cytotoxic T lymphocyte (CTL) cells (IFN-γ+CD8+/CD45+CD3+) in the lung tissues. (B) Representative flow cytometry analysis of CTLs in fine particulate matter (FPM)-exposed lung tissue of mice under the physical conditions (0 day) or at indicated days (0, 1, 3, 5, 10, and 20 days) after intravenous injection with Lewis lung carcinoma (LLC).

Immunofluorescence analysis for the CTLs in FPM-exposed lung tissue after the LLC stimulation for indicated days.
Representative immunofluorescence images of cytotoxic T lymphocytes’ (CTLs’) infiltration into the fine particulate matter (FPM)-exposed lung tissue at indicated days (1, 3, 5, 10, and 20 days) after intravenous injection with Lewis lung carcinoma (LLC). The CTLs are labeled with CD8 and shown in green. Images are representative of three independent experiments. Scale bar = 200 μm.

Representative flow cytometry analysis of cytotoxic T lymphocytes (CTLs) (IFN-γ+CD8+/CD45+CD3+) in fine particulate matter (FPM)-exposed lung tissue of KrasG12DTrp53-/- mice 4 weeks after tumor initiation with the intratracheal injection of AdCre.

Representative flow cytometry analysis of cytotoxic T lymphocytes (CTLs) (IFN-γ+CD8+/CD45+CD3+) in fine particulate matter (FPM)-exposed lung tissue of OT-1 TCR transgenic mice 1 day after ovalbumin-Lewis lung carcinoma (OVA-LLC) stimulation.

Gross lung tissue images in fine particulate matter (FPM)-exposed Foxn1nu nude mice 20 days after they were intravenously injected with Lewis lung carcinoma (LLC).

Flow cytometry analysis of CTLs in FPM-exposed lung tissue of mice after the stimulation of chemokine IP-10 for 2 hr.
(A) Scheme of analyzing the effect of T cell chemokines IP-10 on the cytotoxic T lymphocytes’ (CTLs’) infiltration into the fine particulate matter (FPM)-exposed mice. (B) Statistical flow cytometry analysis of CTL cells (IFN-γ+CD8+/CD45+CD3+) in lung tissue 2 hr after the mice were stimulated with 5 μg/kg IP-10 through intratracheal injection. n = 3. Results are shown as mean ± SD. *p<0.05 after ANOVA with Dunnett’s tests.
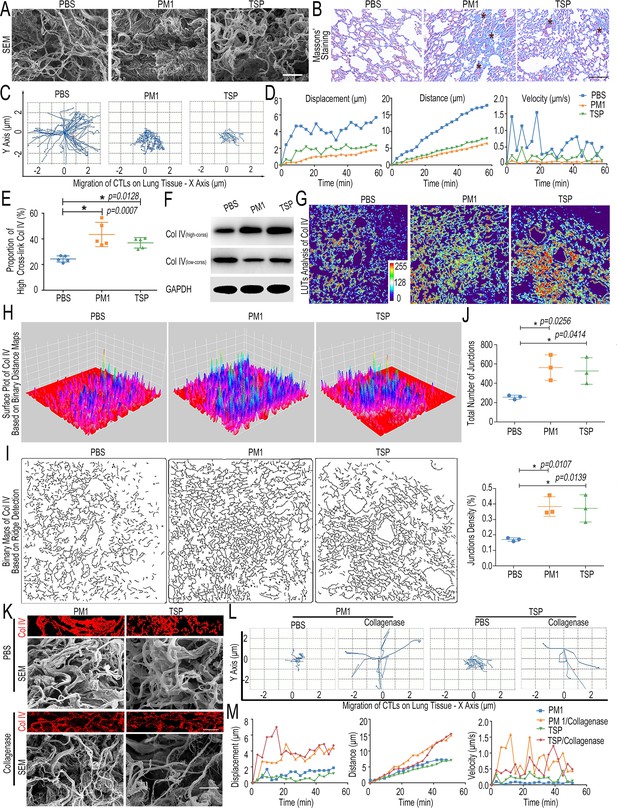
Fine particulate matter (FPM) impaired cytotoxic T lymphocytes’ (CTLs’) migration by increasing Col IV crosslinking in the lung tissue.
(A) Scanning electronic microscope (SEM) images of the interstitial matrix in the lung tissue exposed to FPM for 7 days. Scale bar = 100 μm. (B) Representative Masson’s trichrome histological analysis of lung tissue exposed to FPM for 7 days. Images are representative of three independent experiments. Scale bar = 100 μm. (C) Representative trajectory of CTLs’ migration in lung tissue slice of FPM-exposed mice or phosphate buffer saline (PBS) group. (D) The quantified analysis of migration displacement, distance, and velocity of tracked CTLs vs. time (min) based on panel (C). (E) Proportion of high-crosslink Col IV in lung tissue of mice exposed to FPM or PBS for 7 days, which was calculated by the ‘high-cross’ Col IV fragment divided by the sum of different fractions (‘low-cross’ ones and ‘high-cross’ ones). The content of each part was detected by ELISA. n = 5. (F) Western blotting analysis of ‘low-cross’ collagen and ‘high-cross’ collagen in lung tissue of mice exposed to FPM or PBS for 7 days. (G–J) The in-depth analysis of representative Col IV immunofluorescence images of lung tissue in the mice exposed to FPM for 7 days through ImageJ. (G) Look-up tables (LUTs) analysis of Col IV fluorescence intensity. (H) Surface plot analysis of Col IV distribution based on invert binary distance maps. (I) Binary images of Col IV network generated by ridge detection plugin. (J) Quantification analysis of junction number and density in Col IV network based on panel (I). n = 3. (K) Representative immunofluorescence images of Col IV and SEM images of FMP-exposed lung tissue treated with collagenase D (50 μg/mL). Scale bar = 10 μm. (L) The trajectory of CTLs migrating in FPM-exposed lung tissue slice treated as for panel (K). (M) Average migration displacement, distance, and velocity of tracked CTLs vs. time (min) in lung tissue slice treated as in panel (K). Images are representative of three independent experiments. Results are shown as mean ± SD. *p<0.05 after ANOVA with Dunnett’s tests.
-
Figure 2—source data 1
Excel spreadsheet source file for Figure 2D, E, J and M.
- https://cdn.elifesciences.org/articles/75345/elife-75345-fig2-data1-v2.xlsx

