Sociosexual behavior requires both activating and repressive roles of Tfap2e/AP-2ε in vomeronasal sensory neurons
Figures
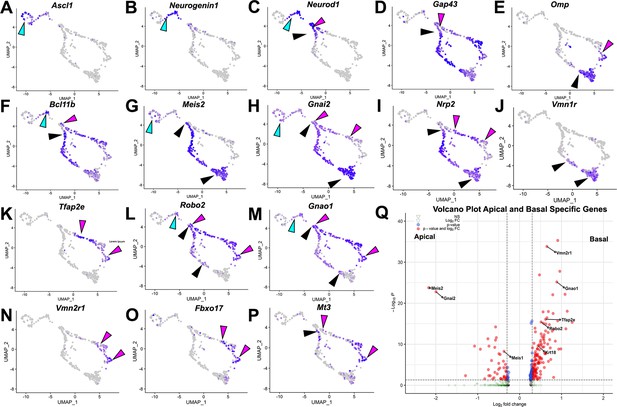
Analysis of single-cell sequencing data of the vomeronasal organ.
P10 male controls shows the developing vomeronasal neurons as they progress through (A–C) neurogenesis (Ascl1, Neurog1, Neurod1), (D–F) maturation (Gap43, Omp, Bcl11b), (G–J) apical vomeronasal sensory neurons (VSNs)’ differentiation/maturation (Meis2, Gnai2, Nrp2, Vmn1r) and (K–P) basal VSNs’ differentiation/maturation (Tfap2e, Robo2, Gnao1, Vmn2r1, Fbxo17, Mt3). (A) Ascl1 is expressed by transiently amplifying progenitor cells (cyan arrow), which transition into the immediate neuronal precursors that turn on pro-neural genes (cyan arrow) Neurog1 (B) and Neurod1 (C) and turn off as the precursors turn into immature neurons (black and magenta). (D) Immature neurons express Gap43, which persists in both apical (black arrow) and basal (magenta arrow) branches, until it declines as neurons begin to reach maturity and express (E) Omp in mature apical (black arrow) and mature basal (magenta arrow) VSNs. Gap43 and Omp expression briefly overlap, as the neurons transition to a fully differentiated mature stage. (F) Bcl11b mRNA expression is found in both apical and basal VSNs but at different developmental timepoints. Bcl11b is found in committed basal precursors near the establishment of the apical/basal dichotomy (magenta arrow) but is not found until later in apical VSN development (black arrow). (G) Meis2 mRNA expression is found in the apical branch (black arrows) and even in early neurogenesis stages (cyan arrow), and their expression does not overlap. (H) Gnai2 expression starts in immature apical and basal VSNs. However, its expression increases in mature apical VSNs but fades in mature basal VSNs. (I) Nrp2 expression is only retained in mature apical VSNs. (J) Vmn1r is only expressed in maturing/mature apical VSNs. (K) Tfap2e is expressed by the basal branch (magenta arrows). (L) Robo2 expression is only retained in mature basal VSNs. (M) Gnao1 expression starts in immature apical and basal VSNs. However, its expression increases in mature basal VSNs but fades in mature apical VSNs. (N) Vmn2r1 is restricted to maturing/mature basal VSNs. (O) Fbxo17 is mainly expressed in mature/maturing basal VSNs. (P) Mt3 is mainly expressed in mature/maturing basal VSNs. (Q) Enhanced volcano plot. Differential gene expression between apical and basal branches of VSNs. Apical-specific genes (55 genes) trend left and basal-specific genes (187 genes) trend right. Significance defined as log2-fold change >0.3 and adjusted p-value ≤0.05.
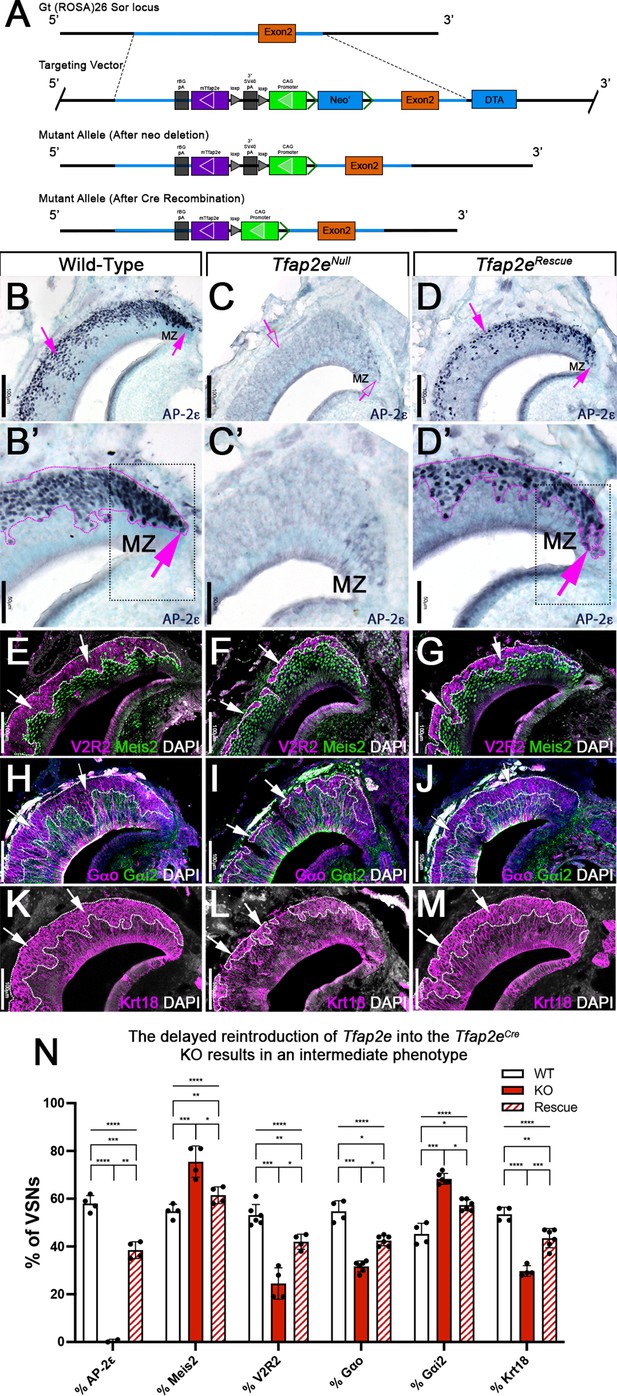
R26AP-2ε mouse line mouse generation and characterization of rescued Tfap2e expression in Tfap2eNull mice.
(A) Knock-in strategy through homologous recombination to generate the R26AP-2ε mouse line. The CAG-loxP-stop-loxP-mouse-Tfap2e cassette as integrated into the first intron of Rosa26. (B–D’) Immunohistochemistry on P21 wild-type (WT) (B, B’), Tfap2eNull (C,C’), and Tfap2eRescue (D,D’) mice against AP-2ε. (B,B’) In WT mice AP-2ε expression is in the marginal zones (MZ) and in the basal regions of the VSNs. (C,C’) In Tfap2eNull mice, some AP-2ε immunoreactivity is observed in the MZ but lost in the central regions where more mature neurons reside. (D,D’) In the Tfap2eRescue, AP-2ε is expressed in the basal region, but with less intensity and density at the MZ and in central regions compared to WT controls. (E–G) Immunostainings against V2R2 (magenta) and Meis2 (green) counterstained with 4’,6’-diamidino-2-phenylindole (DAPI) (white). (H–J) Immunostainings against Gαo (magenta) and Gαi2 (green) counterstained with DAPI. (K–M) Immunostainings against Krt18 (magenta) counterstained with DAPI (white). (N) Quantifications of the percentage of vomeronasal sensory neurons (VSNs) expressing apical/basal markers in WT, Tfap2eNull, and Tfap2eRescue mice. Tfap2eNull mice show a dramatic reduction in basal VSNs and apical VSNs occupy most of the epithelium. The Tfap2eRescue has an intermediate phenotype between WT and Tfap2eNull mice, where the vomeronasal epithelium (VNE) contains more basal VSNs than in the Tfap2eNull mice but does not reach the equivalency of the WT (p<0.05 = *, p<0.01 = **, p<0.001 = ***, p<0.0001 = ****). One-way ANOVA. Error bars are standard deviation. N=4 for WT in % AP-2ε, % meis2, % Gαo, % Gαi2, and % Krt18. N=6 for WT in % V2R2. N=2 for Tfap2eNull in % AP-2ε. N=4 for Tfap2eNull in % Meis2, % V2R2, and % Krt18. N=6 for Tfap2eNull in % Gαo and % Gαi2. N=4 for Tfap2eRescue in % AP-2ε, % Meis2, and % V2R2. N=6 for Tfap2eRescue in % Gαo, % Gαi2, and % Krt18.
