Associative learning drives longitudinally graded presynaptic plasticity of neurotransmitter release along axonal compartments
Figures
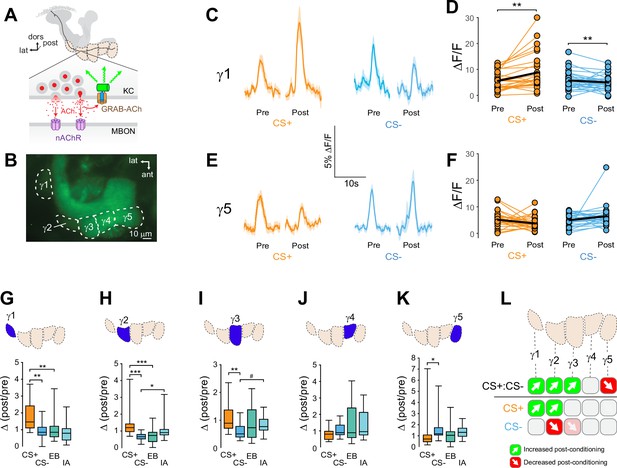
Compartment-specific alterations of acetylcholine (ACh) release in the mushroom body (MB) following appetitive conditioning.
(A) Diagram of the GRAB-ACh reporter expressed in presynaptic terminals of a Kenyon cell (KC), viewed from a frontal plane. nAChR: nicotinic ACh receptor; dors: dorsal; lat: lateral, post: posterior; MBON: mushroom body output neuron. (B) Confocal image of GRAB-ACh driven in KCs with the 238Y-Gal4 driver. ant: anterior. (C) Time series traces of odor-evoked GRAB-ACh responses in the γ1 compartment, pre- and post-conditioning, for the CS+ (ethyl butyrate: EB) and CS- (isoamyl acetate: IA) odor. The line and shading represent the mean ± SEM. (D) Quantification of the pre- and post-conditioning responses to the CS+ and CS- from the γ1 compartment from individual animals, with the mean graphed as a black line. **p<0.01; n = 27 (Wilcoxon rank-sum test). (E) Time series traces imaged from the γ5 compartment, graphed as in panel C. (F) Quantification of peak responses from the γ5 compartment, graphed as in panel D. (G–K) Change in odor-evoked ACh release (Δ(post/pre) responses) following conditioning for the CS+, CS-, and odor-only controls (EB and IA). *p<0.05, **p <0.01, ***p<0.001; n = 27 (Kruskal–Wallis/Dunn). (G) γ1 compartment. (H) γ2 compartment. (I) γ3 compartment. #p = 0.099. (J) γ4 compartment. (K) γ5 compartment. (L) Summary of plasticity in ACh release across γ lobe compartments. Green up arrows indicate increases in the CS+:CS- (first row) or potentiation of the CS+ response relative to odor-only controls (second row), while red down arrows indicate decreases in the CS+:CS- (first row) or depression of the CS- relative to odor-only controls (third row).
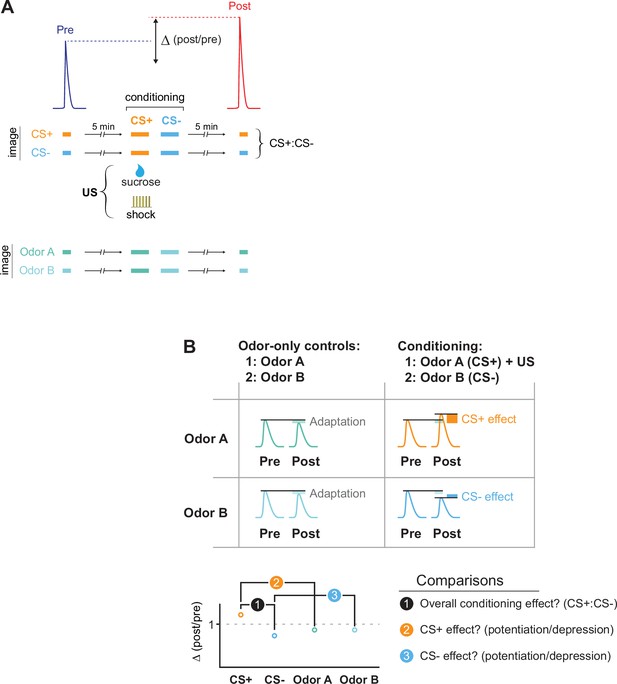
The imaging protocol for conditioning and data analysis.
