A dopamine-gated learning circuit underpins reproductive state-dependent odor preference in Drosophila females
Figures
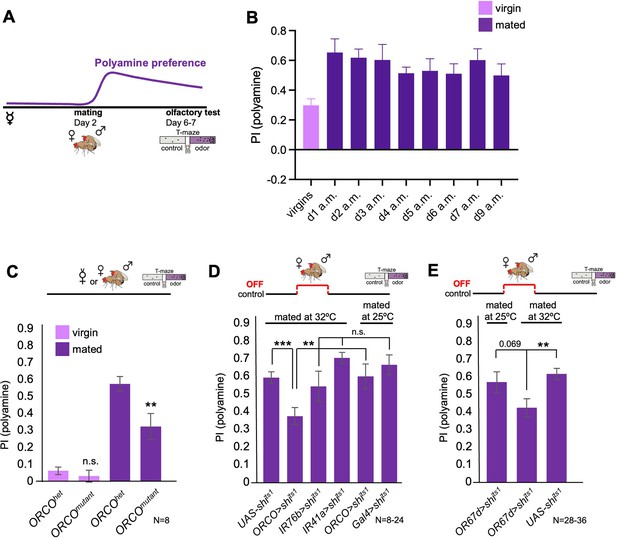
Odor experience at mating changes female attraction to polyamines.
(A) Scheme depicting paradigm used in all experiments. Virgin females were kept alone for 6–7 days or mated on day 2 for 24 hr, then separated, and test at day 6–7 for their preference for the odor of the polyamine, putrescine. Mated females show a high preference for polyamine, which depends on mating-induced modulation of polyamine-detecting olfactory neurons (see text for details). (B) CantonS females before mating (virgins) and 1–9 days after mating (a.m.) were analyzed for their preference to putrescine odor as groups in a T-maze. (C) Females mutant for ORCO (ORCOmutant) showed a significantly reduced preference for polyamines during olfactory preference test compared to controls (ORCOhet). Note that ORCO is not required for the detection of polyamine odor, but for the detection of pheromones and other food related odors. T-maze group assay. Bars depict preference index (PI) ± SEM. Student-s t-test; p-values: n.s.:>0.05, *:<0.05, **:<0.01 (D) T-maze group assay for females mated at restrictive temperature (32 °C) and tested at permissive temperature for their polyamine preference (25 °C). In addition, ORCO>shits1 and pooled IR76b and IR41a-Gal4>shits1 females were mated and tested at 25 °C as a control. Note that synaptic inhibition of ORCO-expressing OSNs during mating significantly reduced the females’ attraction to putrescine odor. T-maze group assay. (E) T-maze group assay for females mated at restrictive temperature (32 °C) or at permissive temperature (25 °C) and tested at permissive temperature for their polyamine preference (25 °C). Note that increased temperature at test leads to a slightly higher attraction to polyamine as compared to testing at 25 °C. This might explain why the difference between flies of the same genotype tested at different temperatures is reduced by not significantly. Bars depict preference index (PI) ± SEM. Student-s t-test; p-values: n.s.:>0.05, *:<0.05, **:<0.01. N>8 groups of 20–40 female flies.
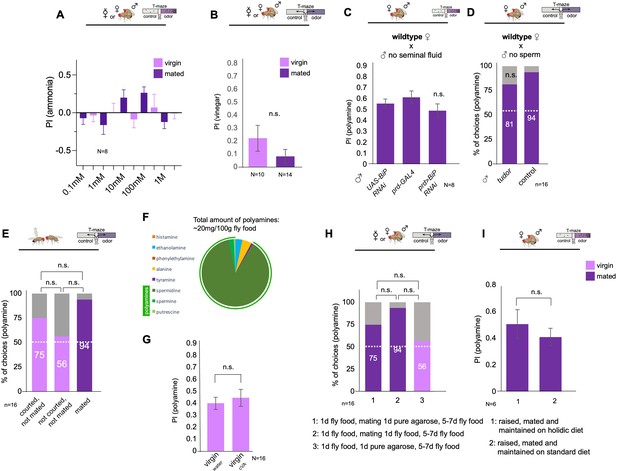
Analysis of the role of polyamines and mating-related cues.
(A) Mating does not significantly increase the attraction of females to different concentrations of ammonia.(B) Mating does not increase the attraction of females to vinegar in the T-maze. Note that, like in the putrescience and ammonia T-maze experiments, females were not starved. (C) Seminal fluid is not required to induce postmating polyamine preference. Wildtype CantonS females were mated with males without seminal fluid (prd-Gal4;UAS-BiP RNAi) or with control males (prd-Gal4/+ or UAS-BiP RNAi/+). (D) Sperm is not essential for postmating polyamine preference induction. Wildtype CantonS females were mated with sterile males unable to produce sperm (sons of tudor-deficient females) or with control males (control). Single female preference for putrescine in T-maze assay. 50% represents chance. (E) Courtship without mating induces higher preference in virgin females. Note that preference of courted, not mated virgins is not significantly different from mated females (p>0.1). Single female preference for putrescine in T-maze assay. 50% represents chance. (F) Standard fly food contains high levels of polyamines, spermine, spermidine and putrescine. (G) Wildtype CantonS females were exposed in food vials for 24 hr to water or to cVA (pure) and then tested in the T-maze assay for their preference for putrescine. No significant difference was found between the two groups. (H) Wildtype CantonS females were mated to wildtype males on different substrates. Mating for 24 hr on pure agarose reduced mated female attraction to polyamines. Females behaved not significantly different from virgins (a, p>0.1) and females mated on regular fly food (b, p>0.1). Single female preference for putrescine in T-maze assay. 50% represents chance. (I) Group T-maze preference assay with females raised and mated on holidic diet or standard fly food. No significant difference was found between the groups. Depicted p-values for single fly assay, unless indicated otherwise, refer to the difference between experimental and control group in virgins or mated females, respectively. Fisher’s exact test. p-values: n.s.:>0.1, *:<0.1, **:<0.05, ***:<0.01. T-maze group assay. Bars depict preference index (PI) ± SEM. Student-s t-test; p-values: n.s.:>0.05, *:<0.05, **:<0.01.
