Proton-transporting heliorhodopsins from marine giant viruses
Figures
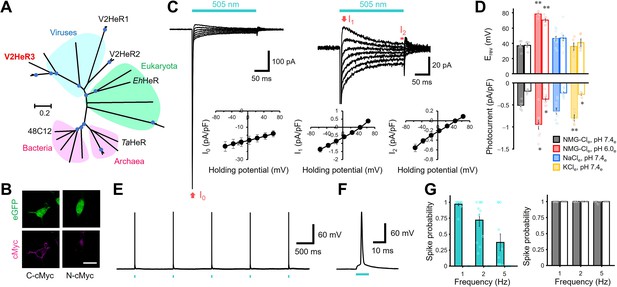
Light-gated inward proton transport of a viral heliorhodopsin (HeR) from Emiliania huxleyi virus 202 (V2HeR3).
(A) Phylogenetic tree of HeRs, which includes three viral HeRs from E. huxleyi 202 (V2HeR1-3), a eukaryotic HeR from E. huxleyi (Ehux-HeR), an archaeal HeR (TaHeR) and a bacterial HeR (48C12). (B) eGFP fluorescence (top, green) and immunofluorescence staining (bottom, magenta) observation of V2HeR3 with a cMyc epitope tag at the C terminus (left) and the N terminus (right) in cultured ND7/23 cells. Scale bar, 20 μm. (C) Electrophysiological measurements of V2HeR3-driven photocurrent in ND7/23 cells. The cells were illuminated with light (λ=505 nm, 24.5 mW/mm2) during the time region shown by the blue bars. The membrane voltage was clamped from −60 to +60 mV for every 20 mV step. The pipette solution was 110 mM NMG-Cli, pH 7.4i, the bath solution was 140 mM NaCle, pH 7.4en=10 cells. (D) Corresponding reversal voltage (Erev) for each internal condition (upper), and comparison of photocurrent amplitudes at 0 mV for different internal cations (bottom). Square-block bar graph indicates Erev or amplitude from peak photocurrent (I1), open bar graphs indicate Erev or amplitude from steady-state photocurrent (I2). The pipette solution was 110 mM NMG-Cli, pH 7.4i, the bath solution was 140 mM NMG-Cle, pH 7.4e (black), 140 mM NMG-Cle, pH 6.0e (red), 140 mM NaCle, pH 7.4e (blue) or 140 mM KCle, pH 7.4e (yellow). n=5–10 cells. (*p<0.05, **p<0.01). (E) Representative responses of a V2HeR3-expressing neuron to 10 ms light pulses (left, λ=505 nm, 24.5 mW/mm2) at 1 Hz. (F) The firstaction potential in E. the X axis is expanded. (G) Comparison of spike probability by electrical stimulation (right, 300 pA current injections) or light stimulation (left, λ=505 nm, 24.5 mW/mm2). The Square-block bar indicates spike probability from V2HeR3-expressing neurons, the open bar indicates spike probability from the neurons without V2HeR3. n=6–11 cells.
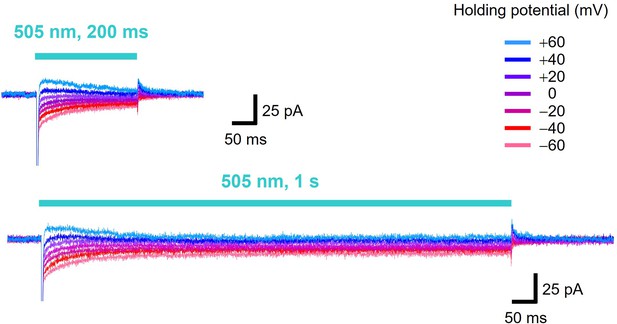
Photocurrents of viral HeR from Emiliania huxleyi virus 202 (V2HeR3) under different illumination periods.
Electrophysiological measurements of V2HeR3-driven photocurrent in ND7/23 cells. The cells were illuminated with light (λ=505 nm, 24.5 mW/mm2) during the time region shown by blue bars. The membrane voltage was clamped from −60 to +60 mV for every 20 mV step. The pipette solution was 110 mM NaCli, pH 7.4i, the bath solution was 140 mM NaCle, pH 7.4e.
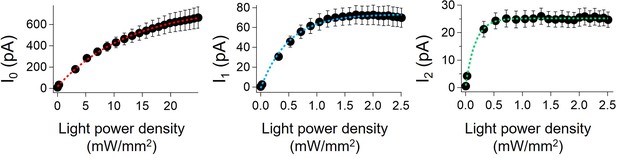
Photocurrents of viral HeR from Emiliania huxleyi virus 202 (V2HeR3) under different light intensities.
Photocurrent amplitude was plotted as a function of light power. Membrane voltage was clamped at −60 mV, 505 nm light were illuminated respectively. Half saturation maxima (EC50) of the transient photocurrent (I0; left), peak photocurrent (I1; middle), and steady-state photocurrent (I2; right) are 13±2.2 mW/mm2 (± SEM), 0.48±0.017 and 0.18±0.016 mW/mm2, respectively. The pipette solution was 110 mM NMG-Cli, pH 7.4i, the bath solution was 140 mM NaCle, pH 7.4e. n=5–8 cells.
