Vision-related convergent gene losses reveal SERPINE3’s unknown role in the eye
Figures
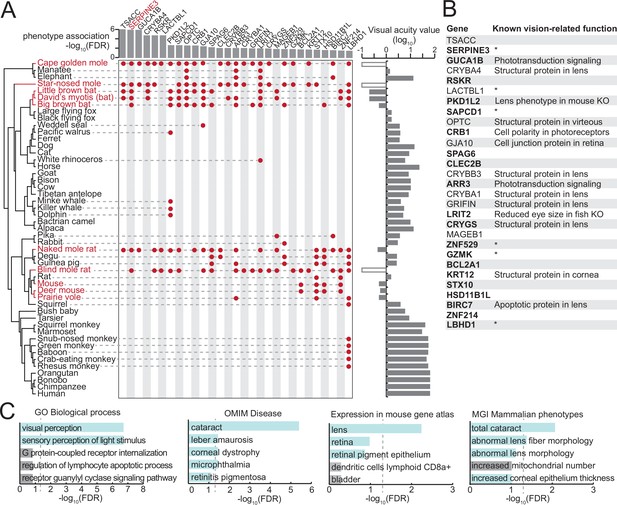
A comparative genomics screen uncovered known and novel vision-related genes.
(A) Phylogeny of the species included in our screen (left). Visual acuity values on a log10 scale are shown on the right as a bar chart; white boxes indicate the three subterranean mammals that lack acuity measurements but are functionally blind. Low-acuity species (red font) are defined here as species with visual acuity <1 (log10(va) <0). At a false discovery rate (FDR) threshold of 0.05, our screen retrieved 29 genes, which are preferentially lost in low-acuity species. Gene losses in individual species are denoted by red dots. The FDR value for the gene loss – phenotype association is shown at the top as a bar chart. (B) List of 29 genes, together with known vision-related functions. Asterisk marks genes that have no known vision-related function but were mentioned in large-scale gene expression data sets of ocular tissues. Genes in bold are expressed in human eyes according to the eyeIntegration database (Bryan et al., 2018) (see Methods). KO - knockout. (C) Functional enrichments of the 29 genes reveals vision-related functions (Gene Ontology, GO of biological processes), associations with human eye disorders (OMIM), expression in ocular tissues in the mouse gene atlas (Kuleshov et al., 2016) and eye phenotypes in mouse gene KOs (MGI Mammalian Phenotype level 4) among the top five most significant terms. Vision-related terms are shown as blue bars. The dashed line indicates statistical significance in a one-sided Fisher’s exact test after correcting for multiple testing using the Benjamini-Hochberg procedure (FDR 0.05).
-
Figure 1—source data 1
Genome assemblies used in this study and visual acuity values of mammalian species.
- https://cdn.elifesciences.org/articles/77999/elife-77999-fig1-data1-v2.xls
-
Figure 1—source data 2
List of candidate genes of the forward genomics screen based on visual acuity <1 including a short description.
- https://cdn.elifesciences.org/articles/77999/elife-77999-fig1-data2-v2.xls
-
Figure 1—source data 3
Enrichment of candidate genes in different processes/functions.
- https://cdn.elifesciences.org/articles/77999/elife-77999-fig1-data3-v2.xls
-
Figure 1—source data 4
A control forward genomics screen does not retrieve vision-related genes.
- https://cdn.elifesciences.org/articles/77999/elife-77999-fig1-data4-v2.xls
-
Figure 1—source data 5
List of candidate genes of the forward genomics screen based on a visual acuity threshold <1.5.
- https://cdn.elifesciences.org/articles/77999/elife-77999-fig1-data5-v2.xls
-
Figure 1—source data 6
List of candidate genes of the forward genomics screen based on visual acuity threshold <0.5.
- https://cdn.elifesciences.org/articles/77999/elife-77999-fig1-data6-v2.xls
-
Figure 1—source data 7
List of candidate genes of the forward genomics screen based on the molecular loss signature of known vision genes.
- https://cdn.elifesciences.org/articles/77999/elife-77999-fig1-data7-v2.xls
-
Figure 1—source data 8
Reading frame %intact values for all investigated genes and species.
- https://cdn.elifesciences.org/articles/77999/elife-77999-fig1-data8-v2.xls
-
Figure 1—source data 9
Enrichment of candidate genes in different stages of the filtering process.
- https://cdn.elifesciences.org/articles/77999/elife-77999-fig1-data9-v2.xls
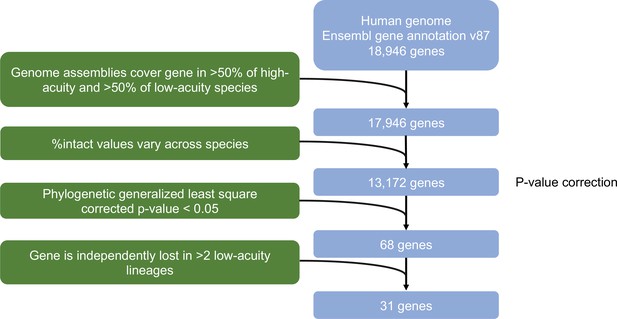
Flow diagram of filtering steps applied in the Forward genomics approach.
The filtering procedure results in a list of 31 candidate genes. Two of the 31 genes are no longer contained in the latest Ensembl annotation version (v106) and were therefore dropped, resulting in 29 genes potentially associated with an eye-related function.
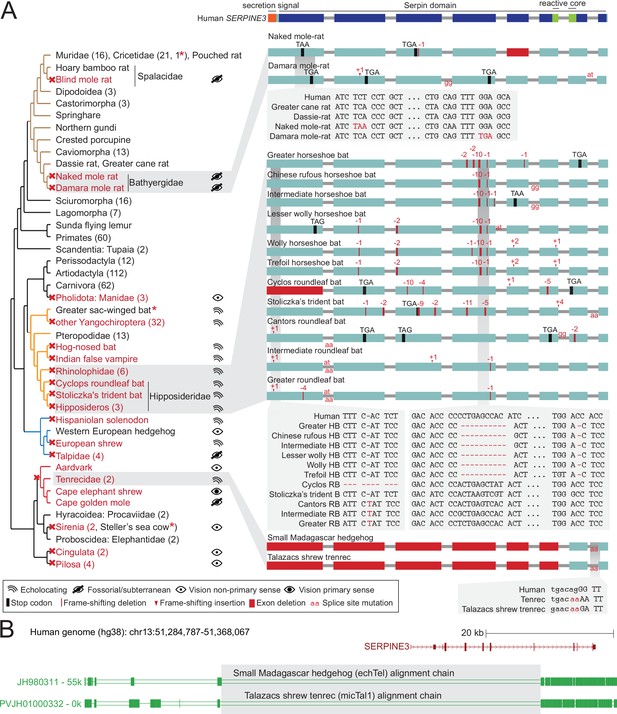
SERPINE3 gene loss pattern across 430 mammalian species.
