Tumor Metastasis: Fishing for drugs
About 90% of all cancer-related deaths are caused by metastasis, which is when cancer cells spread to other parts of the body to form new tumors (Chaffer and Weinberg, 2011; Suhail et al., 2019). Yet, the majority of currently available therapeutics do not inhibit metastasis, and only target the primary tumor where the cancer initially arises from.
To screen anti-cancer drugs, researchers often carry out experiments on mice or cells grown in the laboratory. While these model systems have led to effective treatments, they have limitations when it comes to testing drugs that block metastasis. For instance, cells cultured in the laboratory cannot accurately replicate tumor progression in humans (Katt et al., 2016), and metastasis can take at least several weeks to appear in mouse models, which are expensive to create and maintain (Simons and Brayton, 2017). Now, in eLife, Joji Nakayama, Zhiyuan Gong and co-workers report an innovative zebrafish model for screening anti-metastasis drugs (Nakayama et al., 2021).
The zebrafish was introduced to the research field in 1972, and has become a powerful model system for cancer research, due to its relative transparency, high reproduction rates, and genetic similarity to humans (Brown et al., 2017; Chen et al., 2021; Fazio et al., 2020; Gamble et al., 2021; Hason and Bartůněk, 2019). Early in development, cells in the zebrafish embryo undergo a morphological change and migrate inwards via a process called epiboly (Bruce and Heisenberg, 2020). The way these healthy cells move is similar to how cancer cells travel across tissues during metastasis. Hence, Nakayama et al. proposed that small-molecule inhibitors that interrupt epiboly may also suppress metastasis.
The team (who are based at the National University of Singapore, the National Cancer Center in Japan and other institutes in Singapore and Japan) found that some of the genes expressed during zebrafish epiboly are also activated during tumor metastasis. This finding provides the experimental support that zebrafish epiboly can serve as a model for tumor cell movement. So, Nakayama et al. developed a zebrafish screening platform, which they used to test 1,280 drugs that had already been approved by a government agency, such as the US Food and Drug Administration (FDA) or the European Medicines Agency (EMA).
The screen was carried out on zebrafish embryos exposed to a specific drug at four hours post-fertilization (Figure 1A). Nakayama et al. found that 132 of the drugs tested induced a delay in epiboly after five hours of treatment. Several of these drugs had previously been reported to inhibit molecular mechanisms associated with metastasis (Liu et al., 2013; Nakayama et al., 2020).
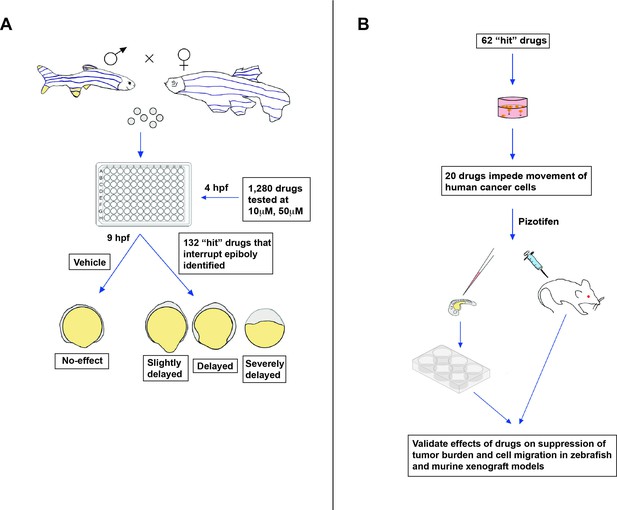
New approach for screening anti-metastasis drugs.
(A) Zebrafish were bred and their embryos were collected and plated into individual wells. Each embryo was treated four hours post-fertilization (hpf) with either the vehicle (an inactive substance that the drug is mixed with to facilitate administration) or a drug that had been approved by the FDA, EMA, or another government agency for cancer treatment: two concentrations were administered (10 µm and 50 µm). Out of the 1,280 drugs tested, 132 interrupted or delayed epiboly five hours after the drug was administered. (B) 62 of these positive hits were then tested on tumor cells cultured in the laboratory. This revealed that 20 of these drugs also impeded the migration of cancer cells, in addition to disrupting epiboly in zebrafish. One of the identified drugs, called Pizotifen, was then administered to zebrafish and mice models that had been injected with fluorescently labelled human cancer cells (commonly referred to as xenografts). This showed that the drug can also suppress metastasis in vivo.
Image credit: Emily Hill; the 6-well plate is adapted from an image by DataBase Center for Life Science (DBCLS; CC BY 4.0).
Nakayama et al. then used cell-based assays to test whether 62 of these 132 ‘positive hits’ (which also delay epiboly in vitro) can suppress the migration of cancer cells (Figure 1B). The tumor cells were placed in a chamber with or without the drug, and the team recorded how many could crawl into the neighboring compartment after a few hours of treatment. This revealed that 20 of the drugs that disrupted epiboly also impeded the movement of human cancer cells.
Finally, Nakayama et al. tested if one of the epiboly-interrupting drugs called Pizotifen could also impair tumor cell movement in living animals: this drug was selected because its primary target (serotonin receptor 2C) is highly expressed in human cancer cells during metastasis. To do this, they injected fluorescently labelled cancer cells into zebrafish embryos, and found that fish exposed to Pizotifen experienced significantly less metastasis than fish treated with a placebo. Similar observations were made in mice that had cancer cells injected into their breast-like tissue, half of which were treated with a daily dose of Pizotifen, and half of which received a placebo (Figure 1B).
