Regulation of inflammation and protection against invasive pneumococcal infection by the long pentraxin PTX3
Figures
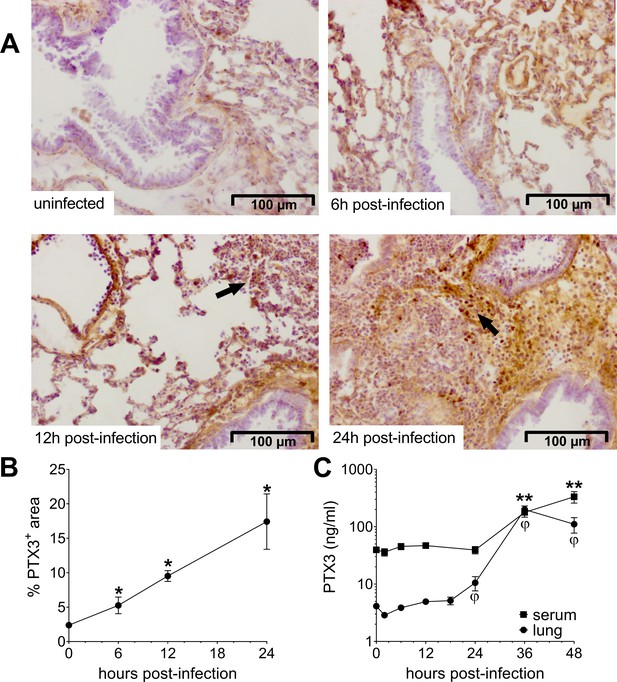
Invasive pneumococcal infection induces PTX3 expression.
Wild-type (WT) mice were infected intranasally with 5 × 104 CFU of S. pneumoniae serotype 3 and sacrificed at the indicated time points for tissue collection. (A, B) Immunohistochemical analysis and quantification of PTX3 expression in lung sections (magnification 20x) from uninfected mice and mice sacrificed 6, 12, and 24 hr post-infection (n = 3–6). (A) One representative image of at least three biological replicates for each condition is reported. Inflammatory cell infiltrates are indicated by arrows. (B) Sections were scanned and analyzed to determine the percentage of PTX3+ area at the indicated time points. (C) PTX3 protein levels determined by ELISA in serum and lung homogenates collected at the indicated time points (n = 4–10). Results are reported as mean ± standard error of the mean (SEM). Statistical significance was determined using the Mann–Whitney test comparing results to uninfected mice (φ or *p < 0.05 and **p < 0.01).
-
Figure 1—source data 1
Individual data values for the graph in Figure 1B.
- https://cdn.elifesciences.org/articles/78601/elife-78601-fig1-data1-v2.xlsx
-
Figure 1—source data 2
Individual data values for the graph in Figure 1C.
- https://cdn.elifesciences.org/articles/78601/elife-78601-fig1-data2-v2.xlsx
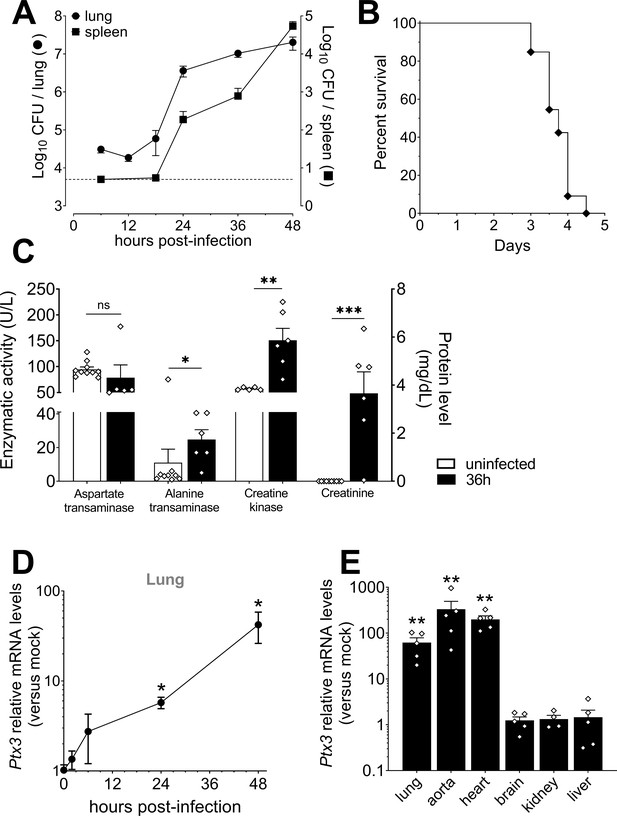
Bacterial colonization and PTX3 production after infection with S. pneumoniae serotype 3.