(A) Left: Phylogeny of mammalian species investigated for the loss of SERPINE3 with mapped gene loss events indicated as red crosses. Branches of major clades are colored (Rodentia – brown, Chiroptera – orange, Eulipotyphla – blue, Afroinsectiphilia - red). The number of species investigated per clade is specified in parenthesis. For all loss lineages (red font), visual capability (classified as echolocating, fossorial/subterranean, vision as non-primary and primary sense) is displayed as pictograms at the right. Asterisk marks indicate species, where SERPINE3 evolved under relaxed selection but did not accumulate not more than one inactivating mutation. Right: The Serpin protein domain (Pfam) spans all eight protein-coding exons (boxes) of the intact human SERPINE3 gene (top). The secretion signal and the reactive core region are conserved in species with an intact SERPINE3 (Figure 2—figure supplements 9 and 10). Gene-inactivating mutations are illustrated for three clades, with stop codon mutations shown in black, frame-shifting insertions and deletions shown in red and mutated splice site dinucleotides shown between exons in red. Deleted exons are shown as red boxes. Insets show codon alignments with inactivating mutations in red font. RB - roundleaf bat, HB - horseshoe bat. (B) An ancestral deletion removed large parts of SERPINE3 in the tenrec lineage. UCSC genome browser (Lee et al., 2022) view of the human hg38 assembly showing the SERPINE3 locus and whole genome alignments between human and two tenrec species, visualized as a chain of co-linear local alignments. In these chains, blocks represent aligning sequence and double lines represent sequence between the aligning blocks that do not align between human and tenrec. A large deletion removed the first five protein-coding exons of SERPINE3 in both species. Shared breakpoints (gray box) indicate that the deletion likely represents an ancestral event in Tenrecidae.
-
Figure 2—source data 1
SERPINE3 intactness in 447 mammalian assemblies.
- https://cdn.elifesciences.org/articles/77999/elife-77999-fig2-data1-v2.xls
-
Figure 2—source data 2
Verification of inactivating mutations in SERPINE3 with raw DNA sequencing reads.
- https://cdn.elifesciences.org/articles/77999/elife-77999-fig2-data2-v2.xls
-
Figure 2—source data 3
Expression of SERPINE3 remnants in public RNA-seq datasets.
- https://cdn.elifesciences.org/articles/77999/elife-77999-fig2-data3-v2.xls
-
Figure 2—source data 4
Analysis of relaxation or intensification of selection in SERPINE3 branches of interest.
- https://cdn.elifesciences.org/articles/77999/elife-77999-fig2-data4-v2.xls
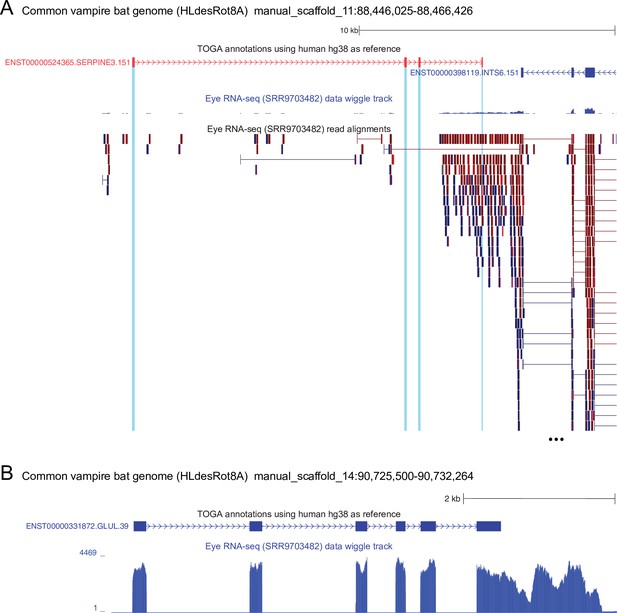
The remnants of SERPINE3 are not expressed in the common vampire bat.
(A) UCSC genome browser screenshot of the common vampire bat (Desmodus rotundus) genome (Blumer et al., 2022) showing the four remaining exons of SERPINE3 (red: TOGA annotation, the other exons are deleted in this bat) and three coding exons of the neighboring INTS6 gene. Eye RNA-seq from D. rotundus (Sadier et al., 2018), shown as a wiggle track and read alignments, show that three SERPINE3 exons (blue highlight) are not expressed. The fourth SERPINE3 exon overlaps the 3' UTR of the neighboring INTS6 gene, which is located on the other strand. This explains why reads cover this SERPINE3 exon and its flanks, but there is not a single read that supports splicing of this exon to other SERPINE3 exons. In contrast, INTS6 exons are robustly expressed and show spliced alignments. (B) For comparison, the conserved GLUL gene, which is a key enzyme in glutamate detoxification, is highly expressed in eyes of the common vampire bat.
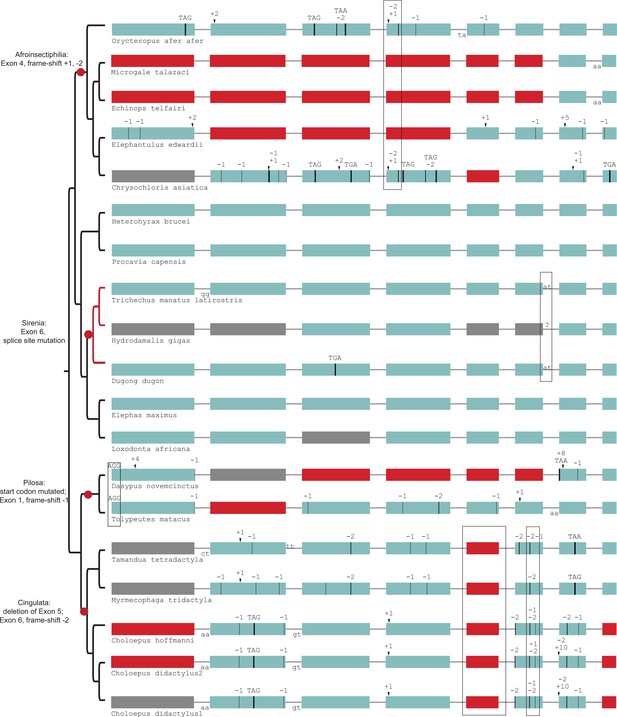
Gene-inactivating mutations support four independent losses of SERPINE3 in Afrotheria and Xenarthra (red dots).
Those shared mutations are marked by boxes, which indicate the loss of SERPINE3 in the common ancestor of related species according to parsimony. For tenrecs (Microgale talazaci, Echinops telfairi) and elephant shrew (Elephantulus edwardii), the putative region of exon 4 was likely deleted after the shared frame-shifting mutations. SERPINE3 in sirenia (branches marked in red) evolve under relaxed selection. Coloring and legend as in Figure 2. Gray exons denote missing information.
