Gene and protein expression and metabolic flux analysis reveals metabolic scaling in liver ex vivo and in vivo
Figures
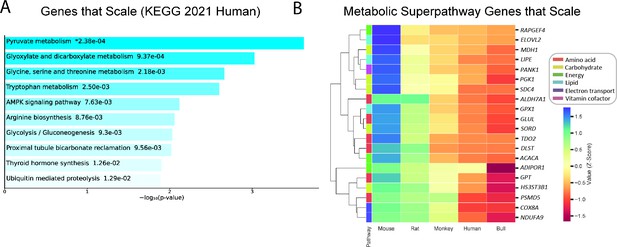
Genes that follow the pattern of allometric scaling are most strongly related to metabolism.
(A) KEGG Pathway enrichment of all genes that are expressed with an inverse correlation to body mass, and (B) clustering heatmap of scaled genes that belong to one of six Reactome metabolic superpathways. All samples were obtained from males. For clarity, the human gene (and style of writing human gene names) are shown. RAPGEF, rap guanine nucleotide exchange factor; ELOVL2, Elongation of Very Long Chain Fatty Acids-Like 2; MDH1, malate dehydrogenase 1; LIPE, hormone-sensitive lipase E; PANK1, pantothenate kinase 1; PGK1, phosphoglycerate kinase 1; SDC4, syndecan 4; ALDH7A1, aldehyde dehydrogenase 7 family member A1; GPX1, glutathione peroxidase 1; GLUL, glutamate-ammonia ligase; SORD, sorbitol dehydrogenase; TDO2, tryptophan 2,3-dioxygenase; DLST, dihydrolipoamide S-succinyltransferase; ACACA, acetyl-CoA carboxylase-alpha; ADIPOR1, adiponectin receptor-1; GPT, glutamic-pyruvate transaminase; HS3ST3B1, heparan sulfate-glucosamine 3-sulfotransferase 3B1; PSMD5, proteasome 26 S subunit, non-ATPase-5; COX8A, cytochrome c oxidase subunit 8 A; NDUFA9, NADH:ubiquinone oxidoreductase subunit A9.
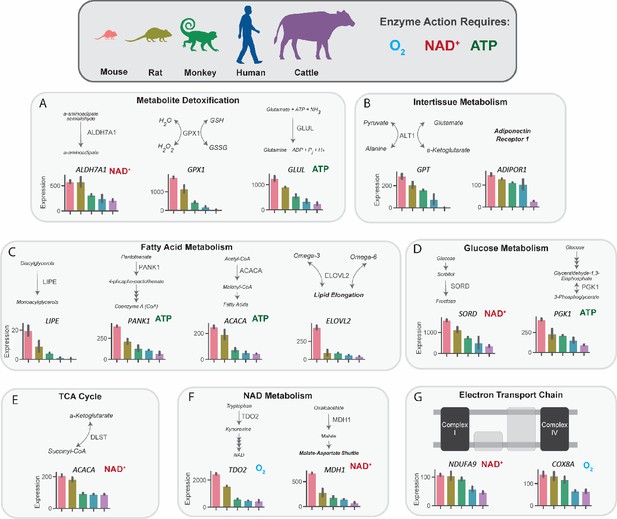
Metabolic genes that are expressed inversely proportionally to body size implicate key pathways in substrate and nucleotide supply, glucose and fatty acid flux, oxygen consumption, and detoxification pathways.
mRNA expression of key regulatory genes related to metabolite detoxication (A), intertissue metabolism (B), fatty acid metabolism (C), glucose metabolism (D), tricarboxylic acid (TCA) cycle, NAD metabolism (F), and the electron transport chain (G) in mice, rats, monkeys, humans, and cattle. Bars denote expression levels by an organism, following the same order shown in the cartoon of organisms. Expression was normalized to counts per million and was then further normalized for sequencing depth and transcript length. All genes met an adjusted p-value threshold of 0.01 using a one-way ANOVA with the Bonferroni correction for multiple comparisons. All samples were obtained from males (n=2 replicates per species). ALDH7A1, aldehyde dehydrogenase 7 family member A1; GPX1, glutathione peroxidase 1; GLUL, glutamate-ammonia ligase; GPT, glutamic-pyruvate transaminase; ADIPOR1, adiponectin receptor-1; LIPE, hormone-sensitive lipase E; PANK1, pantothenate kinase 1; ACACA, acetyl-CoA carboxylase-alpha; ELOVL2, Elongation of Very Long Chain Fatty Acids-Like 2; SORD, sorbitol dehydrogenase; PGK1, phosphoglycerate kinase 1; DLST, dihydrolipoamide S-succinyltransferase; TDO2, tryptophan 2,3-dioxygenase; MDH1, malate dehydrogenase 1; NDUFA9, NADH:ubiquinone oxidoreductase subunit A9; COX8A, cytochrome c oxidase subunit 8 A.
