Measuring the tolerance of the genetic code to altered codon size
Figures
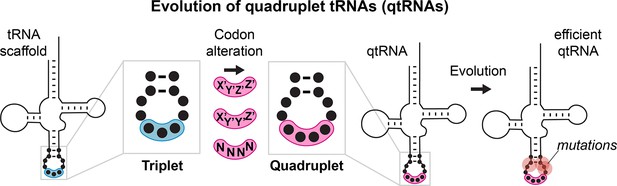
Evolution of quadrupelt tRNAs.
We studied whether tRNAs can arise through simple changes to the anticodon followed by additional mutations accumulated during evolution.
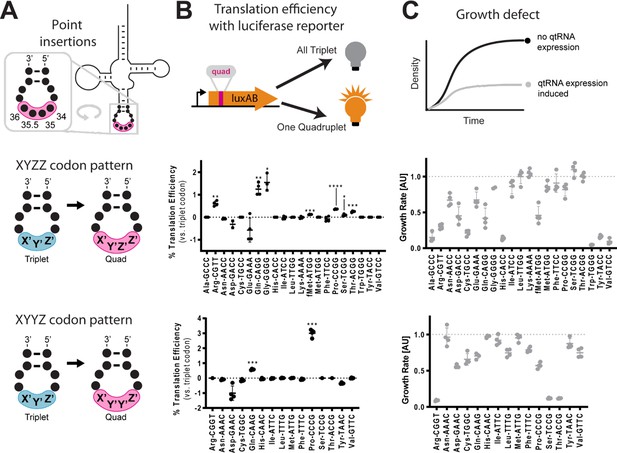
Engineering qtRNAs with codon patterns.
(A) We measured quadruplet tRNAs (qtRNAs) that might arise through point insertions in the anticodon loop.
Each qtRNA is based upon a tRNA from the Escherichia coli genome that serves as a ‘scaffold’ (Supplementary file 1). We tested two quadruplet codon patterns: a tRNA decoding the triplet codon ‘XYZ’ to a qtRNA decoding the quadruplet codon ‘XYZZ’ or ‘XYYZ’. In instances in which XYZZ and XYYZ are the same, the qtRNA is depicted on the XYZZ graph. We use ‘qtRNA’-‘three letter scaffold’-‘four letter codon’ nomenclature to refer to qtRNAs; for example, a serine qtRNA bearing a 5’-UCUA-3’ anticodon that recognizes 5’-UAGA-3’ in mRNA transcripts is referred to as qtRNASerTAGA. (B) We measured qtRNAs using a luciferase readthrough assay. Measurements are taken kinetically and normalized to culture density, and efficiency is reported relative to luminescence produced by a wildtype (WT), all triplet luciferase transcript. qtRNAs that are statistically >0 are annotated with their one-sample t-test p-value: 0.033(*), 0.0021(**), 0.0002(***), < 0.001(****). fMet qtRNAs are measured with a luciferase reporter bearing a quadruplet codon at residue 1; all others are measured with a quadruplet codon at residue 357 of luxAB. (C) Expression of qtRNAs can be toxic. Here, we report the fractional OD600 density difference between cultures where qtRNA expression had been induced versus suppressed. Data in (B and C) represent the mean and standard deviation of three to eight technical replicates in one biological replicate. For raw data, see Figure 2—source data 1.
-
Figure 2—source data 1
Luminescence and growth metric of XYZZ and XYYZ qtRNAs.
- https://cdn.elifesciences.org/articles/76941/elife-76941-fig2-data1-v2.csv
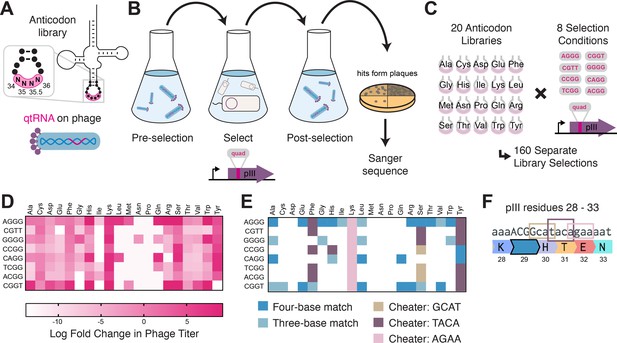
Selection for functional qtRNAs.