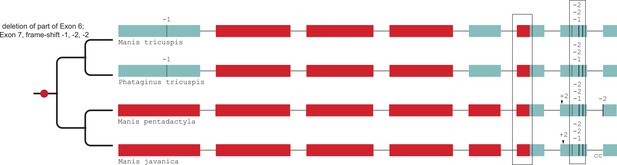
Gene-inactivating mutations support a single loss of SERPINE3 in Pholidota.
A partial deletion of coding exon 6 and three frame-shifting deletions in exon 7 are shared among all four species, indicating a single loss of SERPINE3 in the Pholidota lineage. Coloring and legend as in Figure 2.
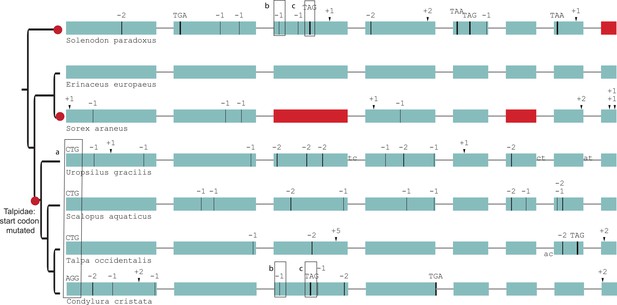
Gene-inactivating mutations support three independent losses of SERPINE3 in Eulipotyphla (red dots).
The start codon is not intact in the four moles (a). Star-nosed mole (Condylura cristata) and Hispaniolan solenodon (Solenodon paradoxus) share two inactivating mutations in exon 3, although they are phylogenetically distant (b, c). Coloring and legend as in Figure 2.
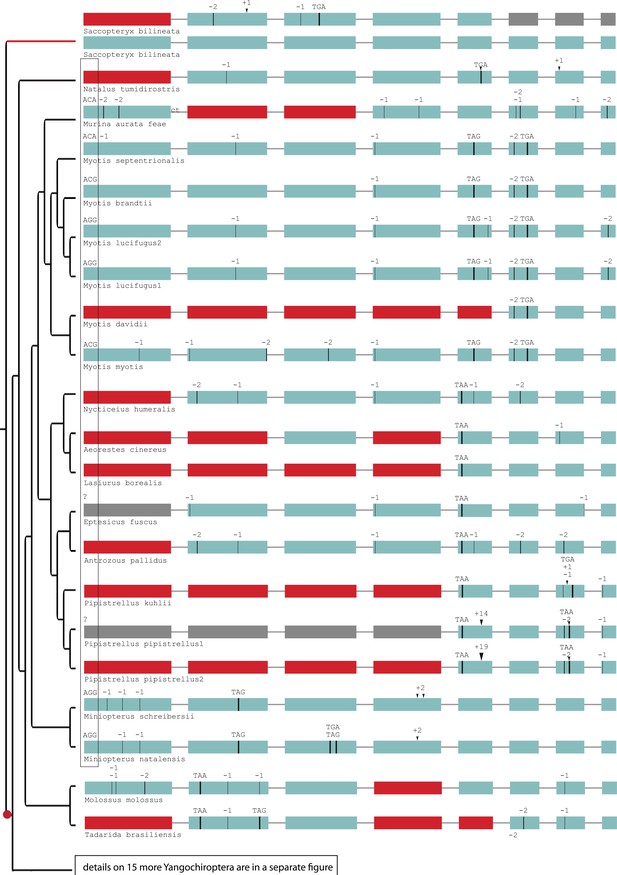
Gene-inactivating mutations support one loss of SERPINE3 in Yangochiroptera.
The ancestral start codon is mutated in most Yangochiroptera (box). The most parsimonious explanation is a shared start codon mutation (red dot) in the ancestral lineage after split from the sac-winged bat (Saccopteryx bilineata). A back mutation to the regular start codon ATG likely occurred in Molossus molossus and Artibeus jamaicensis. Please see Figure 2—figure supplement 6 for gene-inactivating mutations of 15 more Yangochiroptera assemblies. The sac-winged bat has an intact copy of the gene that evolves under relaxed selection (red branch) in addition to a second copy of SERPINE3, which was independently inactivated. Coloring and legend as in Figure 2. Gray exons denote missing information.
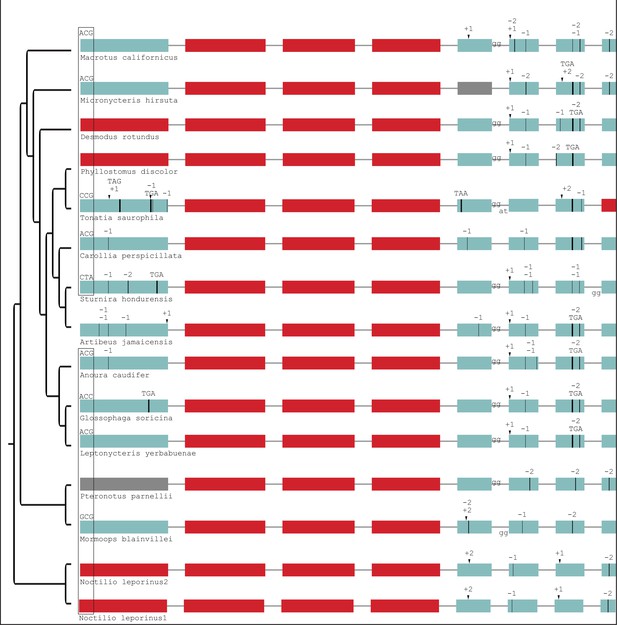
Gene-inactivating mutations in additional Yangochiroptera.
This is an extension of Figure 2—figure supplement 5. The start codon mutation shown is shared with most other Yangochiroptera (Figure 2—figure supplement 5). Coloring and legend as in Figure 2. Gray exons denote missing information.
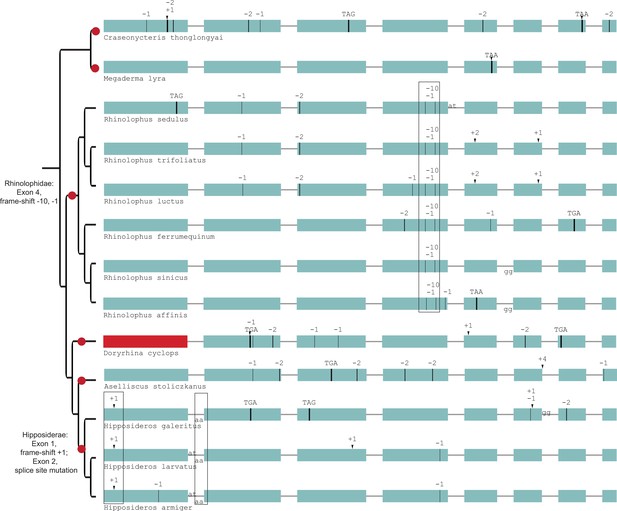
Gene-inactivating mutations support six independent losses of SERPINE3 in Yinpterochiroptera.