Mice were infected intranasally with 5 × 104 CFU of S. pneumoniae serotype 3 and sacrificed at the indicated time points for tissue collection. (A) Bacterial load in lung (●) and spleen (■) collected at indicated time points after infection of WT mice (n = 10–21). (B) Survival of WT mice infected with a lethal inoculum of S. pneumoniae serotype 3. Mice were monitored every 6 hr (n = 33). (C) Aspartate transaminase (AST), Alanine transaminase (ALT), Creatine kinase enzymatic activities, and Creatinine circulating levels were determined in the serum of infected mice. Serum from uninfected mice was also collected as control (n = 5–10). Activities of AST, ALT, and Creatine kinase are expressed as U/L while levels of Creatinine are reported as mg/dL. (D) Relative Ptx3 mRNA expression determined by real-time quantitative PCR in lung homogenates collected at the indicated time points and normalized on uninfected mice (n = 3). (E) Relative Ptx3 mRNA expression determined by real-time quantitative PCR in the indicated organ homogenates collected 48 hr post-infection and compared to uninfected mice (n = 4–5). Results are reported as mean ± SEM. Detection limit in the spleen is 5 CFU (dotted line in panel A). Statistical significance was determined using the Mann–Whitney test comparing results to uninfected mice (*p < 0.05 ; **p < 0.01; ***p < 0.001).
-
Figure 1—figure supplement 1—source data 1
Individual data values for the graph in Figure 1—figure supplement 1A.
- https://cdn.elifesciences.org/articles/78601/elife-78601-fig1-figsupp1-data1-v2.xlsx
-
Figure 1—figure supplement 1—source data 2
Individual data values for the survival graph in Figure 1—figure supplement 1B.
- https://cdn.elifesciences.org/articles/78601/elife-78601-fig1-figsupp1-data2-v2.xlsx
-
Figure 1—figure supplement 1—source data 3
Individual data values for the graph in Figure 1—figure supplement 1C.
- https://cdn.elifesciences.org/articles/78601/elife-78601-fig1-figsupp1-data3-v2.xlsx
-
Figure 1—figure supplement 1—source data 4
Individual data values for the graph in Figure 1—figure supplement 1D.
- https://cdn.elifesciences.org/articles/78601/elife-78601-fig1-figsupp1-data4-v2.xlsx
-
Figure 1—figure supplement 1—source data 5
Individual data values for the graph in Figure 1—figure supplement 1E.
- https://cdn.elifesciences.org/articles/78601/elife-78601-fig1-figsupp1-data5-v2.xlsx
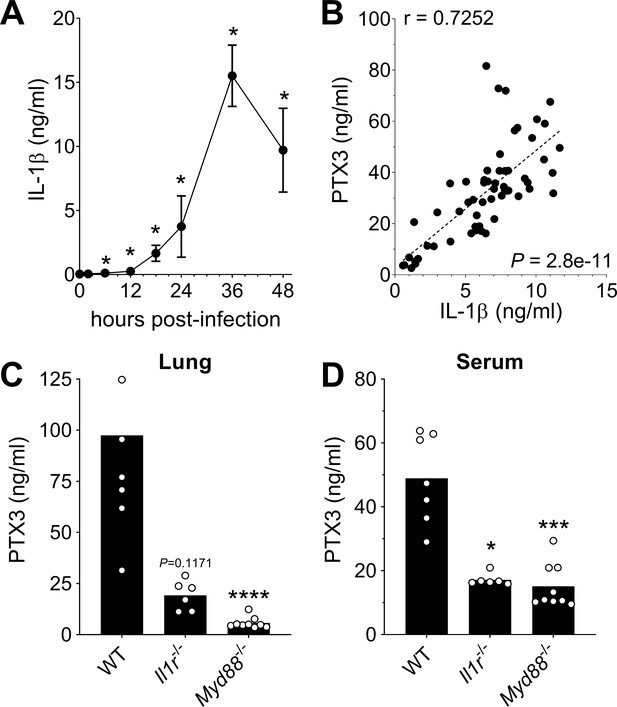
Role of IL-1β in induction of PTX3 during S. pneumoniae infection.