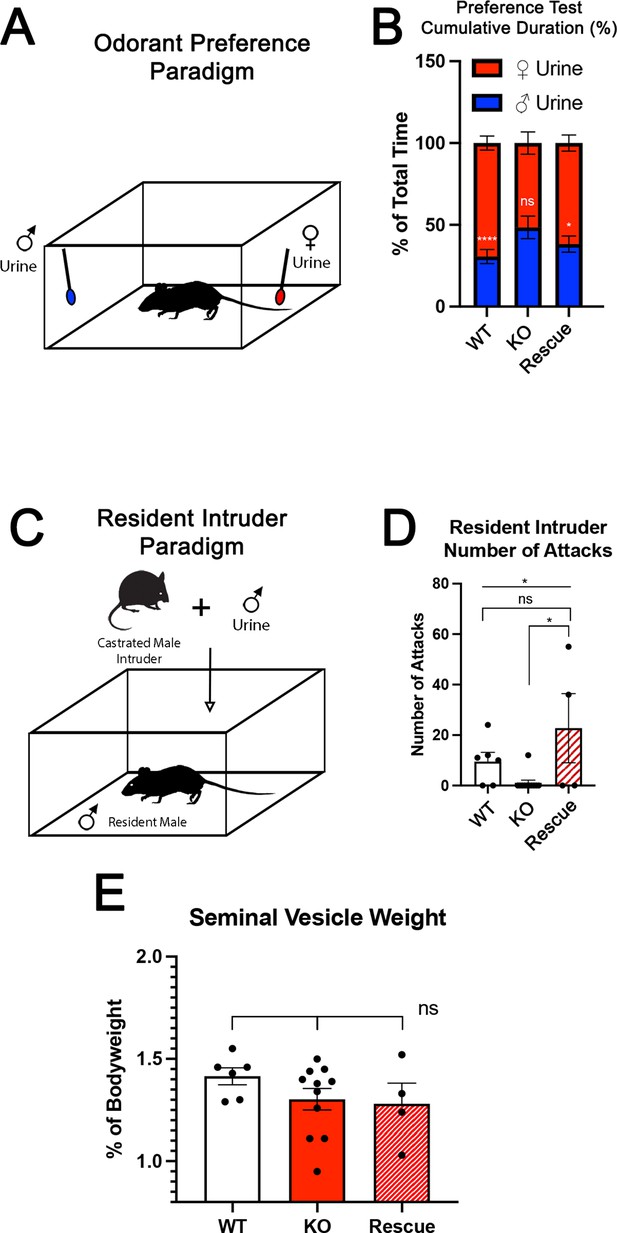
Territorial aggression and sex preference depends on Tfap2e expression in mice.
(A) The odorant preference paradigm where cotton swabs with either male or female whole urine are placed on opposite ends of a test cage and the amount of time spent smelling each odorant is measured. (B) Male wild-type (WT) mice spent significantly more time investigating female odorants than male odorants. Preference for female odorants is lost in Tfap2e knockout (KO) male mice but restored in Tfap2eRescue mice (p<0.05 = *, p<0.01 = **, p<0.001 = ***). Unpaired t-test. Error bars are standard deviation. N=6 for WT, n=11 for Tfap2eNull and n=4 for Tfap2eRescue. (C) Male-male aggression was evaluated using the resident intruder paradigm. (D) WT mice display aggressive behaviors toward male intruders and number of attacks were quantified. Tfap2eNull mice attacked intruders significantly less than WT mice. However, Tfap2eRescue mice showed significantly more aggressive behaviors that is not significantly different than the WT male mice (p<0.05 = *). One-way ANOVA. Error bars are standard deviation. N=6 for WT, n=11 for KO, n=4 for rescue. (E) Seminal vesicle weight was not significantly different across all genotypes when normalized to body weight. One-way ANOVA. Error bars are standard deviation. N=6 for WT, n=11 for KO, n=4 for rescue.
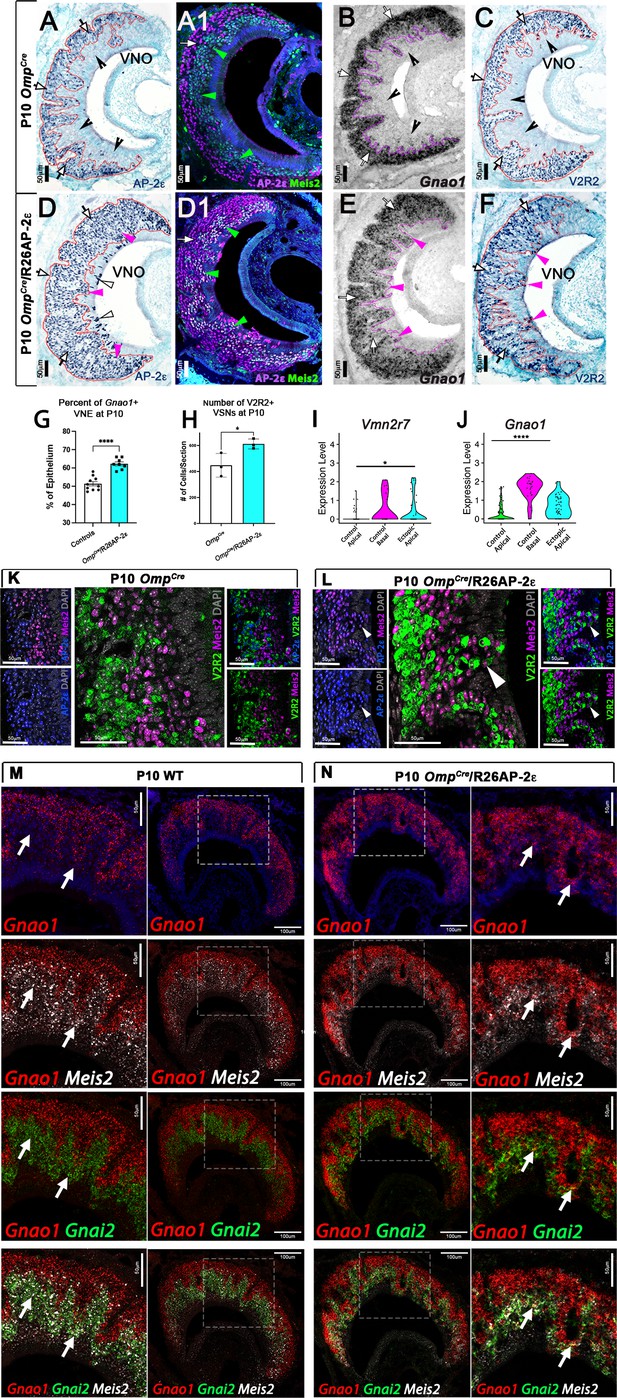
Ectopic expression of Tfap2e in the main olfactory epithelium (MOE) and the vomeronasal epithelium (VNE) promotes expression of basal markers.