Six independent inactivation events (red dots) occurred in SERINE3’s coding sequence in Yinpterochiroptera. All Rhinolophidae share frame-shifting mutations in exon 4 (boxed), while Hipposideridea share a frame-shifting insertion in exon 1 and a splice site mutation at exon 2 (boxed). Coloring and legend as in Figure 2.
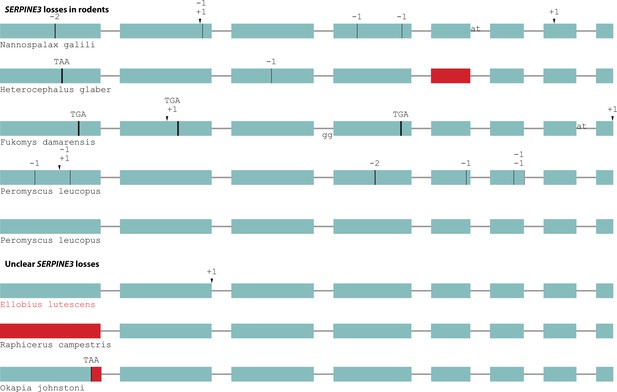
Independent gene-inactivating mutations in other mammals.
A test for shifts in selective pressure revealed that SERPINE3 evolves under relaxed selection in the subterranean mole vole (Ellobius lutescens, marked in red), but not in the other cases of gene-inactivating mutations with unclear consequences (steenbok, okapi). A species-specific duplication with inactivation of one SERPINE3 copy occurred in white-footed mouse (Peromyscus leucopus). Coloring and legend as in Figure 2.
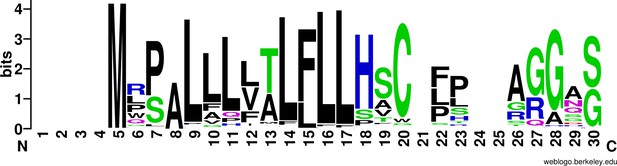
Conservation of the signal peptide in mammalian SERPINE3.
The putative N-terminal signal peptide contains many hydrophobic residues in intact mammalian SERPINE3 and is predicted to guide secretion into extracellular space. We show the first 30 alignment columns. The sequence logo was generated with WebLogo (Crooks et al., 2004).
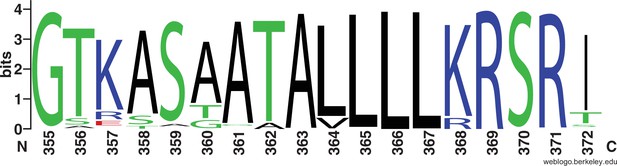
The hinge region and reactive core loop are conserved in intact mammalian SERPINE3.
The numbers refer to human SERPINE3, where R369 likely is the scissile bond (P1)within the reactive core loop positions 366–372, (P4-P4’). The hinge region is located at positions 355–361. The logo was generated with WebLogo (Crooks et al., 2004).
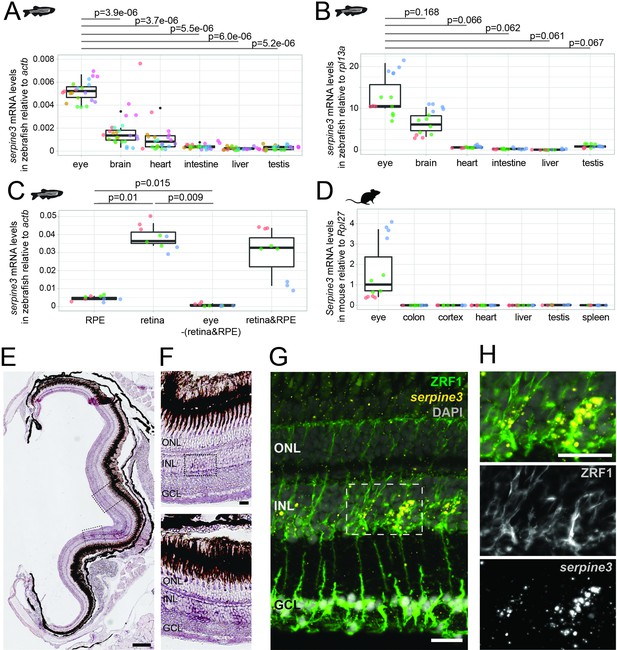
Serpine3 is expressed in zebrafish and mouse eyes.
(A,B) Expression of zebrafish serpine3 mRNA in relation to the normalization genes actb (A) and rpl13a (B) measured with RT-qPCR. Expression levels are consistently the highest in eye. A two-sided unequal variances t-test was used. Boxplots display first quartile, median and third quartile with whiskers extending to the maximum and minimum value. Measurements of technical replicates (n=3–6) are encoded in the same color, different colors represent different biological replicates (n=3–8). Relative rates are displayed as zero, if expression was below the detection limit. (C) Serpine3 mRNA expression in different tissues of the zebrafish eye in relation to the reference gene actb measured with RT-qPCR for three biological replicates. Serpine3 is specifically expressed in the retina but not in other tissues of the eye. The expression level is significantly higher in retina without RPE compared to RPE only (two-sided unequal variances t-test). Although expression in RPE only is low, it is significantly higher than expression in eye tissue after removing retina and RPE (two-sided unequal variances t-test). (D) Serpine3 mRNA expression in mouse in relation to the reference gene Rpl27 measured with RT-qPCR for three biological replicates. Serpine3 is specifically expressed in the eye but not in colon, cortex, heart, liver, spleen and testis. (E–H) Serpine3 mRNA expression pattern in zebrafish retina. (E–F) Chromogenic in situ hybridization (ISH) shows localized expression of serpine3 (purple) in the retina, specifically in the inner nuclear layer (inlet). (G–H) Fluorescence in situ hybridization shows that serpine3 mRNA expression (yellow) is localized to cell bodies of Müller glia cells. Filaments of Müller glia cells are marked by the glial fibrillary acidic protein (ZRF1 antibody, green). Specificity of the serpine3 ISH probe is shown by absence of the signal in homozygous serpine3cbg17 knockout fish (Figure 3—figure supplement 1). Serpine3 mRNA is not expressed in bipolar or amacrine cells (Figure 3—figure supplement 2). Nuclei are stained with DAPI (white). Scale bar is 200 µm in (E) and (G) and 20 µm in (F) and (H). INL – inner nuclear layer, ONL – outer nuclear layer, GCL – ganglion cell layer.
-
Figure 3—source data 1
qPCR analysis of a zebrafish organ series using the normalizer gene actb.