WT mice were infected intranasally with 5 × 104 CFU of S. pneumoniae serotype 3 and sacrificed at the indicated time points for tissue collection. (A) IL-1β protein levels in lung homogenates collected at the indicated time points determined by ELISA (n = 3–4). (B) Correlation between PTX3 and IL-1β protein levels in lung homogenates of all infected mice sacrificed from 2 to 48 hr post-infection (data pooled from five independent experiments, n = 60); Pearson correlation coefficient is reported. PTX3 protein levels determined by ELISA in lung homogenates (C) and serum (D) collected 36 hr post-infection in WT, Il1r−/− and Myd88−/− mice (n = 7–8). Results are reported as mean ± SEM. Statistical significance was determined using the Mann–Whitney test comparing results to uninfected mice (A, B) or the non-parametric Kruskal–Wallis test with post hoc corrected Dunn’s test comparing means in Il1r-/- and Myd88-/- mice to WT infected mice (C, D) (*p < 0.05, ***p < 0.001, and ****p < 0.0001).
-
Figure 2—source data 1
Individual data values for the graph in Figure 2A.
- https://cdn.elifesciences.org/articles/78601/elife-78601-fig2-data1-v2.xlsx
-
Figure 2—source data 2
Individual data values for the graph in Figure 2B.
- https://cdn.elifesciences.org/articles/78601/elife-78601-fig2-data2-v2.xlsx
-
Figure 2—source data 3
Individual data values for the graph in Figure 2C.
- https://cdn.elifesciences.org/articles/78601/elife-78601-fig2-data3-v2.xlsx
-
Figure 2—source data 4
Individual data values for the graph in Figure 2D.
- https://cdn.elifesciences.org/articles/78601/elife-78601-fig2-data4-v2.xlsx
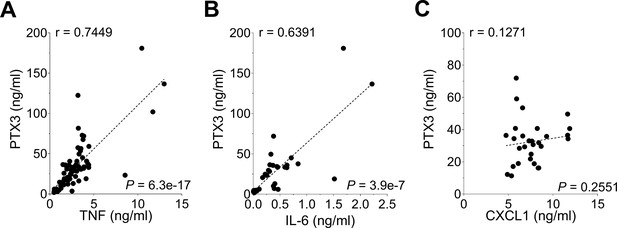
Correlation of PTX3 levels with pro-inflammatory cytokines induced during S. pneumoniae infection.
(A-C) WT mice were infected intranasally with 5 × 104 CFU of S. pneumoniae serotype 3 and sacrificed from 2 to 48 hr post-infection (data pooled from eight independent experiments, n = 29–74) for tissue collection. PTX3, TNF, IL-6, and CXCL1 protein levels in lung homogenates of WT infected mice were measured by ELISA. Pearson correlation coefficients between PTX3 and TNF (A), IL-6 (B) and CXCL1 (C) are reported in each panel.
-
Figure 2—figure supplement 1—source data 1
Individual data values for the graph in Figure 2—figure supplement 1A.
- https://cdn.elifesciences.org/articles/78601/elife-78601-fig2-figsupp1-data1-v2.xlsx
-
Figure 2—figure supplement 1—source data 2
Individual data values for the graph in Figure 2—figure supplement 1B.
- https://cdn.elifesciences.org/articles/78601/elife-78601-fig2-figsupp1-data2-v2.xlsx
-
Figure 2—figure supplement 1—source data 3
Individual data values for the graph in Figure 2—figure supplement 1C.
