Recombinant single-cycle influenza virus with exchangeable pseudotypes allows repeated immunization to augment anti-tumour immunity with immune checkpoint inhibitors
Figures
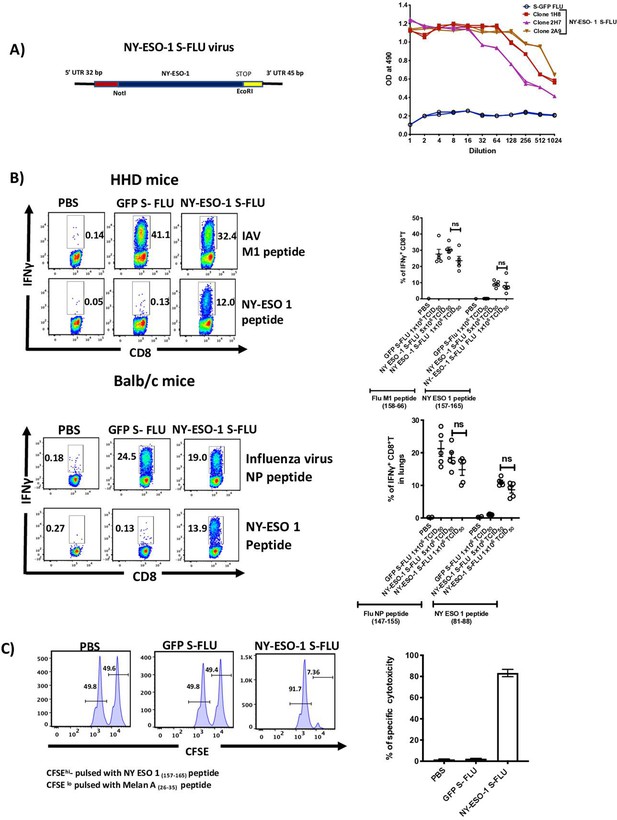
Intranasal infection with NY-ESO-1 S-FLU virus elicits a potent CTL response.
(A) Left: schematic diagram of the design for NY-ESO-1 S-FLU virus. The codon-optimized NY-ESO-1 cDNA sequence between unique NotI site and EcoRI sites in the pPol/S-UL expression cassette surrounded HA packaging sequences. Right: NY-ESO-1 expression in NY-ESO-1 S-FLU-infected MDCK-SIAT1 cells. MDCK-SIA1 cells expressing HA from PR8 were infected with different clones of NY-ESO-1 S-FLU virus or GFP S-FLU virus in twofold serial dilutions and the expression of NY-ESO-1 was analysed after 48 hr. Three positive S-FLU clones were identified, clone 2A9 was chosen and expanded for further experiments. (B) Left: representative flow cytometry dot plots showing the frequency of IFNγ-secreting CD8+ T cells. HHD mice (upper panel) and BALB/c mice (lower panel) were intranasally infected with 1 × 106 TCID50 of GFP S-FLU or 3 × 106 TCID50 and 1 × 106 TCID50 of NY-ESO-1 S-FLU virus on day 0. CTL responses in lungs were analysed on day 10 post infection by ex vivo stimulation with HLA-A2-restricted influenza A virus (IAV) M1 peptide 158-66(GILGFVFTL) or NY-ESO-1 peptide 157-65(SLLMWITQC) and H-2Kd binding IAV NP peptide 147-55(TYQRTRALV) or H-2Dd-restricted NY-ESO-1 peptide 81-88(RGPESRLL). Right panel: bar chart showing the percentage of IFNγ-secreting CD8+ T cells in HHD mice (upper panel) and BALB/c mice (lower panel) lungs. (C) In vivo analysis of cytotoxic T cell functions. Left: representative FACS plots for in vivo killing assay. HHD mice were intranasally infected with 1 × 106 TCID50 of GFP- S-FLU or NY-ESO-1 S-FLU virus, and on day 10 post infection, mice received adoptively transferred CFSE-labelled (CFSEhi) NY-ESO-1157-65 peptide-pulsed splenocytes, and CFSE-labelled (CFSElo) Melan A26-35 peptide-pulsed splenocytes, and CFSE-labelled splenocytes were analysed in spleen after 8 hr. Right: bar chart showing the percentage of NY-ESO-1-specific cytotoxicity in infected mice. The values are expressed as mean ± SEM. Data in (B) is representative of at least two independent experiments. ns, not significant.
-
Figure 1—source data 1
Frequency of antigen specific CD8+T cells in HHD mice.
- https://cdn.elifesciences.org/articles/76414/elife-76414-fig1-data1-v1.xlsx
-
Figure 1—source data 2
Frequency of antigen specific CTL response in Balb/c mice.
- https://cdn.elifesciences.org/articles/76414/elife-76414-fig1-data2-v1.xlsx
-
Figure 1—source data 3
In-vivo killing assay.
- https://cdn.elifesciences.org/articles/76414/elife-76414-fig1-data3-v1.xlsx
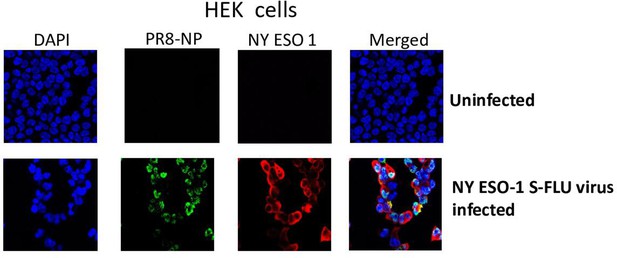
Expression of PR8-NP and NY-ESO-1 proteins in NY-ESO-1 S-FLU virus-infected HEK cells.
HEK cells were infected with NY-ESO-1 S-FLU virus at a multiplicity of infection (MOI) of 3, and the cells were fixed and permeabilized after 18 hr of infection followed by staining with DAPI and immune stained for NP and NY-ESO-1 proteins.