- https://cdn.elifesciences.org/articles/77999/elife-77999-fig3-data1-v2.xls
-
Figure 3—source data 2
qPCR analysis of a zebrafish organ series using the normalizer gene rpl13a.
- https://cdn.elifesciences.org/articles/77999/elife-77999-fig3-data2-v2.xls
-
Figure 3—source data 3
qPCR analysis of zebrafish eye subtissues.
- https://cdn.elifesciences.org/articles/77999/elife-77999-fig3-data3-v2.xls
-
Figure 3—source data 4
qPCR analysis of mouse organs.
- https://cdn.elifesciences.org/articles/77999/elife-77999-fig3-data4-v2.xls
-
Figure 3—source data 5
Data sets supporting expression of SERPINE3 in tissues of the eye in vertebrates other than mouse and zebrafish.
- https://cdn.elifesciences.org/articles/77999/elife-77999-fig3-data5-v2.xls
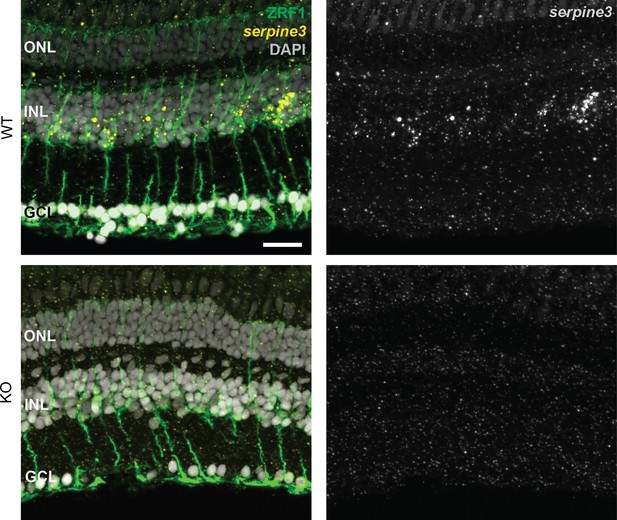
Serpine3 expression co-localizes with glial fibrillary acidic protein in the retina of wild type fish.
The fluorescence in situ signal for zebrafish serpine3 is specific for wild type (WT, yellow), where it is in proximity to staining of the ZRF1-antibody (green). This indicates expression of serpine3 by Müller glia cells. Serpine3 signal is not present in homozygous serpine3cbg17 siblings. In the overlay, nuclei (DAPI) are shown in white. ONL – outer nuclear layer, INL – inner nuclear layer, GCL – ganglion cell layer. Scale bar = 20 µm.
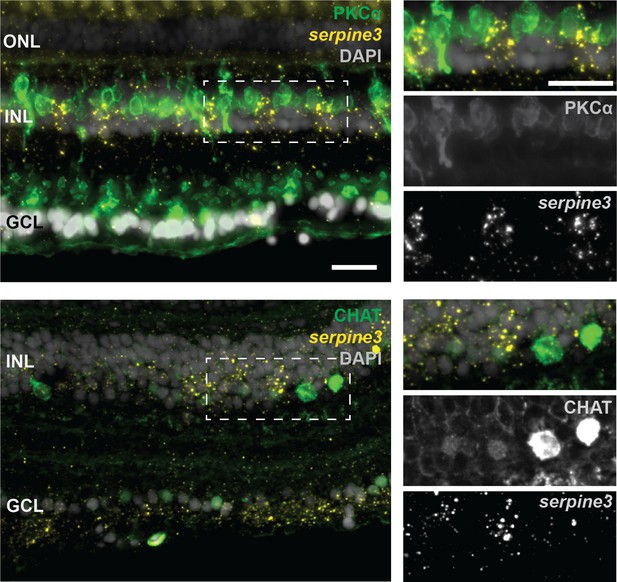
Serpine3 expression does not co-localize with markers for bipolar or amacrine cells in the zebrafish retina.
Fluorescence in situ hybridization of serpine3 mRNA (yellow) does not show co-localization with anti-protein kinase C alpha (PKCa encoded by prkca, green) or choline O-acetyltransferase a (CHAT, encoded by chata, green) proteins. In the overlay, nuclei (DAPI) are shown in white. ONL – outer nuclear layer, INL – inner nuclear layer, GCL – ganglion cell layer. Scale bar overview = 20 µm, details = 20 µm.
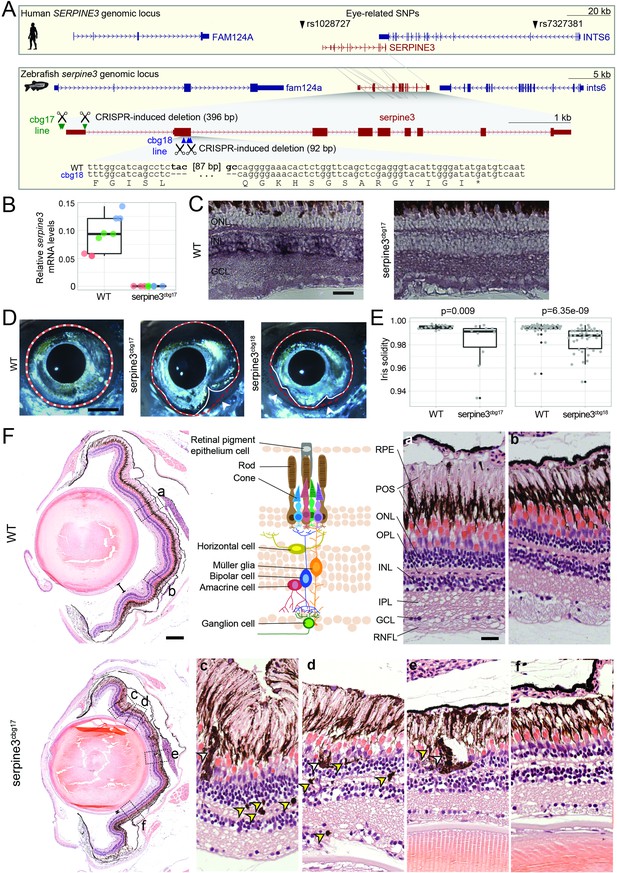
Serpine3 knockout in zebrafish causes defects in eye shape and retinal layering.