(A) Flies were conditioned by pairing an odor (the CS+) with a US (electric shock or sucrose reward), and a second odor (the CS-) was presented after. Odor-evoked GRAB-ACh responses were imaged in the MB and compared before (pre) and after (post) conditioning. Responses were compared to animals in which the same odors were presented, but no US presented (odor-only controls). To examine how conditioning (or odor-only presentation) changed the odor responses, the Δ(post/pre) was calculated for each treatment. (B) Several comparisons were made across conditions. First, we tested whether there was an overall effect of conditioning by comparing the change in response, the Δ(post/pre), between the CS+ and CS- groups (comparison #1). Then we tested whether the CS+ and CS- were potentiated or depressed, normalizing for any olfactory adaptation induced by odor presentation during training. This was done by comparing the Δ(post/pre) of the CS+ and CS- to that of their respective odor-only controls (comparisons #2 and #3).

Effects of appetitive conditioning on GRAB-ACh responses across the γ lobe compartments.
(A) Diagrams of the location of each compartment within the mushroom body. (B) Time series traces pre- and post-conditioning for the CS+ (ethyl butyrate: EB) and CS- (isoamyl acetate: IA). (C) Quantification of the peak pre- and post-conditioning responses for each animal. The thick black line represents the mean. *p<0.05, **p<0.01, ***p<0.001; n = 27 (Wilcoxon rank-sum test).
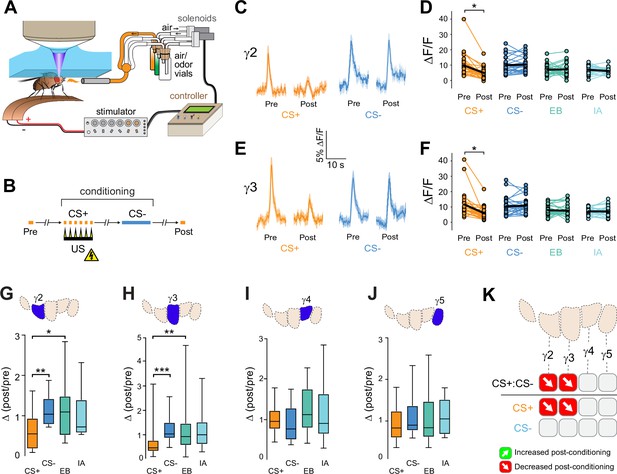
Compartment-specific alterations of acetylcholine (ACh) release in the mushroom body (MB) following aversive conditioning.
(A) Diagram of the aversive conditioning apparatus. (B) Aversive conditioning experimental protocol, pairing an odor (the CS+) with an electric shock unconditioned stimulus (US) (six shocks, 60 V). A second odor, the CS-, was presented 5 min after pairing the CS+ and US. One odor was imaged before (Pre) and after (Post) conditioning per animal (CS+ diagrammed here). (C) Time series traces showing odor-evoked GRAB-ACh responses pre- and post-conditioning in the γ2 compartment. Responses were imaged to both the CS+ (ethyl butyrate: EB) and CS- (isoamyl acetate: IA) odor, and the line and shading represent the mean ± SEM. (D) Quantification of the peak pre- and post-conditioning responses to the CS+ (EB) and CS- (IA) from the γ2 compartment of individual animals, with the mean graphed as a black line. *p<0.05; n = 27 (Wilcoxon rank-sum test). (E) Time series traces imaged from the γ3 compartment, graphed as in panel C. (F) Quantification of peak responses from the γ3 compartment, graphed as in panel D. (G–J) Change in odor-evoked responses (D(post/pre) responses), following conditioning (CS+ and CS-) or odor-only presentation (EB and IA). *p<0.05, **p<0.01, ***p<0.001; n = 27 (Kruskal–Wallis/Dunn). (G) γ2 compartment. (H) γ3 compartment. (I) γ4 compartment. (J) γ5 compartment. (K) Summary of plasticity in ACh release across γ lobe compartments. Red down arrows indicate decreases in the CS+:CS- (first row) or depression of the CS+ relative to odor-only controls (second row).