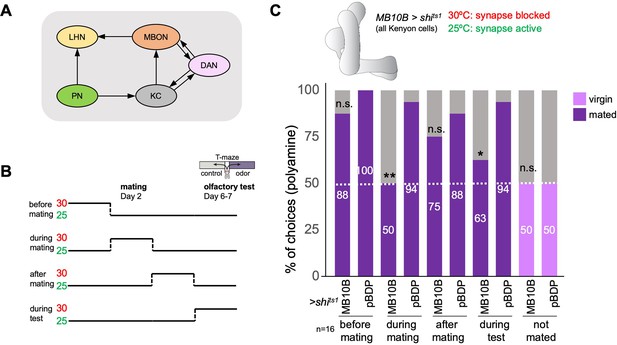
The mushroom body and learning mechanisms are required in long-term preference change upon mating.
(A) Simplified schematic overview of the observed connections between neurons in the olfactory system. PN: projection neuron; LHN: lateral horn neuron; KC: Kenyon cell; DAN: dopaminergic neuron; MBON: mushroom body output neuron. Olfactory sensory neurons connect to PNs in the antennal lobe. PNs project to the two higher olfactory center, the mushroom body (MB) and the lateral horn (LH). In the MB, they provide input to KCs. In the LH, they connect to various types of LHNs. KCs form excitatory synapses onto MBONs. DANs form modulatory synapses onto KCs and MBONs. Note the existence of several recurrent connections. Selected MBONs also project to the LH and make connections with LHNs. This canonical motif can be found in all 15 MB compartments along the MB lobes. Apart from these synaptic connections, additional connections have been shown or proposed. See text for more details. (B) Scheme of experimental paradigm used to block Kenyon cell (KC) synaptic output at precise times during the experiment. Shibirets1 was expressed under the control of MB10B-Gal4 in all Kenyon cells (KCs). Temperature shifts from 25 to 30℃ at four different time points were used to inhibit KC output before, during, after mating and at test, respectively. At days 6–7 after mating, females were given the choice between the odor of water (control) and the odor of putrescine in a T-maze assay. (C) KC synaptic output is required during mating and during preference test. Inhibition of KC output in virgin females during test did not affect putrescine odor preference. Single female preference for putrescine in T-maze assay. 50% represents chance. Fisher’s exact test. All p-values depict the comparison between the respective control (pBDP-Gal4;UAS-shits1) and the text group (MB10B-Gal4;UAS-shits1). p-values: n.s.:>0.1, *:<0.1, **:<0.05, ***:<0.001. n=16 single female flies.
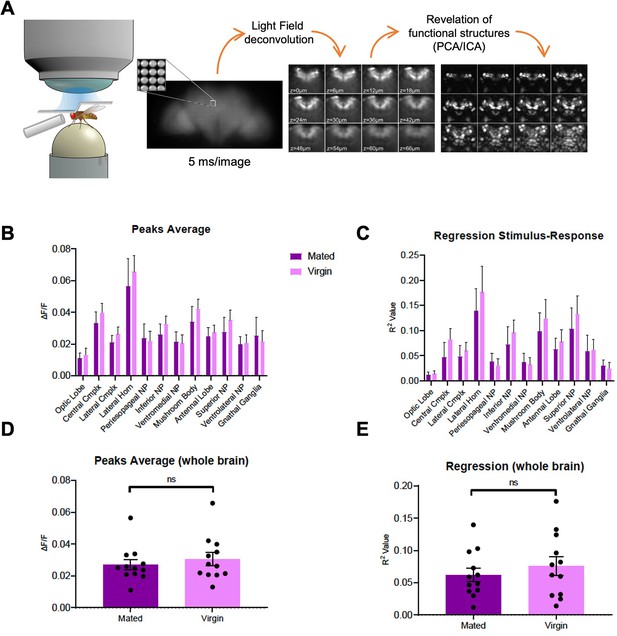
Whole brain imaging of polyamine odor responses in mated and virgin flies.
(A) Schematic overview of whole brain lightfield imaging method. For details see Aimon et al., 2019 (B–E) Peak GCaMP fluorescence (∆F/F) and regression analysis (R-square) of in vivo calcium whole brain lightfield imaging reveals no global differences in brain state or brain region activity between virgin and mated females (ANOVA).
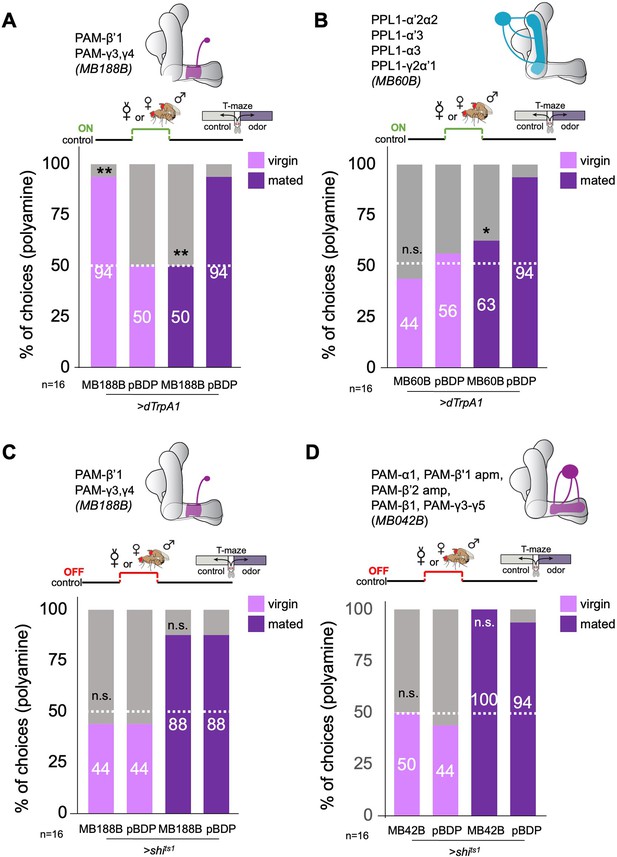
β’1 dopaminergic neuron activation can replace mating to induce higher polyamine preference.