Immunostainings at P10 on OmpCre controls (A–C) and OmpCre/R26AP-2ε mutants (D–F). (A) IHC against AP-2ε in the vomeronasal organs (VNOs) shows that AP-2ε is expressed in only the basal vomeronasal sensory neurons (VSNs) (arrow) in controls but have extended AP-2ε immunoreactivity into apical (magenta arrowheads) and sustentacular regions (white arrowheads) in ectopic mutants (D). (A1) AP-2ε, Meis2 double immunofluorescence shows segregated AP-2ε (white arrow) and Meis2 (green arrowheads) in controls. (D1) In the ectopic mutants AP-2ε expression is detected in Meis2+ cells (green arrowheads). (B,E) ISH against Gnao1 show that in controls (B) Gnao1 mRNA expression is restricted to the basal regions of the VNE. (E) Ectopic mutants show Gnao1 mRNA reactivity in the apical regions of the VNE (magenta arrowheads). (C,F) Immunohistochemistry against V2R2 in the VNO of controls (C) shows no immunoreactivity in the apical regions of the VNE in controls (notched arrows) and is limited to the basal VSNs (white arrows). (F) In mutants, more of the VNE was positive for V2R2 in mutants, as expression expands into the apical regions of the epithelium (magenta arrowheads). (G) Quantifications at P10 show a significant increase (p<0.0001 = ****) in the amount of the neuroepithelium positive for Gnao1 in OmpCre/R26AP-2ε mutants. Significance calculated using arcsine transformation on percent values; unpaired two-tailed t-test analysis. Error bars are standard deviation. N=10 for OmpCre controls and n=8 for OmpCre/R26AP-2ε mutants. (H) Quantifications at P10 show a significant increase in the number of the VSNs positive for V2R2 in OmpCre/R26AP-2ε mutants. Unpaired t-test. Error bars are standard deviation. N=3 for both OmpCre controls and OmpCre/R26AP-2ε mutants (p<0.05 = *). (I,J) Violin plot of Vmn2r7 (I), and Gnao1 (J) mRNA expression between apical and basal VSNs in OmpCre controls and apical VSNs of OmpCre/R26AP-2ε mutant mice show significant upregulation of these basal markers in mutants based on p-value (p<0.05 = *, p<0.0004 = ****). (K,L) Immunofluorescence against V2R2 (magenta) and Meis2 (green) in controls (K) and OmpCre/R26AP-2ε mutants (L). Arrows indicate a Meis2+ cell immunoreactive against anti-V2R2 antibodies in mutants (L). (M,N) Single-molecule FISH (RNAscope) against Gnao1, Gnai2, and Meis2 of P10 wild-type (WT) and OmpCre/R26AP-2ε mutants. In WTs (M), a clear segregation between the Gnao1+ (red) basal VSNs and the apical cells positive for Meis2+ (white) and Gnai2+ (green) could be seen. Arrows show Meis2+/Gnai2+ apical VSNs in control VNO tissue negative for Gnao1. In OmpCre/R26AP-2ε mutants (N) low but obvious expansion of Gnao1 (red) expression to the apical domains of the VNO. Signal highlighting Gnao1 expression could be found in Meis2+ (white) and Gnai2+ (green) cells. The arrows point to Meis2+/Gnai2+ apical VSNs with ectopic expression of Gnao1.
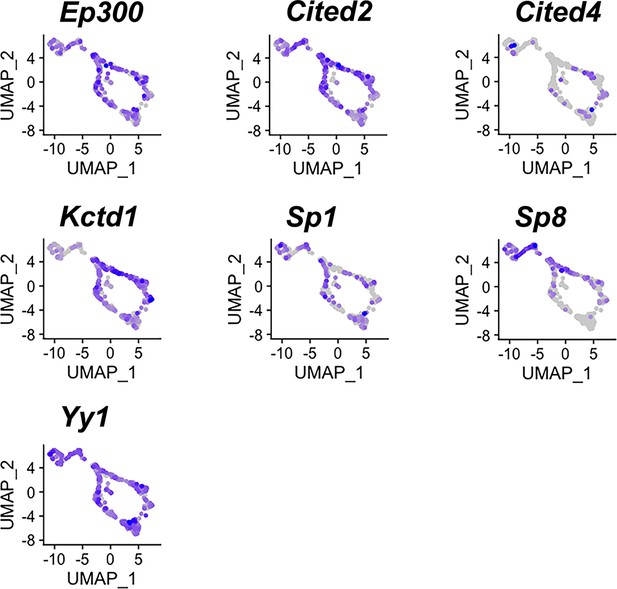
Expression of potential AP-2 cofactors in both apical and basal vomeronasal sensory neurons (VSNs).
Feature plots of known AP-2 family cofactors Ep300, Cited2, Cited4, Kctd1, Sp1, Sp8, Yy1 in P10 VSNs.
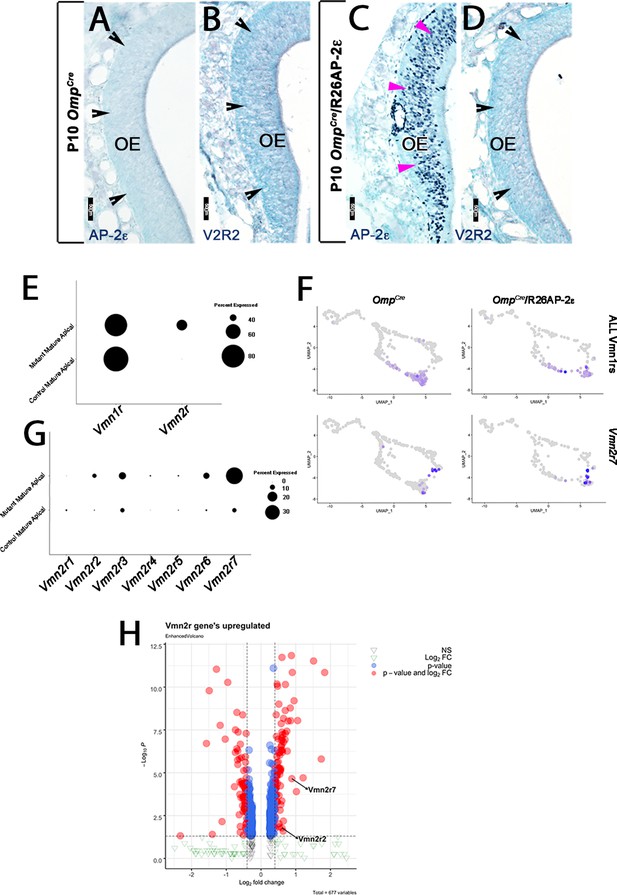
AP-2ε and V2R2 immunoreactivity in the MOE (main olfactory epithelium).
P10 OmpCre controls (A,B) and OmpCre/R26AP-2ε ectopic mutants (C,D). (A,C) Immunohistochemistry against AP-2ε in the main olfactory epithelium (OE) shows no immunoreactivity in controls (black notched arrows) and ectopic AP-2ε expression in the mutants (magenta arrowheads). (B,D) Immunohistochemistry against V2R2 in the main OE shows no expression (black notched arrowheads) in either control or ectopic mutants. (E) Dot plot shows that mutant mature apical neurons ectopically express Vmn2r. (F,G) Feature plot of OmpCre controls and OmpCre/R26AP-2ε mutants (F) and dot plot of mature control and mutant apical vomeronasal sensory neurons (VSNs) (G) shows that in mutants, there is increased expression of Vmn2r7 in apical VSNs. (H) Volcano plot showing upregulation of Vmn2rs in OmpCre/R26AP-2ε mutants.