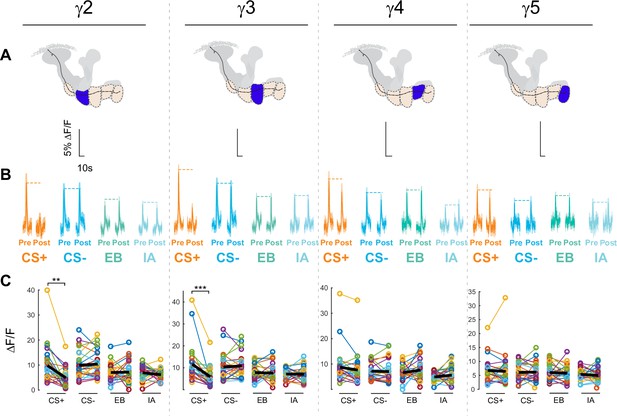
Effects of aversive conditioning on GRAB-ACh responses across the γ lobe compartments.
(A) The top row shows diagrams of the location of each compartment within the mushroom body. (B) The second row shows time series traces pre- and post-conditioning for the CS+, CS-, and respective odor only controls (EB and IA). (C) The third row shows quantification of the peak pre- and post-conditioning responses for each animal. The thick black line represents the mean. **p<0.01, ***p<0.001; n = 27 (Wilcoxon rank-sum test).
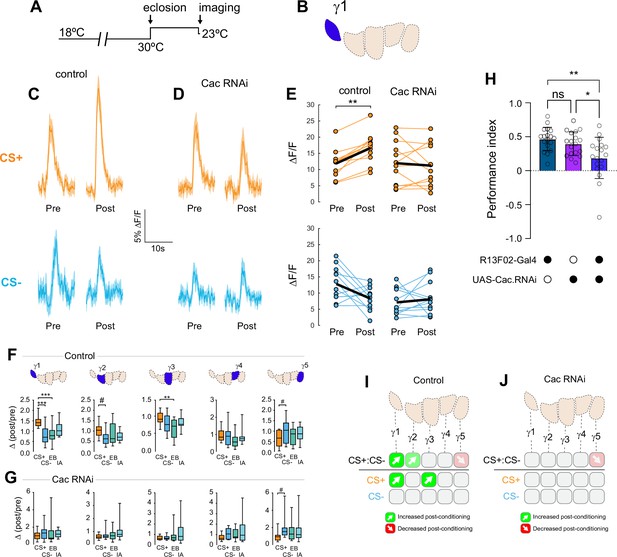
Conditional knockdown of the CaV2 channel Cac in KCs impairs potentiation of ACh release from the MB following appetitive conditioning.
(A) Diagram of the temperature shifts employed for conditional knockdown of Cac with tub-Gal80ts. (B) Diagram of the MB compartments, highlighting the γ1 compartment that was imaged for the data shown in panels C-E. (C) Pre- and post-conditioning CS+ (orange; top) and CS- (blue; bottom) odor-evoked ACh release from the γ1 compartment before and after appetitive conditioning, imaged in control animals (w;UAS-GRAB-ACh/+; R13F02-Gal4/UAS-tub-Gal80ts). Time series trace with line and shading representing mean ± SEM. (D) Effect of conditional Cac knockdown on odor-evoked ACh responses in the γ1 compartment following appetitive conditioning, graphed as in panel C (genotype: w;UAS-GRAB-ACh/UAS-Cac-RNAi; R13F02-Gal4/UAS-tub-Gal80ts). (E) Pre- and post-conditioning ΔF/F CS+ and CS- responses in control and Cac knockdown animals. **p<0.01; n = 12 (Wilcoxon rank-sum test). (F) Change in odor-evoked ACh release (Δ(post/pre)) following appetitive conditioning for the CS+, CS-, and odor-only controls (EB and IA) in control animals across the five MB γ lobe compartments: γ1-γ5 (left to right). **p<0.01, ***p<0.001, γ2 compartment #p=0.052, γ5 compartment #p=0.120; n = 12 (Kruskal–Wallis/Dunn). (G) Effect of conditional knockdown of Cac on odor-evoked ACh responses across compartments, graphed as in panel F. γ5 compartment #p=0.077; n = 12 (Kruskal–Wallis/Dunn). (H) Behavioral appetitive conditioning following conditioning knockdown of Cac. *p<0.05, **p<0.01; n = 12 (ANOVA/Sidak). (I) Summary of plasticity in ACh release across γ lobe compartments in control (uninduced) animals. (J) Summary of plasticity in ACh release (as in I) in animals with conditional Cac knockdown.