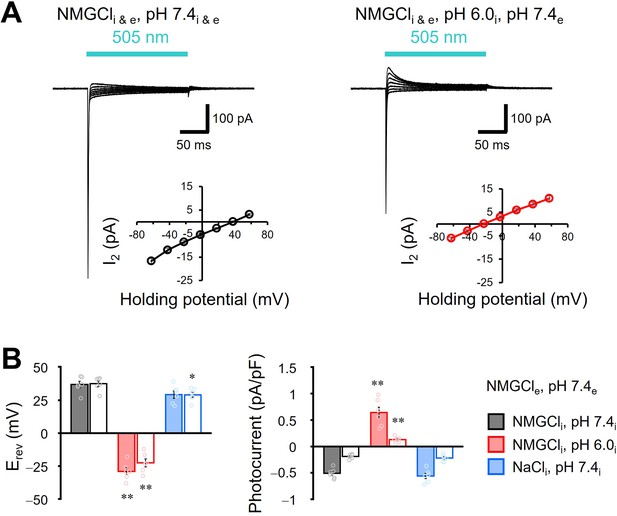
Selectivity of cations in the transport of viral HeR from Emiliania huxleyi virus 202 (V2HeR3).
(A) Representative photocurrent trace of V2HeR3 recorded in ND7/23 cells. The cells were illuminated with light (λ=505 nm, 24.5 mW/mm2) during the time region shown by blue bars. Voltage was clamped from −60 to +60 mV in 20 mV steps. The bath solution was 140 mM NMG-Cle, pH 7.4e. The pipette solution was 110 mM NMG-Cli, pH 7.4i (left). The pipette solution was 110 mM NMG-Cli, pH 6.0i (right). n=6 cells. (B) Corresponding reversal potential (Erev) for each internal condition (left), and comparison of photocurrent density at 0 mV for different internal cations (right). Square-block bars indicate Erev or amplitude from I1, open bars indicate Erev or amplitude from steady-state photocurrent (I2). n=6 cells. (*p<0.05, **p<0.01).
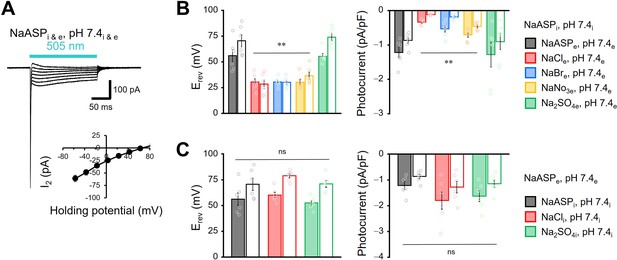
Effect of anions in the transport of viral HeR from Emiliania huxleyi virus 202 (V2HeR3).
(A) Representative photocurrent trace of V2HeR3 recorded in ND7/23 cells. The cells were illuminated with light (λ=505 nm, 24.5 mW/mm2) during the time indicated by a blue bar. Voltage was clamped from −60 to +60 mV in 20 mV steps. The pipette solution was 110 mM NaASPi, pH 7.4i, the bath solution was 140 mM NaASPe, pH 7.4e. n=6 cells. Inset, I-V plot of steady-state current (I2). (B) and (C) Reversal potential (Erev) for each external (B) or internal (C) condition (left), and comparison of photocurrent density at 0 mV for different external (B) or internal (C) anions (right). Square-block bar graphs indicate Erev or amplitude from peak photocurrent (I1), open bar graphs indicate Erev or amplitude from steady-state photocurrent (I2). n=6 cells. (**p<0.01).
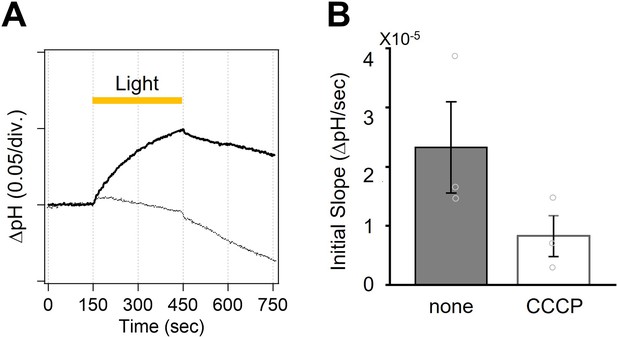
Ion transport activity of viral HeR from Emiliania huxleyi virus 202 (V2HeR3) in Pichia pastoris measured by a pH-electrode.
(A) Ion pump activity of P. pastoris cell suspensions (100 mM NaCl) expressing V2HeR3-WT without (solid line) and with (dotted line) CCCP. (B) Initial slope in light-induced pH changes. n=3.
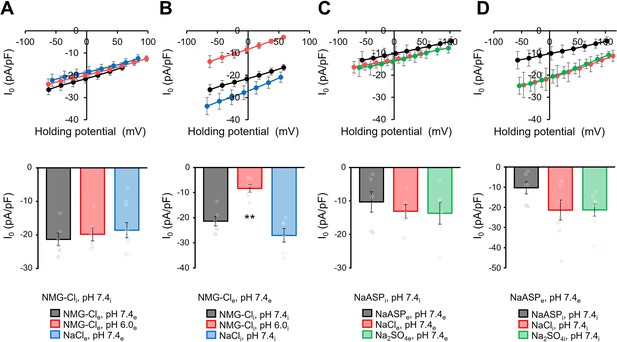
Effect of anions in the I0 component of viral HeR from Emiliania huxleyi virus 202 (V2HeR3).
(A–D) Upper: I-V relationship of the photocurrent (I0) under various ionic conditions. (A) The pipette solution contained 110 mM NMG-Cl at pH 7.4i, while the bath solution was varied as shown below the graph. (B) The bath solution contained 140 mM NMG-Cl at pH 7.4e, while the pipette solution was varied as shown below. (C) The pipette solution contained 110 mM NaASP, pH 7.4i, while the bath solution was varied as shown below. (D) The bath solution contained 140 mM NaASP, pH 7.4e, while the pipette solution was varied as shown below. n=6 cells. (A–D) Lower: Photocurrent density at 0 mV for each external or internal condition. n=6–8 cells. (**p<0.01).