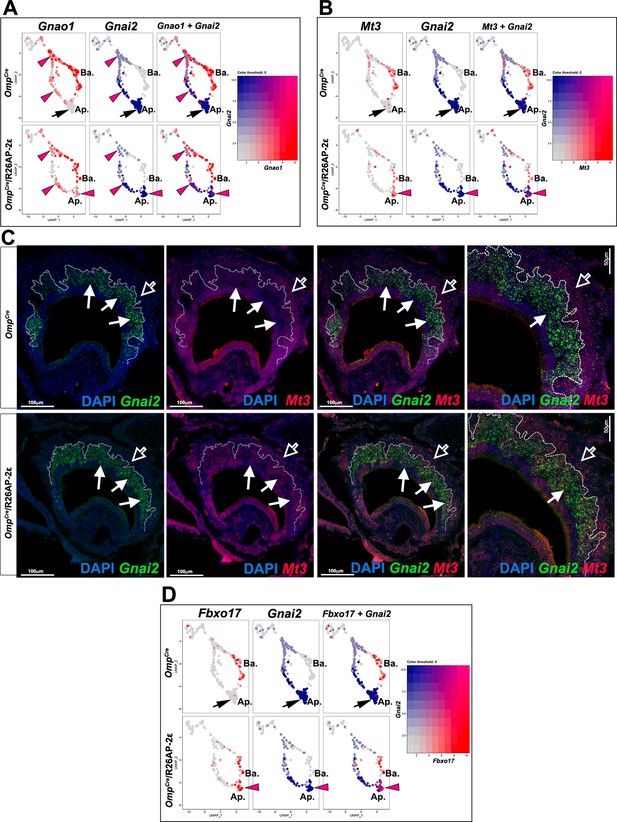
Ectopic expression in apical vomeronasal sensory neurons (VSNs) of indicated genes in OmpCre/R26AP-2ε mutants.
(A) Feature plot showing ectopic expression of Gnao1 in OmpCre/R26AP-2ε mutants. Black arrows indicate mature apical VSNs. Purple arrowheads indicate ectopic expression of Gnao1 in apical VSNs. (B) Feature plot showing ectopic expression of Mt3 in OmpCre/R26AP-2ε mutants. Black arrows indicate mature apical VSNs. Purple arrowheads indicate ectopic expression of Mt3 in apical VSNs. (C) RNAscope analysis shows that Mt3 is mainly expressed in basal VSNs in OMPCre controls (arrow outline) but ectopically expressed in Gnai2+ apical VSNs in OmpCre/R26AP-2ε mutants (arrows). (D) Feature plot showing ectopic expression of Fbxo17 in OmpCre/R26AP-2ε mutants. Black arrows indicate mature apical VSNs. Purple arrowheads indicate ectopic expression of Fbxo17 in apical VSNs.
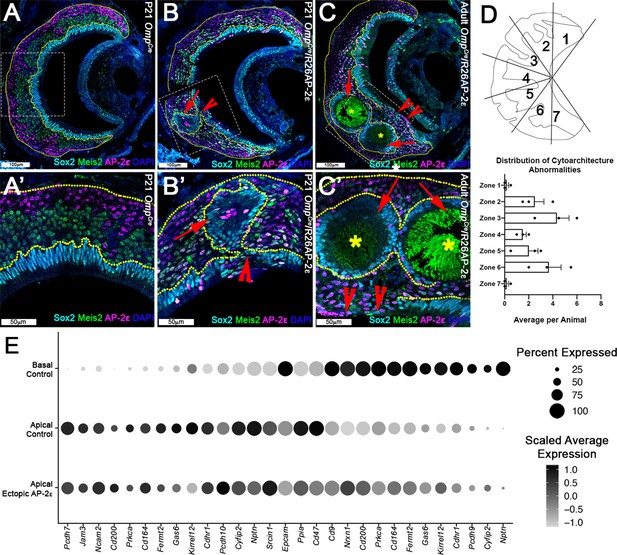
Progressive changes in vomeronasal epithelium (VNE) lamination may reflect the changing expression profiles of cell adhesion molecules in apical vomeronasal sensory neurons (VSNs).
Immunofluorescence against Sox2 (cyan), AP-2ε (magenta), and Meis2 (green) with 4’,6’-diamidino-2-phenylindole (DAPI) (blue) counterstain. Neuroepithelium traced in yellow dotted line. (A) P21 OmpCre controls show highly organized stratified neuroepithelium with contiguous layers of AP-2ε+/basal (magenta), Meis2+/apical (green), and Sox2+/sustentacular cell (cyan) layers. (B–C’) OmpCre/R26AP-2ε vomeronasal organ (VNO) at (B) P21 show that Sox2+/sustentacular cells have intraepithelial cysts with internalized subsidiary lumens (red arrows). (C) Adult (3mo) OmpCre/R26AP-2ε mutants show an increase in the severity of intraepithelial cysts (red arrows) and breaks in the sustentacular layer and expansion of neurons to the luminal surface (red notched arrows). Unidentified matter (*) reactive to anti-mouse Abs was detected within the cysts. (D) Quantifications of the zonal distribution through Zone 1 (dorsal) → Zone 7 (ventral) of these cytoarchitecture abnormalities (which include both cell body abnormalities and dendritic disorganization, each point = 1 animal) show that these disruptions occur in the intermediate and central regions of the VNO, but not in the marginal zones (zones 1, 7). The highest rate of occurrence are in zones 3 and 6, which are intermediate regions in the VNO. N=21. (E) Dot plot showing the composition and intensity of differentially expressed genes involved in cellular adhesion in the VSNs of controls and ectopic mutants.
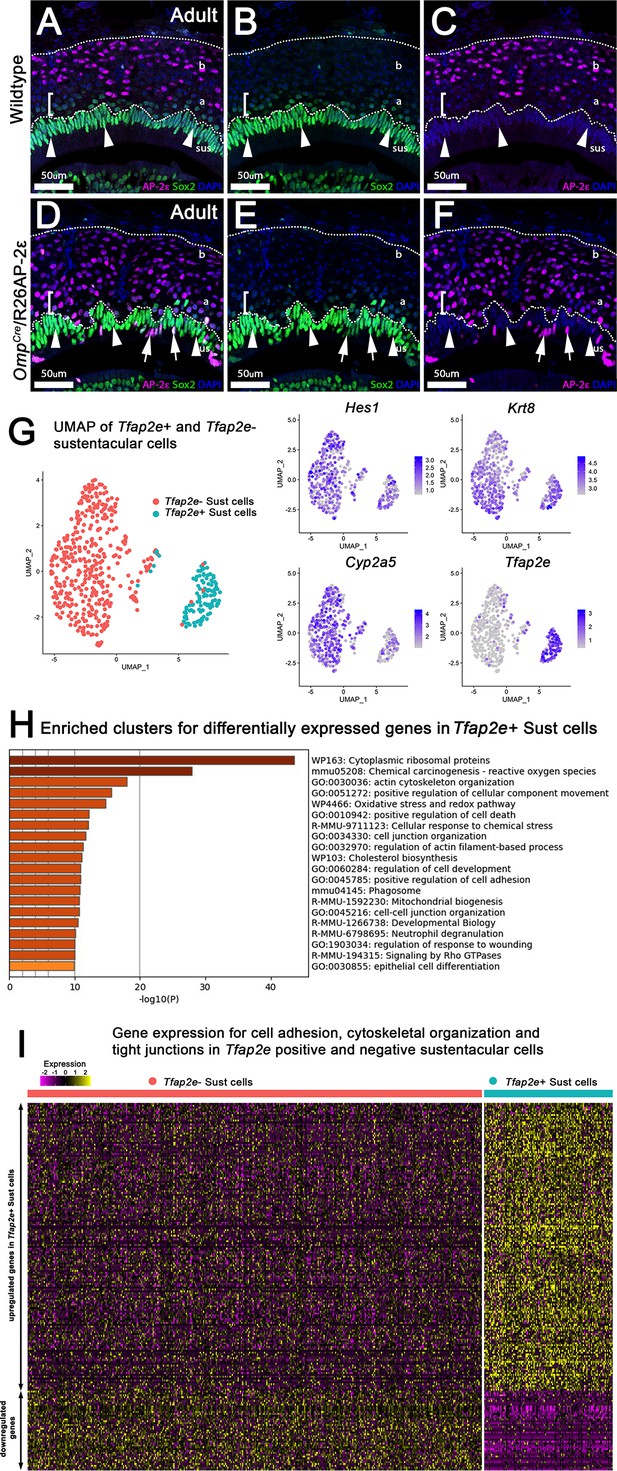
Analysis of sustentacular cells.