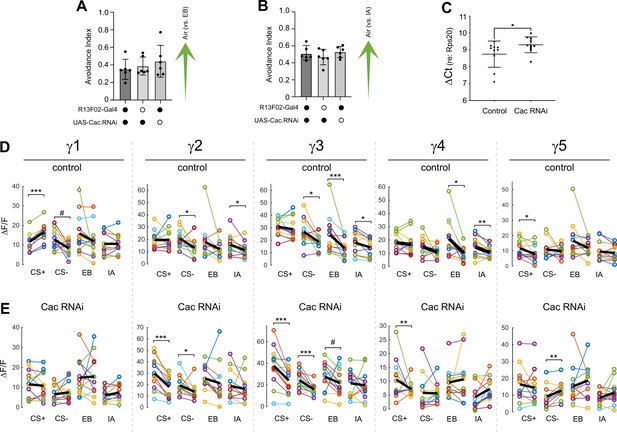
Behavioral avoidance of (A) ethyl butyrate (EB) and (B) isoamyl acetate (IA).
(C) Quantitative PCR analysis of Cac knockdown (driven ubiquitously by tubulin-Gal4). (D,E) Effects of appetitive conditioning on GRAB-ACh responses across the γ lobe compartments in control and Cac conditional knockdown flies. In each panel, thin lines connect the peak pre- and post-conditioning responses for each animal/stimulus and the thick balck line represents the mean. *p<0.05, *p<0.01, ***p<0.001; γ1 control CS- #=0.092, γ2 Cac EB #p=0.092, γ3 Cac EB #p=0.092; n = 12 (Wilcoxon rank-sum test).
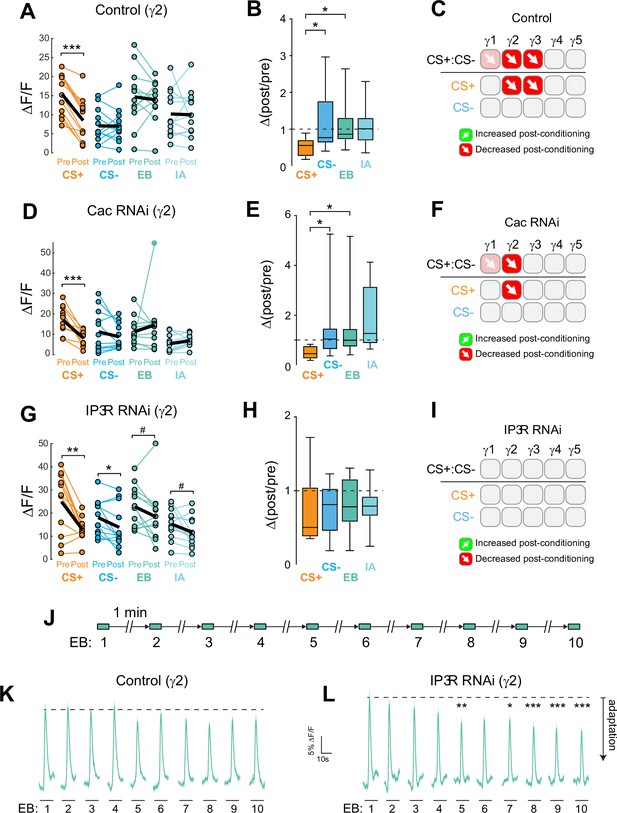
Cac and IP3 R exert distinct effects on synaptic plasticity and maintenance of olfactory responses in the γ2 compartment following aversive conditioning.