- https://cdn.elifesciences.org/articles/78601/elife-78601-fig2-figsupp1-data3-v2.xlsx
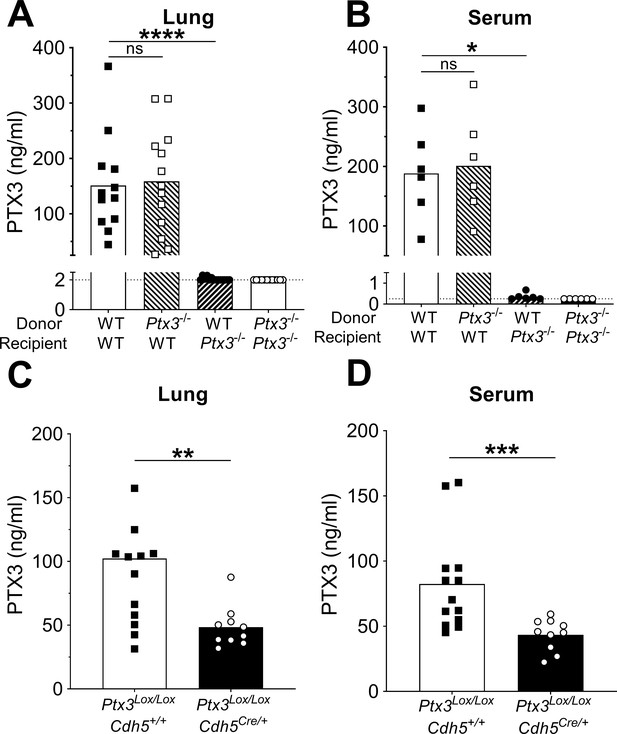
Non-hematopoietic cells are a major source of PTX3 during pneumococcal infection.
Mice were infected intranasally with 5 × 104 CFU of S. pneumoniae serotype 3 and sacrificed 36 hr post-infection for tissue collection. (A, B) PTX3 protein levels determined by ELISA in lung homogenates (n = 12–14, A) and serum (n = 6, B) from chimeric mice. Two independent experiments were performed with similar results. (C, D) PTX3 protein levels determined by ELISA in lung homogenates (C) and serum (D) collected from Ptx3Lox/LoxCdh5+/+, Ptx3Lox/LoxCdh5Cre/+ (n = 10–13). Results are reported as mean; PTX3 detection limit is 2 ng/ml in lung homogenates (A) and 0.25 ng/ml in serum (B) and is represented by a dotted line. Statistical significance was determined using the non-parametric Kruskal–Wallis test with post hoc corrected Dunn’s test comparing means to the WT recipient mice reconstituted with WT bone marrow (A, B) or the Mann–Whitney test (C, D) (*p < 0.05, **p < 0.01, ***p < 0.001, and ****p < 0.0001; ns: not significant).
-
Figure 3—source data 1
Individual data values for the graph in Figure 3A.
- https://cdn.elifesciences.org/articles/78601/elife-78601-fig3-data1-v2.xlsx
-
Figure 3—source data 2
Individual data values for the graph in Figure 3B.
- https://cdn.elifesciences.org/articles/78601/elife-78601-fig3-data2-v2.xlsx
-
Figure 3—source data 3
Individual data values for the graph in Figure 3.
- https://cdn.elifesciences.org/articles/78601/elife-78601-fig3-data3-v2.xlsx
-
Figure 3—source data 4
Individual data values for the graph in Figure 3D.
- https://cdn.elifesciences.org/articles/78601/elife-78601-fig3-data4-v2.xlsx
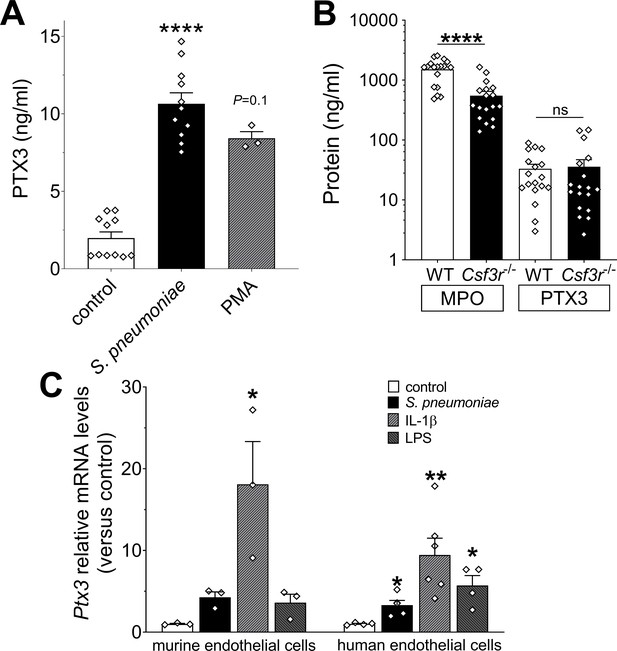
Cellular sources of PTX3 in response to stimulation with S. pneumoniae.