(A–F) Immunofluorescence on wild-type (WT) (A–C) and OmpCre/R26AP-2ε mutants (D–F) against AP-2ε (magenta) and Sox2 (green) counterstained with 4’,6’-diamidino-2-phenylindole (DAPI) (blue). (A–C) WT controls and ectopic mutants (D–F) show sustentacular (sus) cells with high immunoreactivity for Sox2 and no AP-2ε expression. Apical (a) vomeronasal sensory neurons (VSNs) closest to the sustentacular cell layer show low immunoreactivity for Sox2 (brackets, arrowheads) with apical VSNs closer to basal (b) VSNs with no immunodetectable Sox2 (notched arrows). No colocalization between AP-2ε and Sox2+ sustentacular cells (sus). (D–F) OmpCre/R26AP-2ε mice have some Sox2+ sustentacular cells that have ectopic AP-2ε expression (arrows). (G) Uniform Manifold Approximation and Projections (UMAPs) of sustentacular cells from OmpCre/R26AP-2ε adults showed that Tfap2e positive and negative clusters segregated from each other with accompanying feature plots showing the expression of sustentacular cell markers Hes1, Krt8, and Cyp2a5 along with ectopic Tfap2e mRNA expression. (H) Gene Ontology analysis of Tfap2e+ sustentacular cells showed an enrichment of dysregulated genes related to cytoskeleton organization, cell-cell junction organization, cytoskeletal organization, and cytoplasmic ribosomal proteins. (I) Heatmap of up- and downregulated genes found related to cytoskeleton organization, cell-cell junction organization, and cytoskeleton organization in Tfap2e positive and negative sustentacular cells.
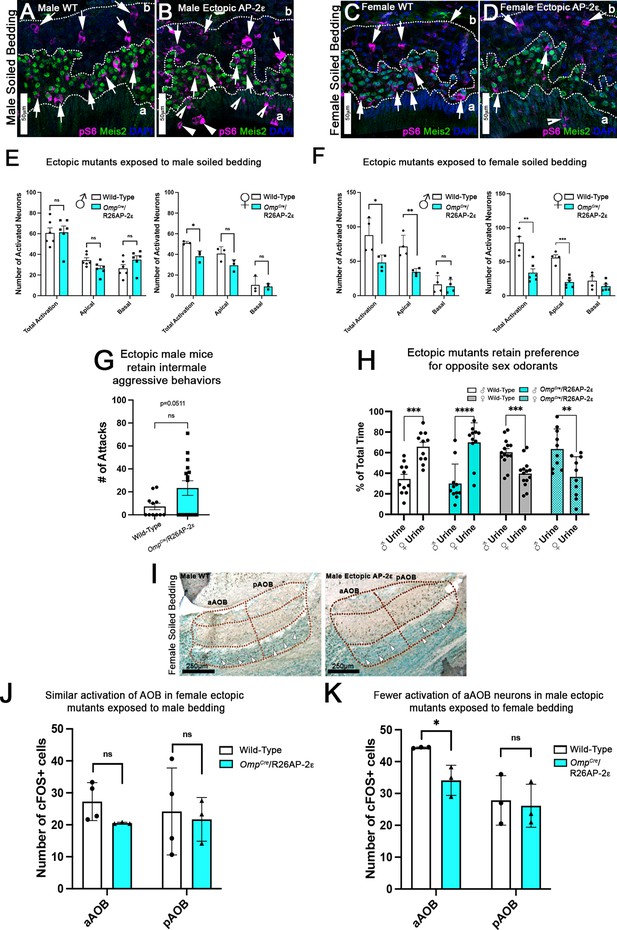
Ectopic Tfap2e expression alters the detection of sex-specific odorants.
(A–D) Immunofluorescence against pS6 (magenta, arrows) and Meis2 (green) with 4’,6’-diamidino-2-phenylindole (DAPI) counterstain (blue) in the vomeronasal epithelium (VNE) of controls (A,C) and OmpCre/R26AP-2ε mutants (B,D). (A,B) VNE of adult male wild-type (WT) and ectopic mutants when exposed to male-soiled bedding show similar activation or pS6 immunoreactivity (arrows) in Meis2+/apical and Meis2-/basal vomeronasal sensory neurons (VSNs). (C,D) VNE of adult female WT and ectopic mutants when exposed to female-soiled bedding show that while WT females displayed a higher proportion of pS6+ apical VSNs, OmpCre/R26AP-2ε mutants showed a decreased number of activated Meis2+/apical VSNs. In both exposure conditions ectopic activation of sustentacular cells (notched arrows) and VSNs near the lumen (arrowheads). (E) Quantifications of activated VSNs in male and female mice after exposure to male-soiled bedding show a non-significant decrease in activated apical VSNs in mutants of both sexes. Mutant females show a significant decrease in total activation of VSNs (p<0.05) while mutant males show a small but non-significant increase in the total number of activated basal VSNs. Unpaired t-test. Error bars are standard deviation. N=6 for males and n=3 females for both genotypes. (F) Quantifications of activated VSNs after exposure to female-soiled bedding in male and female mice show a significant decrease in the number of activated apical VSNs as well as total activation of VSNs between mutants and controls of both sexes (p<0.05 = *, p<0.01 = **, p<0.001 = ***). Unpaired t-test. Error bars are standard deviation. N=4 for males and n=6 for females for both genotypes. (G) Quantifications of the number of attacks for all WT and OmpCre/R26AP-2ε mutants in a resident intruder test show male ectopic mutant mice display higher levels of intermale aggression but are not significantly different than controls. Unpaired t-test. Error bars are standard deviation. N=11 for WTs and n=17 for OmpCre/R26AP-2ε mutants. (H) Quantifications of odorant preference tests in male and female mice show that male and female OmpCre/R26AP-2ε mutants retain the preference for opposite sex odorants (p<0.05 = *, p<0.01 = **, p<0.001 = ***, p<0.0001 = ****). Unpaired t-test. Error bars are standard deviation. N=11 for WT males, n=14 for WT females, n=11 for OmpCre/R26AP-2ε mutant males, and n=10 for OmpCre/R26AP-2ε mutant females. (I) Accessory olfactory bulb (AOB) of adult male WT and ectopic mutants when exposed to female-soiled bedding show that ectopic mutants have lower levels of cFOS activated neurons in the anterior AOB (aAOB) but similar levels of activated neurons in the posterior AOB (pAOB). (J) Quantification of cFOS activated neurons in the AOB of female mice exposed to male-bedding show no significant differences between aAOB and pAOB activation in mutants and WT. Unpaired t-test. Error bars are standard deviation. N=4 for WT and n=3 for OmpCre/R26AP-2ε mutants. (K) Quantification of cFOS activated neurons in the AOB of male mice exposed to female-soiled bedding show a significant reduction in aAOB activation (p<0.05) in mutants compared to WT. Unpaired t-test. Error bars are standard deviation. N=3 for both WT and OmpCre/R26AP-2ε mutants.