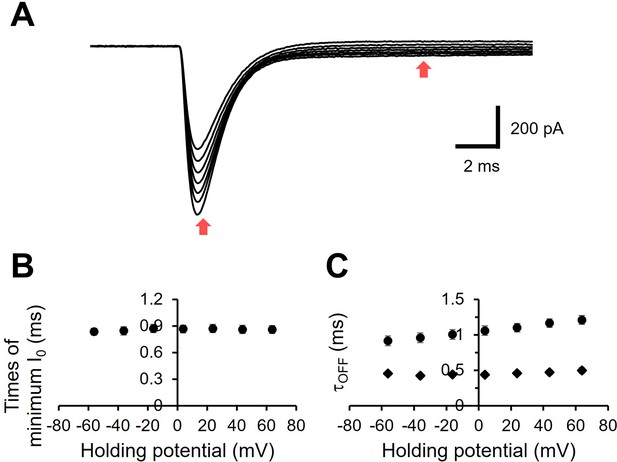
Kinetics properties of the I0 component.
(A) The same photocurrent traces as Figure 1C. The X axis is expanded. (B) Time of the negative-going peak was plotted as a function of membrane voltage. (C) The voltage dependency of the time constants. Exponential fitting was performed to determine the time constant of I0 relaxation. The fitting was made between the time points indicated by two red arrows in panel A. Two time constants were obtained from each current trace.
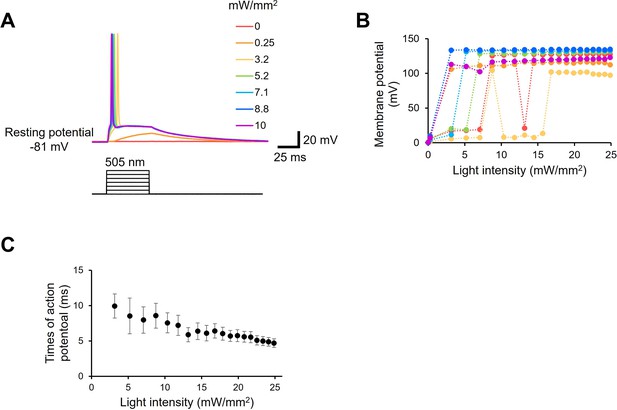
Light power dependency of neuronal manipulation by viral HeR from Emiliania huxleyi virus 202 (V2HeR3).
(A) Representative action potentials evoked by various light power. 505 nm light was applied to neurons for 50 ms as indicated by black bars. (B) Evoked action potentials from seven neurons were plotted as a function of light power. (C) Time of the peak potential was fitted as a function of the light power.
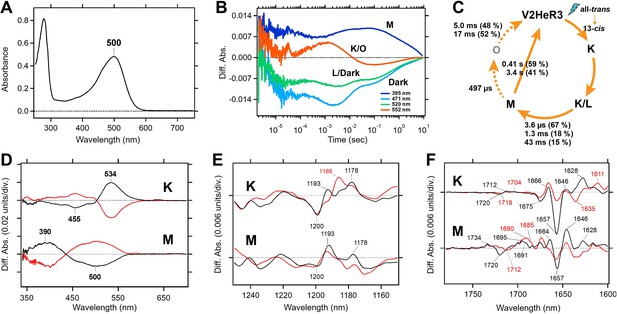
Molecular properties of the purified viral HeR from Emiliania huxleyi virus 202 (V2HeR3) proteins expressed in Pichia pastoris cells.
(A) UV-visible absorption spectrum of V2HeR3 in detergent (0.1% n-dodecyl-β-D-maltoside [DDM]). (B) Time evolutions of transient absorption changes at characteristic wavelengths of specific photointermediates of V2HeR3. (C) Photocycle of V2HeR3 determined by analyzing the time evolution with multiexponential functions. (D) Light-induced low-temperature K-minus-dark (top) and M-minus-dark (bottom) difference UV-visible spectra of V2HeR3 obtained at 100 and 230K, respectively. Black curves represent the formation of the K and M intermediates by illuminating at 500 and>490nm, respectively, while red curves represent the reversion from the intermediates by illuminating at>530 and 400nm, respectively. (E) Light-induced low-temperature K-minus-dark (top) and M-minus-dark (bottom) difference FTIR spectra of V2HeR3 obtained at 100 and 230K, respectively, in the 1250–1150 cm–1 region. (F) Light-induced low-temperature K-minus-dark (top) and M-minus-dark (bottom) difference FTIR spectra of V2HeR3 in H2O (black) and D2O (red) obtained at 100 and 230K, respectively, in the 1780–1600 cm–1 region.
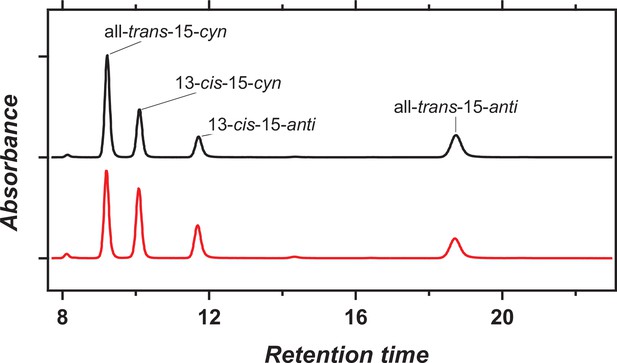
HPLC pattern of the retinal chromophore in viral HeR from Emiliania huxleyi virus 202 (V2HeR3).