(A) PTX3 protein levels, measured by ELISA, released by 106 human purified neutrophils/100 µl stimulated for 6 hr at 37°C with 107 CFU/ml of S. pneumoniae serotype 3 or 10 ng/ml of phorbol myristate acetate (PMA). (B) MPO and PTX3 protein levels determined by ELISA in lung homogenates collected 36 hr post intranasal infection of WT and Csfr3−/− mice with 5 × 104 CFU of S. pneumoniae serotype 3 (data pooled from two experiments, n = 18). (C) Relative Ptx3 mRNA expression determined by real-time quantitative PCR in human and murine endothelial cells after 6 hr stimulation with 106 CFU S. pneumoniae, 20 ng/ml IL-1β or 100 ng/ml lipopolysaccharide (LPS; n = 3–6). Results are reported as mean ± SEM. Statistical significance was determined using the non-parametric Kruskal–Wallis test with post hoc corrected Dunn’s test comparing means to control group (A, C) or the Mann–Whitney test (B) (*p < 0.05, **p < 0.01, and ****p < 0.0001; ns: not significant).
-
Figure 3—figure supplement 1—source data 1
Individual data values for the graph in Figure 3—figure supplement 1A.
- https://cdn.elifesciences.org/articles/78601/elife-78601-fig3-figsupp1-data1-v2.xlsx
-
Figure 3—figure supplement 1—source data 2
Individual data values for the graph in Figure 3—figure supplement 1B.
- https://cdn.elifesciences.org/articles/78601/elife-78601-fig3-figsupp1-data2-v2.xlsx
-
Figure 3—figure supplement 1—source data 3
Individual data values for the graph in Figure 3—figure supplement 1C.
- https://cdn.elifesciences.org/articles/78601/elife-78601-fig3-figsupp1-data3-v2.xlsx
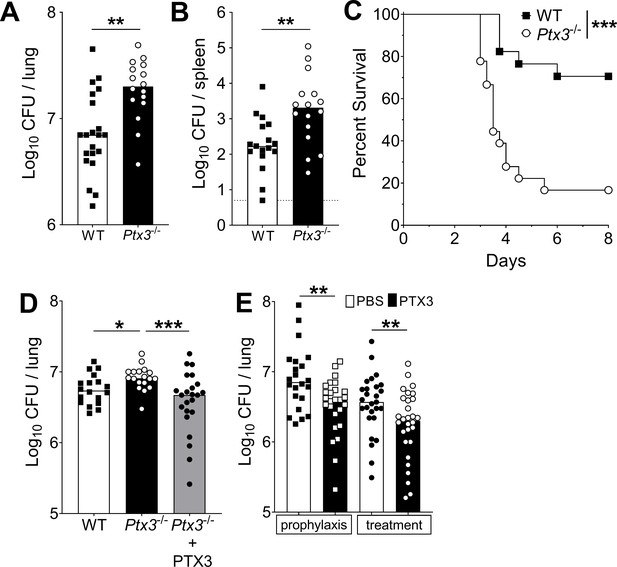
Defective resistance of Ptx3-deficient mice to invasive pneumococcal infection.