(A) UCSC genome browser visualization of the SERPINE3 genomic locus in human (hg38 assembly, top box) and zebrafish (danRer11 assembly, bottom box) shows that both species have a 1:1 ortholog with the same number of coding exons in a conserved gene order context. In the human locus, two single nucleotide polymorphisms (SNPs) are in linkage with SERPINE3 and associated with eye phenotypes. In zebrafish, we used CRISPR-Cas9 to generate two independent knockout (KO) lines. The position of guide RNAs is indicated as scissors. In the serpine3cbg17 line, we deleted the promoter and first exon. In the serpine3cbg18 line, we introduced a 92 bp frame-shifting deletion in exon 2 (coding exon 1) that results in three early stop codons in the original reading frame. (B) Relative expression of serpine3 mRNA in wild type (WT) zebrafish and serpine3cbg17 individuals quantified by RT-qPCR relative to the expression of rpl13a. Serpine3 mRNA expression is close to zero in serpine3cbg17 fish and significantly reduced in comparison to wild type fish (p=0.049, two-sided unequal variances t-test). Technical replicates of the qPCR are shown as individual data points; different colors represent different biological replicates. Boxplots display first quartile, median and third quartile with whiskers extending to the maximum and minimum of the three biological replicates. (C) In situ hybridization showing that serpine3 is expressed in the inner nuclear layer (INL) of WT zebrafish but not in the homozygous serpine3cbg17. Scale bar = 25 µm. (D) Serpine3 knockout leads to changes in eye shape in adult, homozygous knockout (KO) fish of serpine3cbg17 and serpine3cbg18 lines in comparison to their WT siblings (18 and 11 months, respectively). In WT, the eye shape almost perfectly corresponds to the concave shape of the iris (overlay of white and red dotted lines). In contrast, many KO individuals have alterations in eye shape, evident by notches (arrow heads) in the white line that follows the iris. Scale bar = 1 mm. (E) Iris solidity (ratio of eye shape/ concave eye shape) significantly differs between WT and KO siblings for both the serpine3cbg17 (16 vs 10 eyes) and the serpine3cbg18 (40 vs 40 eyes) line. A Wilcoxon Rank sum test was used. Boxplots display first quartile, median, and third quartile with whiskers extending to the maximum and minimum within 1.5 times interquartile range. Outliers are shown in black. Iris circularity, another quantification measure for the phenotype, is shown in Figure 4—figure supplement 3. (F) Hematoxylin/eosin histology staining of the eye of serpine3cbg17 fish (22 months) reveals histological differences in comparison to their WT siblings (dorsal top, ventral bottom). In comparison to WT, distance between lens and retina of serpine3cbg17 fish is reduced (distance bars). The WT retina (top) has a distinct lamination with clear separation of the single retinal layers (a, b) as shown in the schematic (RPE – retinal pigment epithelium layer, POS – photoreceptor outer segment, ONL – outer nuclear layer, OPL – outer plexiform layer, INL – inner nuclear layer, IPL – inner plexiform layer, GCL – ganglion cell layer, RNFL – retinal nerve fiber layer). Although all retinal layers are present in serpine3cbg17 fish, the layering appears distorted and the density of cells is reduced (c–f). Specifically, the RPE cells display an altered distribution and even local clusters (empty arrows), and displaced pigmented cells emerge in all retinal layers (yellow arrows). This was confirmed also for the cbg18 allele (Figure 4—figure supplement 5). Scale bar in the overviews = 200 µm, scale bar in the magnifications = 20 µm.
-
Figure 4—source data 1
qPCR analysis of zebrafish serpine3cbg17 homozygotes (KO) vs WT.
- https://cdn.elifesciences.org/articles/77999/elife-77999-fig4-data1-v2.xls
-
Figure 4—source data 2
Macroscopic eye phenotype in serpine3 knockout lines.
- https://cdn.elifesciences.org/articles/77999/elife-77999-fig4-data2-v2.xls
-
Figure 4—source data 3
Single-nucleotide polymorphism near SERPINE3 (13: 51, 341, 032–51, 362,101) are associated with human eye phenotypes.
- https://cdn.elifesciences.org/articles/77999/elife-77999-fig4-data3-v2.xls
-
Figure 4—source data 4
Raw gel images of genotyping of the serpine3cbg17 line with two primers (labeled image is shown in Figure 4 - figure supplement 2A).
- https://cdn.elifesciences.org/articles/77999/elife-77999-fig4-data4-v2.zip
-
Figure 4—source data 5
Raw gel images of genotyping of the serpine3cbg17 line with a mix of three primers (labeled image is shown in Figure 4 - figure supplement 2B).
- https://cdn.elifesciences.org/articles/77999/elife-77999-fig4-data5-v2.zip
-
Figure 4—source data 6
Raw gel images of genotyping of the serpine3cbg18 line with two primers (labeled image is shown in Figure 4 - figure supplement 2C).
- https://cdn.elifesciences.org/articles/77999/elife-77999-fig4-data6-v2.tif
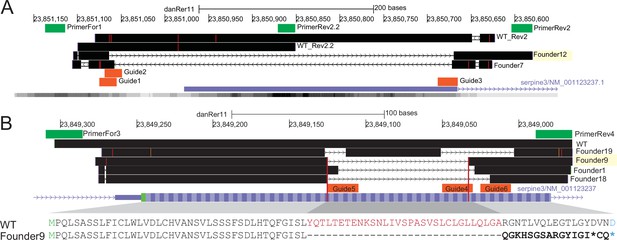
Knockout of serpine3cbg17 and serpine3cbg18 alleles in zebrafish by CRISPR-Cas9 is confirmed by sequencing results.
Sequenced reads are mapped against the danRer11 zebrafish genome assembly with Blat and are visualized in the UCSC genome browser (black) together with the serpine3 RefSeq annotation (blue). (A) PCRs with primers For1 and Rev2 or For1 and Rev2.2 (green) amplify the expected regions around the transcription start site on chromosome 9 in wild type (WT_Rev2, WT_Rev2.2). Injection of CRISPR guides 1, 2, and 3 (orange) results in a deletion of about 400 bp for founder individuals 7 and 12 in serpine3cbg17. Offspring of founder 12 (394 bp deletion) was raised and further crossed. The location of a single serpine3 transcription start site is supported by annotation and activating histone marks H3K4me3 within the respective region (lower gray bar with darkness of color correlating with signal intensity). (B) PCR with primers For3 and Rev4 (green) amplifies a region around coding exon1 of serpine3. Injection of CRISPR guides 4, 5, and 6 (orange) results in a deletion of about 100 bp for founders 1, 9, 18, and 19 in serpine3cbg18. For founder 9, this 92 bp deletion induces a frame-shift in the reading frame (bold) with three early stop codons (two shown as *, third stop located in coding exon 2) and offspring was grown. The deletion is equivalent to deletion of 31 amino acids (red) and +1 nt insertion. The amino acid encoded by a split codon is shown in blue.
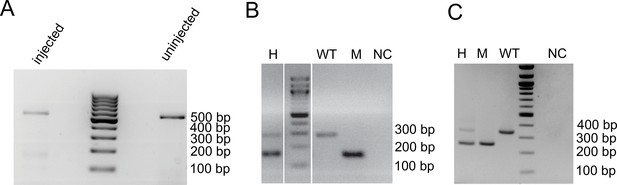
PCRs confirm expected CRISPR-Cas9 induced deletions in serpine3cbg17 and serpine3cbg18 fish.
