A transcriptomic atlas of Aedes aegypti reveals detailed functional organization of major body parts and gut regional specializations in sugar-fed and blood-fed adult females
Figures
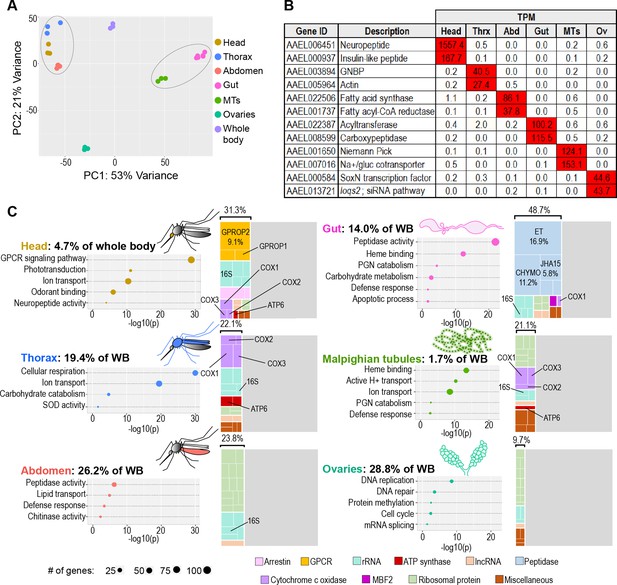
The transcriptomes of Aedes aegypti body parts reflect their embryonic origins and functional specializations.
(A) Principal component analysis of the transcriptomes of whole body and body parts; 3–6 replicates per body part. Circled clusters contain body parts of dominantly endodermal (left) and mesodermal/ectodermal (right) derivation. (B) Expression of body-part-specific putative tissue markers (in TPM) (C) Calculated estimates of body parts’ contributions to whole body transcriptome; Bubble plots: classic Fisher topGO gene ontology enrichment analysis of genes enriched 5 x (head, gut thorax, Malpighian tubules, abdomen) or 2 x (ovaries) relative to whole body (DESeq2, padj <0.05); Treemaps: 20 highest expressed genes per body part, grouped by category/function; percentages reflect the proportion of the transcriptome comprised by the displayed genes.
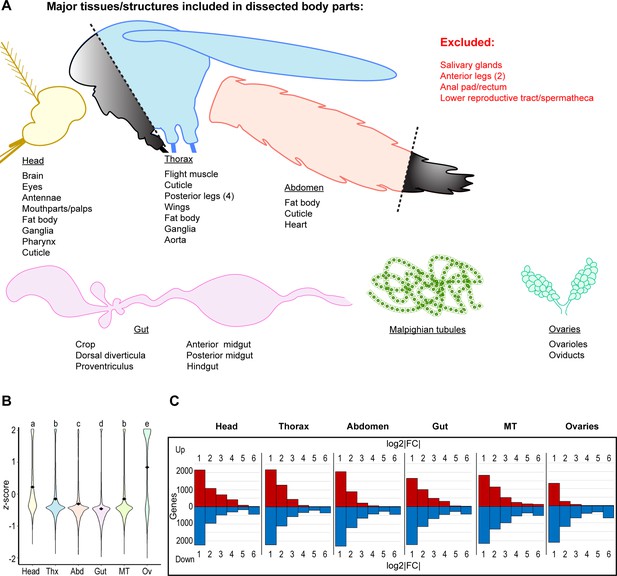
Ovaries possess many highly enriched genes relative to other body parts, but few relative to whole body.
(A) Diagram of included/excluded structures/tissues in dissected body parts (B) Transcriptome-wide z-scores for gene expression by body part. Data are z-scores for genes expressed >2 TPM in one or more body parts; black diamonds mark mean z-scores; statistics: One-way ANOVA, Tukey HSD. (C) Transcriptomic variation from whole body expression by body part (fold change adjusted by DESeq2, no significance criterion applied).
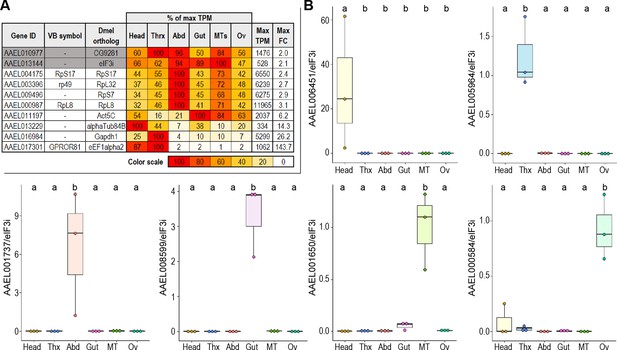
RT-qPCR confirms the anatomical specificity of putative marker genes.