WT and Ptx3-/- mice were infected intranasally with different doses of S. pneumoniae serotype 3 and sacrificed at the indicated time points for tissue collection. Bacterial load in lung (A) and spleen (B) was analyzed at 36 hr post-infection with 5 x 104 CFU of bacteria (data pooled from two independent experiments, n = 16–21). (C) Survival of WT and Ptx3−/− mice (data pooled from two independent experiments, n = 18) was monitored every 6 hr after infection with 5 × 103 CFU. (D) Bacterial load was analyzed in lungs collected 36 hr post-infection from WT, Ptx3−/− and Ptx3−/− mice treated intraperitoneally with recombinant PTX3 (10 µg/100 µl) before the infection and 24 hr post-infection (n = 18–23). (E) Bacterial load in lungs collected 36 hr post-infection from WT mice treated intranasally before the infection (prophylaxis, data pooled from two independent experiments, n = 22–26) or 12 hr post-infection (treatment, data pooled from three independent experiments, n = 37–40) with 1 µg/30 µl of recombinant PTX3 or phosphate-buffered saline (PBS). Results are reported as median CFU. Detection limit in the spleen is 5 CFU (dotted line in panel B). Statistical significance was determined using the Mann–Whitney test (A, B, E), the non-parametric Kruskal–Wallis test with post hoc corrected Dunn’s test comparing means to the WT mice (D) and log-rank (Mantel–Cox) test for survival (C) (*p < 0.05, **p < 0.01, and ***p < 0.001).
-
Figure 4—source data 1
Individual data values for the graph in Figure 4A.
- https://cdn.elifesciences.org/articles/78601/elife-78601-fig4-data1-v2.xlsx
-
Figure 4—source data 2
Individual data values for the graph in Figure 4B.
- https://cdn.elifesciences.org/articles/78601/elife-78601-fig4-data2-v2.xlsx
-
Figure 4—source data 3
Individual data values for the survival graph in Figure 4C.
- https://cdn.elifesciences.org/articles/78601/elife-78601-fig4-data3-v2.xlsx
-
Figure 4—source data 4
Individual data values for the graph in Figure 4D.
- https://cdn.elifesciences.org/articles/78601/elife-78601-fig4-data4-v2.xlsx
-
Figure 4—source data 5
Individual data values for the graph in Figure 4E.
- https://cdn.elifesciences.org/articles/78601/elife-78601-fig4-data5-v2.xlsx
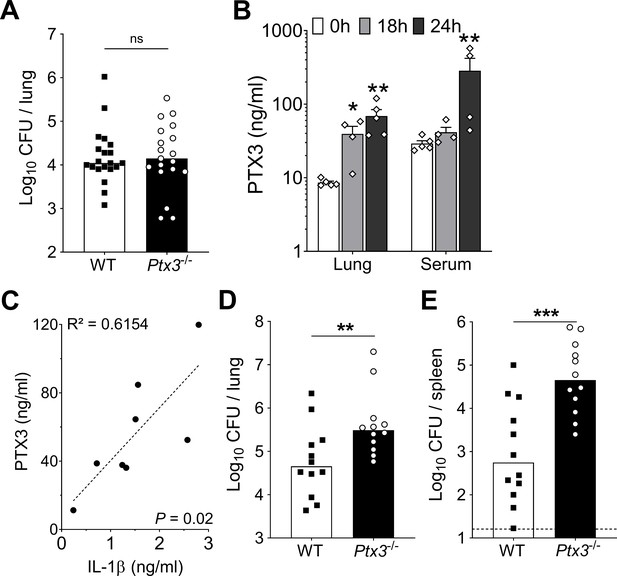
Infection with S. pneumoniae serotype 3 or 1 of WT and Ptx3-/- mice.
Mice were infected intranasally with 5 × 104 CFU of S. pneumoniae serotype 3 (A) or with 106 CFU of S. pneumoniae serotype 1 (B–E) and sacrificed at the indicated time points for tissue collection. (A) Bacterial load in lung was analyzed in WT and Ptx3−/− mice 18 hr post-infection (data pooled from two independent experiments, n = 19–20). (B) PTX3 protein levels determined by ELISA in lung homogenates and serum collected at the indicated time points (n = 4–5) from WT mice infected with S. pneumoniae serotype 1. (C) Correlation between PTX3 and IL-1β protein levels in lung homogenates of all mice sacrificed from 18 to 24 hr post-infection (n = 8). Bacterial load in lung (D) and spleen (E) collected at the indicated time points after infection of WT and Ptx3−/− mice with S. pneumoniae serotype 1 (n = 12). Results are reported as mean ± SEM (B). Detection limit in the spleen is 5 CFU (dotted line in panel E). Statistical significance was determined using the non-parametric Kruskal–Wallis test with post hoc corrected Dunn’s test comparing means to uninfected mice (B) and the Mann–Whitney test (A, D, E) (*p < 0.05, **p < 0.01, ***p < 0.001; ns: not significant).