(A,D,G) Quantification of odor-evoked responses in control, Cac RNAi, and IP3 R RNAi animals, respectively. *p<0.05, **p<0.01, ***p<0.001; n = 12 (Wilcoxon rank-sum test). IP3 R RNAi: EB #p=0.092, IA #p=0.064. (B,E,H) Change in odor-evoked ACh release (Δ(post/pre) responses) following conditioning for the CS+, CS-, and odor-only controls (EB and IA). *p<0.05; n = 12 (Kruskal–Wallis/Dunn). (C,F,I) Summary of plasticity in ACh release across γ lobe compartments in control, Cac RNAi, and IP3 R RNAi animals, respectively. Red down arrows indicate decreases in the CS+:CS- (first row) or depression of the CS + relative to odor-only controls (second row). (J) Olfactory adaptation protocol. (K) Odor-evoked ACh release (ΔF/F time series traces) measured across repeated odor presentations in control flies. (L) Odor-evoked ACh release (ΔF/F time series traces) measured across repeated odor presentations in IP3 R knockdown flies. *p<0.05, **p<0.01, ***p<0.001; n = 27 (two-way ANOVA/Sidak).
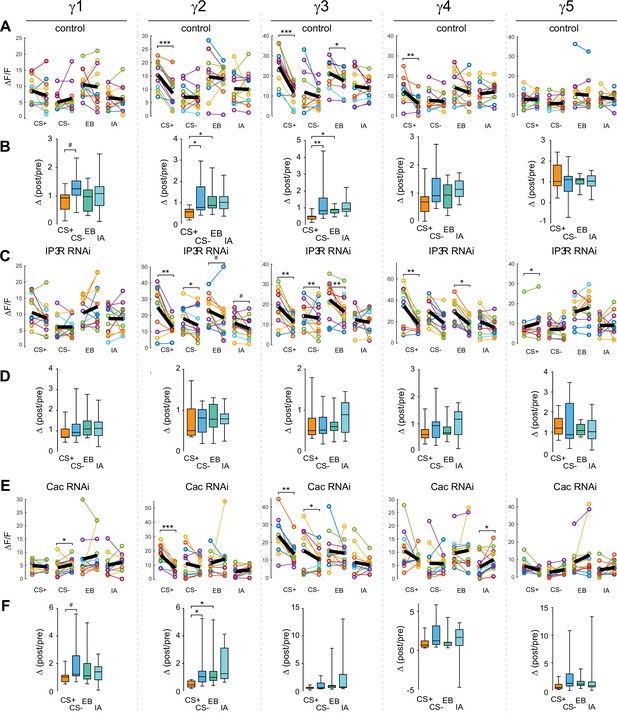
Effects of aversive conditioning on GRAB-ACh responses across the γ lobe compartments using GRAB-ACh with control, Cac RNAi and IP3 R RNAi flies.
(A) Quantification of the pre- and post-conditioning responses in control flies for the CS+, CS-, and odor only controls (EB and IA), with the thick black line representing the mean. (B) Change in odor-evoked ACh release (Δ(post/pre)) in control flies following aversive conditioning for the CS+, CS-, and odor-only control odors (EB and IA). γ1 #P = 0.115. (C) Quantification of the pre- and post-conditioning responses Cac RNAi flies, graphed as in panel A. γ2 EB #p=0.092, IA #p=0.064. (D) Change in odor-evoked ACh release in IP3 R RNAi flies, graphed as in panel B. (E) Quantification of the pre- and post-conditioning responses Cac RNAi flies, graphed as in panel A. (F) Change in odor-evoked ACh release in Cac RNAi flies, graphed as in panel B. γ1 #p=0.086. For all genotypes, n = 12; pre- vs post-comparisons: *p<0.05, **p<0.01, ***p<0.001 (Wilcoxon rank-sum test); Δ(post/pre) comparisons: *p<0.05, **p<0.01 (Kruskal–Wallis/Dunn).

Adaptation of odor-evoked GRAB-ACh responses across the γ lobe compartments in control and IP3 R knockdown flies upon repeated odor presentation.
Each trace represents the odor response within the corresponding compartment (mean ± SEM). *p<0.05, *p<0.01, ***p<0.001 (two-way ANOVA/Sidak), n = 12, comparing the peak ΔF/F for each response to that of the first (naïve) odor presentation.