(A) Comparison of candidate reference genes for RT-qPCR analysis of putative marker genes; expression values are scaled as a percentage of the maximum expression observed. The body part with the highest expression (in TPM) is scored as 100, with its TPM displayed in the ‘Max TPM’ column. ‘Max FC’ column displays the variation (in fold-change) across all conditions. Genes in white are previously published reference genes; genes in gray are candidate reference genes identified in this study. (B) RT-qPCR validation of putative marker gene expression; reference gene: AAEL013144; 3 replicates; statistics: One-way ANOVA, Tukey HSD.
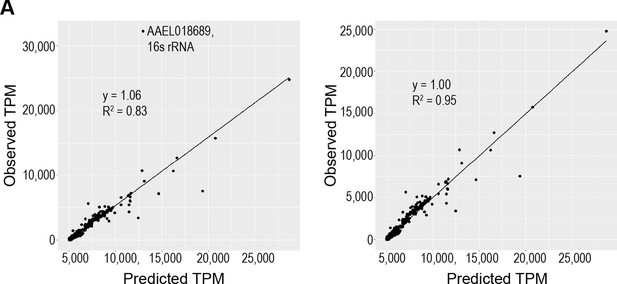
Calculated scaling factors accurately predict whole body gene expression.
(A) Validation of estimated scaling factors for body part transcript contribution to whole body expression, with (left) and without (right) outlying gene.
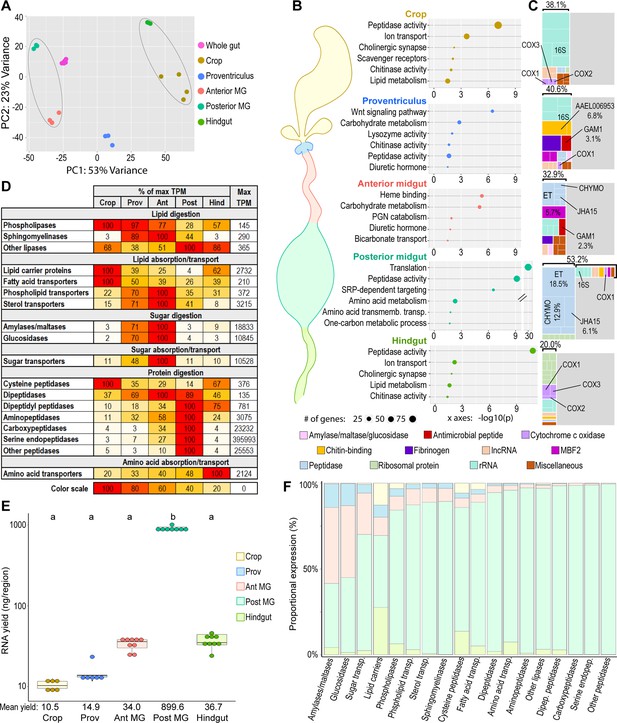
Mosquito gut regions’ transcriptomic investments and outputs reveal digestive specializations.
(A) Principal component analysis of the transcriptomes of whole gut and gut regions; 3–6 replicates. Circled clusters contain body parts of partial endodermal (left) and ectodermal (right) derivation. (B) Bubble plots: classic Fisher topGO gene ontology enrichment analysis of regionally enriched genes (for crop, proventriculus, and anterior midgut: 5 x enriched over whole-gut, DESeq2 padj < 0.05; for posterior midgut: enriched over all other gut regions, DESeq2 padj < 0.05) (C) Treemaps: 20 highest expressed genes per region, grouped by category/function; percentages reflect the proportion of the transcriptome comprised by the displayed genes. (D) Regional investments in categories of digestive enzymes and transporters. Colored cells are scaled as percentages of the region with the highest transcriptional investment in the category (as measured by cumulative TPM). The region with the highest investment is scored as ‘100’, with its cumulative TPM displayed in the rightmost column (‘Max TPM’). (E) Estimates of regions’ transcriptional output by empirical quantification of RNA yield; statistics: One-way ANOVA, Tukey HSD. (F) Estimated output of digestive enzymes/transporters by region (categorical investments weighted by regional RNA yield).