(A) Activation of PAM-β’1 output (MB188B-Gal4;UAS-dTrpA1) instead of mating significantly increased virgin female preference for putrescine at the time of test. Vice versa, it significantly decreased the mated female’s preference for the same at testing. (B) Activation of PPL1-α’2α2, α3, γ2α’1 output (MB60B-Gal4;UAS-dTrpA1) instead of mating also significantly decrease mated female preference for putrescine at the time of test. Note that virgin behavior was not affected. Single female preference for putrescine in T-maze assay. 50% represents chance. (C) Inhibition of PAM-β’1 dopaminergic neuron output (MB188B-Gal4;UAS-shits1) during or instead of mating had no significant effect on mated or virgin female preference for putrescine at the time of test, respectively. (D) Inhibition of a large subset of PAM dopaminergic neuron output (MB042B-Gal4;UAS-shits1) during or instead of mating had no significant effect on mated or virgin female preference for putrescine at the time of test, respectively. Single female preference for putrescine in T-maze assay. 50% represents chance. Fisher’s exact test; All p-values depict the comparison between the respective control (pBDP-Gal4;UAS-xx) and the text group (MBx-Gal4;UAS-xx). p-values: n.s.:>0.1, *:<0.1, **:<0.05, ***:<0.001. n=16 single female flies.
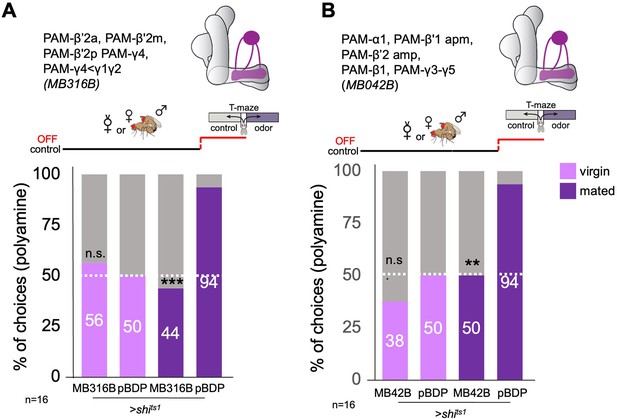
Role of PAM dopaminergic neurons during preference test.
(A) Inhibition of PAM dopaminergic neuron output (MB316B-Gal4;UAS-shits1) during test significantly decreased mated female preference for putrescine at the time of test. (B) Inhibition of PAM dopaminergic neuron output (MB042B-Gal4;UAS-shits1) during test significantly decreased mated female preference for putrescine at the time of test. Single female preference for putrescine in T-maze assay. 50% represents chance. Fisher’s exact test; All p-values depict the comparison between the respective control (pBDP-Gal4;UAS-xx) and the text group (MBx-Gal4;UAS-xx). p-values: n.s.:>0.1, *:<0.1, **:<0.05, ***:<0.001. n=16 single female flies.
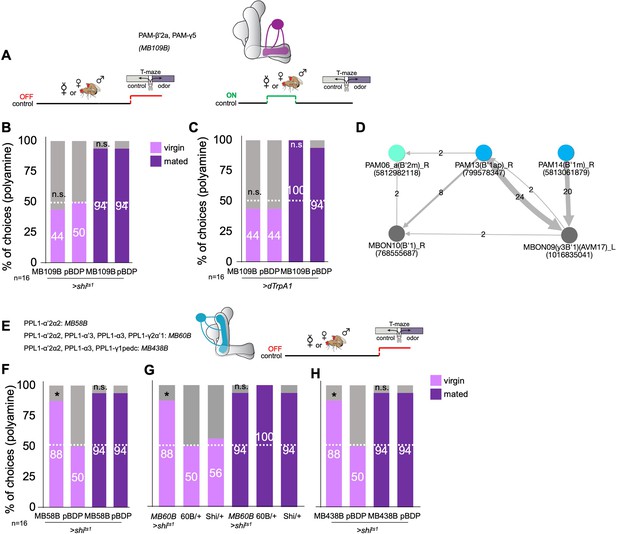
PAM and PPL dopaminergic neurons during mating or at choice.
(A) Left, schematic representation and description of PAM neuron transgenic lines. right, scheme of experimental paradigm. Neuronal output was blocked during odor preference test. (B) Inhibition of PAM dopaminergic neuron output (MB109B-Gal4;UAS-shits1) during test had no significant effect on mated female preference for putrescine at the time of test. Single female preference for putrescine in T-maze assay. 50% represents chance. (C) Activation of PAM dopaminergic neuron output (MB109B-Gal4;UAS-shits1) during or instead of mating had no significant effect on mated or virgin female preference for putrescine at the time of test. Single female preference for putrescine in T-maze assay. 50% represents chance. (D) EM-based connectome showing synaptic connections of PAM-β’1 neurons. (E) Left, scheme of PPL1 cluster of dopaminergic neurons and expression of three different transgenic lines. right, scheme showing experimental paradigm. Neuronal output was inhibited at the time of olfactory test. (F) Inhibition of PPL1-α’2α2 output (MB58B-Gal4;UAS-shits1) during testing significantly increased virgin female preference for putrescine at the time of test. Single female preference for putrescine in T-maze assay. 50% represents chance. (G) Inhibition of PPL1-α’2α2, α3, γ2α’1 output (MB60B-Gal4;UAS-shits1) during testing also significantly increased virgin female preference for putrescine at the time of test. Single female preference for putrescine in T-maze assay. 50% represents chance. (H) Inhibition of PPL1-α’2α2, α3, γ1pedc output (MB438B-Gal4;UAS-shits1) during testing significantly increased virgin female preference for putrescine at the time of test. Single female preference for putrescine in T-maze assay. 50% represents chance. All p-values depict the comparison between the respective control (pBDP-Gal4;UAS-shits1) and the text group (MB10B-Gal4;UAS-shits1). p-values: n.s.:>0.1, *:<0.1, **:<0.05, ***:<0.001. n=16 single female flies.