Transcriptional level of typical markers related to cytotoxic T lymphocytes’ (CTLs’) migration, integrin-1 (ITGB-1), C-X-C motif chemokine receptor 3 (CXCR 3), and Rho-associated kinase (ROCKi) in Jurkat T cells after they were stimulated with fine particulate matter (FPM) for 48 hr (A) or in the CTLs separated from lung tissue exposed to FPM for 7 days (B).
n = 3. Results are shown as mean ± SD. n.s., no statistical significance. p-Values are listed underneath.
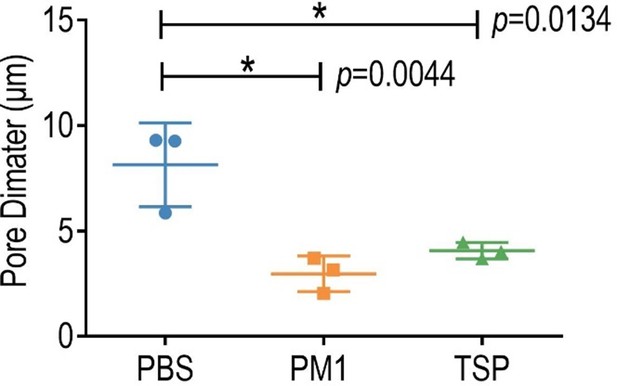
The quantified analysis of pore diameter of interstitial matrix in the lung tissue, based on the scanning electron microscope (SEM) images and analyzed with ImageJ.
n = 3. Results are shown as mean ± SD. *p<0.05 after ANOVA with Dunnett’s tests.

Schematic diagram of analyzing of cytotoxic T lymphocytes’ (CTLs’) migration in lung tissue slice of fine particulate matter (FPM)-exposed mice or phosphate buffer saline (PBS) group.
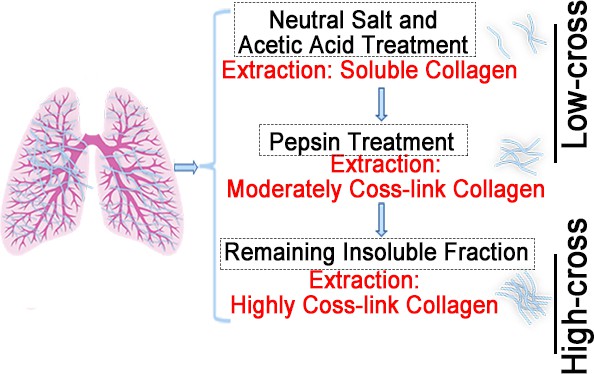
Schematic diagram of separating collagen fraction with different crosslink level.
The part separated by neutral salt, acetic acid, and pepsin is defined as ‘low-cross’ and the residual ones are regarded as ‘high-cross’ according to the reported literature (Popov et al., 2011).
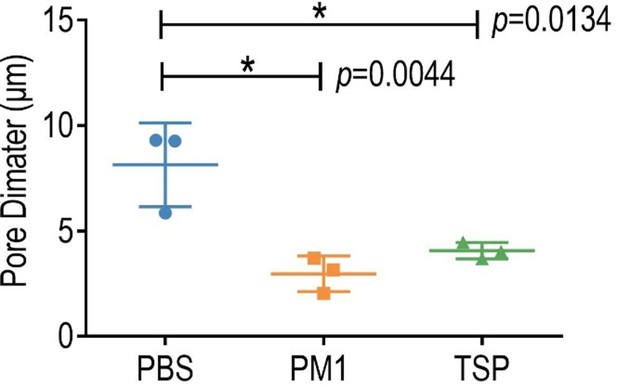
The relative intensity of high-crosslink Col IV to low-crosslink ones according to the Western blotting (WB) results of lung tissue exposed to fine particulate matter (FPM) for 7 days based on the ImageJ analysis.
n = 3. Results are shown as mean ± SD. *p<0.05 after ANOVA with Dunnett’s tests. n.s., no statistical significance.