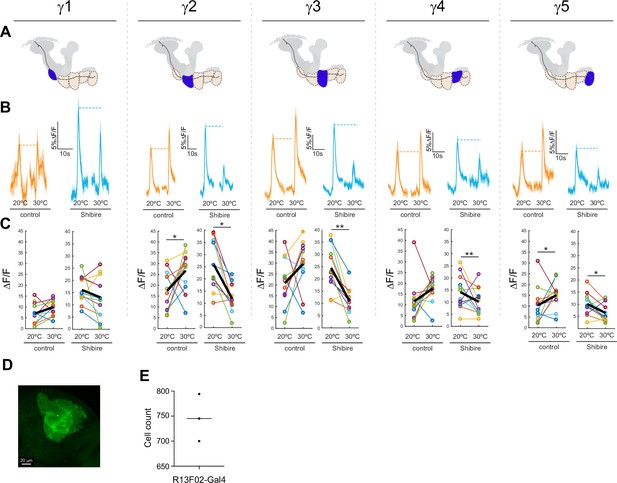
Effect of silencing KCs on GRAB-ACh responses across the γ lobe compartments.
(A) Diagrams of the location of each compartment within the mushroom body. (B) Time series traces of the pre- and post-temperature changes for odor (ethyl butyrate) responses. (C) Quantification of the peak pre- and post-temperature shift odor responses for each animal (n = 12). The thick black line represents the mean. *p<0.05, **p<0.01 (Wilcoxon rank-sum test). (D,E) Counts of GFP+ KCs labeled per hemisphere by the R13F02-Gal4 driver.
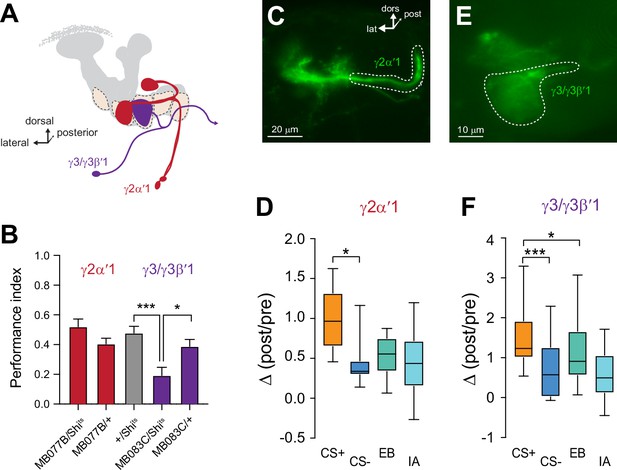
Plasticity in MBON Ca2+ responses mirrors compartmental plasticity in the mushroom body (MB) neurons.
(A) Diagram of MBONs innervating specific γ lobe compartments, viewed from a frontal plane. Each MBON is bilaterally paired, though only one is drawn here for visual clarity. (B) Behavioral appetitive conditioning when synaptic output from the γ2α′1 and γ3 MBONs was blocked with Shibirets (Shits), driven with the MB077B and MB083C split Gal4 drivers, respectively. *p<0.05; ***p<0.001; n = 16 (ANOVA/Sidak). (C,E) Representative confocal images of the γ2α′1 and γ3 MBONs, respectively. The region of interest circumscribed for quantification is drawn with a dotted white line. lat: lateral, dors: dorsal, post: posterior. (D,F) Change in odor-evoked responses (Δ(post/pre responses)) in the γ2α′one and γ3 MBONs, respectively, following appetitive conditioning. *p<0.05; ***p<0.001; n = 12 (Kruskal–Wallis/Dunn).

Plasticity in MBON Ca2+ responses mirrors compartmental plasticity in the MB neurons.
(A) Diagram of MBONs innervating specific γ lobe compartments, viewed from a frontal plane. Each MBON is bilaterally paired, though only one is drawn here for visual clarity. (B,D) Representative confocal images of the γ1pedc>α/β and γ5β′2 a MBONs, respectively. The region of interest circumscribed for quantification is drawn with a dotted white line. lat: lateral, dors: dorsal, post: posterior. (C,E) Change in odor-evoked responses (Δ(post/pre) responses) in the γ1pedc>α/β and γ5β′2 a MBONs, respectively, following appetitive conditioning. *p<0.05; **p<0.01; n = 12 (Kruskal–Wallis/Dunn).