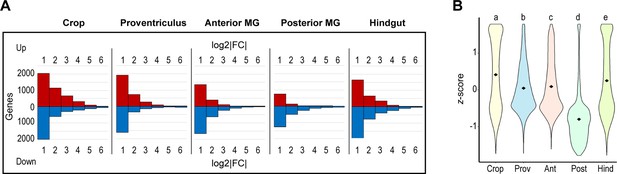
The transcriptome of the posterior midgut displays little deviation from the whole-gut transcriptome and low transcriptomic diversity.
(A) Transcriptomic variation from whole gut expression by gut region (fold change adjusted by DESeq2, no significance criterion applied). (B) Transcriptome-wide z-scores for gene expression by gut region. Data are z-scores for genes expressed >2 TPM in one or more regions; black diamonds mark mean z-scores; statistics: One-way ANOVA, Tukey HSD.
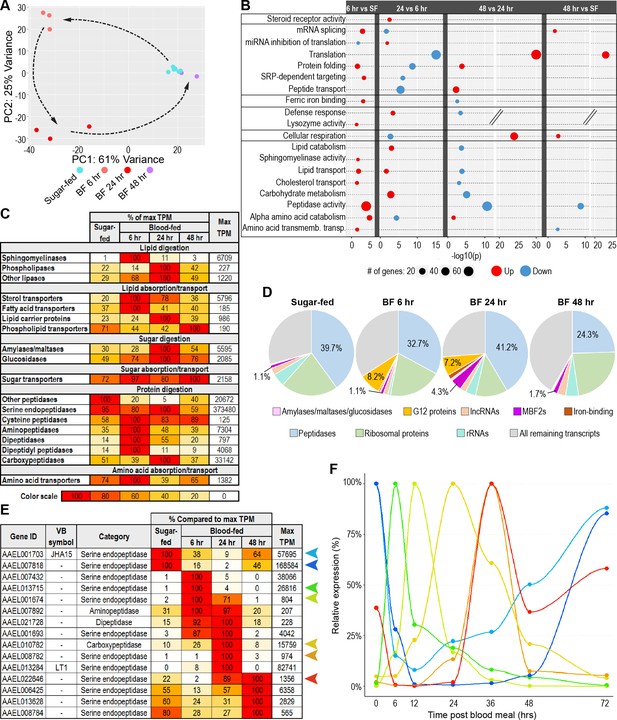
The gut’s transcriptome shifts continuously throughout blood meal digestion.
(A) Principal component analysis of the transcriptomes of sugar-fed and blood-fed guts; 3–6 replicates per condition. (B) Classic Fisher topGO gene ontology enrichment analysis of blood-fed guts at each timepoint pbm, in comparison to prior timepoint (DESeq2, padj <0.05, minimum of 2 x fold-change). (C) Investments in categories of digestive enzymes and transporters by timepoint/condition. Colored cells are scaled as percentages of the region with the highest transcriptional investment in the category (as measured by cumulative TPM). The region with the highest investment is scored as ‘100’, with its cumulative TPM displayed in the rightmost column. (D) Proportional investment (out of whole transcriptome) in selected gene categories/families by timepoint/condition. (E) Expression of selected peptidases, scaled as a percentage of maximum expression. The region with the highest expression (in TPM) is scored as ‘100’, with its TPM displayed in the rightmost column. (F) Relative expression of selected peptidases at 0, 6, 12, 24, 36, 48, and 72 hrs post-blood meal measured by RT-qPCR; reference gene: AAEL009653; 3 replicates.
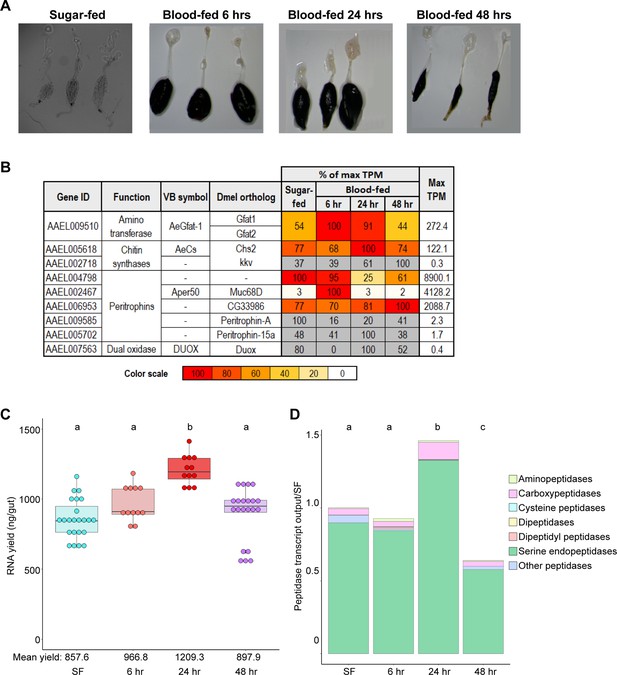
Increasing RNA yield drives higher gut peptidase output at 24 hr post blood meal.