(A) Genotyping of the serpine3cbg17 with two primers (F0 generation). Injection of CRISPR guides into the one-cell embryo leads to mosaic embryos (several pooled at 72 hpf) with a strong wild type (WT) band at about 557 bp and a weak mutant band at about 161 bp, while uninjected embryos just have a single WT band (557 bp). The raw image is provided as Figure 4—source data 4. (B) Genotyping of serpine3cbg17 with a mix of three primers (F2 generation). Heterozygous animals (H) have two bands, a WT (expected height: 286 bp) and mutant band (expected height: 161 bp), while homozygous mutants (M) and homozygous WT animals show a single band. NC – negative control (water). The raw image is provided as Figure 4—source data 5. (C) Genotyping of serpine3cbg18 with two primers. Heterozygous animals (H) have two bands, a WT (expected height: 342 bp) and mutant band (expected height: 250 bp), while homozygous mutants (M) and homozygous WT animals show a single band. NC – negative control (water). The raw image is provided as Figure 4—source data 6.
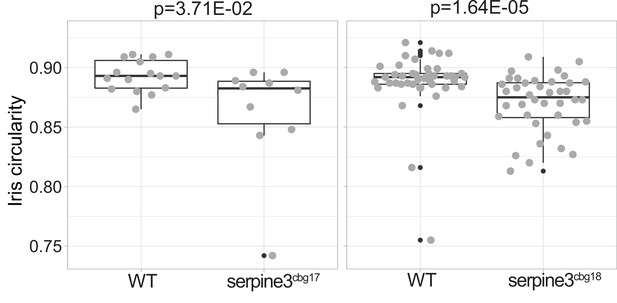
Iris circularity differs between serpine3cbg17 and serpine3cbg18 fish and their respective wild type siblings (WT).
Iris circularity is another descriptor for the deviation of eye shape and is defined as 4π × [Area]/[Perimeter]2. A value of 1 indicates a perfect circle. Boxplots show that iris circularity significantly differs between WT (n=14) and serpine3cbg17 eyes (n=10). The same holds for the comparison of WT (n=40) and serpine3cbg18 eyes (n=40). A Wilcoxon Rank sum test was used. Boxplots display first quartile, median and third quartile with whiskers extending to the maximum and minimum within 1.5 times inter-quartile range. Outliers are shown in black.
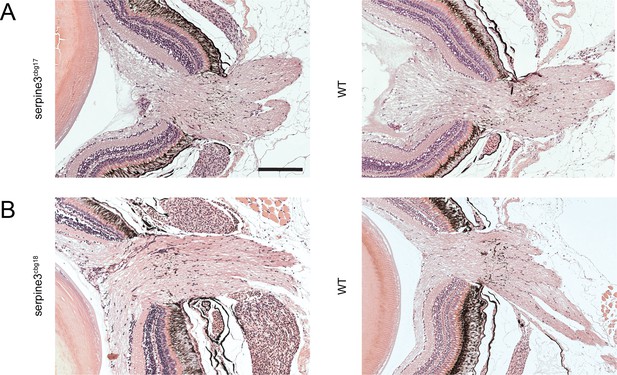
The optic nerve is intact in serpine3cbg17 and serpine3cbg18 fish as shown with a hematoxylin/eosin histology staining.
No obvious difference in optic nerve morphology is observed between serpine3cbg17 (A) and serpine3cbg18 fish (B) and their respective wild type (WT) siblings. Scale bar = 200 µm.
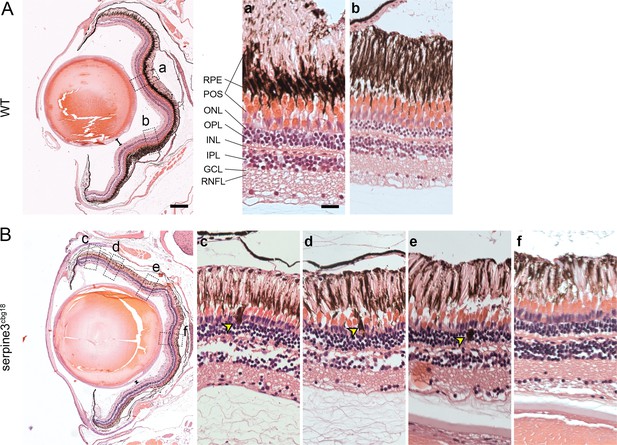
Hematoxylin/eosin histology staining of serpine3cbg18 eyes (14 months) reveals histological differences in comparison to their wild type (WT) siblings (dorsal top, ventral bottom).
We show details of representative overview images for one eye of each genotype on the right. In comparison to WT (A), distance between lens and retina of serpine3cbg18 fish is reduced (distance bars). In the serpine3cbg18 eye (B), all retinal layers are present and distinguishable, although they are not as tightly packed and clearly separated as in the WT (c–f). Moreover, we observed displaced pigmented cells that are located mainly in the photoreceptor outer segment and the outer nuclear layer yellow arrows, (c–e). Furthermore, the photoreceptor outer segment and RPE layer are not clearly separated in the serpine3cbg18 retina. Scale bar in the overviews represents 200 µm and in the magnifications 20 µm.