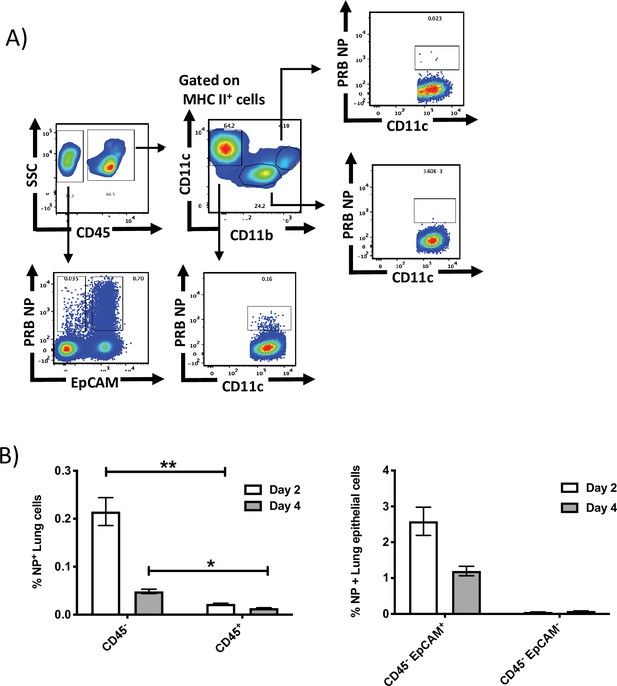
Intranasally administered NY-ESO-1 S-FLU virus mainly infects lung epithelial cells.
(A) Representative FACS plot showing the infected lung cell subsets on day 2 post infection. BALB/c mice were intranasally infected with NY-ESO-1 S-FLU virus, and lung cell subsets were analysed for virus infection by PR8-NP staining. (B) Bar graph (left) showing the frequency of NP+ non-immune cells (CD45-) and immune cells (CD45+) in lungs following infection with NY-ESO-1 S-FLU virus on days 2 and 4. Bar graph (right) showing the frequency of NP+ lung epithelial cells on days 2 and 4 post infection. Data in Figure S2 is representative of two independent experiments with similar results. *p<0.05, **p<0.01 (two-tailed Student’s t-test). Error bars: mean ± SEM.
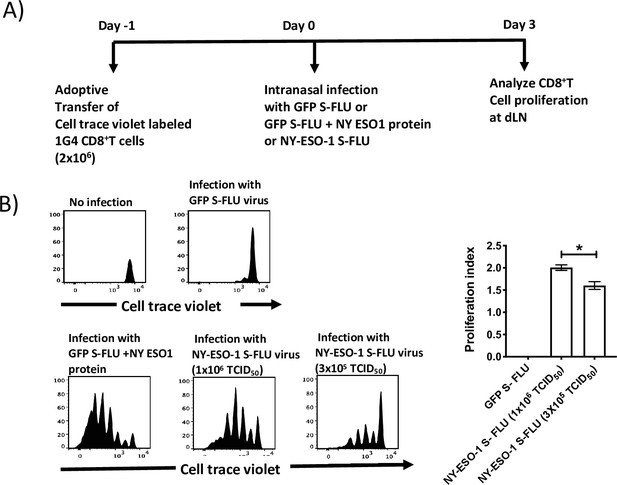
In vivo T cell priming at draining lymph node following infection with NY-ESO-1 S-FLU virus.
CellTrace Violet (CTV)-labelled NY-ESO-1-specific CD8+ T cells (1G4) were adoptively transferred to HHD mice followed by infection either with GFP-S-FLU (S-FLU-expressing GFP reporter protein) or NY-ESO-1 S-FLU virus, and the proliferation was analysed on day 3 post infection. (A) Experimental design. n = 5–6 mice per group. Data are representative of at least two independent experiments with similar results. (B) Histograms represent the analysis of CTV-labelled 1G4 CD8+ T cells proliferation at mediastinal lymph node 3 days post infection with GFP S-FLU or NY-ESO-1 S-FLU virus. The graph on the right shows the respective proliferation index for the proliferating 1G4 CD8+ T cells. *p<0.05 (two-tailed Student’s t-test). Error bars: mean ± SEM.
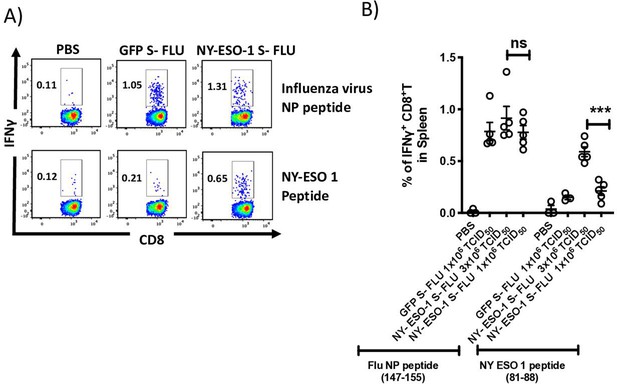
Intranasal infection with NY-ESO-1 S-FLU virus elicits specific CTL response in spleen.
(A) Representative FACS plots showing IFNγ-secreting CD8+ T cells in spleen. On day 10 post intranasal infection, splenocytes were stimulated ex vivo with influenza A virus (IAV) NP peptide or NY-ESO-1 CTL peptide and IFNγ-secreting CD8+ T cells were analysed. (B) Bar graph showing the frequencies of IFNγ-secreting CD8+ T cells in spleen following ex vivo stimulation IAV NP peptide or NY-ESO-1 CTL peptide. The results shown are representative of three independent experiments with similar results (n = 4–5 mice/group). ***p<0.001, ns, not significant (two-tailed Student’s t test). Error bars: mean ± SEM.