HPLC pattern of retinal extracted from V2HeR3 in the dark (black) and during light illumination at λ=500 ± 10nm (red). The populations of the all-trans and 13-cis forms are 64% and 36% in the dark, and 51% and 49% in the light, respectively.
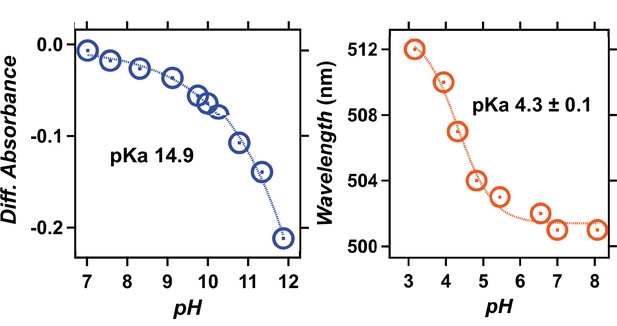
pH titration of viral HeR from Emiliania huxleyi virus 202 (V2HeR3).
UV-visible absorption spectra (left) and the λmax (right, orange solid circles) of heliorhodopsin 48C12 at pH 2.8–8.4. When pH is lowered, a red-shift of the absorption is observed, which is commonly reported for many type-1 rhodopsins and reflects protonation of counterions. Thus, the red-shift of heliorhodopsin 48C12 originates from protonation of E107, which is fitted with the Henderson–Hasselbalch equation (blue dashed line), and the pKa of counterion (E107) is estimated to be 3.7. At pH<2.8, a large blue shift to 443nm is observed, presumably owing to the acid denaturation of the protein.
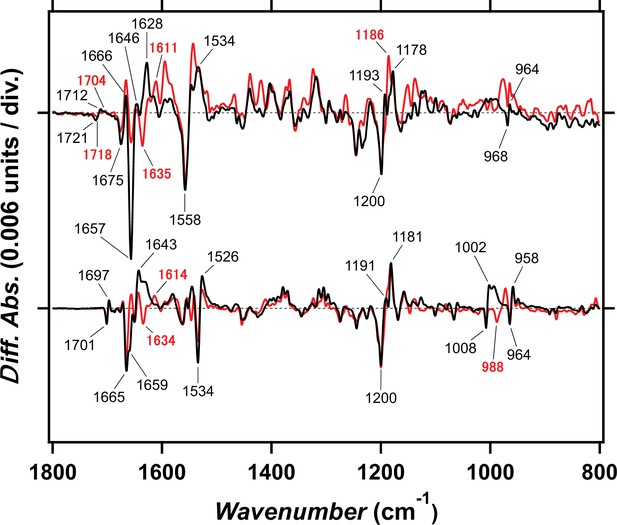
Light-induced difference FTIR spectra of viral HeR from Emiliania huxleyi virus 202 (V2HeR3) and TaHeR at 77K.
Light-induced difference FTIR spectra of V2HeR3 (upper) and TaHeR (lower) in the 1800–800 cm–1 region at 100 and 77K, respectively. The spectra of TaHeR are reproduced from Shihoya et al., 2019. Positive and negative bands represent vibrations of the primary K intermediate and the unphotolyzed state, respectively, and characteristic vibrational bands are tagged. C=C stretch, C-C stretch, and hydrogen-out-of-plane (HOOP) vibrations of the retinal chromophore appear at 1550–1500, 1250–1100, and 1000–900 cm–1, respectively. These signals resemble between V2HeR3 and TaHeR, suggesting common structural changes such as all-trans to 13-cis isomerization with distorted chromophore. Amide-I vibration of peptide backbone and carboxylic C=O stretch appear at 1700–1600 and 1800–1700 cm–1, respectively. Intense band at 1657 (−)/1628 (+) cm–1 in V2HeR3 is a signature of larger changes in the secondary structure in V2HeR3 than that in TaHeR. Carboxylic C=O stretch vibrations appear only for V2HeR3 at 1721 (−)/1712 (+) cm–1, not for TaHeR.
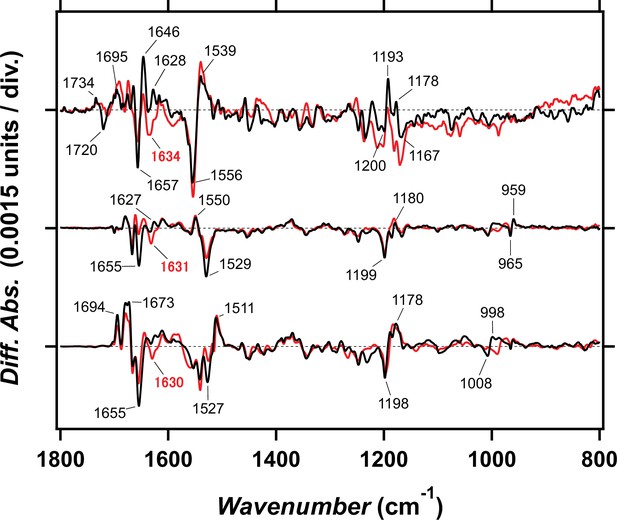
Light-induced difference FTIR spectra for late intermediates of viral HeR from Emiliania huxleyi virus 202 (V2HeR3) and TaHeR.