-
Figure 2—source data 1
Source data for Figure 2 and Figure 2—figure supplement 1.
- https://cdn.elifesciences.org/articles/78335/elife-78335-fig2-data1-v1.xlsx
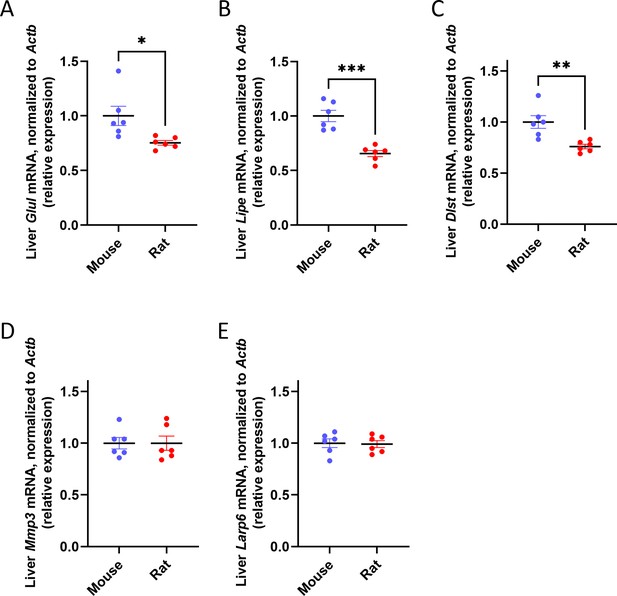
Liver metabolic genes, but not structural genes, are expressed inversely proportionally to body size in rodents.
mRNA expression of metabolic genes: (A) Glul, (B) Lipe, (C) Dlst, and structural genes: (D) Mmp3, (E) Larp6, all expressed relative to Actb (β-actin). *p<0.05, **p<0.01, ***p<0.0001.
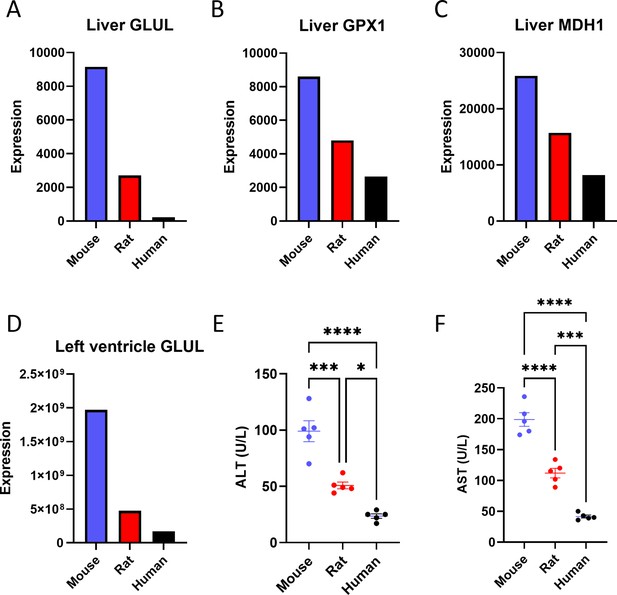
Proteomics reveals a negative correlation between body size and the expression of some liver proteins.
Liver (A) glutamate-ammonia ligase (GLUL), (B) glutathione peroxidase 1 (GPX1), and (C) malate dehydrogenase 1 (MDH1) protein expression. (D) GLUL protein expression in the left ventricle of the heart. The proteomics analysis was performed on n=1 per species, so statistical comparisons were not possible. (E) Plasma alanine aminotransferase (ALT) and (F) aspartate aminotransferase (AST) concentrations (for both transaminases, n=5 per species). *p<0.05, ***p<0.001, ****p<0.0001.