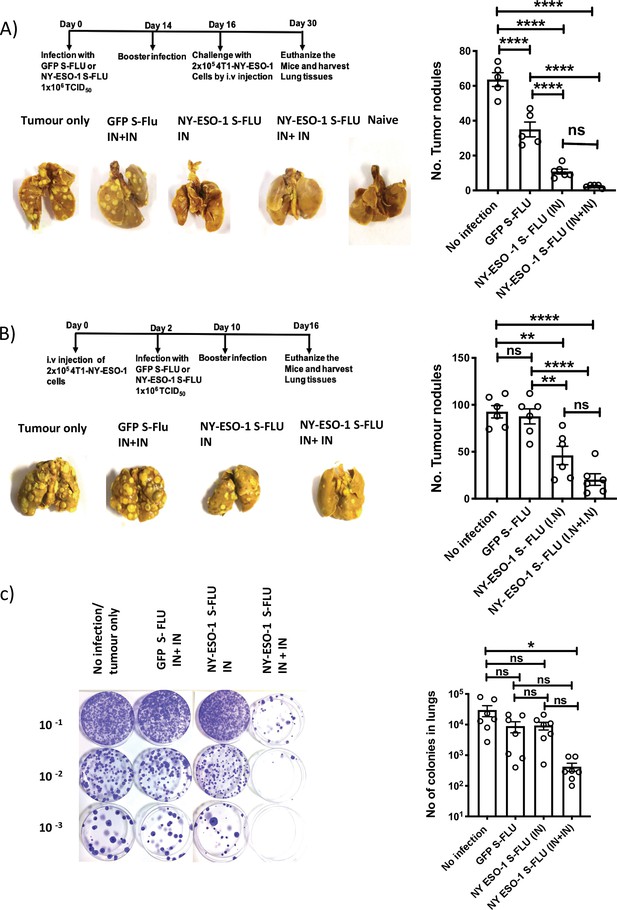
Intranasal infection with NY-ESO-1 S-FLU virus protects mice from tumour development in lungs.
(A) Left: prophylactic tumour model – photographs of the lungs of the mice immunized with NY-ESO-1 S-FLU or GFP S-FLU virus followed by tumour challenge with 4-T1-NY-ESO-1 cells. Right: a bar chart representing the number of tumour nodules enumerated in the lungs. (B) Left: therapeutic tumour model – photographs of the lungs of the mice intravenously injected with 4-T1-NY-ESO-1 cells followed by the treatment with NY-ESO-1 S-FLU or GFP S-FLU virus. Right: a bar graph representing the number of tumour nodules enumerated in the lungs. Photographs are representative of five mice in two different experiments with similar results. (C) Spontaneous tumour metastasis model/clonogenic assay. Left: photographs showing the spontaneous metastasis of 4T1-NY-ESO-1 cells to lungs. 4T1-NY-ESO-1 cells were injected subcutaneously in BALB/c mice, and mice were intranasally infected with GFP S-FLU or NY-ESO-1 S-FLU virus on days 4 and 18 post tumour cell injection. On days 26–28 post tumour cell injection, mice were euthanized and lung cells were plated in 6-thioguonine media. The number of colonies was counted, and the right panel shows the bar chart representing the number of colonies in lungs of the mice treated with GFP S-FLU or NY-ESO-1 S-FLU virus. The results shown in (A) and (B) are representative of two independent experiments with similar results (n = 5–6 mice/group). Data shown in (C) are pooled results of two independent experiments (n = 7 mice/ group). *p<0.05, **p<0.01, ***p<0.001, ****p<0.0001, ns, not significant (one-way ANOVA multiple comparisons). Error bars: mean ± SEM.
-
Figure 2—source data 1
Number of tumour nodules.
- https://cdn.elifesciences.org/articles/76414/elife-76414-fig2-data1-v1.xlsx
-
Figure 2—source data 2
Number of tumour nodules.
- https://cdn.elifesciences.org/articles/76414/elife-76414-fig2-data2-v1.xlsx
-
Figure 2—source data 3
Number of colonies.
- https://cdn.elifesciences.org/articles/76414/elife-76414-fig2-data3-v1.xlsx
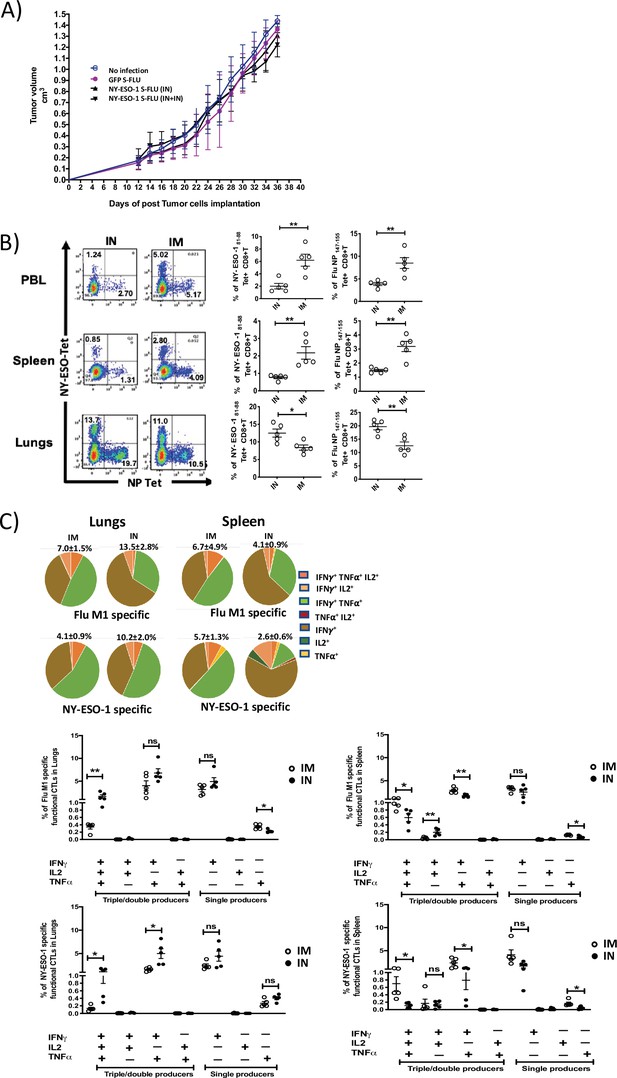
Intranasal infection with NY-ESO-1 S-FLU virus fails to protect the mice from subcutaneous tumour challenge and elicits only a modest systemic T cell response.