(A) Dissected guts from sugar-fed mosquitoes and mosquitoes at 6, 24, and 48 hrs pbm. (B) Expression of selected genes associated with peritrophic matrix synthesis, scaled as a percentage of maximum expression. The region with the highest expression (in TPM) is scored as ‘100’, with its TPM displayed in the rightmost column. Cells for very low-expressed genes (Max TPM <5) are displayed in gray. (C) Estimates of gut’s transcriptional output in sugar-fed mosquitoes and at 6, 24, and 48 hrs pbm by empirical quantification of RNA yield; statistics: One-way ANOVA, Tukey HSD. (D) Estimated output of peptidases by category (categorical investments weighted by RNA yield, scaled to SF output); statistics: One-way ANOVA, Tukey HSD.
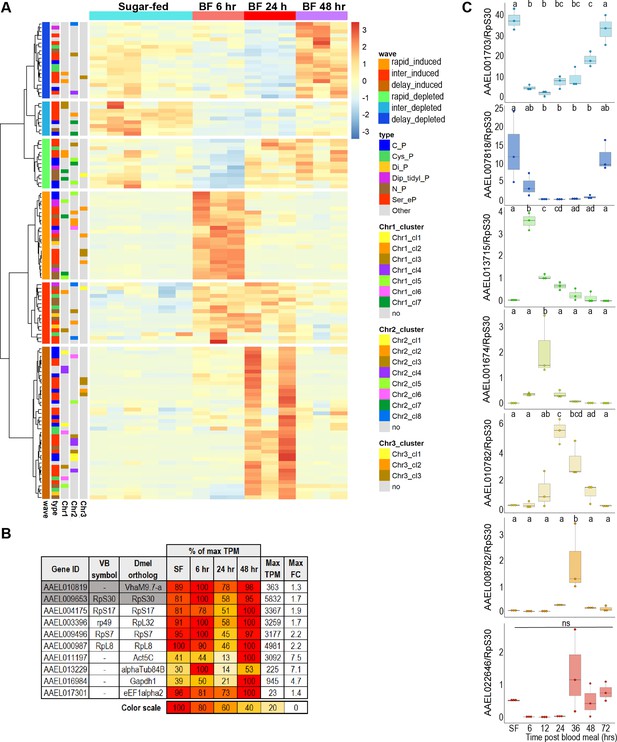
Gut peptidase transcripts are induced and depleted asynchronously during blood meal digestion.
(A) Clustering analysis of the expression of peptidases across all timepoints of blood feeding time series. (B) Comparison of candidate reference genes for RT-qPCR analysis of peptidases in blood-fed guts; expression values are scaled as a percentage of the maximum expression observed. The condition with the highest expression (in TPM) is scored as 100, with its TPM displayed in the ‘Max TPM’ column. ‘Max FC’ column displays the variation (in fold-change) across all conditions. Genes in white are previously published reference genes; genes in gray are candidate reference genes identified in this study. (C) Unscaled results of RT-qPCR for selected peptidases relative to reference gene (AAEL009653); 3 replicates; statistics: One-way ANOVA, Tukey HSD.
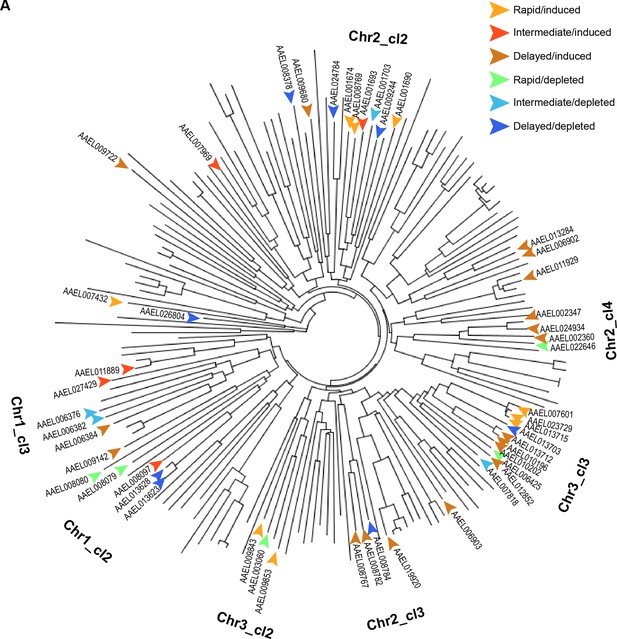
Temporal clustering of serine endopeptidases is independent of physical proximity and sequence similarity.