Representative Col IV immunofluorescence images of lung tissue in the mice exposed to fine particulate matter (FPM) for 7 days, with the blue DAPI staining images shown in the inserted box.
Scale bar = 100 μm.
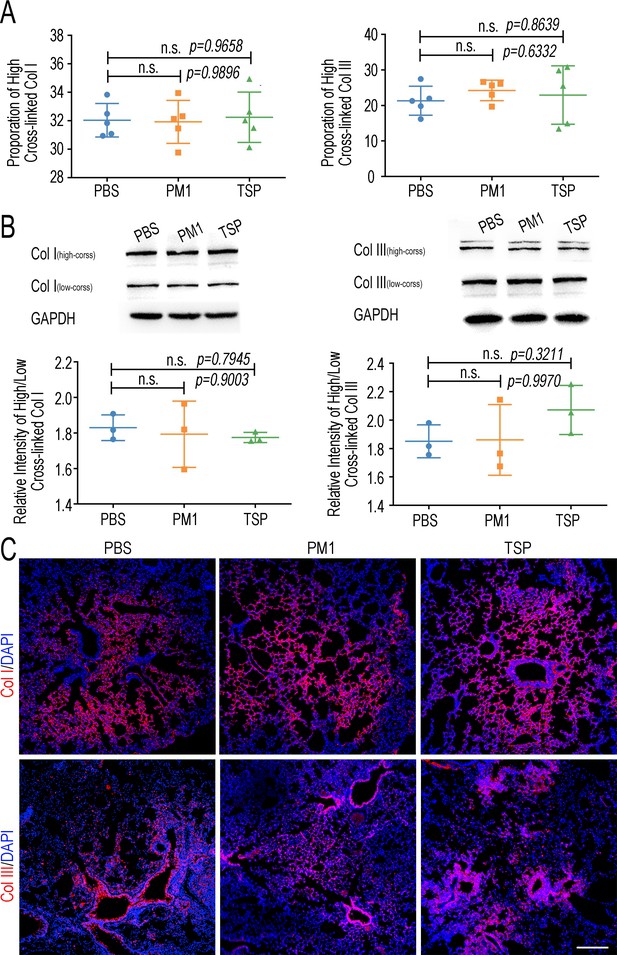
Analysis of crosslinking level of Col I and Col III in lung tissue of mice exposed to FPM.
(A) Proportion of high-crosslink Col I (left) and Col III (right) in lung tissue of mice exposed to fine particulate matter (FPM) or phosphate buffer saline (PBS) for 7 days, which was calculated by the ‘high-cross’ fragment divided by the sum of different fraction (‘low-cross’ ones and ‘high-cross’ ones). The content of each part was detected by ELISA. n = 5. (B) Western blotting analysis of ‘low-cross’ collagen and ‘high-cross’ Col I (left) and Col III (right) in lung tissue of mice exposed to FPM or PBS for 7 days. Their relative intensity analyzed with ImageJ is shown underneath. n = 3. (C) Representative Col I and Col III immunofluorescence images of lung tissue in the mice exposed to FPM for 7 days. Scale bar = 100 μm. Results are shown as mean ± SD. n.s., no statistical significance.
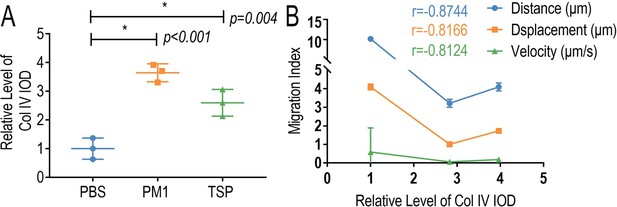
The correlation analysis of CTLs' migration index with the level of Col IV crosslink.
(A) The integrated optical density (IOD) of Col IV immunofluorescence images of lung tissue exposed to fine particulate matter (FPM) for 7 days based on ImageJ analysis. n = 3. Results are shown as mean ± SD. *p<0.05 after ANOVA with Dunnett’s tests. (B) Pearson’s correlation line of the migration index (migration distance, displacement, and velocity) of different groups with IOD of Col IV in corresponding lung tissue slice as panel (A). n = 3. Results are shown as mean ± SD. *p<0.05 after ANOVA with Dunnett’s tests.

Schematic diagram about cytotoxic T lymphocytes’ (CTLs’) migration in the lung tissue exposed to fine particulate matter (FPM).
Dynamic migration video of T cells in the slice of native lung tissue.
Jurkat T cells were pre-stained with Calcein-AM and labeled as green in video.
Dynamic migration video of T cells in the slice of lung tissue exposed to PM1.
Jurkat T cells were pre-stained with Calcein-AM and labeled as green in video.
Dynamic migration video of T cells in the slice of lung tissue exposed to TSP.
Jurkat T cells were pre-stained with Calcein-AM and labeled as green in video.
Dynamic migration video of T cells on PM1-exposed lung tissue pretreated with with PBS.
Jurkat T cells were pre-stained with Calcein-AM and labeled as green in video. Collagen IV in lung tissue are labeled as red.
Dynamic migration video of T cells on PM1-exposed lung tissue pre-treated with collagenase D.
Jurkat T cells were pre-stained with Calcein-AM and labeled as green in video. Collagen IV in lung tissue are labeled as red.
Dynamic migration video of T cells on TSP-exposed lung tissue pre-treated with PBS.
Jurkat T cells were pre-stained with Calcein-AM and labeled as green in video. Collagen IV in lung tissue are labeled as red.
Dynamic migration video of T cells on TSP-exposed lung tissue pre-treated with collagenase D.
Jurkat T cells were pre-stained with Calcein-AM and labeled as green in video. Collagen IV in lung tissue are labeled as red.
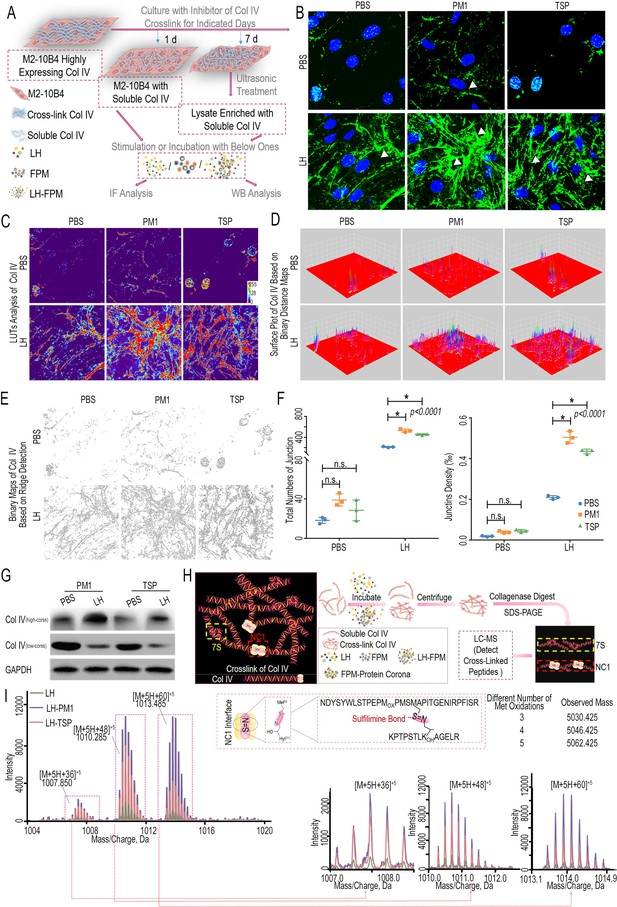
Fine particulate matter (FPM) increased Col IV crosslink via promoting sulfilimine bond formation at the NC1 domain.
(A) Schematic representation of the procedures to generate soluble Col IV and analyze the effect of FPM on its crosslink. Briefly, the M2-10B4 cells highly expressing Col IV were treated with crosslink inhibitor for 1 or 7 days and then treated with FPM or the mixture of lung homogenate (LH)-FPM, stimulating the scenario of FPM per se or its interface with LH, to initiate the crosslink. The crosslink level of Col IV was analyzed with diversity methods. (B) Representative immunofluorescence capture of Col IV in M2-10B4 cells stimulated with FPM or LH-FPM for 24 hr after pretreated with crosslink inhibitor for 1 day. Scale bar = 20 μm. Images are representative of three independent experiments. (C–F) The in-depth analysis of Col IV immunofluorescence images in panel (B) through ImageJ. (C) Look-up tables (LUTs) analysis of Col IV fluorescence intensity. (D) Surface plot analysis of Col IV distribution based on invert binary distance maps. (E) Binary images of Col IV network generated by ridge detection plugin. (F) Quantification analysis of junction number and density in Col IV network based on panel (E). (G) Western blotting of ‘low-cross’ and ‘high-cross’ Col IV fraction in M2-10B4 cells lysate enriched with soluble collagen after their treatment with FPM or LH-FPM. (H) Schematic diagram of separating fragments containing the NC1 domain crosslink site in Col IV. The general crosslink network generated by Col IV is displayed on the left, with the important crosslink sites (7S domain and NC1 domain) respectively labeled in the yellow and red dotted box. (I) High-resolution mass spectrum depicting tryptic peptides containing sulfilimine bond (-S=N-), with magnified spectrum displayed on the bottom. The formation of -S=N- and the known peptide sequence with different oxidation containing the sulfilimine bond are shown on the upper right.
-
Figure 3—source data 1
Excel spreadsheet source file for Figure 3F and I.
- https://cdn.elifesciences.org/articles/75345/elife-75345-fig3-data1-v2.xlsx