(A) Tumour growth of 4T1-NY-ESO-1-bearing mice. BALB/c mice were subcutaneously injected at right flank with 2 × 105 cultured 4T1-NY-ESO-1 cells on day 0. On days 4 and 18, mice were intranasally infected with GFP S-FLU or NY-ESO-1 S-FLU virus. Tumour volumes were monitored every other day. (B) NP- or NY-ESO-1-specific CTL responses in blood, lungs, and spleen following intranasal or intramuscular infection. BALB/c mice were intranasally or intramuscularly infected with NY-ESO-1 S-FLU virus, and PR8-NP- or NY-ESO-1-specific CTL responses were analysed by tetramer staining. Left: representative FACS plots showing the percentage of NP- or NY-ESO-1-specific CD8+ T cells in peripheral blood leukocytes (PBL), spleen, and lungs. Right: the bar graphs display the frequency of NP- or NY-ESO-1-specific CD8+ T cells. (C) Top panel shows the pie charts representing the frequencies of Flu M1-specific or NY-ESO-1-specific polyfunctional CD8+ T cells (triple cytokines or double cytokine producers) in lungs or spleen. HHD mice were intranasally or intramuscularly infected with NY-ESO-1 S-FLU virus and Flu M1-specific or NY-ESO-1-specific polyfunctional CD8+ T cells in lungs (left) and spleen (right) were analysed by ex vivo peptide stimulation on day 10 post infection. Middle panel (Flu-M1 specific) and lower panel (NY-ESO-1 specific) show the bar graphs displaying the frequencies of polyfunctional CD8+ T cells in lungs (left) and spleen (right). The results shown are representative of two independent experiments with similar results (n = 4–5 mice/group). *p<0.05, **p<0.01, ns, not significant (two tailed Student’s t-test). Error bars: mean ± SEM.
-
Figure 3—source data 1
Frequency of polyfunctional NY-ESO-1 specific CD8+T cells in spleen.
- https://cdn.elifesciences.org/articles/76414/elife-76414-fig3-data1-v1.xlsx
-
Figure 3—source data 2
Frequency of polyfunctional NY-ESO-1 specific CD8+T cells in lungs.
- https://cdn.elifesciences.org/articles/76414/elife-76414-fig3-data2-v1.xlsx
-
Figure 3—source data 3
Frequency of polyfunctional FLU-M1 specific CD8+T cells in spleen.
- https://cdn.elifesciences.org/articles/76414/elife-76414-fig3-data3-v1.xlsx
-
Figure 3—source data 4
Frequency of polyfunctional FLU M1 specific CD8+T cells in lungs.
- https://cdn.elifesciences.org/articles/76414/elife-76414-fig3-data4-v1.xlsx
-
Figure 3—source data 5
Frequency of FLU NP specific CD8+T cell response in Balb/c mice.
- https://cdn.elifesciences.org/articles/76414/elife-76414-fig3-data5-v1.xlsx
-
Figure 3—source data 6
Frequency of NY-ESO-1 specific CD8+T cell response in Balb/c mice.
- https://cdn.elifesciences.org/articles/76414/elife-76414-fig3-data6-v1.xlsx
-
Figure 3—source data 7
Frequncy of FLU-NP specific CD8+T cell reponse in spleen.
- https://cdn.elifesciences.org/articles/76414/elife-76414-fig3-data7-v1.xlsx
-
Figure 3—source data 8
Frequency of NY-ESO-1 specific CD8+T cell response in spleen.
- https://cdn.elifesciences.org/articles/76414/elife-76414-fig3-data8-v1.xlsx
-
Figure 3—source data 9
Frequency of NP specific CD8+T cell responnse in PBL.
- https://cdn.elifesciences.org/articles/76414/elife-76414-fig3-data9-v1.xlsx
-
Figure 3—source data 10
Frequency of NY-ESO-1 specific CD8+T cell response in PBL.
- https://cdn.elifesciences.org/articles/76414/elife-76414-fig3-data10-v1.xlsx
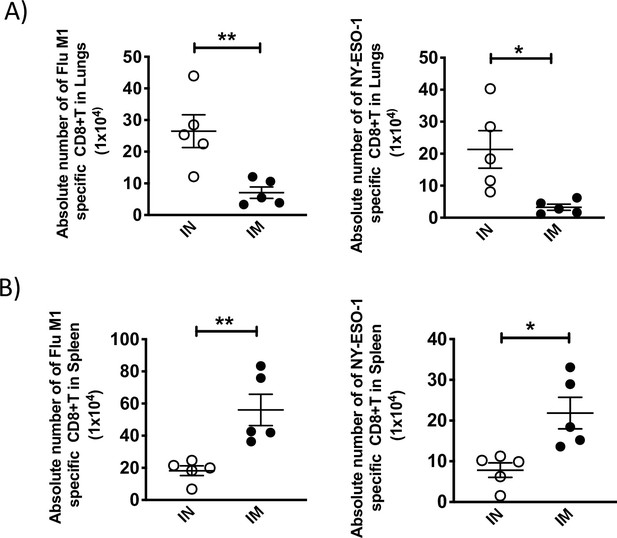
Absolute number of NY-ESO-1-specific CD8+ T cells in lungs and spleen following intranasal or intramuscular infection.
(A) Bar chart showing the absolute number of IFNγ-producing CD8+ T cells in lungs in ex vivo stimulation with Flu M1 peptide (left) or NY-ESO-1 peptide (right). (B) Bar chart showing the absolute number of IFNγ-producing CD8+ T cells in spleen in ex vivo stimulation with Flu M1 peptide (left) or NY-ESO-1 peptide (right). HHD mice were intranasally or intramuscularly infected with NY-ESO-1 S-FLU virus and Flu M1-specific or NY-ESO-1-specific IFNγ-producing CD8+ T cells in lungs (left) and spleen (right) were analysed by ex vivo peptide stimulation on day 10 post infection. *p<0.05, **p<0.01 (two-tailed Student’s t-test). Error bars: mean ± SEM.