(A) Phylogenetic tree for serine endopeptidases in Aedes aegypti with regulated genes labeled by temporal cluster; Note: superimposed chromosomal cluster labels indicate where a plurality of genes from that locus are located on the diagram, but not all genes proximal to a label belong to that chromosomal locus.
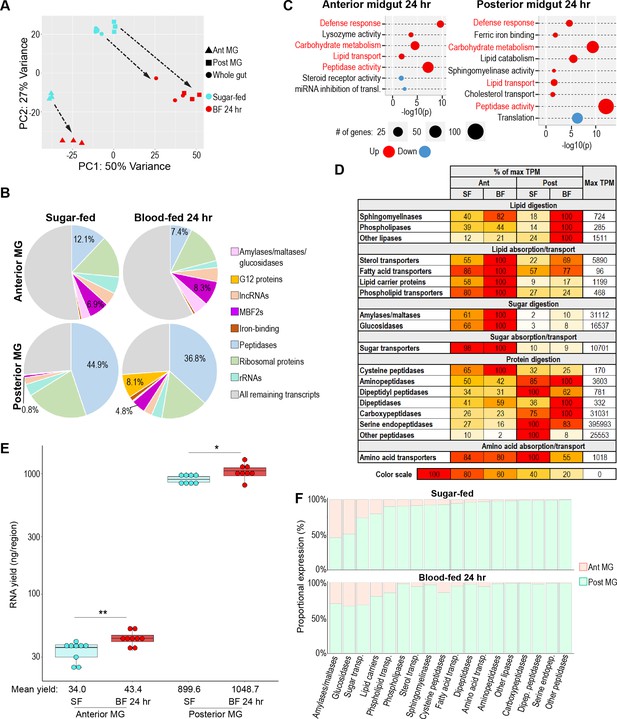
Regional specializations in sugar and protein digestion are preserved in the blood-fed midgut.
(A) Principal component analysis of the transcriptomes of sugar-fed and 24 hr blood-fed whole guts and anterior and posterior midguts; 3–6 replicates per condition. (B) Proportional expression (out of whole transcriptome) of selected gene categories/families by region/condition. (C) Classic Fisher topGO gene ontology enrichment analysis of blood-fed versus sugar-fed regions (DESeq2, padj <0.05, minimum of 2 x fold-change). Categories that are regulated in both regions are shown in red. (D) Investments in categories of digestive enzymes and transporters by region/condition. Colored cells are scaled as percentages of the region/condition with the highest transcriptional investment in the category (as measured by cumulative TPM). The treatment with the highest investment is scored as ‘100’, with its cumulative TPM displayed in the rightmost column. (E) Estimates of midgut regions’ transcriptional yield by empirical quantification of RNA in sugar-fed guts versus 24 hr after feeding with an artificial blood replacement diet; statistics: unpaired t-test. (F) Estimated output of digestive enzymes/transporters by midgut region in sugar-fed and blood-fed mosquitoes (categorical investments weighted by regional RNA yield).
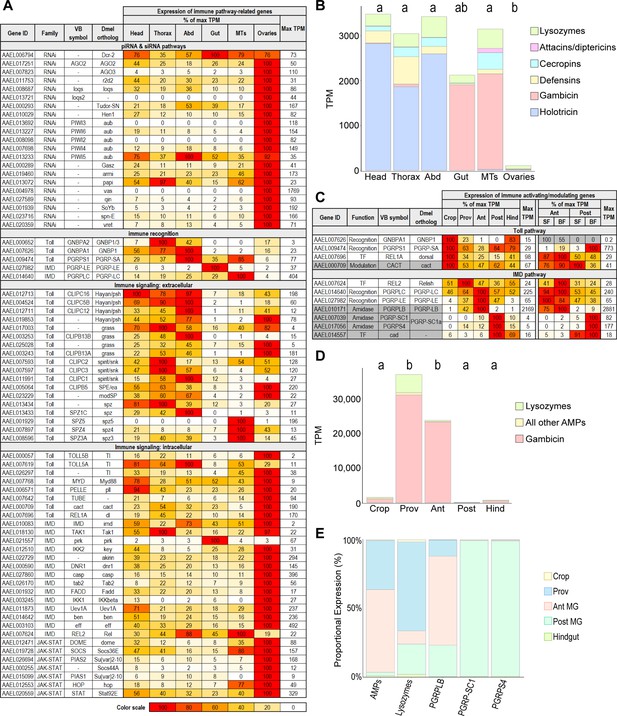
Immune gene patterning reveals zones of immune activity and tolerance in the mosquito body and gut.