Western blotting analysis of ‘soluble’ and ‘crosslinked’ Col IV fraction in M2-10B4 cells lysate after the cells were treated with different concentrations of crosslink inhibitor phloroglucinol (PHG) for 7 days.
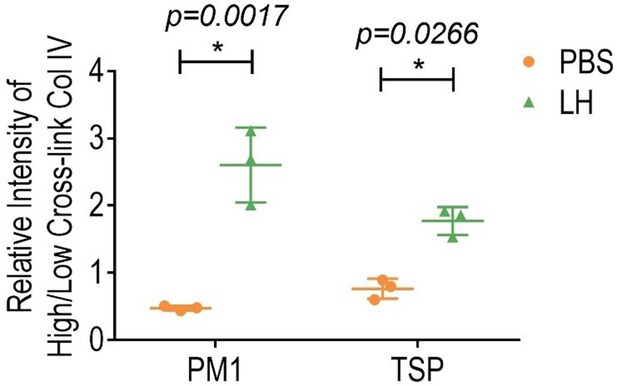
The relative intensity of high-crosslink Col IV to low-crosslink ones according to the Western blotting (WB) results in M2-10B4 cells lysate enriched with soluble collagen after their treatment with fine particulate matter (FPM) or the mixture of lung homogenate (LH) and FPM, that is, LH-FPM.
n = 3. Results are shown as mean ± SD. *p<0.05 after ANOVA with Dunnett’s tests.
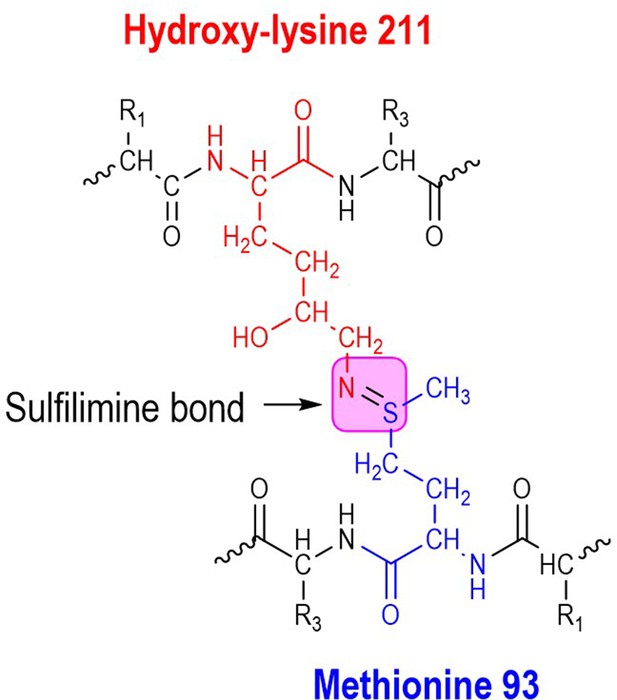
Structure of sulfilimine bond formed at the covalent crosslinks of NC1 domains, shown in the lilac box.