The screening platform created by Nakayama et al. makes it easy to rapidly find new drugs that suppress metastasis, while circumventing the limitations of cell culture and mouse model systems. In addition, zebrafish injected with human cancer cells can serve as an additional means for narrowing down which drugs to test in mouse models. Having zebrafish join the drug discovery platform will hopefully result in more and better treatments for patients with metastatic cancers.
References
-
Zebrafish xenograft models of cancer and metastasis for drug discoveryExpert Opinion on Drug Discovery 12:379–389.https://doi.org/10.1080/17460441.2017.1297416
-
Mechanisms of zebrafish epiboly: a current viewCurrent Topics in Developmental Biology 136:319–341.https://doi.org/10.1016/bs.ctdb.2019.07.001
-
A perspective on cancer cell metastasisScience 331:1559–1564.https://doi.org/10.1126/science.1203543
-
Benefits of zebrafish xenograft models in cancer researchFrontiers in Cell and Developmental Biology 9:616551.https://doi.org/10.3389/fcell.2021.616551
-
Zebrafish patient avatars in cancer biology and precision cancer therapyNature Reviews Cancer 20:263–273.https://doi.org/10.1038/s41568-020-0252-3
-
In vitro tumor models: advantages, disadvantages, variables, and selecting the right platformFrontiers in Bioengineering and Biotechnology 4:12.https://doi.org/10.3389/fbioe.2016.00012
-
BookChapter 3 - Challenges and Limitations of Mouse Xenograft Models of CancerIn: Simons BW, editors. Patient Derived Tumor Xenograft Models: Promise, Potential and Practice. Academic Press. pp. 25–36.https://doi.org/10.1016/B978-0-12-804010-2.00003-5
-
Systems biology of cancer metastasisCell Systems 9:109–127.https://doi.org/10.1016/j.cels.2019.07.003
Article and author information
Author details
Acknowledgements
HF acknowledges grants from the National Institutes of Health (NIH; CA215059), the American Cancer Society (RSG-17-204-01-TBG), the National Science Foundation (1911253), and Boston University; CK thanks the diversity grant support from the National Institutes of Health (NIH; CA215059S); and EH is grateful for the Undergraduate Research of Program Award from Boston University.
Publication history
Copyright
© 2022, Kemet et al.
This article is distributed under the terms of the Creative Commons Attribution License, which permits unrestricted use and redistribution provided that the original author and source are credited.
Metrics
-
- 992
- views
-
- 96
- downloads
-
- 0
- citations
Views, downloads and citations are aggregated across all versions of this paper published by eLife.
Download links
Downloads (link to download the article as PDF)
Open citations (links to open the citations from this article in various online reference manager services)
Cite this article (links to download the citations from this article in formats compatible with various reference manager tools)
Further reading
-
- Developmental Biology
The structural integrity of the sperm is crucial for male fertility, defects in sperm head-tail linkage and flagellar axoneme are associated with acephalic spermatozoa syndrome (ASS) and the multiple morphological abnormalities of the sperm flagella (MMAF). Notably, impaired head-tail coupling apparatus (HTCA) often accompanies defects in the flagellum structure, however, the molecular mechanisms underlying this phenomenon remain elusive. Here, we identified an evolutionarily conserved coiled-coil domain-containing (CCDC) protein, CCDC113, and found the disruption of CCDC113 produced spermatozoa with disorganized sperm flagella and HTCA, which caused male infertility. Further analysis revealed that CCDC113 could bind to CFAP57 and CFAP91, and function as an adaptor protein for the connection of radial spokes, nexin-dynein regulatory complex (N-DRC), and doublet microtubules (DMTs) in the sperm axoneme. Moreover, CCDC113 was identified as a structural component of HTCA, collaborating with SUN5 and CENTLEIN to connect sperm head to tail during spermiogenesis. Together, our studies reveal that CCDC113 serve as a critical hub for sperm axoneme and HTCA stabilization in mice, providing insights into the potential pathogenesis of infertility associated with human CCDC113 mutations.
-
- Cell Biology
- Developmental Biology
How cells regulate the size of their organelles remains a fundamental question in cell biology. Cilia, with their simple structure and surface localization, provide an ideal model for investigating organelle size control. However, most studies on cilia length regulation are primarily performed on several single-celled organisms. In contrast, the mechanism of length regulation in cilia across diverse cell types within multicellular organisms remains a mystery. Similar to humans, zebrafish contain diverse types of cilia with variable lengths. Taking advantage of the transparency of zebrafish embryos, we conducted a comprehensive investigation into intraflagellar transport (IFT), an essential process for ciliogenesis. By generating a transgenic line carrying Ift88-GFP transgene, we observed IFT in multiple types of cilia with varying lengths. Remarkably, cilia exhibited variable IFT speeds in different cell types, with longer cilia exhibiting faster IFT speeds. This increased IFT speed in longer cilia is likely not due to changes in common factors that regulate IFT, such as motor selection, BBSome proteins, or tubulin modification. Interestingly, longer cilia in the ear cristae tend to form larger IFT compared to shorter spinal cord cilia. Reducing the size of IFT particles by knocking down Ift88 slowed IFT speed and resulted in the formation of shorter cilia. Our study proposes an intriguing model of cilia length regulation via controlling IFT speed through the modulation of the size of the IFT complex. This discovery may provide further insights into our understanding of how organelle size is regulated in higher vertebrates.