(A) Body parts’ investments in immune pathway-related genes, scaled as a percentage of maximum expression. The body part with the highest expression (in TPM) is scored as ‘100’ with its TPM displayed in the rightmost column. Note: immune-related genes may serve an alternate, developmental role in ovaries. (B) Cumulative antimicrobial peptide expression by body part (TPM); statistics: One-way ANOVA, Tukey HSD. (C) Expression of immune activating (white) /modulating (gray) genes, scaled as a percentage of maximum expression. The region with the highest expression (in TPM) is scored as ‘100’ with its TPM displayed in the rightmost column of each half of the table. For genes with very low expression (Max TPM <1) color scale is replaced with gray. (D) Cumulative antimicrobial peptide expression by gut region (TPM); statistics: One-way ANOVA, Tukey HSD. (E) Output of immune-related genes and gene categories by gut region (TPM weighted by regional RNA yield).
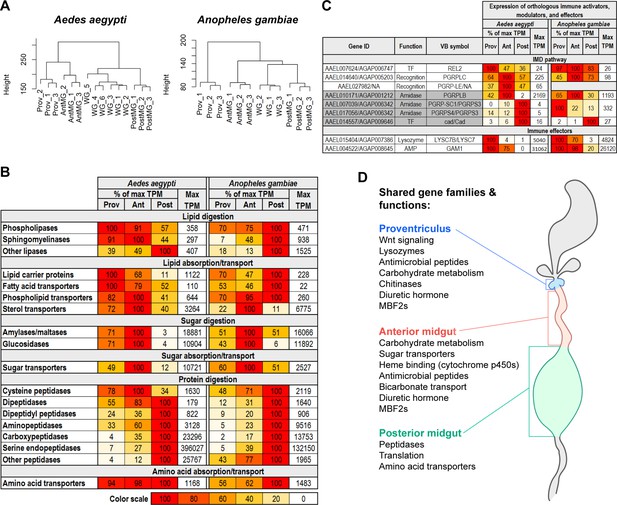
Digestive and defensive regional specializations are well conserved between the Aedes aegypti and Anopheles gambiae midguts.
(A) Clustering analyses of the transcriptomes of Aedes aegypti and Anopheles gambiae whole gut and midgut regions; 3–6 replicates (B) Regional investments in categories of digestive enzymes and transporters. Colored cells are scaled as percentages of the region with the highest transcriptional investment in the category (as measured by cumulative TPM). The region with the highest investment is scored as ‘100’, with its cumulative TPM displayed in the rightmost column of each half of the table. (C) Expression of immune activating (white)/modulating (gray) genes and effectors, scaled as a percentage of maximum expression. The region with the highest expression (in TPM) is scored as ‘100’ with its TPM displayed in the rightmost column of each half of the table. (D) Conserved regional enrichment of gene families and functions in Ae. aegypti and An. gambiae midgut regions, as evaluated by classic Fisher topGO gene ontology enrichment analysis, investment analysis, and a comparison of the twenty highest expressed transcripts.
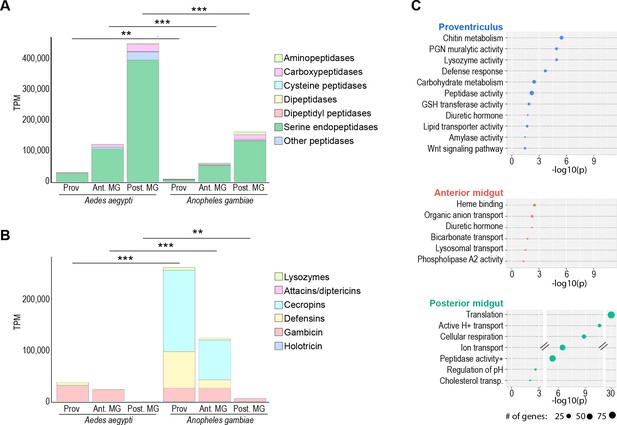
Midgut regions in Aedes aegypti and Anopheles gambiae display similar regional specializations, but disparate amplitudes of investment in key functions.