The analysis of allysine to reflect the 7S domain generated during the Col IV crosslinking.
(A) The potential crosslink site formed at 7S domain, containing methylenimine bond (-C=N-, left) or pyridinium crosslink (right), shown in the lilac box. (B) The allysine generated during the Col IV crosslinking after soluble Col IV were incubated with lung homogenate (LH) per se or the mixture of LH-fine particulate matter (FPM) (LH-PM 1 and LH-TSP) for 30 min, detected with specific probes for allysine, with the oxidized bovine serum albumin containing known aldehydes as the standard (Waghorn et al., 2017). n = 3. Results are shown as mean ± SD. n.s., no statistical significance.
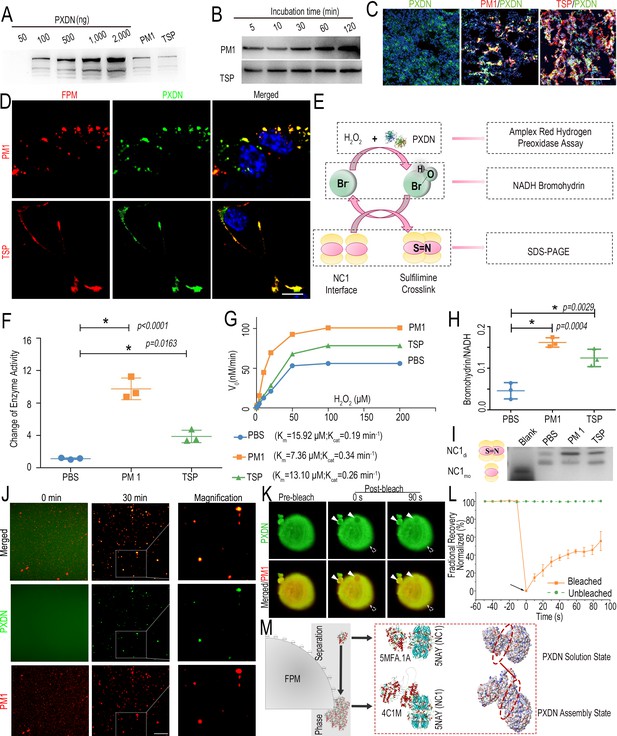
Fine particulate matter (FPM) increased peroxidasin (PXDN) activity by triggering the enzyme’s phase transition.
(A) Quantitative Western blotting analysis of PXDN harvested from FPM corona formed in lung homogenate (LH) after incubation for 2 hr, with a serial content of recombinant PXDN protein as the standard control. (B) Western blotting analysis of PXDN at indicated time points to identify its time evolution in FPM’s protein corona. (C) Representative confocal microscopic photographs showing the co-localization of rhodamine-labeled FPM (shown in red) and PXDN in lung tissue. PXDN is indicated as green. Scale bar = 100 μm. (D) Representative fluorescent photographs of M2-10B4 cells treated with rhodamine-labeled FPM and fluorescein isothiocyanate (FITC)-labeled PXDN for 1 hr. Rhodamine-labeled FPM is shown in red, FITC-PXDN in green, and DAPI staining for the nuclei in blue. Scale bar = 10 μm. (E) Schematic representation of the procedure for the formation of sulfilimine bond catalyzed by PXDN. Aimed at the substrate H2O2, the intermediates HOBr and the final NC1 domain with sulfilimine bond, different experimental analyses were respectively performed. (F, G) Fold change of enzyme activity (F) and enzyme kinetics (G) of PXDN stimulated with FPM, determined with Amplex Red Hydrogen Peroxidase Assay Kit. (H) Ration of NADH bromohydrin relative to NADH based their intensity of peaks detected by liquid chromatography-mass spectrometry (LC-MS). The analysis was performed after PXDN was incubated with FPM for 30 min and then catalyzed in the presence of 100 μM H2O2 and 200 μM NaBr at 37°C for 30 min. (I) SDS-PAGE and Coomassie staining of NC1 domain 4 hr after they were incubated with PXDN, following the latter’s incubation with PBS or FPM for 30 min. The crosslinked dimeric (NC1di) and un-crosslinked monomeric subunits (NC1mo) are respectively labeled. Images are representative of three independent experiments. (J) The confocal microscopy of FITC-labeled PXDN was incubated with rhodamine-labeled FPM in LH for the indicated time (0 and 30 min). Shown at the right are images with higher magnification for the assemblies of PXDN’s liquid-like droplets on the FPM at 30 min. Scale bar = 5 μm. (K) Representative images from fluorescence recovery after photobleaching (FRAP) experiments showing the dynamic and reversible characteristics of PXDN droplets. The rhodamine-labeled FPM is shown in red and FITC-labeled PXDN in green. The bleached region of interest (ROI) is indicated with white triangles, and the unbleached control ROI is labeled with dotted white ones. (L) Quantification of fluorescence recovery percentage in the ROIs of PXDN’s liquid-like droplets. The black arrow indicates the initiation of laser bleach treatment. (M) Interactive docking model on the effect of PXDN’s phase separation on its enzymatic performance at the catalytic interface of NC1 domain. The structures of PXDN solution state (PDB ID: 5MFA.1) and its assembly (PDB ID: 4C1M; created through homology modeling) are respectively displayed as the lateral stereo view of transparent chain model (left) and SWISS-MODEL (right). The interface site of contact between NC1 domain (PDB ID: 5NAY) and PXDN is labeled with the red dotted ellipses. n = 3. Results are shown as mean ± SD. *p<0.05 after ANOVA with Dunnett’s tests.
-
Figure 4—source data 1
List of protein components identified by liquid chromatography-mass spectrometry (LC-MS) for PM 1’s and tobacco smoke particulates’ protein corona.
- https://cdn.elifesciences.org/articles/75345/elife-75345-fig4-data1-v2.xlsx
-
Figure 4—source data 2
Excel spreadsheet source file for Figure 4F–H,L.
- https://cdn.elifesciences.org/articles/75345/elife-75345-fig4-data2-v2.xlsx