Light-induced FTIR spectra of V2HeR3 at 230K (upper), TaHeR at 240K (middle), and TaHeR at 277K (lower) in the 1800–800 cm–1 region. The spectra of TaHeR are reproduced from Chen et al., 2000. While negative bands correspond to vibrations of the unphotolyzed state, positive bands of the upper, middle, and lower panels correspond to the M intermediate of V2HeR3, the M intermediate of TaHeR, and the O intermediate of TaHeR, respectively. Strong amide-I band, observed at 1657 (−)/1646 (+) cm–1 in V2HeR3 (upper), is similar to the case in the O intermediate of TaHeR (lower), not the M intermediate of TaHeR (middle).
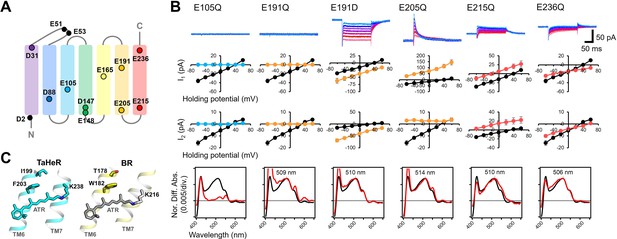
Electrophysiological analysis of the carboxylate mutants of viral HeR from Emiliania huxleyi virus 202 (V2HeR3).
(A) Aspartate (D) or glutamate (E) in V2HeR3. Among 13 residues, 10 transmembrane aspartate and glutamate were replaced with asparagine (D-to-N) and glutamine (E–to–Q), respectively. (B) Photocurrents (top), I-V plots (middle), and absorption spectra (bottom) of mutants which differ from those of the wild type. Black curves in I-V plots and absorption spectra are the results of the wild type. The pipette solution was 110mM NaCli, pH 7.4i, the bath solution was 140mM NaCle, pH 7.4e. n=6–7cells. (C) Crystal structures of TaHeR (left) and a light-driven proton pump bacteriorhodopsin (BR) (right). Corresponding residues of E191 in V2HeR3 are I199 in TaHeR and T178 in BR. In TM6, highly conserved phenylalanine and tryptophan exist in HeRs and type-1 rhodopsins, respectively, while V2HeR3 contains tryptophan at this position.
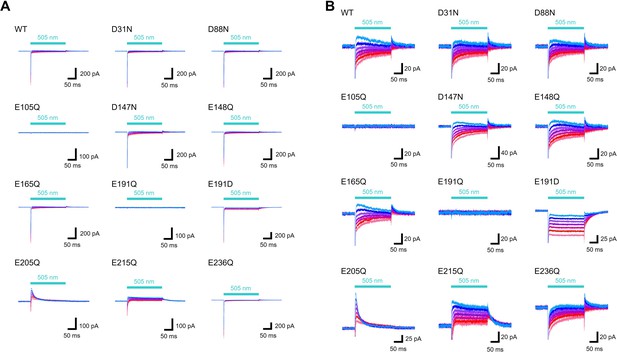
Representative photocurrent traces of the wild-type and mutants of viral HeR from Emiliania huxleyi virus 202 (V2HeR3).
(A) Electrophysiological measurements of V2HeR3-wt and the variants in ND7/23cells. The cells were illuminated with light (λ=505nm, 24.5 mW/mm2). The membrane voltage was clamped from −60 to+60mV for every 20mV step. The pipette solution was 110mM NaCli, pH 7.4i, the bath solution was 140mM NaCle, pH 7.4e. (B) The same traces as (A).The Y axis is expanded.
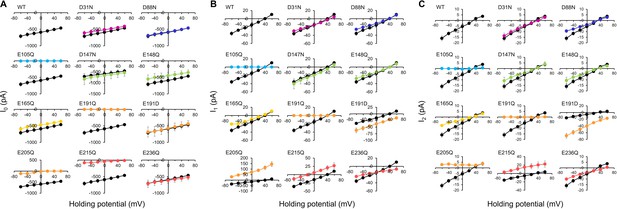
Photocurrent-voltage plots (I-V plot) of the wild-type and mutants of viral HeR from Emiliania huxleyi virus 202 (V2HeR3).
Electrophysiological measurements of V2HeR3-wt or variants-driven photocurrent in ND7/23cells. The cells were illuminated with light (λ=505nm, 24.5 mW/mm2). The membrane voltage was clamped from −60 to+60mV for every 20mV step. The I-V plot of the transient current component (I0) (A), the I1 component (B), and the I2 component are depicted. Black curves are the results of V2HeR3-wt. The pipette solution was 110mM NaCli, pH 7.4i, the bath solution was 140mM NaCle, pH 7.4e. n=6–7cells.
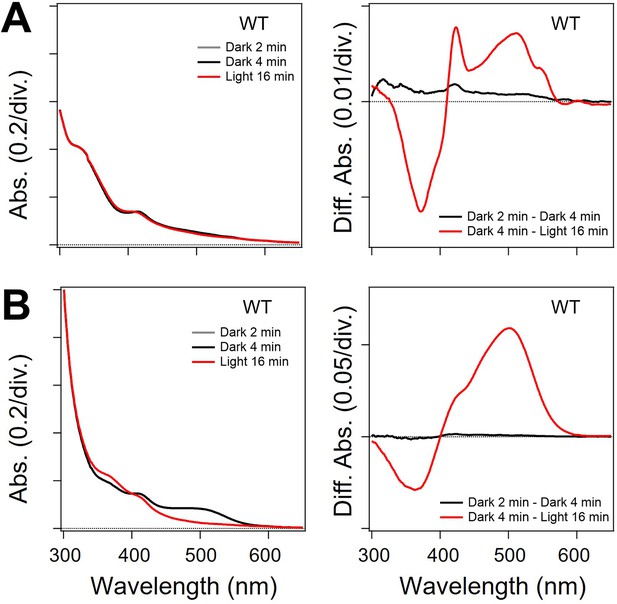
Absolute and difference absorption spectra of viral HeR from Emiliania huxleyi virus 202 (V2HeR3) in the experiments of hydroxylamine bleach.