Midgut regional investments in (A) digestive peptidases and (B) Antimicrobial peptides and lysozymes in Ae. aegypti and An. gambiae; statistics: unpaired t-test. (C) Classic Fisher topGO gene ontology enrichment analysis of regionally enriched genes (for proventriculus, and anterior midgut: 5 x enriched over whole-gut, DESeq2 padj <0.05; for posterior midgut: enriched over other midgut regions, DESeq2 padj <0.05).
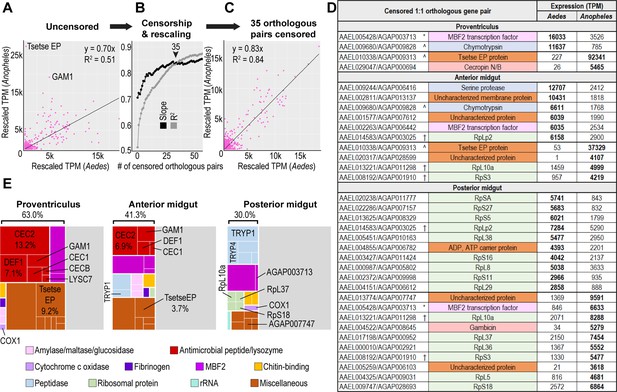
A small number of disparately expressed genes constitute a ‘species signature’ differentiating midgut transcriptomes in Aedes aegypti and Anopheles gambiae.
(A–C) Correlation of one-to-one orthologous genes before and after censorship of the 35 most disparately expressed orthologous pairs. (D) One-to-one orthologs censored in a regional correlation analysis; (^) signifies orthologous pairs censored in both proventriculus and anterior midgut, (†) signifies orthologous pairs censored in both anterior midgut and posterior midgut (*) signifies orthologous pairs censored in both proventriculus and posterior midgut. (E) 20 highest expressed transcripts per region, grouped by category/function; percentages reflect the proportion of the transcriptome comprised by the displayed genes.
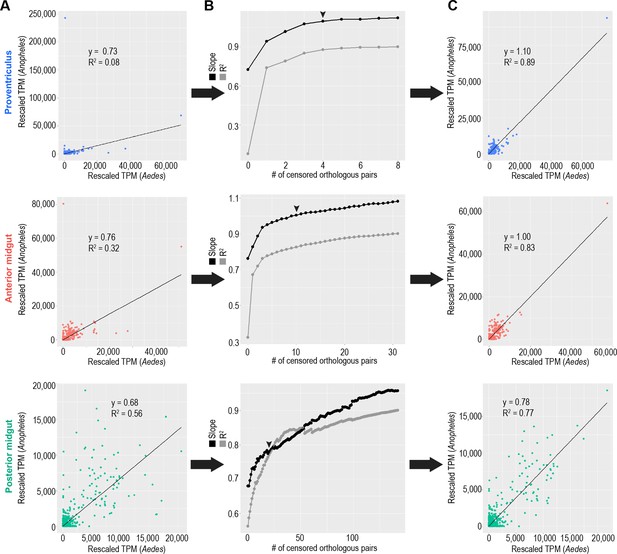
A small number of highly/disparately transcribed genes obscure the transcriptional correlation between one-to-one orthologous genes in Aedes aegypti and Anopheles gambiae midgut regions.
(A) Correlation of expression of one-to-one orthologous genes in the Aedes aegypti and Anopheles gambiae midgut regions. (B) Plotted slopes and correlation coefficients for correlations of one-to-one orthologous genes during a process of sequential censoring of disparately expressed orthologs and re-scaling of TPMs; Black arrows indicate the number of genes censored in: (C) Adjusted correlation plots reflecting the relationships of one-to-one orthologs post-censorship/rescaling.
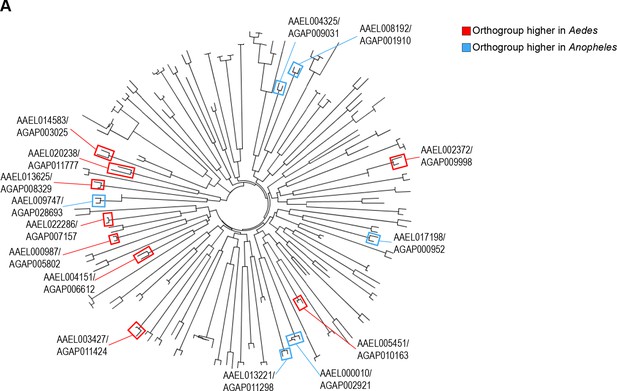
Phylogenetic analysis confirms disparately expressed one-to-one orthologous ribosomal proteins are sister sequences.