-
Figure 3—source data 1
Source data for Figure 3 and Figure 3—figure supplement 1.
- https://cdn.elifesciences.org/articles/78335/elife-78335-fig3-data1-v1.xlsx
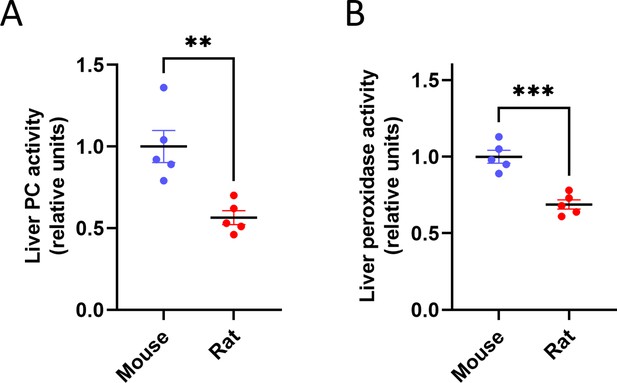
Liver metabolic enzyme activity is inversely proportional to body size.
(A) Pyruvate carboxylase and (B) Peroxidase activity. **p<0.01, ***p<0.001; n=5 per species.
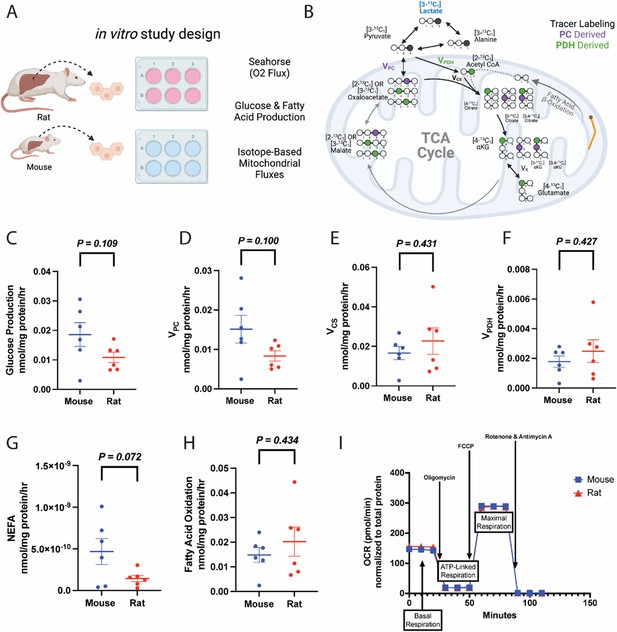
Metabolic fluxes are not different between mouse and rat hepatocytes in vitro.
(A) Study design. This figure was made using Biorender.com. (B) Tracer labeling strategy. (C) Glucose production. (D) Gluconeogenesis from pyruvate (pyruvate carboxylase flux, VPC). (E) Citrate synthase flux (VCS), i.e., mitochondrial oxidation. (F) Pyruvate dehydrogenase flux (VPDH), i.e., the contribution of glucose via glycolysis to total mitochondrial oxidation. (G) Non-esterified fatty acid (NEFA) production. (H) The contribution of fatty acid oxidation to citrate synthase flux. (I) Oxygen consumption rate (OCR) during a mitochondrial stress test. In all panels, hepatocytes from wild-type males were studied, and groups were compared using the two-tailed unpaired Student’s t-test. No significant differences were observed. In all panels, the mean ± SEM. of six biological replicates (averaged from three technical replicates per biological replicate) is shown.
-
Figure 4—source data 1
Source data for Figure 4 and Figure 4—figure supplement 1.