Schematic diagram of separation and preparation of fine particulate matter’s (FPM‘s) protein corona in lung homogenate (LH).
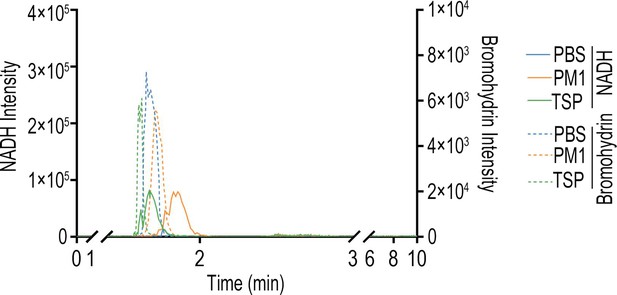
Liquid chromatography-mass spectrometry (LC-MS) spectrum for NADH (dotted line) and the bromohydrin (line), according to the reported literature (Bathish et al., 2018).
The analysis was performed after the enzyme peroxidasin (PXDN) was incubated with fine particulate matter (FPM) for 30 min and then catalyzed in the presence of 100 μM H2O2 and 200 μM NaBr at 37°C for 30 min.
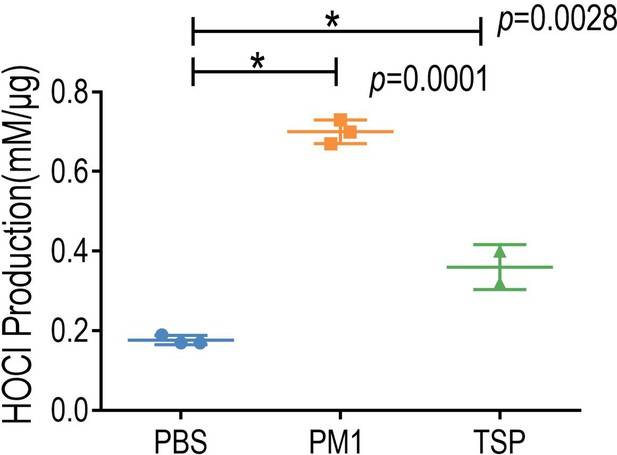
HOCl production induced by peroxidasin (PXDN) measured with HOCl detecting fluorescent probes after the enzyme was incubated with fine particulate matter (FPM) for 30 min and then catalyzed in the presence of 100 μM H2O2 and 200 mM NaCl, with a serial concentration of HOCl as internal control.
n = 3. Results are shown as mean ± SD. *p<0.05 after ANOVA with Dunnett’s tests.
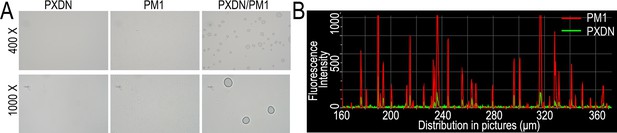
Effect of fine particulate matter (FPM) on peroxidasin’s (PXDN’s) phase separation.
(A) Phase-contrast microscopy of PXDN per se or incubated with FPM for 30 min in lung homogenate (LH). (B) The fluorescence distribution profiles of the cross-sectional region of liquid-like droplets on the FPM after fluorescein isothiocyanate (FITC)-labeled PXDN was incubated with rhodamine-labeled FPM in LH for 30 min.
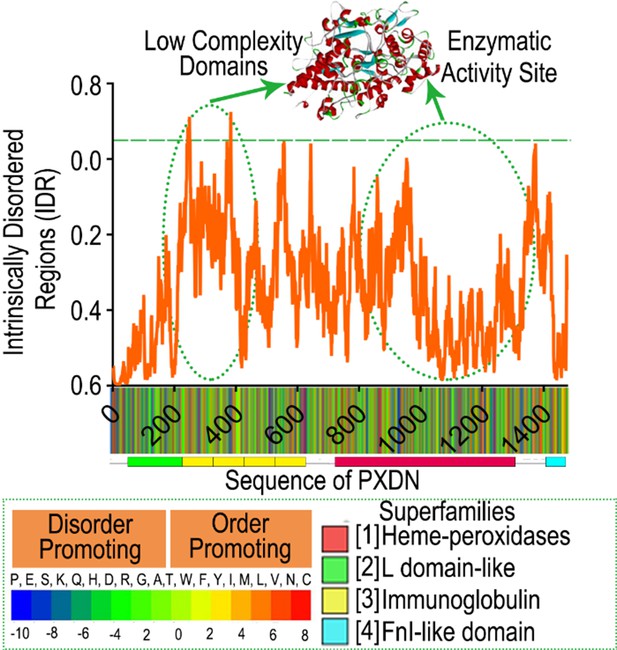
Intrinsically disordered region (IDR) analysis of peroxidasin (PXDN) domains by the IUPred algorithm.
The low-complexity domains and enzymatic activity site are respectively labeled with dotted green circles. PXDN’s template crystal structures are shown on the upper. Besides, bioinformatics analysis of the amino acid sequence of full-length PXDN is shown underneath. The kinds of amino acids with order or disorder potential are listed with different colors, and the superfamily of PXDN is shown on the lower right (Bernardes et al., 2015).
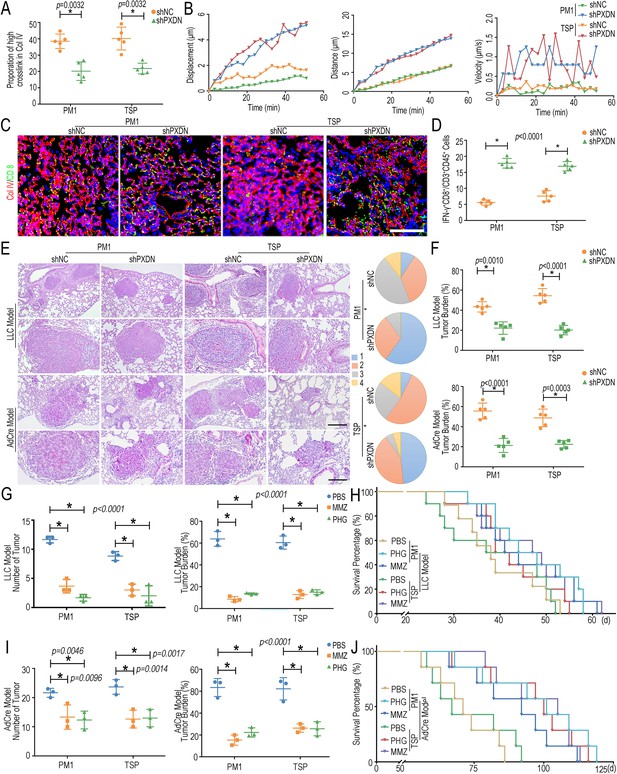
Peroxidasin (PXDN) inhibitor alleviated fine particulate matter (FPM)-induced lung tumorigenesis.