(A) Geneious phylogenetic tree depicting the evolutionary relatedness of ribosomal proteins in Aedes aegypti and Anopheles gambiae. Pairs of censored one-to-one orthologs from the correlational analysis are shown in colored boxes. A red box indicates that the Aedes gene was higher expressed compared to the Anopheles gene. A blue box indicates that the Anopheles gene was higher expressed.
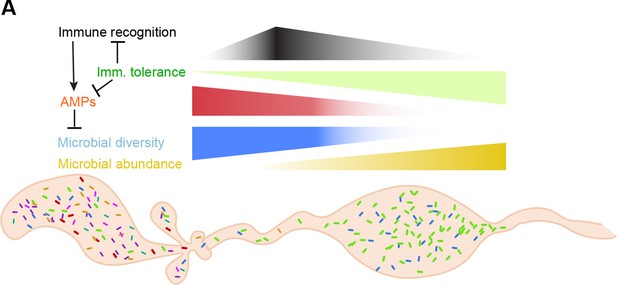
A model for regionalized microbe selection and immune tolerance in the mosquito gut.
(A) A model of the proposed effects of regional specializations in immune recognition/antimicrobial peptide expression (proventriculus/anterior midgut) and tolerance (posterior midgut).
Tables
2x | 5x | |
---|---|---|
Head | *2664 | 1376 |
Thorax | *2271 | 761 |
Abdomen | 1871 | 359 |
Gut | *2163 | 985 |
MT | *2567 | 1193 |
Ovaries | 555 | *44 |
Crop | *2501 | 1427 |
Prov | 1234 | 414 |
Ant | 757 | 188 |
Post | *3 | *1 |
Hind | 1449 | 620 |
2x | 5x | |
---|---|---|
Upregulated | ||
6 vs SF | 828 | 228 |
24 vs 6 | 911 | 381 |
48 vs 24 | 469 | *55 |
48 vs SF | 102 | *1 |
BF post vs Post | 989 | 409 |
BF ant vs Ant | 317 | *95 |
Downregulated | ||
6 vs SF | 1048 | 371 |
24 vs 6 | 741 | 170 |
48 vs 24 | 569 | 268 |
48 vs SF | *85 | *10 |
BF post vs Post | 737 | 135 |
BF ant vs Ant | 525 | 108 |
Additional files
-
Supplementary file 1
TPMs for putative tissue markers in major body parts of Aedes aegypti.
- https://cdn.elifesciences.org/articles/76132/elife-76132-supp1-v2.xlsx
-
Supplementary file 2
Gene ontology categories enriched in Aedes aegypti body parts, gut regions, and blood-fed guts; and in Anopheles gambiae (s.l.) midgut regions.
- https://cdn.elifesciences.org/articles/76132/elife-76132-supp2-v2.xlsx
-
Supplementary file 3
Index of VectorBase IDs for genes named in the text.
- https://cdn.elifesciences.org/articles/76132/elife-76132-supp3-v2.xlsx
-
Supplementary file 4
TPMs for digestive enzymes and transporters expressed in the Aedes aegypti gut.
- https://cdn.elifesciences.org/articles/76132/elife-76132-supp4-v2.xlsx
-
Supplementary file 5
Relative expression (investment) and TPMs for immune-related genes in Aedes aegypti body parts, gut regions, and blood-fed guts; and in Anopheles gambiae (s.l.) midgut regions.
- https://cdn.elifesciences.org/articles/76132/elife-76132-supp5-v2.xlsx
-
Supplementary file 6
TPMs for digestive enzymes and transporters expressed in the Aedes aegypti midgut.
- https://cdn.elifesciences.org/articles/76132/elife-76132-supp6-v2.xlsx
-
Supplementary file 7
TPMs for digestive enzymes and transporters expressed in the Anopheles gambiae (s.l.) midgut.
- https://cdn.elifesciences.org/articles/76132/elife-76132-supp7-v2.xlsx
-
Supplementary file 8
Output of orthogroups analysis relating genes from Aedes aegypti and Anopheles gambiae.
- https://cdn.elifesciences.org/articles/76132/elife-76132-supp8-v2.xlsx
-
Supplementary file 9
Details of replicates for RNAseq experiments in Aedes aegypti and Anopheles gambiae (s.l.).
- https://cdn.elifesciences.org/articles/76132/elife-76132-supp9-v2.xlsx
-
Supplementary file 10
Sequences of primers for RTqPCR assays.
- https://cdn.elifesciences.org/articles/76132/elife-76132-supp10-v2.xlsx