- https://cdn.elifesciences.org/articles/78335/elife-78335-fig4-data1-v1.xlsx
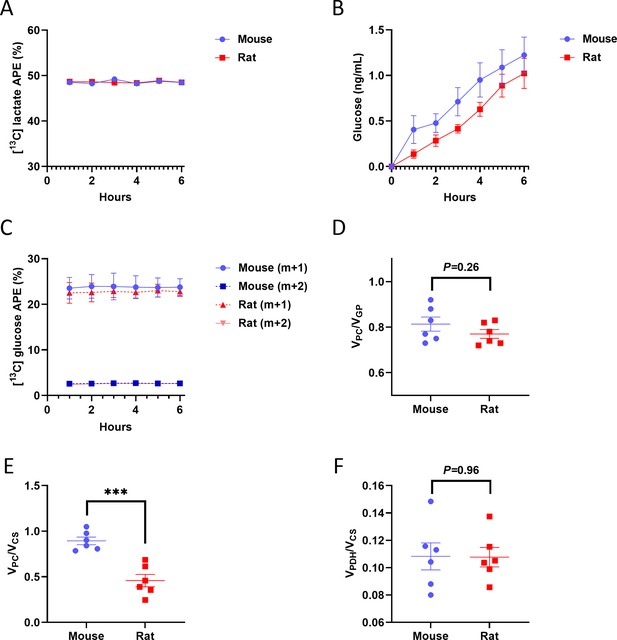
Validation of tracer assumptions and flux ratios in the in vitro hepatocyte PINTA flux studies.
(A) Media 13C lactate m+1 enrichment. (B) Media 13C glucose m+1 and m+2 enrichment. (C) Media glucose concentration. (D) VPC/VGP. (E) VPC/VCS. ***p<0.001. (F) VPDH/VCS. In all panels, the mean ± SEM. of six biological replicates (averaged from three technical replicates per biological replicate) is shown.
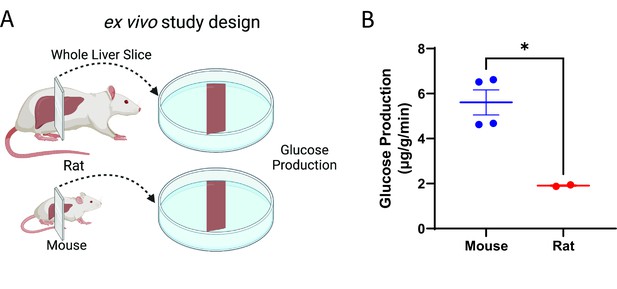
Glucose production scales ex vivo in liver slices.
(A) Study design. This figure was made using Biorender.com. (B) Glucose production. Groups were compared by the two-tailed unpaired Student’s t-test. Liver slices from male, wild-type animals (n=4 mice and 2 rats, three technical replicates per biological replicate) were studied.
-
Figure 5—source data 1
Source data for Figure 5.
- https://cdn.elifesciences.org/articles/78335/elife-78335-fig5-data1-v1.xlsx
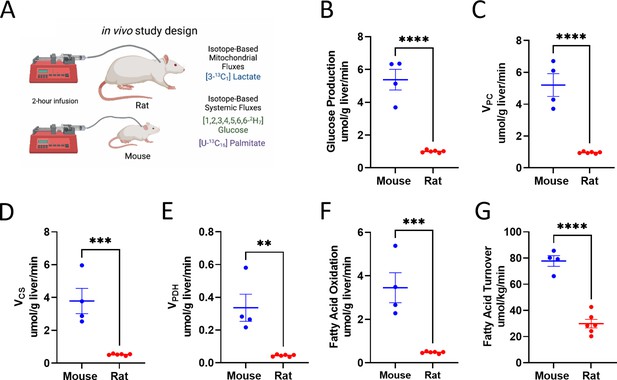
Analysis of systemic metabolic fluxes suggests in vivo metabolic scaling in mice vs. rats.
(A) Study design. (B) Endogenous glucose production. (C) Gluconeogenesis from pyruvate (VPC). (D) VCS, i.e., mitochondrial oxidation. (E) VPDH, i.e., the contribution of glucose via glycolysis to total mitochondrial oxidation. (F) Palmitate (fatty acid) turnover. (G) The contribution of fatty acid oxidation to citrate synthase flux. In all panels, groups were compared using the two-tailed unpaired Student’s t-test. Male rodents (n=4 mice and 6 rats) were studied.
-
Figure 6—source data 1
Source data for Figure 6 and Figure 6—figure supplement 1.