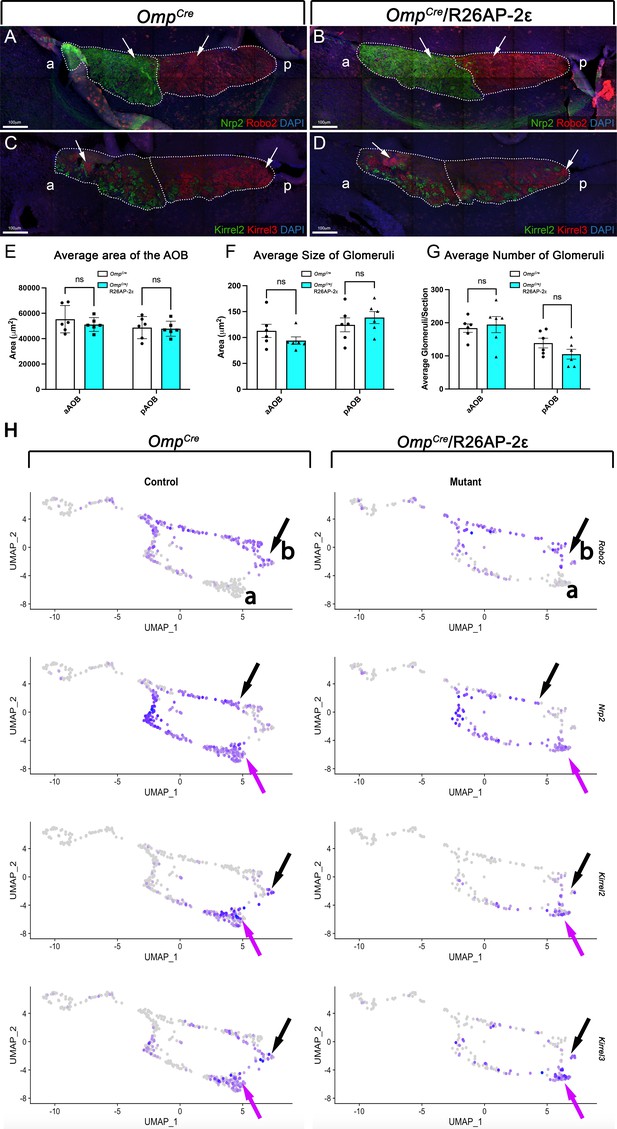
Analysis of the accessory olfactory bulbs (AOBs) from adult wild-type (WT) and OmpCre/R26AP-2ε mice.
(A,B) Immunofluorescence against Nrp2 (green) and Robo2 (red) and counterstained with 4’,6’-diamidino-2-phenylindole (DAPI) (blue) showed that the expression of guidance cue molecules in WT (A) and OmpCre/R26AP-2ε mutant (B) mice was comparable with distinct anterior and posterior AOB regions (arrows). (C,D) Immunofluorescence against Kirrel2 (green) and Kirrel3 (red) and DAPI (blue) counterstain on WT (C) and OmpCre/R26AP-2ε mutant mice (D) AOBs showed that the glomerular organization and morphology was comparable between genotypes (arrows). (E) Quantifications of the average area of the AOB was not significantly different across genotypes. Analysis of the glomeruli size (F) and number (G) using IF against Kirrel2 and Kirrel3 showed no significant changes across genotypes. Unpaired t-test. Error bars are standard deviation. N=6 for OmpCre controls and OmpCre/R26AP-2ε mutants (E–G).
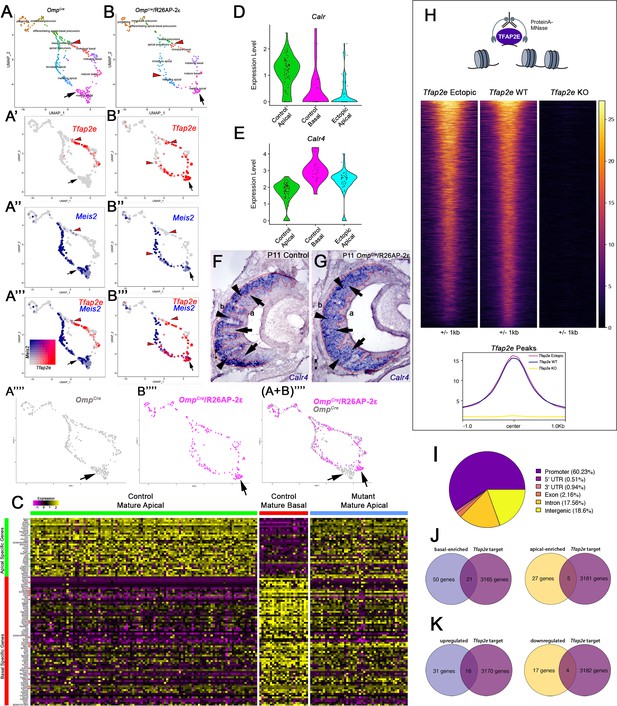
Single-cell sequencing of P10 OmpCre control and OmpCre/R26AP-2ε mutant vomeronasal sensory neurons (VSNs) indicate a shift in apical cells toward basal cells in the mutant.
(A–A’’’) Uniform Manifold Approximation and Projection (UMAP) clustering of VSNs from progenitor cells to differentiated mature apical and basal cells of control. (B–B’’’) Mutant mice split by genotype. (A’–A’’’, B’–B’’’) Blended feature plots of Tfap2e expression (red) and Meis2 (blue). Red arrowheads indicate the onset of Tfap2e expression. Black arrow indicates mature apical VSNs. OmpCre controls (A’–A’’’) show a divergent pattern of expression where the onset of Tfap2e (red, red arrowhead) is only on the basal branch. Meis2 expression (blue) occurs only on the apical branch where the cells lack Tfap2e expression. OmpCre/R26AP-2ε mutants (B’–B’’’) start to express Tfap2e on the basal branch in immature basal VSNs (red) however, onset of Tfap2e mRNA expression also occurs on the apical branch (blue). (B’’’) In ectopic mutants, Tfap2e mRNA is co-expressed with Meis2 in apical VSNs (purple cells). (A’’’’) Feature plot of apical cells in OmpCre controls (black arrow). (B’’’’) Feature plot of apical cells in OmpCre/R26AP-2ε mutants (black arrow). (A+B’’’’) Overlay shows that apical cells with ectopic Tfap2e expression (magenta, black arrow) clustered separately from mature apical cells of OmpCre controls (gray, black arrow). (C) Heatmap showing up/downregulated genes in mature apical and basal VSNs of OmpCre controls and in apical VSNs of OmpCre/R26AP-2ε mutants. Apical VSNs of ectopic mutants express genes enriched in both apical and basal VSNs. Gene names and values are available in Supplementary file 1, Supplementary file 2. (D) Violin plot shows Calreticulin (Calr) mRNA expression levels in apical VSNs from the OmpCre/R26AP-2ε mutants are reduced to levels similar to basal VSNs from OmpCre controls. (E) Violin plot shows Calreticulin-4 (Calr4) mRNA expression levels. (E) In control cells, Tfap2e mRNA expression (red arrowheads) and Meis2 mRNA expression (blue) are not co-expressed in the same cells. Tfap2e expression is upregulated in immature basal VSNs and not apical VSNs. (F,G) In situ hybridization (ISH) against Calr4 against P11 OmpCre controls (F) and OmpCre/R26AP-2ε mutants (G) show that while Calr4 mRNA is normally enriched in basal VSNs (arrowheads), ectopic mutants show expansion of Calr4 positivity in the apical regions when compared to controls (arrows). (H–K) Analysis of CUT&RUN against AP-2ε. (H) Tornado plot of AP-2ε occupancy in Tfap2e ectopic, wild-type (WT) and knockouts (KOs) in the dissociated tissue of the vomeronasal organ (VNO). AP-2ε signal in ectopic mutants and WTs is similar across all AP-2ε peaks, while Tfap2e KOs show no signal. The genomic regions are defined as the summit±1 kb. (I) Pie chart depicting the genomic distribution of putative AP-2ε binding sites show that most of AP-2ε peaks are found in promoter regions of putative target genes and to a lesser extent in intergenic and intronic regions of the genome. (J) Venn diagram of the determined AP-2ε targets and the genes enriched in the basal and apical VSNs. (K) Venn diagram of the determined AP-2ε targets and all the upregulated and downregulated genes in the ectopic mutant mouse. Significance defined as adjusted p-value ≤0.05.