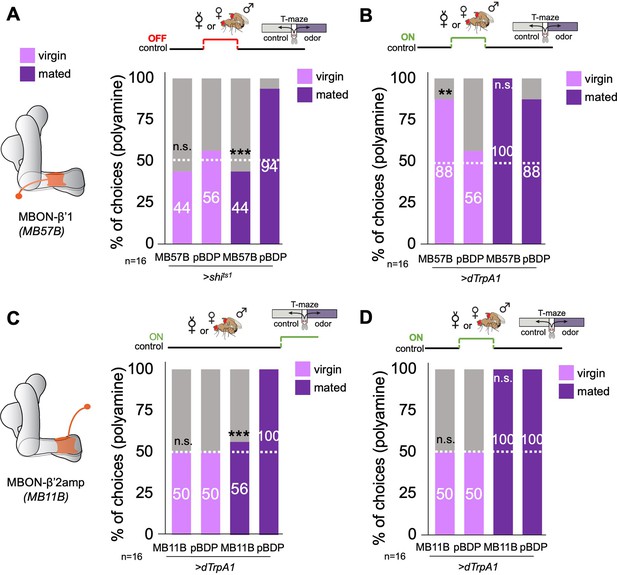
β’1 mushroom body output at mating regulates polyamine preference.
(A) Inhibition of MBON-β’1 output (MB57B-Gal4;UAS-shits1) during mating significantly reduced mated female preference for putrescine at the time of test. Single female preference for putrescine in T-maze assay. 50% represents chance. (B) Activation MBON-β’1 output (MB57B-Gal4;UAS-dTrpA1) instead of mating significantly increased virgin female preference for putrescine at the time of test. (C) Activation of MBON-β’2 output (MB11B-Gal4;UAS-dTrpA1) during test significantly reduced mated female preference for putrescine. (D) Activation MBON-β’2 output (MB11B-Gal4;UAS-dTrpA1) instead of or during mating did not change female preference for putrescine at the time of test. Single female preference for putrescine in T-maze assay. 50% represents chance. Fisher’s exact test; All p-values depict the comparison between the respective control (pBDP-Gal4;UAS-xx) and the text group (MBx-Gal4;UAS-xx). p-values: n.s.:>0.1, *:<0.1, **:<0.05, ***:<0.001. n=16 single female flies.
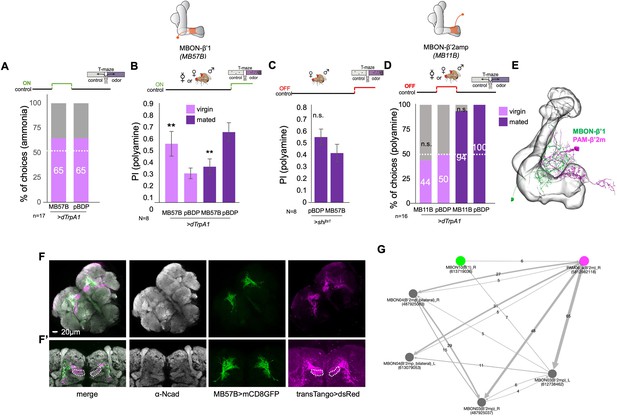
Mushroom body output neurons are involved in mating state-dependent choice behavior.
(A) Activation MBON-β’1 output (MB57B-Gal4;UAS-dTrpA1) instead of mating did not increase the virgin female’s preference for ammonia at the time of test. Single female preference for ammonia in T-maze assay. 50% represents chance. p-Values depict the comparison between the respective control (pBDP-Gal4;UAS-dTrpA1) and the test group (MB57B-Gal4;UAS-dTrpA1). (B) Activation MBON-β’1 output (MB57B-Gal4;UAS-dTrpA1) during testing significantly increased virgin female preference and decreased mated female preference for putrescine at the time of test, respectively. T-maze group assay. (C) Inhibition MBON-β’1 output (MB57B-Gal4;UAS-shits1) during testing did not change mated female behavior. T-maze group assay. Bars depict preference index (PI) ± SEM. Student-s t-test; p-values: n.s.:>0.05, *:<0.05, **:<0.01. (D) Activation MBON-β’2 output (MB11B-Gal4;UAS-dTrpA1) instead of or during mating did not change female preference for putrescine at the time of test. Single female preference for putrescine in T-maze assay. 50% represents chance. p-values depict the comparison between the respective control (pBDP-Gal4;UAS-shits1) and the text group (MB10B-Gal4;UAS-shits1). p-values: n.s.:>0.1, *:<0.1, **:<0.05, ***:<0.001. n=16 single female flies. (E) Reconstruction of neuronal morphology of MBON-β’1 (MBON10) and a PAM-β’2ma DAN in the MB. Trans-TANGO experiment reveals a possible direct synaptic connection between MBON-β‘1 and MBON-β’2. Grey, anti-Ncad stains neuropil, green, anti-GFP shows expression in MBON-β’1, magenta, anti-dsred stains expression of trans-TANGO mediated expression of dsRed in synaptically connected MBON-β’2. (F’) Higher magnification of images shown in (F). (G) MBON-β’1 form direct synaptic connections with PAM- β’2 DANs.