(A) We created quadruplet tRNAs (qtRNA) libraries using degenerate primers to randomize the four bases in the anticodon. The qtRNA library is expressed from a ΔpIII M13 bacteriophage. (B) We selected these libraries by challenging the phage to infect and propagate in bacteria that require a quadruplet codon to be translated in order to produce a functional version of the essential phage gene, pIII. Plaques from selected libraries were Sanger sequenced. (C) We crossed each library with each pIII-based reporter for a total of 160 separate library selections. (D) The log fold change in phage titer when comparing the post-selection population to the pre-selection population. Data represent the mean in one biological replicate. For raw data, see Figure 3—source data 1. (E) For every filled square, we sequenced two plaques in order to determine the anticodon identity. Results include instances in which the qtRNAs match the reporter at all four positions, or that match with the first three bases of the codon (shades of blue). Additionally, we show instances in which qtRNAs were discovered that suppress a different quadruplet codon near residue 29. (F) Although we intend for the phage to suppress the quadruplet codon located at permissive residue 29 of pIII (Bryson et al., 2017), in several instances the selection identified qtRNAs that suppress a nearby quadruplet codon instead.
-
Figure 3—source data 1
Log fold change in phage titer.
- https://cdn.elifesciences.org/articles/76941/elife-76941-fig3-data1-v2.csv
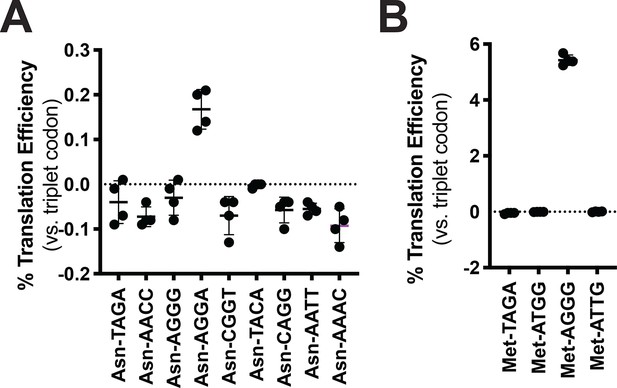
Codon reassignment for asparagine and methionine.
Quadruplet codon experimentation for asparagine and methionine. Measurements are taken kinetically and normalized to culture density. Efficiency is reported relative to luminescence produced by a wildtype (WT), all triplet luciferase transcript. (A) Characterization of nine unique quadruplet codons within the tRNAAsn scaffold. (B) Characterization of four quadruplet codons within the tRNAMet scaffold.
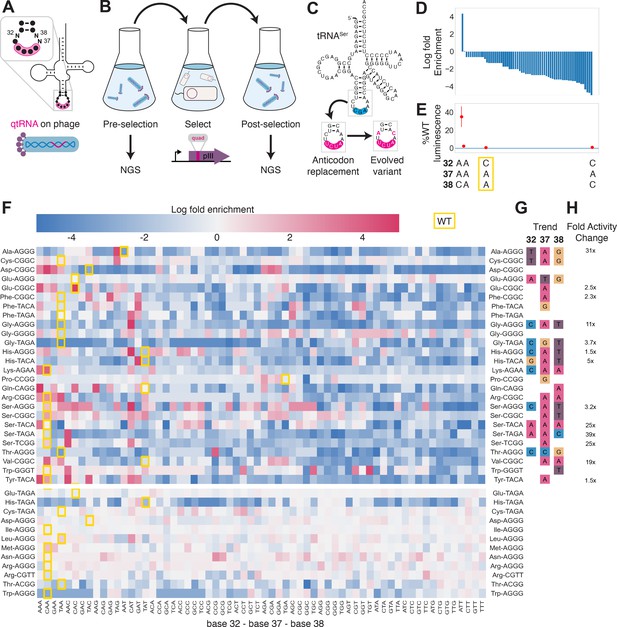
Directed evolution of anticodon loop sides.
(A) We created quadruplet tRNAs (qtRNA) libraries using degenerate primers to randomize positions 32, 37, and 38 of the anticodon. The qtRNA library is expressed from a ΔpIII M13 bacteriophage. (B) We selected these libraries by challenging the phage to infect and propagate in bacteria that require a quadruplet codon to be translated in order to produce a functional version of the essential phage gene, pIII. Libraries were next-generation sequencing (NGS) sequenced to >10× library size before and after selection. (C) tRNASerTCG is known to be a scaffold for the functional qtRNASerTAGA after anticodon replacement alone. Additional mutations to the sides of the anticodon loop are known to improve quadruplet codon translation efficiency. (D) Log fold enrichment of the population abundance and (E) translation efficiency as measured by a luciferase readthrough assay of the 64 possible combinations of nucleotides at positions 32, 37, and 38. (F) Log fold enrichment of all 41 qtRNA libraries for each of the 64 library members. Libraries are separated by those that exhibit abundance changes during selection (above) from those that exhibit no significant abundance changes (below). The anticodon loop sides present in the wildtype (WT) tRNA scaffold are boxed in gold. For raw data, see Figure 4—source data 1. (G) For each library, the trend in nucleotide preference for each position is listed. (H) Nucleotide preferences for select libraries were measured by cloning a qtRNA variant and measuring it using a luciferase readthrough assay. The fold improvement in activity over the WT values of base 32, 37, and 38 are listed. In (e and f), the original identities of bases 32, 37, and 38 found in the WT triplet tRNA scaffold are boxed in gold.