- https://cdn.elifesciences.org/articles/78335/elife-78335-fig6-data1-v1.xlsx
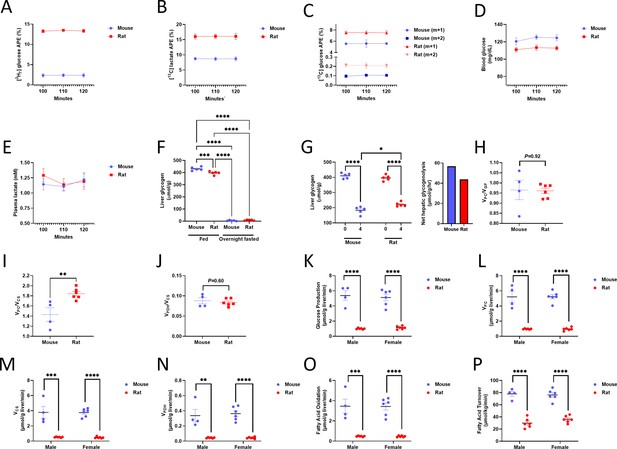
Validation of tracer assumptions, flux ratios, and lack of sex differences in the in vivo flux studies.
(A) Plasma 2H7 glucose enrichment. (B) Plasma 13C lactate m+1 enrichment. In panels (A)-(C), n=4 mice and 6 rats. (C) Plasma 13C glucose m+1 and m+2 enrichment. (D) Blood glucose and (E) Plasma lactate concentrations. n=6 per group (panels (D)-(E)). In panels (A)-(E), data are the mean ± SEM. of n=6 per species. (F) Liver glycogen concentration in fed and overnight fasted rodents, as well as in (G) recently fed (0 and 4 hr fasted) rodents. n=5 mice and 5 rats. (H) /n vivo VPC/VEGP, (I) VPC/VCS, and (J) VPDH/VCS (n=4 mice and 6 rats). (K) Endogenous glucose production, (L) Pyruvate carboxylase flux, and (M) Citrate synthase flux in male and female rodents (in panels (K)-(P), n=4 male mice and 6 for all other groups; the data from males are duplicated from Figure 6B–G). The contributions of (N) Glucose (through pyruvate dehydrogenase) and (O) Fatty acids to total mitochondrial oxidation in male and female rodents. (P) In vivo fatty acid (palmitate) turnover in male and female rodents. In all panels, **p<0.01, ***p<0.001, ****p<0.0001.
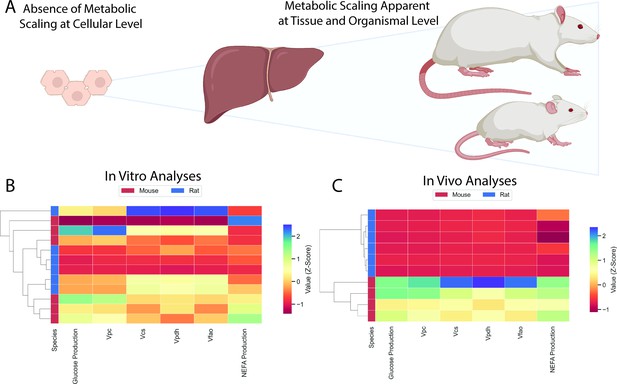
Comparison of in vitro and in vivo results.
(A) Study workflow. (B) Clustering heatmap demonstrating the absence of metabolic differences in vitro. (C) Clustering heatmap demonstrating metabolic differences between mice and rats in vivo. In panels (B) and (C), mouse and rat color legends correspond to the species label attached to the dendrogram on the leftmost of each graph. Vpc = pyruvate carboxylase flux, Vcs = citrate synthase flux, Vpdh = pyruvate dehydrogenase flux, Vfao = fatty acid oxidation, NEFA = non-esterified fatty acid concentrations. All data presented in Figures 3 and 5 were utilized in the classical clustering analysis and are included in this figure.
Additional files
-
Supplementary file 1
Primer sequences used for qPCR.
- https://cdn.elifesciences.org/articles/78335/elife-78335-supp1-v1.docx
-
Supplementary file 2
Flux ratios and absolute rates measured in mice infused with [3-13C] lactate.
APE indicates the atom percent enrichment (in animals infused with 13C tracer), TCA denotes the tricarboxylic acid cycle, and GNG denotes gluconeogenesis. By convention, Va represents the flux through pathway a.
- https://cdn.elifesciences.org/articles/78335/elife-78335-supp2-v1.docx
-
MDAR checklist
- https://cdn.elifesciences.org/articles/78335/elife-78335-mdarchecklist1-v1.docx