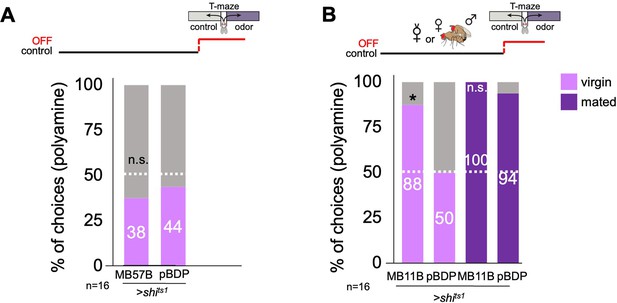
Mushroom body output neuron activity influences choice.
(A) Neuronal output was inhibited during preference test. Inhibition of MBON-β’1 output (MB57B-Gal4;UAS-shits1) at the time of test had no influence on virgin female preference for putrescine. (B) Inhibition of MBON-β’2 output (MB11B-Gal4;UAS-shits1) during testing significantly increased virgin female preference for putrescine at the time of test. Single female preference for putrescine in T-maze assay. 50% represents chance. Fisher’s exact test; All p-values depict the comparison between the respective control (pBDP-Gal4;UAS-xx) and the text group (MBx-Gal4;UAS-xx). p-values: n.s.:>0.1, *:<0.1, **:<0.05, ***:<0.001. n=16 single female flies.
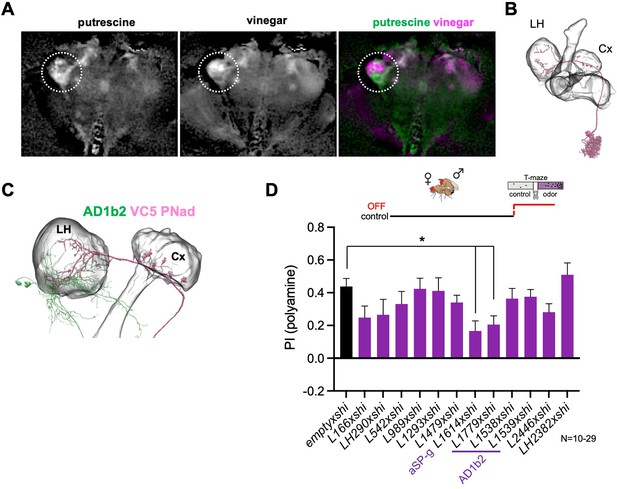
Lateral horn pathway contributes to the expression of polyamine attraction.
(A) In vivo whole brain lightfield calcium imaging upon stimulation with putrescine or vinegar (nsyb-Gal4; UAS-GCaMP6m). Putrescine and vinegar activate different areas in the lateral horn (LH, dotted line). (B) Electron microscopy (EM)-based reconstruction showing projection neurons (PN) innervating the VC5 glomerulus. (C) VC5 PNad (pink) innervates the LH and the mushroom body (MB). (D) T-maze behavioral screen at restrictive temperature at test (30 °C) of candidate LHONs in mated females reveals that two LHON types are required for full attraction to putrescine. ‘empty x shi’ represents the control group and stands for pBDPGAL4;UAS-shits1, the Gal4 vector used to generate all MB-Gal4 lines without an expression driving enhancer. One-way Anova, Bonferroni corrected; p-values: *:<0.05.
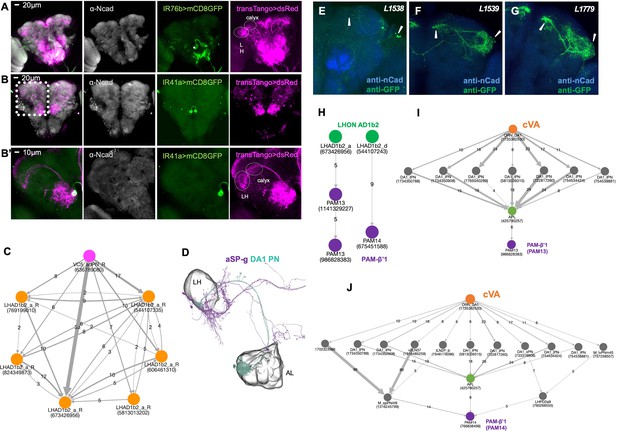
Projection neuron and lateral horn neuron connectivity.
(A) Trans-TANGO experiment reveals projection neurons (PNs) connecting the VC5 glomerulus to mushroom body (calyx) and lateral horn (LH). Grey, anti-Ncad stains neuropil, green, anti-GFP shows expression in IR76b neurons (IR76b>mCD8 GFP), magenta, anti-dsred stains expression of trans-TANGO mediated expression of dsRed in synaptically connected neurons including PNs. (B) Trans-TANGO experiment reveals projection neurons (PNs) connecting the VC5 glomerulus to mushroom body (calyx) and lateral horn (LH). Grey, anti-Ncad stains neuropil, green, anti-GFP shows expression in IR76b neurons (IR41a>mCD8 GFP), magenta, anti-dsred stains expression of trans-TANGO mediated expression of dsRed in synaptically connected neurons including PNs. (B’) Higher magnification of images shown in (B). (C) NeuPrint-generated connectome of VC5 adPN and its synaptic connections to the LHON AD1b2. (D) EM-Reconstruction. LHON aSP-g receives input from the DA1 PN. Note that the DA glomerulus is responds to the pheromone cVA. (E) mCD8-GFP expression driven by Split-Gal4 lines L1538. (F) mCD8-GFP expression driven by Split-Gal4 lines L1539. (G) mCD8-GFP expression driven by Split-Gal4 lines L1779. (H) NeuPrint-generated connectome between VC5/polyamine sensitive ORNs and PAM-β’1. (I) NeuPrint-generated connectome between VC5/polyamine sensitive ORNs and PAM-β’1.