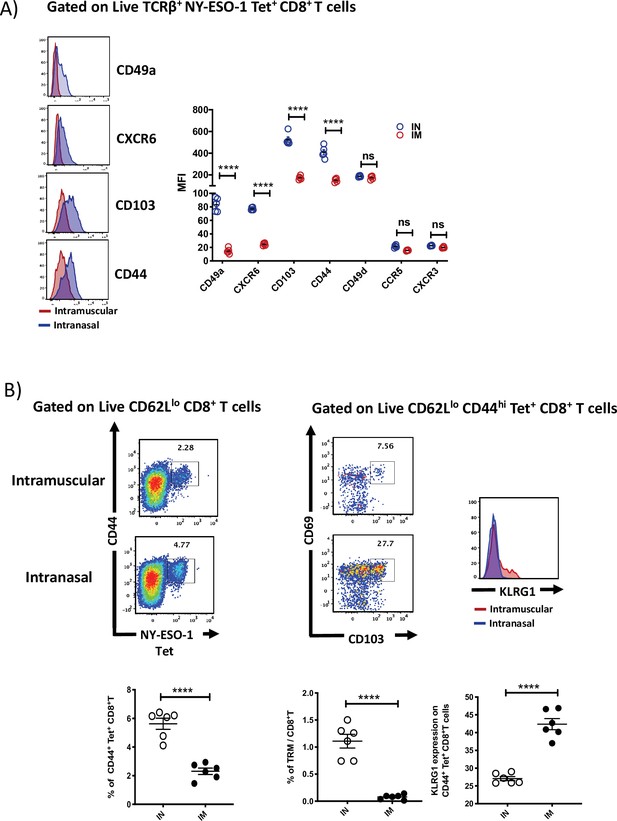
Intranasal infection induces tissue retention signals and generates a higher frequency of tissue-resident memory CD8+ T cells.
(A) Left: representative histograms showing the expression of tissue retention molecules CD49a, CXCR6, CD103, and CD44 on NY-ESO-1 tetramer-positive lung CD8+ T cells. Right: bar graphs showing the quantification of mean fluorescence index of CD49a, CXCR6, CD103, CD44, CD49d, CCR5, and CXCR3 expressed by NY-ESO-1-specific CD8+ T cells in lungs. BALB/c mice were intranasally or intramuscularly infected with NY-ESO-1 S-FLU virus, and day 10 post infection, NY-ESO-1-specific CD8+ T cells were analysed for various tissue retention molecules expression. The results shown are representative of two independent experiments with similar results (n = 5–6 mice/group). (B) Compared to intranasal infection, intramuscular infection results in decreased number of tissue-resident memory T cells in the lung parenchyma. BALB/c mice were intranasally or intramuscularly infected with NY-ESO-1 S-FLU virus, and on day 35 post infection, the frequency of lung-resident memory CD8+ T cells was analysed by flow cytometry. Left: representative FACS plots (gated on CD8β- CD8α+ CD62Llo) showing CD44hi NY-ESO-1 tetramer+ CD8+ T cells, and the FACS plots on the right display further analysis of CD44hi NY-ESO-1 tetramer+ CD8+ T cells for the expression of resident memory T cell markers CD69 CD103 and KLRG1 (far-right histogram). Lower panel displays the bar graphs showing the percentage of tetramer-positive CD8+ T cells, tissue-resident memory CD8+ T cells among the total number of CD8+ T cells, and the percentage of KLRG1 expression on tetramer-positive CD8+ T cells. ****p<0.0001, ns, not significant (two-tailed Student’s t-test). Error bars: mean ± SEM.
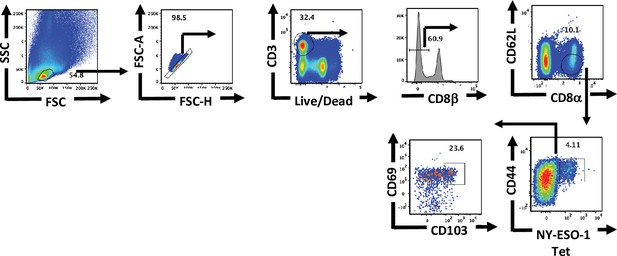
Gating strategy for flow cytometric analysis of lung tissue-resident memory T cells.
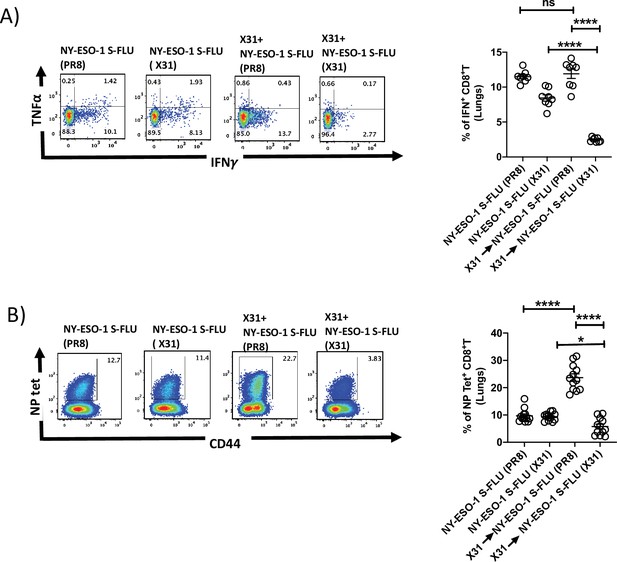
Infection with HA-switched NY-ESO-1 S-FLU virus overcomes the inhibitory effect of pre-existing neutralizing antibody.
(A, left) Representative FACS plot showing IFNγ- and TNFα-secreting and (B, left) NP tetramer-positive CD8+ T cells in lungs. Bar graph shows the quantification of IFNγ-secreting CD8+ T cells (A, right) and NP tetramer-positive CD8+ T cells (B, right) in lungs. BALB/c mice were intranasally infected with X31 virus and reinfected with NY-ESO-1 S-FLU (X31) or NY-ESO-1 S-FLU (PR8) on day 24 post infection, and NY-ESO-1- and NP-specific CD8+ T cell responses in lungs were analysed on day 7 post secondary infection by ex vivo stimulation with NY-ESO-1 CTL peptide 81-88(RGPESRLL) and NP tetramer staining, respectively. Data shown in (A) and (B) are pooled results of two (n = 8 mice/group) and three independent experiments (n = 12 mice/group), respectively. *p<0.05, ****p<0.0001, ns, not significant (one-way ANOVA multiple comparisons). Error bars: mean ± SEM.