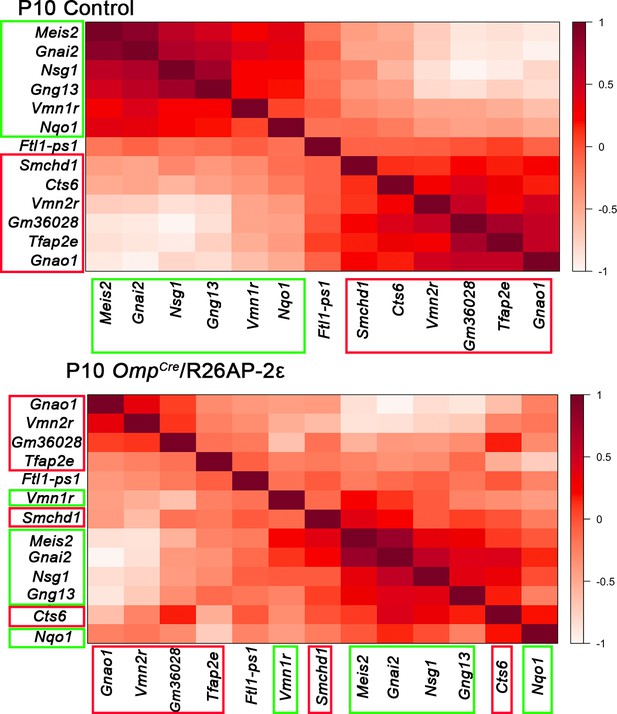
Gene expression correlation changes after ectopic Tfap2e expression.
In control animals, specific sets of genes are expressed with high correlation level in either apical vomeronasal sensory neurons (VSNs) (boxed in green) or basal VSNs (boxed in red). Genes with higher expression correlation are close to each other in either X or Y axis, the legend indicates higher correlation of expression with darker shade of red. In OmpCre/R26AP-2ε mutants, ectopic expression alters the cell type-specific genes indicated by the different order of genes and different shades of red/expression correlation. These show that ectopic Tfap2e expression alters the normal correlation of apical and basal enriched genes.
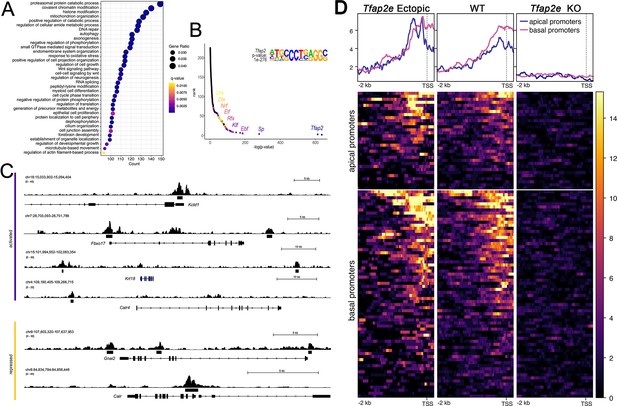
CUT&RUN analysis of Tfap2e ectopic, wild-type (WT) and knockout (KO) mice.
(A) Dot plot of enriched Gene Ontology (GO) terms shows many putative AP-2ε target genes being chromatin or histone modifiers. (B) Motif enrichment analysis validated our analysis as Tfap2 was the top motif found at putative binding sites. It also yielded transcription factor (TF) family motifs of potential cofactors including Sp, Klf, Ebf, Rfx, Elk, Nrf, Dlx, and Lhx TF families. (C) Tracks from CUT&RUN sequencing where AP-2ε peaks are found in the promoter regions of activated (purple) and repressed (yellow) genes. (D) Promotor plots of AP-2ε binding in ectopic mutants, WTs and KOs. In WTs, there is more Tfap2e binding at basal promotors compared to apical, however, in ectopic mutants, apical and basal binding is similar due to Tfap2e ectopic expression. Tfap2e KOs show little to no signal.
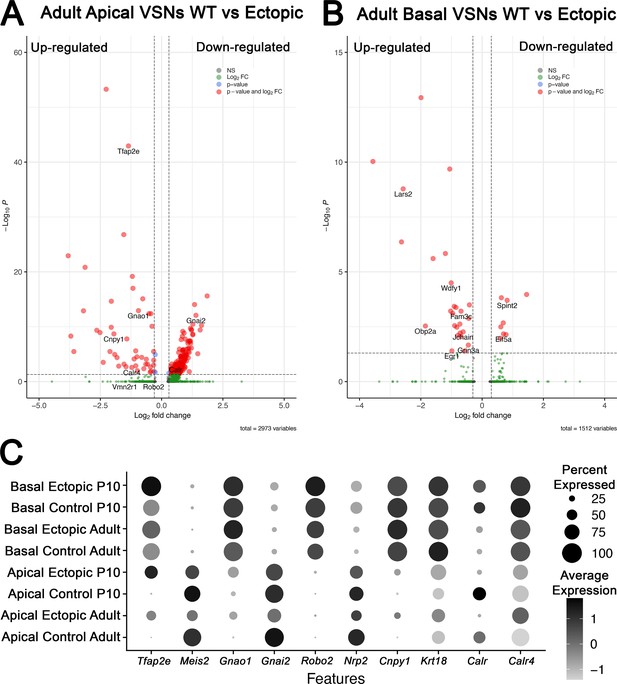
Differential gene expression analysis from single-cell RNA sequencing (scRNA-seq) in adult animals of apical and basal populations scRNA-seq in adults wild-type (WT) controls and OmpCre/R26AP-2ε vomeronasal organs (VNOs).
(A) Volcano plot of significantly up- and downregulated genes in apical vomeronasal sensory neurons (VSNs) in adult WT and OMPCre/R26AP-2ε mice show dysregulation of many key basal genes and downregulation of apical-enriched genes. (B) Volcano plot of significantly up- and downregulated genes in basal VSNs in adult WT and OMPCre/R26AP-2ε mice show that some genes may respond to Tfap2e regulation in a dose-dependent manner. (C) Dot plot of canonical genes expressed by apical and basal VSNs, and key genes identified in scRNA-seq analysis for P10 and adult control and mutant mice.