-
Figure 4—source data 1
Log fold enrichment of anticodon loop side libraries.
- https://cdn.elifesciences.org/articles/76941/elife-76941-fig4-data1-v2.csv
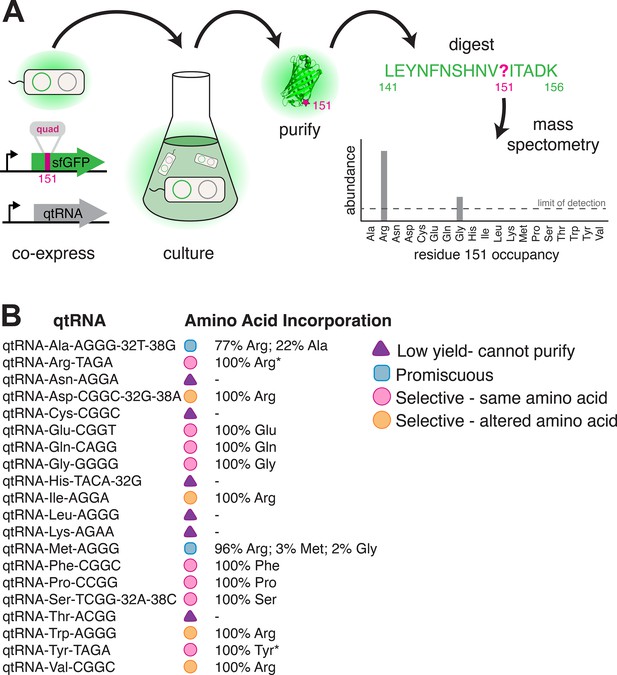
Characterization of amino acid incorporation by quadruplet tRNAs (qtRNAs).
(A) We characterized the amino acid incorporated during translation by co-expressing a qtRNA and an sfGFP-151-quad transcript, purifying the resulting GFP, and analyzing the occupancy of residue 151 using mass spectrometry (Supplementary file 3). (B) Results of applying this pipeline to at least one qtRNA based on each of the 20 canonical scaffolds (Materials and methods – ‘Quantification of qtRNA charging using mass spectrometry’). Charging of (*) qtRNAArgTAGA and qtRNATyrTAGA has been previously reported (DeBenedictis et al., 2021). Data represents the mean of one biological replicate. For some qtRNAs, yield was too low to allow for charging characterization even when purified at 1 L scale (Supplementary file 2). Raw spectra have been deposited in the PRIDE database (Perez-Riverol et al., 2022), dataset identifier PXD031925 and 10.6019/PXD031925.
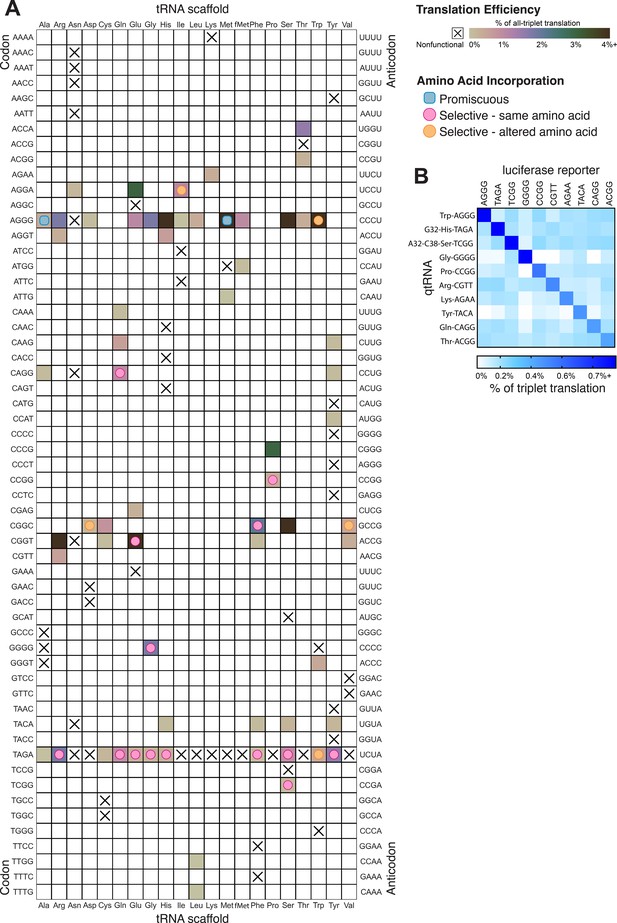
Compiled qtRNA measurements.