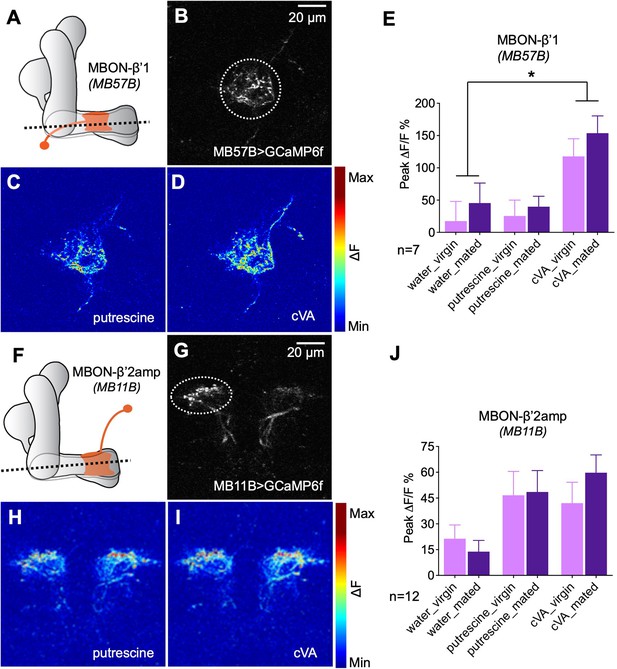
Odor responses of MBONs before and after mating.
(A) Scheme depicting imaging plane through MBON-β’1. (B) GCaMP6f was expressed under the control of MB57B-Gal4 in β’1 MBONs. Dashed circle indicates the region of interest (ROI) used for quantification of the signal. (C) Representative image showing response of MBON-β’1 to putrescine. (D) Representative image showing the response of MBON-β’1 to cVA (11-cis-Vaccenyl Acetate). (E) Peak ∆F/F GCaMP signals in response to water, putrescine or cVA. Responses of virgins and mated females are shown. (F) Scheme depicting imaging plane through MBON-β’2. (G) GCaMP6f was expressed under the control of MB11B-Gal4 in β’2 MBONs. Dashed circle indicates the region of interest (ROI) used for quantification of the signal. (H) Representative image showing response of MBON-β’2 to putrescine. (I) Representative image showing the response of MBON-β’2 to cVA (cis-Vaccenyl Acetate). (J) Peak ∆F/F GCaMP signals in response to water, putrescine or cVA. Responses of virgins and mated females are shown. ANOVA, p-values: n.s.:>0.05, *:<0.05, **:<0.01, ***:<0.001.
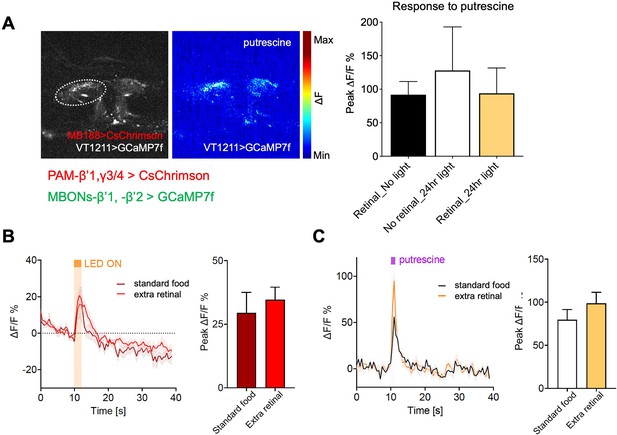
Optogenetic manipulation paired with calcium imaging of mushroom body output neuron.
(A) Left panel shows the expression of GCaMP7s under the control of VT1211 in β’1/2 MBONs. The same flies express CsChrimson under the control of MB188B (PAM-β’1,γ3/4). Middle panel shows a representative image of a response to putrescine odor. Right graph shows the peak ∆F/F GCaMP responses in β’1 and 2 MBONs in three different groups of flies of the same genotype (VT1211-GCaMP7s;MB188B-CsChrimson). Flies were fed with retinal but not exposed to light (black bar), exposed to red light (white bar) or fed with retinal and exposed to red light (test group, yellow bar). No significant difference was found between the groups. n=8–9 (B) Optogenetic stimulation of PAM-β’1,γ3/4 induced a change in calcium levels in the β’1 MBON. The response in flies (VT1211-GCaMP7s;MB188B-CsChrimson) fed with retinal-supplement food and flies of the same genotype fed with standard food is shown. Retinal is necessary to activate Cs-Chrimson. No significant difference was found between groups. Note that retinal is present in low amounts in standard fly food. Therefore, it is possible the PAM-β’1,γ3/4 neurons activate the connected MBONs. (C) The MBON response to putrescine (100 mM) of the flies in (B) is shown. Retinal-supplemented food- and standard fly food-fed flies responded similarly to putrescine odor. n=6 flies fed with retinal, and 6 flies fed with solvent. Bars depict response in % ± SEM. Student t-test; No significant difference was found between groups.
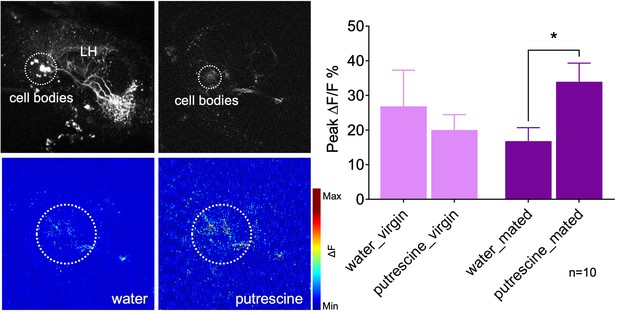
Mating modulates the putrescine response of lateral horn neuron AD1b2.
Upper left panels: High-resolution two-phtoton image of expression of Gal4-line LH1779 (LH1779-Gal4; UAS-GCaMP7s) in the region of the lateral horn (LH). Lower left panels: Representative images showing response of the neurons labeled by LH1779 to water control and to putrescine. Right panels: Peak ∆F/F% GCaMP signals in response to water or putrescine in virgins or mated females. Dashed circles indicate the region of interest (ROI) used for quantification of the signal. ANOVA, p-values: n.s.:>0.05, *:<0.05.