-
Figure 4—source data 1
Frequency of NY-ESO-1 specific IFNg+ CD8+T cells in lungs.
- https://cdn.elifesciences.org/articles/76414/elife-76414-fig4-data1-v1.xlsx
-
Figure 4—source data 2
Frequency of NP specific IFNg+ CD8+T cells.
- https://cdn.elifesciences.org/articles/76414/elife-76414-fig4-data2-v1.xlsx
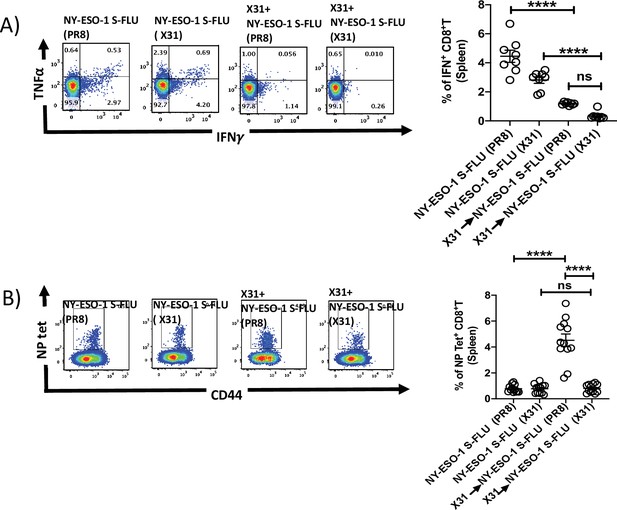
Infection with HA-switched NY-ESO-1 S-FLU virus elicited a stronger NP-specific CTL responses in spleen.
(A, left) Representative FACS plot showing IFNγ- and TNFα-secreting and (B, left) NP tetramer-positive CD8+ T cells in spleen. Bar graph shows the quantification of IFNγ-secreting CD8+ T cells (A, right) and NP tetramer-positive CD8+ T cells (B, right) in spleen. BALB/c mice were intranasally infected with X31 virus and reinfected with NY-ESO-1 S-FLU (X31) or NY-ESO-1 S-FLU (PR8) on day 24 post infection, and NY-ESO-1- and NP-specific CD8+ T cell responses in spleen were analysed on day 7 post secondary infection by ex vivo stimulation with NY-ESO-1 CTL peptide 81-88(RGPESRLL) and NP tetramer staining, respectively. Data shown in (A) and (B) are pooled results of two (n = 8 mice/group) and three independent experiments (n = 12 mice/group), respectively. ****p<0.0001, ns, not significant (one-way ANOVA multiple comparisons). Error bars: mean ± SEM.
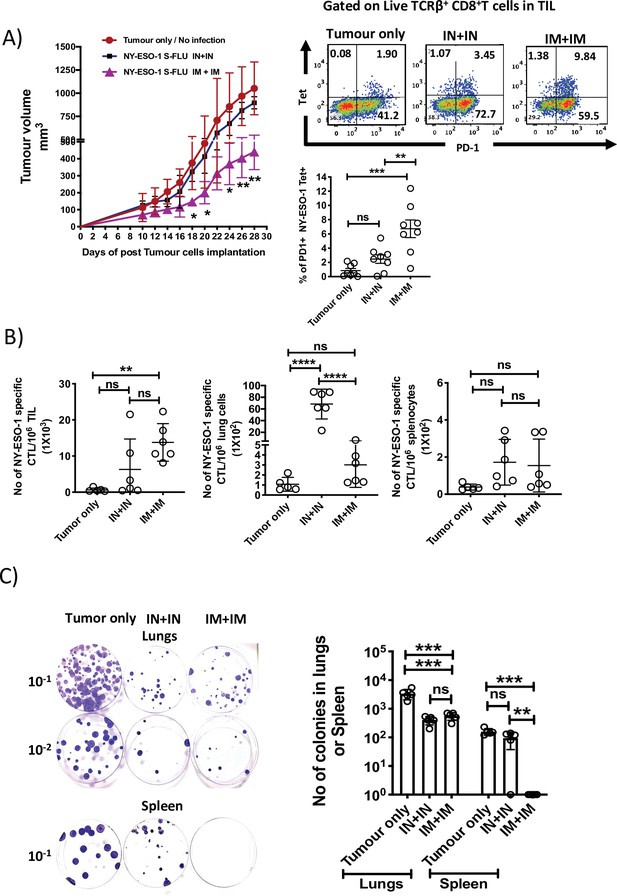
Intramuscular injection of NY-ESO-1 S-FLU virus induces a higher infiltration of NY-ESO-1-specific CD8+ T cells at tumour site and reduces tumour burden.
(A) Intramuscular infection with NY-ESO-1 S-FLU virus reduces tumour burden. BALB/c mice were subcutaneously injected with 4T1-NY-ESO-1 cells, and day 4 post injection, mice were intranasally or intramuscularly infected with NY-ESO-1 S-FLU virus followed by booster infection on day 18. Tumour growth was monitored over time. Tumour volume measured 28 days post inoculation in uninfected vs. intranasal infection vs. intramuscular infection is shown (left). Right: the representative FACS plots of PD-1+ NY-ESO-1 tetramer+ CD8+ T cells infiltrating the tumour. Bar graph shows the frequencies of PD-1+ Tet+ CD8+ T cells in tumour-infiltrating leukocytes (TIL) in the mice received no infection or intranasal or intramuscular infection with NY-ESO-1 S-FLU virus. (B) Bar graphs show the number of NY-ESO-1 tetramer+ CD8+ T cells in TIL or lungs or spleen in tumour-bearing mice received no infection or intranasal or intramuscular infection. (C) Clonogenic assay: photographs (left) showing the colonies which represent the spontaneous metastasis of 4T1-NY-ESO-1 cells to lungs. On day 28 post tumour cells inoculation, mice were euthanized followed by digestion of lungs and spleen, and the cells were plated in 6-thioguonine media. The number of colonies was counted after 14 days of incubation. The bar chart (right) represents the number of colonies in lungs and spleen. The results shown are representative of two independent experiments with similar results (n = 4–6 mice/group). Data shown in bar graph in (A) are pooled results of two independent experiments (n = 8 mice/ group). *p<0.05, **p<0.01, ***p<0.001, ****p<0.0001, ns, not significant (one-way ANOVA multiple comparisons). Error bars: mean ± SEM.