Light-induced difference absorption spectra in the presence of hydroxylamine. Left, Absolute absorption of n-dodecyl-β-D-maltoside (DDM)-solubilized sample. Right, Difference absorption of before and after illumination: (A) ND7/23cells; (B)Pichia pastoris cells.
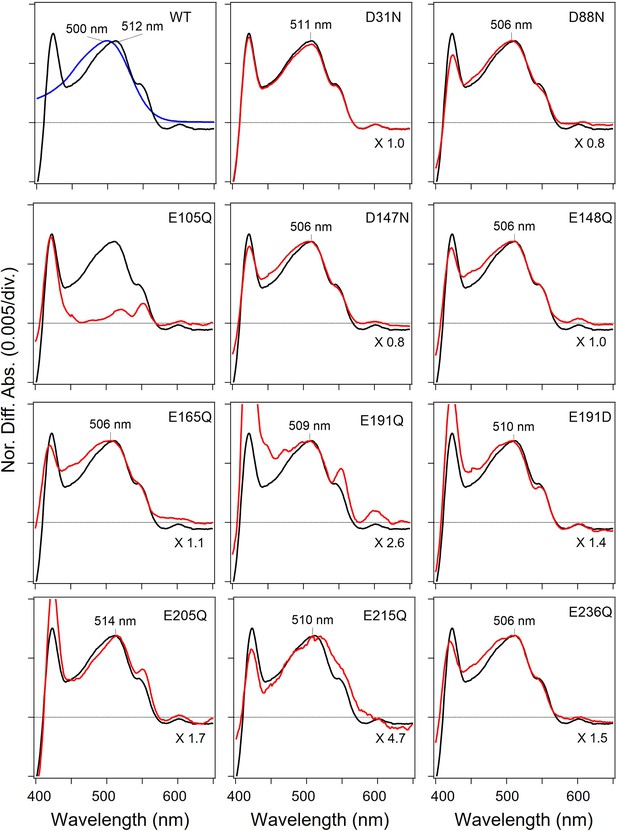
Absorption spectra of the wild-type (WT) and mutants of viral HeR from Emiliania huxleyi virus 202 (V2HeR3) obtained by the hydroxylamine bleach of ND7/23cells.
Light-induced difference absorption spectra in the presence of 50mM hydroxylamine of the WT (black line) and the mutants (red line). Mutants and WT spectra were normalized by use of a positive peak at each λmax. Blue line in WT shows the absorption spectra of the purified V2HeR3.
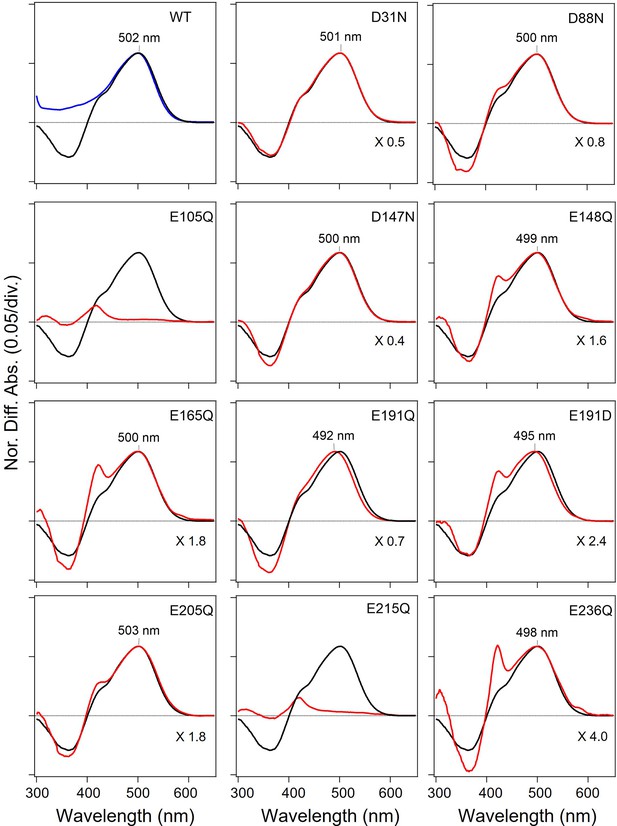
Absorption spectra of the wild-type (WT) and mutants of viral HeR from Emiliania huxleyi virus 202 (V2HeR3) obtained by the hydroxylamine bleach of Pichia pastoris cells.
Light-induced difference absorption spectra in the presence of 0.5M hydroxylamine of the WT (black line) and the mutants (red line). Mutants and WT spectra were normalized by use of a positive peak at each λmax. Blue line in WT shows the absorption spectra of the purified V2HeR3.
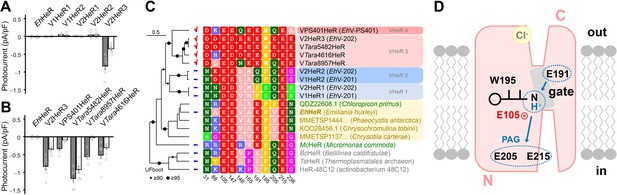
Electrophysiological measurements of related proteins of viral HeR from Emiliania huxleyi virus 202 (V2HeR3) and a functional model.