(A) Compiled results of quantifying quadruplet tRNAs (qtRNA) translation efficiency (Materials and methods, ‘Luciferase readthrough assay’) and charging (Materials and methods, ‘Quantification of qtRNA charging using mass spectrometry’). The qtRNA scaffold (columns) and codon (rows) are indicated. The translation efficiency is indicated on each qtRNA that could be measured, or marked as ‘nonfunctional’, indicating that the qtRNA exhibits too strong a growth defect to measure, or does not produce a measurable increase luminescence upon induction of qtRNA expression. Translation efficiency is measured as a percent of all-triplet translation (Materials and methods, ‘Luciferase readthrough assay’). fMet qtRNAs are measured with a luciferase reporter bearing a quadruplet codon at residue 1; all others are measured with a quadruplet codon at residue 357 of luxAB. Charging results are indicated on the table for qtRNAs derived from each scaffold-codon pair; the measured qtRNAs may contain additional mutations; see Supplementary files 2 and 3. Data represent the mean of three to eight technical replicates in each of between one and eight biological replicates. For complete compiled raw data, see Figure 6—source data 1. (B) A miniature all-quadruplet genetic code. For each of the 10 qtRNAs (rows), we measured readthrough of a luciferase transcript containing the indicated quadruplet codon at residue 357 (columns). For raw data, see Figure 6—source data 2.
-
Figure 6—source data 1
Luminescence measurements of qtRNAs.
- https://cdn.elifesciences.org/articles/76941/elife-76941-fig6-data1-v2.csv
-
Figure 6—source data 2
Luminescence measurements of qtRNA crosstalk.
- https://cdn.elifesciences.org/articles/76941/elife-76941-fig6-data2-v2.csv
Tables
Reagent type (species) or resource | Designation | Source or reference | Identifiers | Additional information |
---|---|---|---|---|
Strain, strain background (Escherichia coli) | S2060 | Addgene | #105064 | K12 derivative optimized for directed evolution |
Recombinant DNA reagent | pED1a6 (plasmid) | Addgene | #134787 | Luciferase reporter for quadruplet codon translation |
Recombinant DNA reagent | pED17 × 11 (plasmid) | Addgene | #134814 | sfGFP reporter for quadruplet codon translation |
Recombinant DNA reagent | pED7 × 10 (plasmid) | Addgene | #134812 | pIII reporter for quadruplet codon translation |
Recombinant DNA reagent | pED14xS-1(plasmid) | Addgene | #134800 | IPTG inducible expression of qtRNA |
Additional files
-
Supplementary file 1
Table of tRNA scaffolds.
Canonical triplet tRNAs were cloned from Escherichia coli K12 genomic DNA. Body text Figures 2 and 3 concern mutations to the anticodon (bolded caps); body text Figure 4 concerns mutation to positions 32, 37, and 38 (bolded lower case). We use qtRNAthree letter scaffoldfour letter DNA codon nomenclature to refer to qtRNAs; for example, to create qtRNASerTAGA, the CGA anticodon in tRNASer scaffold would be replaced by TCTA.
- https://cdn.elifesciences.org/articles/76941/elife-76941-supp1-v2.docx
-
Supplementary file 2
Purification scale of qtRNA-translated proteins.
For sfGFP purification, qtRNA expression plasmids (as listed) were co-expressed with C-terminal 6xHis-tagged sfGFP with the appropriate quadruplet codon replacing permissive residue 151. For example, sfGFP-151-GGGG,https://benchling.com/s/seq-bl1bixktGKegGwboMYIP. Peptides detected are listed in Supplementary file 3. Raw spectra have been deposited in the PRIDE database (Perez-Riverol et al., 2022), dataset identifier PXD031925 and 10.6019/PXD031925.
- https://cdn.elifesciences.org/articles/76941/elife-76941-supp2-v2.docx
-
Supplementary file 3
Mass spectrometry of peptides flanking residue 151.
List of peptides flanking residue 151 that were detected during mass spectrometry. Raw spectra have been deposited in the PRIDE database (Perez-Riverol et al., 2022), dataset identifier PXD031925 and 10.6019/PXD031925.
- https://cdn.elifesciences.org/articles/76941/elife-76941-supp3-v2.docx
-
Transparent reporting form
- https://cdn.elifesciences.org/articles/76941/elife-76941-transrepform1-v2.pdf