-
Figure 5—source data 1
Tumour volumes (mm3) measured.
- https://cdn.elifesciences.org/articles/76414/elife-76414-fig5-data1-v1.xlsx
-
Figure 5—source data 2
Frequency of PD1+ NY-ESO-1 specific CD8+T cells in tumour.
- https://cdn.elifesciences.org/articles/76414/elife-76414-fig5-data2-v1.xlsx
-
Figure 5—source data 3
Frequency of NY-ESO-1 specific CD8+T cells in spleen in tumour bearing mice.
- https://cdn.elifesciences.org/articles/76414/elife-76414-fig5-data3-v1.xlsx
-
Figure 5—source data 4
Frequency of NY-ESO-1 specific CD8+T cells in TLL in tumour bearing mice.
- https://cdn.elifesciences.org/articles/76414/elife-76414-fig5-data4-v1.xlsx
-
Figure 5—source data 5
Frequency of NY-ESO-1 specific CD8+T cells in lungs in tumour bearing mice.
- https://cdn.elifesciences.org/articles/76414/elife-76414-fig5-data5-v1.xlsx
-
Figure 5—source data 6
Number of colonies in lungs or spleen.
- https://cdn.elifesciences.org/articles/76414/elife-76414-fig5-data6-v1.xlsx
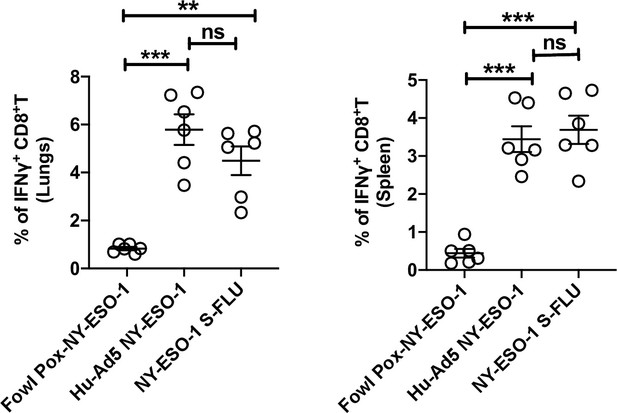
T cell responses elicited by intramuscular injection of different virus vaccines.
Bar graphs show the percentage of IFNγ-secreting CD8+ T cells in lungs (left) and spleen (right) in ex vivo stimulation of lung cells or splenocytes with NY-ESO-1 CTL peptide 81-88(RGPESRLL). BALB/c mice were intramuscularly infected with 1 × 107 TCID50 NY-ESO-1 S-FLU virus or 1 × 109 PFU Hu Ad5 NY-ESO-1 virus or 5 × 107 PFU Fowl Pox NY-ESO-1 virus, and NY-ESO-1-specific CTL responses were analysed in lungs and spleen on day 10 post infection. The results shown are representative of two independent experiments with similar results (n = 5–6 mice/group). **p<0.01, ***p<0.001, ns, not significant (one-way ANOVA multiple comparisons). Error bars: mean ± SEM.
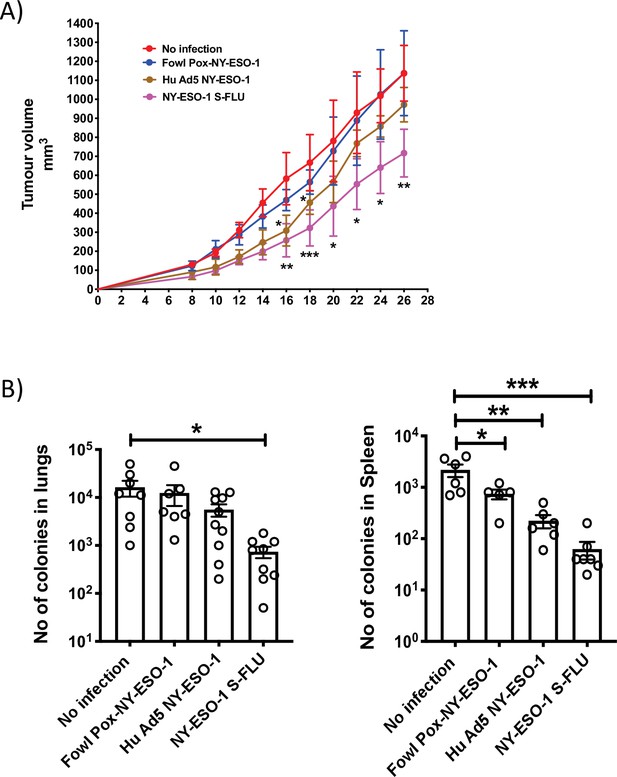
Comparison of the anti-tumour effect induced by NY ESO-1 S-FLU virus with contemporary recombinant virus vaccines.
(A) Intramuscular injection with NY-ESO-1 S-FLU virus demonstrates a better tumour reduction than Hu Ad5 NY-ESO-1 virus or Fowl Pox NY-ESO-1 virus. BALB/c mice were subcutaneously injected with 4T1-NY-ESO-1 cells, and on day 4 post injection, mice were intramuscularly infected with 5 × 107 PFU NY-ESO-1-expressing Fowl Pox virus or 1 × 109 PFU human adenovirus 5 or 6 × 106 TCID50 NY-ESO-1 S-FLU virus followed by same dose booster infection on day 18. Tumour growth was monitored over time. Tumour volume measured 28 days post inoculation in uninfected vs. NY-ESO-1 S-FLU vs. Hu Ad5 NY-ESO-1 vs. Fowl Pox NY-ESO-1 virus infection. (B) Clonogenic assay: bar graphs showing the number of colonies which represent spontaneous metastasis of 4T1-NY-ESO-1 cells to lungs (left) and spleen (right) in different virus infections described in (A). *p<0.05, ** p<0.01, ***p<0.001, ns, not significant (one-way ANOVA multiple comparisons). Error bars: mean ± SEM.