(A) Electrophysiological measurements of a heliorhodopsin (HeR) from the host Emiliania huxleyi (Ehux), two HeRs from E. huxleyi virus 201 (V1HeR1, 2) and three HeRs from E. huxleyi virus 202 (V2HeR1-3). Although some HeRs exhibit transient photocurrents (positive or negative peaks), steady-state photocurrent was only observed for V2HeR3. Comparison of photocurrent densities at −40mV. Square-block bar indicates amplitude from peak photocurrent (I1), open bar indicates amplitude from steady-state photocurrent (I2). The pipette solution was 110mM NaCli, pH 7.4i, the bath solution was 140mM NaCle, pH 7.4e. n=5–8cells. (B) Electrophysiological measurements and the obtained I-V plots of homologous proteins of V2HeR3. Comparison of photocurrent amplitudes at −40mV. Square-block bar indicates amplitude from peak photocurrent (I1), open bar indicates amplitude from steady-state photocurrent (I2). The pipette solution was 110mM NaCli, pH 7.4i, the bath solution was 140mM NaCle, pH 7.4e. n=5–8cells. (C) Key residues for ion transport of HeRs. (D) Schematic drawing of suggested proton-transporting mechanism in V2HeR3.
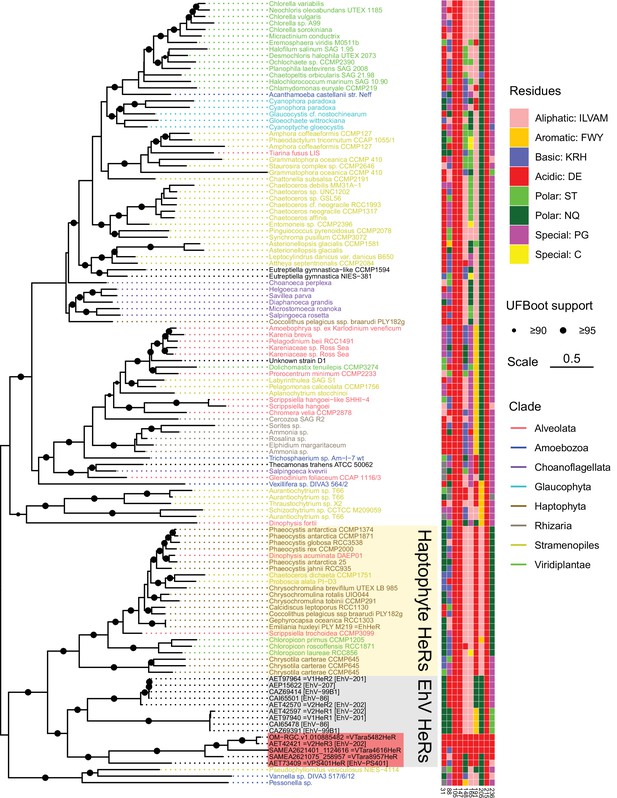
Phylogenetic relationships of the EhV heliorhodopsins (HeRs) and their eukaryotic cognates.
Eukaryotic HeRs most similar to HeRs from EhV were collected from a collection of transcriptomes and genomes of algae and other unicellular eukaryotes. Eukaryotic HeRs are named after the corresponding species/strains and colored by their taxonomic origin. The tree is midpoint-rooted which places HeRs from EhVs as a sister clade to the predominantly haptophyte HeRs, a group that also includes EhHeR from the host species, Emiliania huxleyi. Distribution of the amino acid residues among a selected set of viral HeR from Emiliania huxleyi virus 202 (V2HeR3) positions is indicated to the right. Data and code used to generate the phylogeny are available from https://github.com/BejaLab/ViralHeRs, (copy archived at swh:1:rev:0a2cc9a502c5d0d9dbc6dbcac962d89c3a5a254d, Rozenberg, 2022).
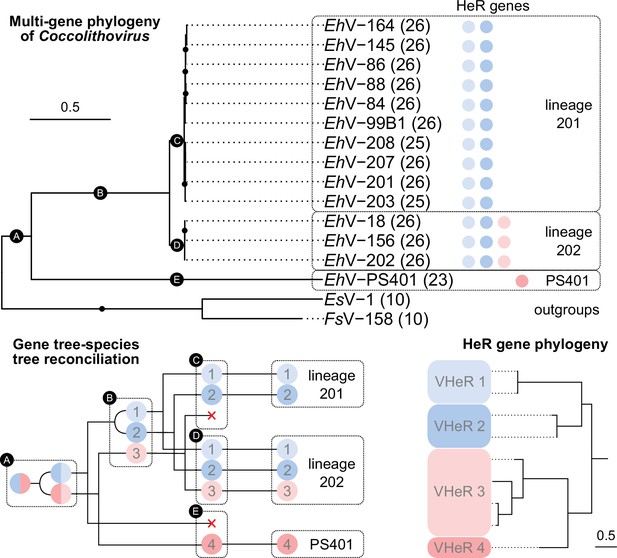
Evolution of coccolithoviruses and their heliorhodopsin (HeR) genes.
Multigene phylogeny of EhV isolates using 26 single-copy genes (number of orthogroups used per genome is indicated in parentheses) was rooted using two genomes from of phaeoviruses (top). Black dots represent highly supported branches (ultrafast bootstrap support≥95). For each EhV genome the presence of HeR genes from the four HeR clades is indicated with circles colored according to the HeR phylogeny (bottom right). The two clades showing proton transport are highlighted in red. Gene tree-species tree reconciliation providing a hypothesis about the evolution of the HeR genes among EhVs (bottom left) is based on the assumption of no horizontal transfer of HeR genes between viruses. ⊏ forks represent co-divergence, ⊂ forks represent gene duplication events, × representsgene losses. The numbers in the black circles correspond to branches on the species tree. List of phylogenetic markers, accession numbers of HeR genes, and lineage assignments are available in Supplementary file 2. Code used to generate the phylogenies is available from https://github.com/BejaLab/ViralHeRs.