(A) Proportion of high-crosslink Col IV in lung tissue of FPM-exposed mice pretreated with PXDN-specific short hairpin RNA (PXDN shRNA, shPXDN), which was calculated by the ‘high-cross’ Col IV fragment divided by the sum of different fractions (‘low-cross’ ones and ‘high-cross’ ones) based on ELISA. (B) Migration displacement, distance, and velocity vs. time (min) of tracked cytotoxic T lymphocytes (CTLs) in lung tissue slice of FPM-exposed mice administrated with PXDN shRNA (shPXDN). (C) Representative immunofluorescence images of CTLs’ infiltration into the FPM-exposed lung tissue pretreated with shPXDN 1 day after intravenous injection of Lewis lung carcinoma (LLC). Scale bar = 100 μm. (D) The statistical analysis of CTLs (IFN-γ+CD8+/CD45+CD3+) based on flow cytometry in lung tissue of mice treated as in panel (C). n = 5. (E) Representative hematoxylin and eosin (H&E) staining images of lung tissue (the lower ones: captured with higher magnification) yielded from LLC model and KrasG12DTrp53-/- model after mice pretreated with shPXDN. Scale bar = 200 μm (upper) and 100 μm (lower). Tumor stage (stages 1–4) in lungs of KrasG12DTrp53-/- mice is shown on the right. p-Values are for comparisons of the percentage of stage 3 and 4 tumors in different groups. n = 5. (F) Statistical analysis of tumor burden of mice in LLC model and KrasG12DTrp53-/- model administrated with shPXDN. n = 5. (G–J) Statistical analysis of number and burden of tumors and survival curve of mice in LLC model (G, H) and KrasG12DTrp53-/- model (I, J) administrated with methimazole (MMZ) or phloroglucinol (PHG). Images are representative of three independent experiments. n = 3. Results are shown as mean ± SD. *p<0.05 after ANOVA with Dunnett’s tests.
-
Figure 5—source data 1
Excel spreadsheet source file for Figure 5.
- https://cdn.elifesciences.org/articles/75345/elife-75345-fig5-data1-v2.xlsx
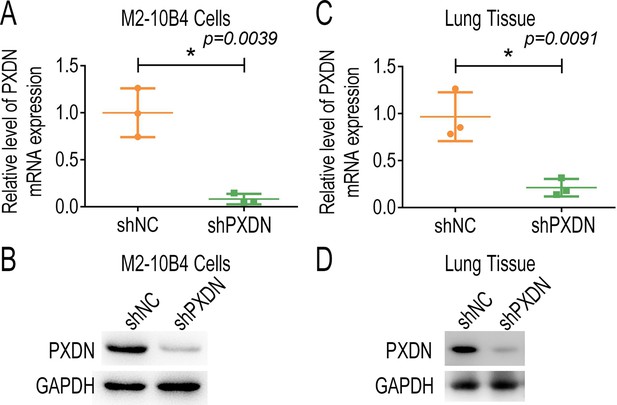
The interference efficiency of PXDN-specific short hairpin RNA detected in cellular and lung tissue experiment.
(A, B) The transcriptional-level analysis (A) and Western blotting analysis (B) of peroxidasin (PXDN) in M2-10B4 cells after they were transfected with plasmids capable of ectopically expressing PXDN-specific short hairpin RNA (PXDN shRNA, shPXDN) or control shRNA (shNC) for 48 hr. (C, D) The transcriptional-level analysis (C) and Western blotting analysis (D) of PXDN in lung tissue after the mice were administrated with 4 μg plasmids capable of ectopically expressing shPXDN mixed in the in vivo-jetPEI gene transfer regent through trachea injection every 3 days four times.
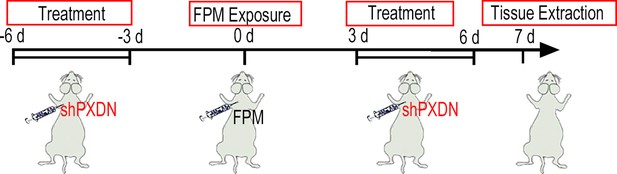
Schematic diagram of analyzing the effect of peroxidasin (PXDN)-specific short hairpin RNA interference (PXDN shRNA, shPXDN) on the structure of fine particulate matter (FPM)-exposed lung tissue.