Tables
Reagent type (species) or resource | Designation | Source or reference | Identifiers | Additional information |
---|---|---|---|---|
gene (Danio rerio) | serpine3 | Ensembl | ENSDART00000132915.2 | |
gene (Homo sapiens) | SERPINE3 | Ensembl | ENST00000524365 | |
strain, strain background (Danio rerio) | WT (AB) | Zebrafish International Resource Center | ||
strain, strain background (Mus musculus) | WT (C57BL/6JOlaHsd) | Envigio | ||
genetic reagent (Danio rerio) | cbg17 | This paper | See Materials and Methods “Generation of KO lines and genotyping” | |
genetic reagent (Danio rerio) | cbg18 | This paper | See Materials and Methods “Generation of KO lines and genotyping” | |
Antibody | Anti-digoxigenin-AP (polyclonal sheep) | Sigma-Aldrich | Cat. #: 11093274910, RRID: AB_2734716 | IF (1:4000) |
Antibody | Anti-digoxigenin-POD conjugated (polyclonal sheep) | Roche | Cat. #: 11207733910, RRID: AB_514500 | IF (1:500) |
Antibody | Anti-gfap ZRF-1 (monoclonal mouse) | Zebrafish International Resource Center | Cat. #: zrf-1, RRID: AB_10013806 | IF (1:200) |
antibody | Anti-choline acetyltransferase (polyclonal goat) | Sigma-Aldrich | Millipore Cat. #: AB144P, RRID: AB_2079751 | IF (1:500) |
antibody | Anti-protein kinase C alpha (polyclonal rabbit) | Santa Cruz Biotechnology | Cat. #: sc-208, RRID: AB_2168668 | IF (1:500) |
antibody | Anti-goat lgG (H+L) Alexa 488 (polyclonal chicken) | Invitrogen | Cat. #: A21467 | IF (1:750) |
antibody | Anti-rabbit lgG (H+L) Alexa 488 (polyclonal goat) | Invitrogen | Cat. #: A11034 | IF (1:750) |
recombinant DNA reagent | serpine3-pCRII-topo vector | This paper | See Materials and Methods “Generation of KO lines and genotyping” | |
sequence-based reagent | Zf_serp_F | This paper | qPCR primer | GAGACCCAAAACCTGCCCTT |
sequence-based reagent | Zf_serp_R | This paper | qPCR primer | AGCCGGAAATGACCGATATTGA |
sequence-based reagent | Mm_serp_F | This paper | qPCR primer | TGGAGCTTTCAGAGGAGGGTA |
sequence-based reagent | Mm_serp_R | This paper | qPCR primer | GATACTGAAGACAAACCCTGTGC |
sequence-based reagent | Zf_rpl13a_F | https://doi.org/10.1111/j.1745-7270.2007.00283.x | qPCR primer | TCTGGAGGAACTGTAAGAGGTATGC |
sequence-based reagent | Zf_rpl13a_R | https://doi.org/10.1111.i.1745-7270.2007.00283.x | qPCR primer | AGACGCACAATCTTGAGAGCGA |
sequence-based reagent | Zf_actb_F | https://doi.org/10.1007/s00441-020-03318-2 | qPCR primer | CGAGCAGGAGATGGGAACC |
sequence-based reagent | Zf_actb_R | https://doi.org/10.1007/s00441-020-03318-2 | qPCR primer | CAACGGAAACGCTCATTGC |
sequence-based reagent | Mm_Rpl27_F | This paper | qPCR primer | TTGAGGAGCGATACAAGACAGG |
sequence-based reagent | Mm_Rpl27_R | This paper | qPCR primer | CCCAGTCTCTTCCCACACAAA |
sequence-based reagent | Guide1 | This paper | Crispr guide | GGTATTTGTACTCTAATGAA |
sequence-based reagent | Guide2 | This paper | Crispr guide | TGTACTCTAATGAAAGGAAC |
sequence-based reagent | Guide3 | This paper | Crispr guide | CTCACACAGGACAATCCGGCAGG |
sequence-based reagent | Guide4 | This paper | Crispr guide | TCTTCTGCAACTCGGGGCCA |
sequence-based reagent | Guide5 | This paper | Crispr guide | TCTCTGTGAGCGTCTGGTAG |
sequence-based reagent | Guide6 | This paper | Crispr guide | AACACTCTGGTTCAGCTCGA |
sequence-based reagent | For1 | This paper | Genotyping primer | GAAATCGCATGTCACGCAGAAAT |
sequence-based reagent | Rev2 | This paper | Genotyping primer | ATATCGGAACTGACATACTGAACG |
sequence-based reagent | Rev2.2 | This paper | Genotyping primer | GTGAGCTTCGTGTTTGTGGT |
sequence-based reagent | For3 | This paper | Genotyping primer | GGCATTGTTGAGATTCAGTAGTCA |
sequence-based reagent | Rev4 | This paper | Genotyping primer | CAGTTTACTCCTACCATTGACATC |
peptide, recombinant protein | Alt-R CRISPR-Cas 9 Nuclease | IDT | Cat. #: 10000735 | |
commercial assay or kit | RNeasy mini kit | Qiagen | Cat. #: 74,106 | |
commercial assay or kit | RNeasy midi kit | Qiagen | Cat. #: 75,144 | |
commercial assay or kit | ProtoScript II First Strand Synthesis kit | NEB | Cat. #: E6560S | |
commercial assay or kit | TSA Plus Cy3/Cy5 kit | Perkin-Elmer | Cat. #: NEL744001KT | |
commercial assay or kit | Alt-R CRISPR-Cas9 tracr RNA | IDT | Cat. #: 1072532 | |
software, algorithm | Enrichr | https://doi.org/10.1093/nar/gkw377 | RRID:SCR_001575 | |
software, algorithm | TOGA | Kirilenko et al., titled 'Integrating gene annotation with orthology inference at scale', under review (https://github.com/hillerlab/TOGA; Kirilenko and Hiller, 2022) | ||
software, algorithm | CESAR 2.0 | https://doi.org/10.1093/bioinformatics/btx527 | ||
software, algorithm | RELAX | https://doi.org/10.1093/molbev/msu400 | RRID:SCR_016162 | |
software, algorithm | MACSE v2.0 | https://doi.org/10.1093/molbev/msy159 | ||
software, algorithm | Jalview | https://doi.org/10.1093/molbev/msy159 | RRID:SCR_006459 | |
software, algorithm | Human protein atlas | https://doi.org/10.1038/nbt1210-1248 | RRID:SCR_006710 | |
software, algorithm | Gene Expression Atlas | https://doi.org/10.1093/nar/gkz947 | RRID:SCR_007989 | |
software, algorithm | PyMOL | Schrödinger and DeLano, 2020 | RRID:SCR_000305 | |
software, algorithm | Fiji | https://doi.org/10.1038/nmeth.2019 | RRID:SCR_002285 | |
software, algorithm | R | Other | RRID:SCR_001905 | https://cran.r-project.org/src/base/R-3/ |
software, algorithm | ggplot2 | Other | RRID:SCR_014601 | http://docs.ggplot2.org/current/ |
software, algorithm | tseries | Other | https://CRAN.R-project.org/package=tseries | |
software, algorithm | effsize | Other | https://cran.r-project.org/web/packages/effsize/index.html | |
software, algorithm | SignalP5 | https://doi.org/10.1038/s41587-019-0036-z | ||
software, algorithm | DeepLoc | https://doi.org/10.1093/bioinformatics/btx431 | ||
software, algorithm | ChopChop v2 | https://doi.org/10.1093/nar/gkw398 | RRID:SCR_015723 |
Additional files
-
Supplementary file 1
Annotated protein alignment of intact and complete mammalian SERPINE3.jvp format is to be opened in Jalview.
- https://cdn.elifesciences.org/articles/77999/elife-77999-supp1-v2.zip
-
Supplementary file 2
Predicted structure of human SERPINE3 (AlphaFold 2).
- https://cdn.elifesciences.org/articles/77999/elife-77999-supp2-v2.zip
-
Supplementary file 3
Comparison of 3D structures of crystallized SERPINs to the predicted human SERPINE3.
- https://cdn.elifesciences.org/articles/77999/elife-77999-supp3-v2.xls
-
Transparent reporting form
- https://cdn.elifesciences.org/articles/77999/elife-77999-transrepform1-v2.docx