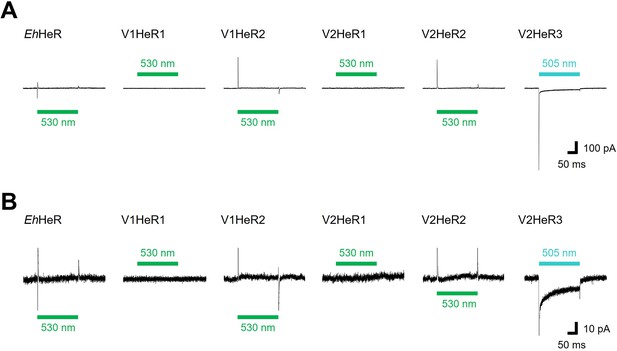
Representative photocurrent traces of heliorhodopsins (HeRs) from Emiliania huxleyi and its viruses.
(A) Electrophysiological measurements of virus HeRs-driven photocurrent in ND7/23cells. The cells were illuminated with light (λ=505nm, 24.5 mW/mm2, λ=530nm, 27.8 mW/mm2) during the time region shown by green bars. The membrane voltage was clamped at –40mV. The pipette solution was 110mM NaCli, pH 7.4i, the bath solution was 140mM NaCle, pH 7.4e. (B) The same traces as (A) with expanded Y axis.
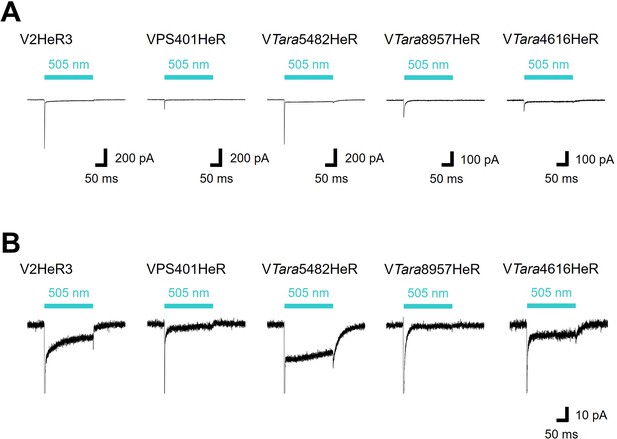
Representative photocurrent traces of viral HeR from Emiliania huxleyi virus 202 (V2HeR3)-like heliorhodopsins (HeRs).
(A) Electrophysiological measurements of V2HeR3-like HeRs-driven photocurrent in ND7/23cells. The cells were illuminated with light (λ=505nm, 24.5 mW/mm2) during the time region shown by blue bars. The membrane voltage was clamped at –40mV. The pipette solution was 110mM NaCli, pH 7.4i, the bath solution was 140mM NaCle, pH 7.4e. (B) The same traces as (A) with expanded Y axis.
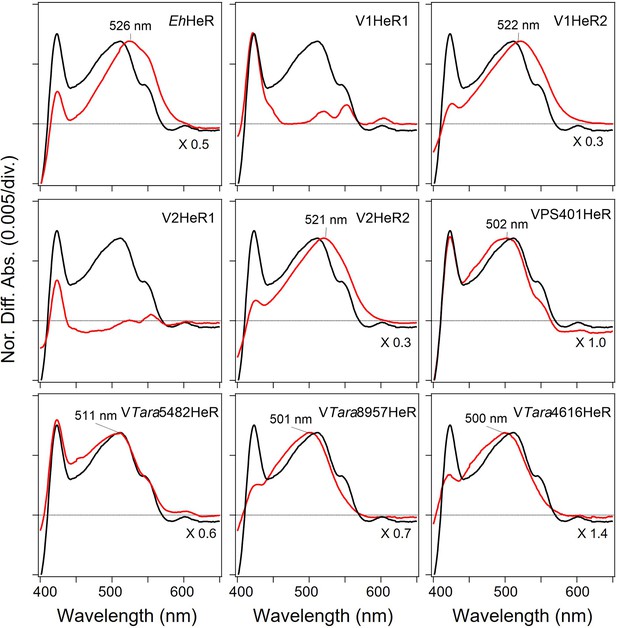
Absorption spectra of heliorhodopsins (HeRs) from Emiliania huxleyi and its viruses, and viral HeR from Emiliania huxleyi virus 202 (V2HeR3)-like HeRs obtained by the hydroxylamine bleach of ND7/23cells.
Light-induced difference absorption spectra in the presence of 50mM hydroxylamine of HeRs from E. huxleyi and its viruses, and V2HeR3-like HeRs (red line). Absorption spectra were normalized by use of a positive peak at each λmax.
Additional files
-
Supplementary file 1
GenBank flat file.
Annotated sequences of the metagenomic contigs containing VTara5482HeR, VTara8957HeR, and VTara4616HeR.
- https://cdn.elifesciences.org/articles/78416/elife-78416-supp1-v1.zip
-
Supplementary file 2
Excel spreadsheet.
List of viral genomes and phylogenetic markers used in this study. For each virus, accessions of heliorhodopsins (HeRs) genes and accessions for the indicated phylogenetic markers are provided.
- https://cdn.elifesciences.org/articles/78416/elife-78416-supp2-v1.xlsx
-
Supplementary file 3
Excel spreadsheet.
Supplementary information for methods.
- https://cdn.elifesciences.org/articles/78416/elife-78416-supp3-v1.xlsx
-
MDAR checklist
- https://cdn.elifesciences.org/articles/78416/elife-78416-mdarchecklist1-v1.docx