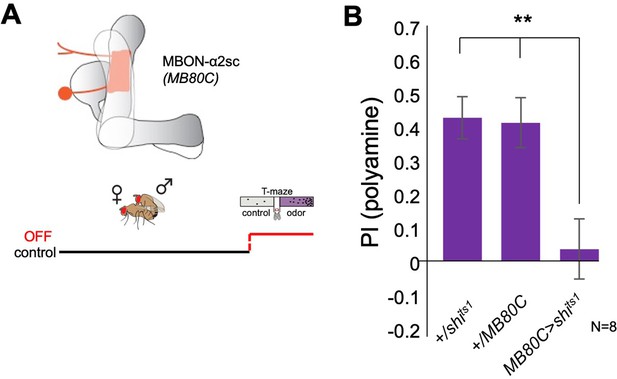
Mushroom body output to lateral horn is involved in polyamine choice behavior.
(A) Top, scheme of MBON-α2sc and expression of line MB80C. bottom, scheme showing experimental paradigm. Neuronal output was blocked at the time of preference test. (B) Inhibition of MBON-α2sc output (MB80C-Gal4;UAS-shits1) at the time of test significantly reduced mated female preference for putrescine. T-maze group assay. Bars depict preference index (PI) ± SEM. Student’s t-test; **<0.01.
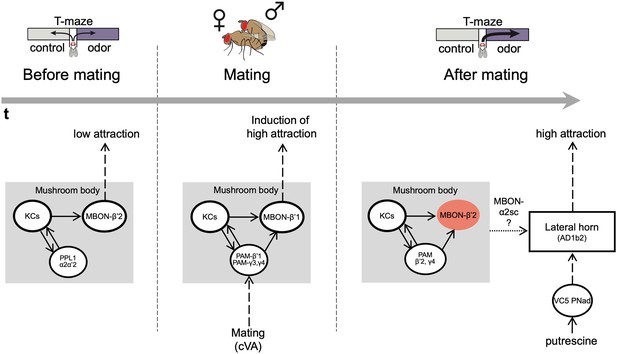
The mushroom body regulates odor preference behavior of females before and after mating.
Working model showing that in virgin females (before mating), the activity of PPL1 dopaminergic neurons and MBONβ’2 suppress the expression of polyamine odor preference. During mating, β’1,γ3/4 PAM dopaminergic neurons and β’1-MBONs convey the experience of mating, possibly through the detection of the pheromone cVA, to the mushroom body. This mechanism induces a long-lasting change in polyamine preference in mating females. Moreover, mating changes the polyamine odor response of the AD1b2 LHONs. After mating, mated females express their higher attraction to polyamine odor through a circuit involving the activity of PAM-β’2,γ4 dopaminergic neurons and the suppression of MBON-β’2 output. In addition, output of the lateral horn through at least two different types of output neurons (AD1b2 and aSP-g) is required for the expression of high polyamine preference. It is conceivable that the lateral horn and the mushroom body circuits are connected through specific output neurons such as MBON-α2sc.
Tables
Reagent type (species) or resource | Designation | Source or reference | Identifiers | Additional information |
---|---|---|---|---|
Antibody | Anti-Mouse Alexa488, goat polyclonal | Molecular Probes | AB_ 150113 | (1:200) |
Antibody | Anti-Mouse Alexa633, goat polyclonal | Molecular Probes | AB_141431 | (1:200) |
Antibody | Anti-Rabbit Alexa568, goat polyclonal | Molecular Probes | AB_141416 | (1:200) |
Antibody | Anti-Rabbit Alexa633, goat polyclonal | Molecular Probes | AB_2535731 | (1:200) |
Antibody | Anti-Rat Alexa568, goat polyclonal | Molecular Probes | AB_141874 | (1:200) |
Antibody | Anti-ChAT, mouse monoclonal | Yasuyama et al., 1995 | N/A | (1:100) |
Antibody | Anti-OA, mouse monoclonal | Jena Bioscience | AB_2315000 | (1:200) |
Antibody | Anti-dsRed, rabbit polyclonal | Clontech | AB_10013483 | (1:400) |
Antibody | Anti-GFP [3H9], rat monoclonal | Chromotek | AB_10773374 | (1:200) |
Antibody | Anti-Ncadherin, rat monoclonal | DSHB | AB_528121 | (1:200) |
other | All original data is available online. | Mendeley Data | http://dx.doi.org/10.17632/5rz28jr8gc.1 | Source data |
genetic reagent (D. melanogaster) | D.mel/Canton-S | Bloomington DSC | Flybase: FBst0064349 | |
genetic reagent (D. melanogaster) | D.mel/GMR95A10-LexA | Bloomington DSC | FlyBase: FBst0061633 | |
genetic reagent (D. melanogaster) | D.mel/LexAop2-mCD8-GFP | Bloomington DSC | FlyBase: FBst0056182 | |
genetic reagent (D. melanogaster) | D.mel/LexAop2-GCaMP7f | Bloomington DSC | FlyBase: FBti0202377 | |
genetic reagent (D. melanogaster) | D.mel/MB10B | Janelia RC | Flybase: FBst0068293 | Aso et al., 2014a |
genetic reagent (D. melanogaster) | D.mel/MB11B | Janelia RC | Flybase: FBst0068294 | Aso et al., 2014a |
genetic reagent (D. melanogaster) | D.mel/MB438B | Janelia RC | Flybase: FBst0068326 | Aso et al., 2014a |
genetic reagent (D. melanogaster) | D.mel/MB57B | Janelia RC | Flybase: FBst0068277 | Aso et al., 2014a |
genetic reagent (D. melanogaster) | D.mel/MB60B | Janelia RC | Flybase: FBst0068279 | Aso et al., 2014a |
genetic reagent (D. melanogaster) | D.mel/MB58B | Janelia RC | FlyBase: FBst0068278 | Aso et al., 2014a |
genetic reagent (D. melanogaster) | D.mel/MB80C | Janelia RC | FlyBase: FBst0068285 | Aso et al., 2014a |
genetic reagent (D. melanogaster) | D.mel/MB188B | Janelia RC | gift of Yoshinori Aso | Aso et al., 2014a |
genetic reagent (D. melanogaster) | D.mel/MB42B | Janelia RC | FlyBase: FBst0068303 | Aso et al., 2014a |