The histological analysis and SEM images of FPM-exposed lung tissue administrated with shPXDN.
(A) Representative Masson’s trichrome histological analysis of lung tissue in fine particulate matter (FPM)-exposed mice administrated with short hairpin peroxidasin (shPXDN) (PXDN shRNA). Scale bar = 100 μm. (B) Representative scanning electronic microscope (SEM) images of interstitial matrix in the lung tissue in FPM-exposed mice administrated with shPXDN. Scale bar = 50 μm.

Representative flow cytometry analysis of cytotoxic T lymphocytes’ (CTLs’) infiltration (IFN-γ+CD8+/CD45+CD3+) into lung tissue of fine particulate matter (FPM)-exposed group pretreated with short hairpin peroxidasin (shPXDN) 1 day after they were stimulated with the Lewis lung carcinoma (LLC).

Schematic diagram of administration of shPXDN on lung tumor related to FPM exposure.
Schematic diagram of analyzing the therapeutic effect of short hairpin peroxidasin (shPXDN) on Lewis lung carcinoma (LLC)-stimulated or KrasG12DTrp53-/--transgenic lung cancer model with fine particulate matter (FPM) exposure.

Therapeutic effect of shPXDN on lung tumor related to FPM exposure.
(A, B) Gross lung tissue images in Lewis lung carcinoma (LLC)-stimulated (A) or KrasG12DTrp53-/--transgenic lung cancer model (B) administrated with short hairpin peroxidasin (shPXDN). (C, D) Statistical analysis of tumor number in LLC-stimulated (C) or KrasG12DTrp53-/--transgenic lung cancer model (D) administrated with shPXDN. n = 5. Results are shown as mean ± SD. *p<0.05 after ANOVA with Dunnett’s tests.
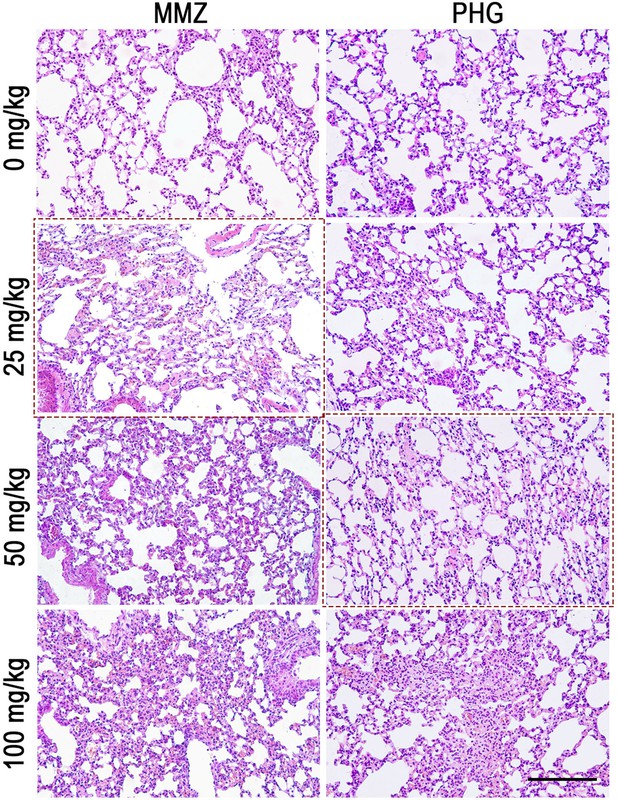
Representative hematoxylin and eosin (H&E) staining images of lung tissue with intratracheal injection with different concentrations of methimazole (MMZ) and phloroglucinol (PHG) twice every three days.
Scale bar = 100 μm. The concentration in the indicated group with red dotted lines was chosen as the subsequent administration dose.

Administration and therapeutic effect of PXDN inhibitors on lung tumor related to FPM exposure.
(A) Schematic diagram of analyzing the therapeutic effect of peroxidasin (PXDN) inhibition (methimazole [MMZ] or phloroglucinol [PHG]) on Lewis lung carcinoma (LLC)-stimulated or KrasG12DTrp53-/--transgenic lung cancer model with fine particulate matter (FPM) exposure. (B, C) Gross images of lung tissue yielded from LLC-induced model (B) or KrasG12DTrp53-/--transgenic lung cancer model (C) 20 or 50 days after mice pretreated with MMZ or PHG were stimulated with LLC or AdCre. (D, E) Representative hematoxylin and eosin (H&E) staining images of lung tissue yielded from LLC-induced model (D) or KrasG12DTrp53-/--transgenic lung cancer model (E) as panels (B) and (C). Images are representative of three independent experiments.
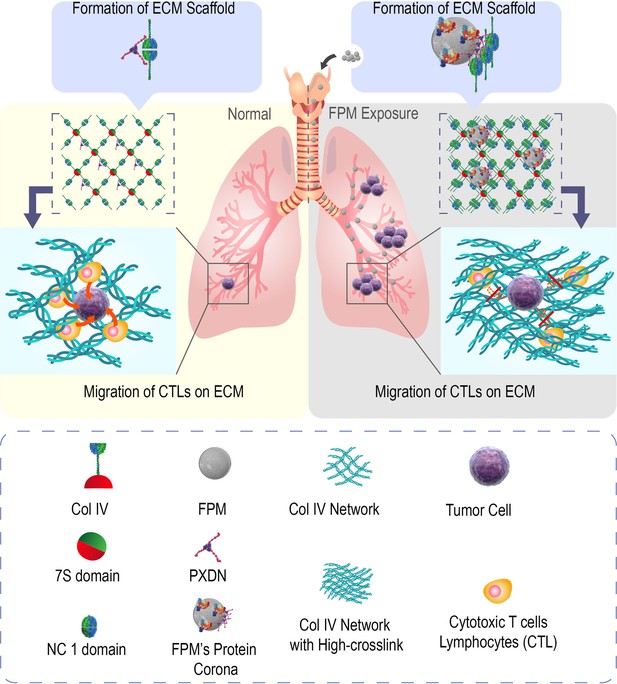
Schematic diagram of the mechanism underlying how fine particulate matter (FPM) promotes lung tumorigenesis.
The adsorption of FPM triggers the phase separation of peroxidasin (PXDN) to generate an aberrant catalytic activity and induce a disordered crosslink of Col IV. Reinforced Col IV network impaired cytotoxic T lymphocytes’ (CTLs’) migration and local immunosurveillance, which considerably increases tumorigenesis in lung tissue.
Additional files
-
Transparent reporting form
- https://cdn.elifesciences.org/articles/75345/elife-75345-transrepform1-v2.pdf
-
Source data 1
Collected raw data for gels and blots.
- https://cdn.elifesciences.org/articles/75345/elife-75345-data1-v2.zip