Tables
Reagent type (species) or resource | Designation | Source or reference | Identifiers | Additional information |
---|---|---|---|---|
Antibody | Anti-gao (Rabbit polyclonal) | Invitrogen | PA5-59337 | (1:1000) |
Antibody | Anti-gao (Mouse monoclonal) | Synaptic Systems | 271 111 | (1:250) |
Antibody | Anti-gai2 (Mouse monoclonal) | Millipore | MAB3077 | (1:250) |
Antibody | Anti-keratin 18 (Rabbit polyclonal) | Abcam | ab52948 | (1:500) |
Antibody | Anti-kirrel2 (Goat polyclonal) | R&D Systems | AF2930 | (1:500) |
Antibody | Anti-kirrel3 (Mouse monoclonal) | NeuroMab | 75–333 | (1:100) |
Antibody | Anti-meis2 (Mouse monoclonal) | Santa Cruz | sc-81986 | (1;500) |
Antibody | Anti-meis2 (Rabbit polyclonal) | Abcam | ab73164 | (1:1000) |
Antibody | Anti-Nrp2 (Goat polyclonal) | R&D Systems | AF567 | (1:4000) |
Antibody | Anti-olfactory marker protein (Goat polyclonal) | WAKO | 54410001 | (1:4000) |
Antibody | Anti-phospho-S6 Ribosomal Protein (Ser240/244) (Rabbit monoclonal) | Cell Signaling Technology | D68F8 | (1:500) |
Antibody | Anti-robo2 (Mouse monoclonal) | Santa Cruz | sc-376177 | (1:250) |
Antibody | Anti-sox2 (Goat polyclonal) | R&D Systems | AF2018 | (1:800) |
Antibody | Anti-AP-2ε (goat polyclonal) | R&D Systems | AF5060 | (1:500) |
Antibody | Anti-AP-2ε (Rabbit polyclonal) | ProteinTech Group | 25829–1-AP | (1:500) |
Antibody | Anti-c-Fos (Rabbit monoclonal) | Abcam | ab53212 | (1:500) |
Antibody | V2R2 (Rabbit polyclonal) | Roberto Tirindelli (University of Parma) | (1:5000) | |
Antibody | Anti-goat (Alexa488) (Donkey polyclonal) | Molecular Probes | a11055 | (1:1000) |
Antibody | Anti-goat (Alexa594) (Donkey polyclonal) | Molecular Probes | a11058 | (1:1000) |
Antibody | Anti-goat (biotinylated) (Horse polyclonal) | Vector | ba9500 | (1:1000) |
Antibody | Anti-mouse (Alexa488) (Donkey polyclonal) | Molecular Probes | a21202 | (1:1000) |
Antibody | Anti-mouse (Alexa594) (Donkey polyclonal) | Molecular Probes | a10037 | (1:1000) |
Antibody | Anti-mouse (biotinylated) (Horse polyclonal) | Vector | ba2000 | (1:1000) |
Antibody | Anti-rabbit (Alexa488) (Donkey polyclonal) | Molecular Probes | a21206 | (1:1000) |
Antibody | Anti-rabbit (Alexa594) (Donkey polyclonal) | Jackson Labs | 711-585-152 | (1:1000) |
Antibody | Anti-rabbit (biotinylated) (Horse polyclonal) | Vector | ba1100 | (1:1000) |
Sequence-based reagent | RNAscope Probe-Mm-Abca7-C4 | Acdbio | 489021 | |
Sequence-based reagent | RNAscope Probe-Mm-Gnai2 | Acdbio | 868051 | |
Sequence-based reagent | RNAscope Probe-Mm-Gnao1-E4-E6-C2 | Acdbio | 444991 | |
Sequence-based reagent | RNAscope Probe-Mm-Mt3-C3 | Acdbio | 504061 | |
Sequence-based reagent | RNAscope Probe-Mm-Meis2-C3 | Acdbio | 436371 | |
Chemical compound, drug | Neuron Isolation Enzyme (with papain) | Thermo Fisher Scientific | 88285 | |
Chemical compound, drug | Neurobasal Medium | Thermo Fisher Scientific | 21103049 | |
Chemical compound, drug | Dimethyl sulfoxide (DMSO) | Sigma-Aldrich | 472301 | |
Chemical compound, drug | Diaminobenzidine (DAB) | Sigma | D9015-100MG | (250 μg/ml) |
Chemical compound, drug | DAPI | CALBIOCHEM | 268298 | (1:3000) |
Commercial assay or kit | DIG Labelling Kit | Roche | 11 175 025 910 | |
Commercial assay or kit | DIG Detection Kit | Roche | 11 175 041 910 | |
Chemical compound, drug | 37% Formaldeyde | Sigma | F1635-500ML | |
Chemical compound, drug | Tissue-Tek O.C.T. Compound | VWR | 25608-930 | |
Commercial assay or kit | ABC HRP Kit | Vector | PK-6100 | |
Commercial assay or kit | NEBNext Ultra II DNA Library Prep Kit for Illumina | New England Biolabs | E7645 | |
Commercial assay or kit | RNAscope Intro Pack for Multiplex Fluorescent Reagent Kit v2- Mm | Acdbio | 323136 | |
Commercial assay or kit | RNAscope 4-Plex Ancillary Kit for Multiplex Fluorescent Kit v2 | Acdbio | 323120 | |
Biological sample (Mus musculus) | Tfap2eCre | Trevor Williams (University of Colorado, Denver) | ||
Biological sample (Mus musculus) | B6;129P2-Omptm4(cre)Mom/MomJ | Jackson Labs | Stock No: 006668 | |
Biological sample (Mus musculus) | B6.Cg-Gt(ROSA)26Sor tm(CAG-mTfap2e)For (R26AP-2ε) | This paper | Will Be available from Jackson Labs | |
Software, algorithm | FIJI ImageJ software (Version 2.1.0/1.53c) | NIH | https://imagej.nih.gov/ij/ | |
Software, algorithm | GraphPad Prism 9 software | GraphPad | https://www.graphpad.com/ | |
Software, algorithm | Photoshop CC 2020 | Adobe | https://www.adobe.com/products/photoshop.html | |
Software, algorithm | RStudio (Version 1.3.1073) | RStudio | https://rstudio.com/ | |
Software, algorithm | R version 4.0.2 | The R Project | https://www.r-project.org/ | |
Software, algorithm | Seurat 4.0.5 | Satija Lab | https://satijalab.org/seurat/ | |
Software, algorithm | ButtonBox v.5.0 | Behavioral Research Solutions, LLC | Shared by Damien Zuloaga (University at Albany) as Software is discontinued | |
Software, algorithm | Cutadapt | Martin, 2011 | https://cutadapt.readthedocs.io/en/stable/ | |
Software, algorithm | Bowtie 2 | Langmead and Salzberg, 2012 | http://bowtie-bio.sourceforge.net/bowtie2/index.shtml | |
Software, algorithm | MACS2 | Zhang et al., 2008 | https://chipster.csc.fi/manual/macs2.html | |
Software, algorithm | ChIPseeker | Yu et al., 2015 | https://bioconductor.org/packages/release/bioc/html/ChIPseeker.html | |
Software, algorithm | clusterProfiler | Yu et al., 2012 | https://bioconductor.org/packages/release/bioc/html/clusterProfiler.html | |
Software, algorithm | HOMER | Benner Lab | http://homer.ucsd.edu/homer/ | |
Sequence-based reagent | GGA GGG GGG CTCT GAG AT | This paper | R26-AP-2ε Common | Will Be available from Jackson Labs |
Sequence-based reagent | GGC TGG TGT GGC CAA TGC | This paper | R26-AP-2ε Mutant | Will Be available from Jackson Labs |
Sequence-based reagent | GTC GTG AGG CTG CAG GTC | This paper | R26-AP-2ε WT | Will Be available from Jackson Labs |
Sequence-based reagent | AGT TCG ATC ACT GGA ACG TG | Jackson Labs | Omp WT Fwd | |
Sequence-based reagent | CCC AAA AGG CCT CTA CAG TCT | Jackson Labs | Omp WT Rvs | |
Sequence-based reagent | TAG TGA AAC AGG GGC AAT GG | Jackson Labs | Omp Mutant Fwd | |
Sequence-based reagent | AGA CTG CCT TGG GAA AAG CG | Jackson Labs | Omp Mutant Rvs | |
Sequence-based reagent | GCT GGT GAG TCA ACC TGC CTG CAG | Trevor Williams (University of Colorado, Denver) | AP-2ε WT AK19 | |
Sequence-based reagent | GGT CAC CTT GTA CTT GGA TGT TGA G | Trevor Williams (University of Colorado, Denver) | AP-2ε WT AK20 | |
Sequence-based reagent | AGG TGT AGA GAA GGC ACT TAG C | Jackson Labs | Cre Fwd | |
Sequence-based reagent | CTA ATC GCC ATC TTC CAG CAG G | Jackson Labs | Cre Rvs |
Additional files
-
Supplementary file 1
Differentially expressed genes in mature apical and basal vomeronasal sensory neurons (VSNs).
- https://cdn.elifesciences.org/articles/77259/elife-77259-supp1-v2.docx
-
Supplementary file 2
Significantly dysregulated genes when Tfap2e is ectopically expressed in apical vomeronasal sensory neurons (VSNs).
- https://cdn.elifesciences.org/articles/77259/elife-77259-supp2-v2.docx
-
Transparent reporting form
- https://cdn.elifesciences.org/articles/77259/elife-77259-transrepform1-v2.docx