-
Figure 6—source data 1
Tumour volumes measured (mm3).
- https://cdn.elifesciences.org/articles/76414/elife-76414-fig6-data1-v1.xlsx
-
Figure 6—source data 2
Number of colonies in lungs.
- https://cdn.elifesciences.org/articles/76414/elife-76414-fig6-data2-v1.xlsx
-
Figure 6—source data 3
Number of colonies in spleen.
- https://cdn.elifesciences.org/articles/76414/elife-76414-fig6-data3-v1.xlsx
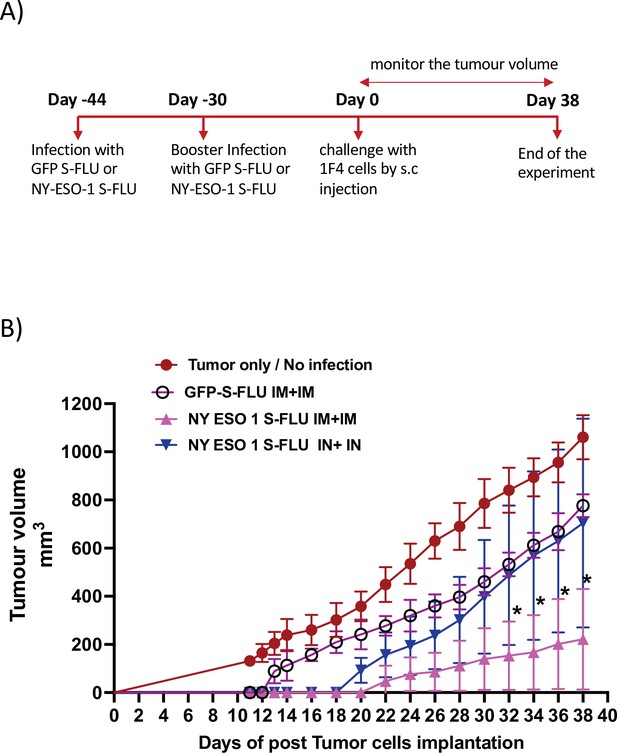
Memory T cell responses elicited by intramuscular injection of NY-ESO-1 S-FLU protect the mice against tumour challenge.
(A) Experimental design. (B) Tumour growth curves from the experiment described in (A) (n = 5–6 mice/group). *p<0.05, **p<0.01, ns, not significant (two-tailed Student’s t-test). Error bars: mean ± SEM.
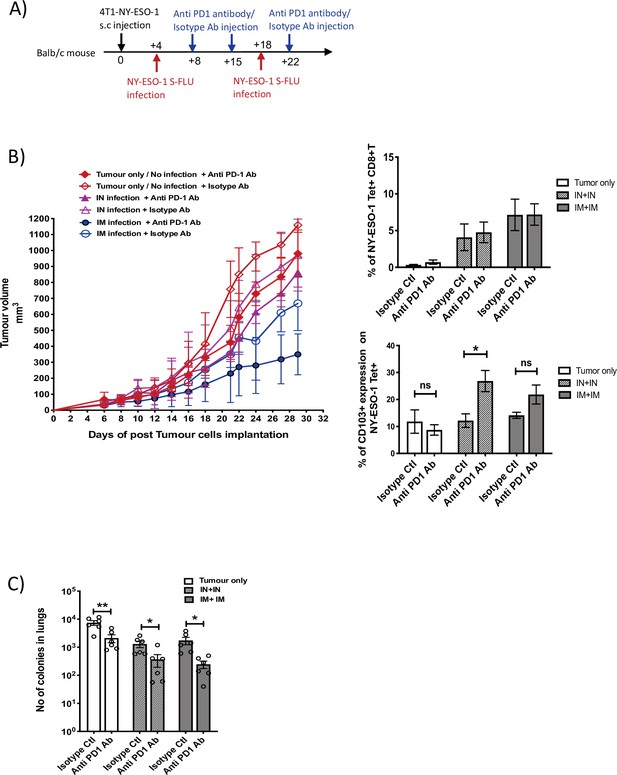
Blockade of PD-1 augments the anti-tumour effect of NY ESO-1 S-FLU virus infection.
(A) Experimental design. (B) Tumour growth curves from the experiment described in (A) (left) and bar graphs show the frequency of NY-ESO-1 tetramer+ CD8+ T cells in TIL (right upper) and the percentage of CD103 expression on NY-ESO-1 tetramer+ CD8+ T cells in tumour-infiltrating leukocytes (TIL) (right lower). (C) Clonogenic assay: bar chart shows the number of colonies in lungs. The results shown in Figure 7 are representative of two independent experiments with similar results (n = 5–6 mice/group). *p<0.05, **p<0.01, ns, not significant (two-tailed Student’s t-test). Error bars: mean ± SEM.
-
Figure 7—source data 1
Tumour volumes measured (in mm3).
- https://cdn.elifesciences.org/articles/76414/elife-76414-fig7-data1-v1.xlsx
-
Figure 7—source data 2
Frequency of NY-ESO-1 specific CD8+T cells measured by tetramer staining.
- https://cdn.elifesciences.org/articles/76414/elife-76414-fig7-data2-v1.xlsx
-
Figure 7—source data 3
Number of colonies in lungs.
- https://cdn.elifesciences.org/articles/76414/elife-76414-fig7-data3-v1.xlsx
-
Figure 7—source data 4
Percentage of CD103 expression on NY-ESO-1 tetramer positive CD8+T cells in tumour.
- https://cdn.elifesciences.org/articles/76414/elife-76414-fig7-data4-v1.xlsx