genetic reagent (D. melanogaster) | D.mel/MB109B | Janelia RC | gift of Yoshinori Aso | Aso et al., 2014a |
genetic reagent (D. melanogaster) | D.mel/MB316B | Janelia RC | gift of Yoshinori Aso | Aso et al., 2014a |
genetic reagent (D. melanogaster) | D.mel/L166 | Janelia RC | gift of Greg Jefferis | Dolan et al., 2019 |
genetic reagent (D. melanogaster) | D.mel/L290 | Janelia RC | gift of Greg Jefferis | Dolan et al., 2019 |
genetic reagent (D. melanogaster) | D.mel/L542 | Janelia RC | gift of Greg Jefferis | Dolan et al., 2019 |
genetic reagent (D. melanogaster) | D.mel/L989 | Janelia RC | gift of Greg Jefferis | Dolan et al., 2019 |
genetic reagent (D. melanogaster) | D.mel/L1293 | Janelia RC | gift of Greg Jefferis | Dolan et al., 2019 |
genetic reagent (D. melanogaster) | D.mel/L1479 | Janelia RC | gift of Greg Jefferis | Dolan et al., 2019 |
genetic reagent (D. melanogaster) | D.mel/L1614 | Janelia RC | gift of Greg Jefferis | Dolan et al., 2019 |
genetic reagent (D. melanogaster) | D.mel/L1538 | Janelia RC | gift of Greg Jefferis | Dolan et al., 2019 |
genetic reagent (D. melanogaster) | D.mel/L1539 | Janelia RC | gift of Greg Jefferis | Dolan et al., 2019 |
genetic reagent (D. melanogaster) | D.mel/L1779 | Janelia RC | gift of Greg Jefferis | Dolan et al., 2019 |
genetic reagent (D. melanogaster) | D.mel/L2446 | Janelia RC | gift of Greg Jefferis | Dolan et al., 2019 |
genetic reagent (D. melanogaster) | D.mel/L2382 | Janelia RC | gift of Greg Jefferis | Dolan et al., 2019 |
genetic reagent (D. melanogaster) | D.mel/pBDP-Gal4U (‚empty-Gal4’) | Bloomington DSC | Flybase: FBst0068384 | |
genetic reagent (D. melanogaster) | D.mel/UAS-CsChrimson | Bloomington DSC | FlyBase: FBst0055134 | |
genetic reagent (D. melanogaster) | D.mel/UAS-DenMark | Bloomington DSC | FlyBase: FBst0033062 | |
genetic reagent (D. melanogaster) | D.mel/UAS-GCaMP6f | Bloomington DSC | FlyBase: FBst0042747 | |
genetic reagent (D. melanogaster) | D.mel/UAS-GCaMP7s | Bloomington DSC | FlyBase: FBst0079032 | |
genetic reagent (D. melanogaster) | D.mel/UAS-mCD8-GFP | Bloomington DSC | FlyBase: FBst0030001 | |
genetic reagent (D. melanogaster) | D.mel/UAS-Shibirets1 | Bloomington DSC | FlyBase: FBst0044222 | |
genetic reagent (D. melanogaster) | D.mel/UAS-syt-GFP | Bloomington DSC | FlyBase: FBst0006926 | |
genetic reagent (D. melanogaster) | D.mel/w1118 | Bloomington DSC | Flybase: FBst0003605 | |
genetic reagent (D. melanogaster) | D.mel/prd-gal4 | gift of Anne von Philipsborn | ||
genetic reagent (D. melanogaster) | D.mel/UAS-Bip RNAi | gift of Anne von Philipsborn | ||
genetic reagent (D. melanogaster) | D.mel/tud[1] bw[1] sp[1]/CyO, l(2)DTS513[1] | Bloomington DSC | stock # 1786 | |
genetic reagent (D. melanogaster) | D.mel/ovoD | Bloomington DSC | stock # 38444 | |
Software/ algorithm | Matplotlib 1.4.2 | Hunter, 2007 | https://matplotlib.org/ | |
Software/ algorithm | Numpy 1.8 | Harris et al., 2020 | https://numpy.org/ | |
Software/ algorithm | Prism 6 and 7 | GraphPad | https://www.graphpad.com/scientific-software/prism/ | |
Software/ algorithm | Python 2.7 | Van and Fred, 1995 | https://www.python.org/ | |
Software/ algorithm | Pyvttbl 0.5.2.2 | Galbally et al., 2011 | https://github.com/rogerlew/pyvttbl | |
Software/ algorithm | FIJI | ImageJ | https://imagej.net/software/fiji/ | |
Software/ algorithm | Scipy.stats 0.14 | Jones et al., 2001 | https://scipy.org/ | |
Software/ algorithm | Igor Pro 6.37 | Wave Metrics | https://www.wavemetrics.com/ | |
Software/ algorithm | NeuroMatic 3.0 | Rothman and Silver, 2018 | http://neuromatic.thinkrandom.com/ | |
Software/ algorithm | LAS AF E6000 and LAS X | Leica Microsystems | https://www.leica-microsystems.com/ | |
Software/ algorithm | FV10-ASW | Olympus | https://olympus-lifescience.com/ | |
Software/algorithm | ScanImage | Vidrio Technologies | https://vidriotechnologies.com |
100 mMPutrescine | not activated | activated |
---|---|---|
trp MB025B 25C, 100 mM Putrescine | trp MB025B 32C, 100 mM Putrescine | |
percent choice | 90 | 94.4 |
n(virgins) = | 21 | 18 |
10 mM Putrescine | ||
trp MB025B 18C, 10 mM Putrescine | trp MB025B 32C, 10 mM Putrescine | |
percent choice | 58 | 71 |
n(virgins) = | 36 | 35 |