-
Figure 4—figure supplement 1—source data 1
Individual data values for the graph in Figure 4—figure supplement 1A.
- https://cdn.elifesciences.org/articles/78601/elife-78601-fig4-figsupp1-data1-v2.xlsx
-
Figure 4—figure supplement 1—source data 2
Individual data values for the graph in Figure 4—figure supplement 1B.
- https://cdn.elifesciences.org/articles/78601/elife-78601-fig4-figsupp1-data2-v2.xlsx
-
Figure 4—figure supplement 1—source data 3
Individual data values for the graph in Figure 4—figure supplement 1C.
- https://cdn.elifesciences.org/articles/78601/elife-78601-fig4-figsupp1-data3-v2.xlsx
-
Figure 4—figure supplement 1—source data 4
Individual data values for the graph in Figure 4—figure supplement 1D.
- https://cdn.elifesciences.org/articles/78601/elife-78601-fig4-figsupp1-data4-v2.xlsx
-
Figure 4—figure supplement 1—source data 5
Individual data values for the graph in Figure 4—figure supplement 1E.
- https://cdn.elifesciences.org/articles/78601/elife-78601-fig4-figsupp1-data5-v2.xlsx
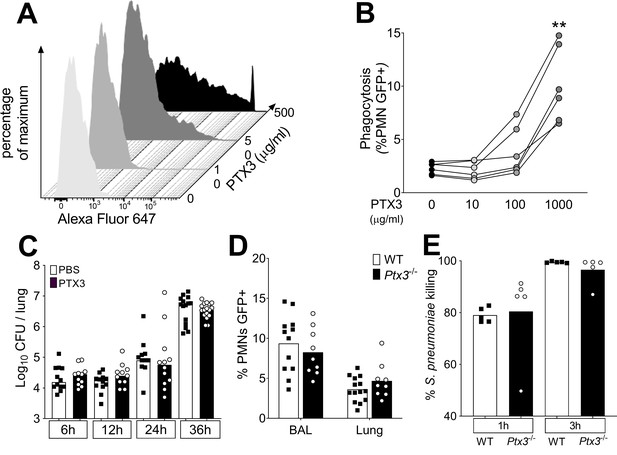
Role of phagocytosis in PTX3-mediated resistance to S. pneumoniae.
(A) Binding of biotinylated recombinant PTX3 at the indicated concentration with 106 CFU of S. pneumoniae serotype 3 was analyzed by flow cytometry after incubation with Streptavidin-Alexa Fluor 647. (B) S. pneumoniae serotype 1 expressing GFP (S pneumoniae-GFP; 106 CFU) was pre-opsonized with the indicated concentration of recombinant PTX3 and incubated 30 min with 105 purified human neutrophils from six independent donors. GFP-positive neutrophils were analyzed by flow cytometry. Results are expressed as mean of five technical replicates for each time point and donor. (C) Bacterial load in lungs collected at indicated time points from WT mice infected intranasally with S. pneumoniae serotype 3 pre-opsonized with 33 µg/ml of recombinant PTX3 or non-opsonized (data pooled from two independent experiments, n = 11–17). (D) Neutrophil phagocytosis of S. pneumoniae-GFP was analyzed by flow cytometry. BAL and lungs from WT and Ptx3−/− mice were collected 24 hr after infection with a lethal inoculum of S. pneumoniae (data pooled from two independent experiments, n = 9–14). (E) AlamarBlue-based killing assay performed with neutrophils purified from WT and Ptx3−/− mice assessed after 1 and 3 hr incubation at a MOI S. pneumoniae/neutrophils 2/1. Bars rapresent median values (C) or mean values (D, E). Statistical significance was determined using the one-way analysis of variance (ANOVA) with Sidak’s multiple comparison test (B), the non-parametric Kruskal–Wallis test with post hoc corrected Dunn’s test comparing means to the WT mice of each time point (C, E) and the Mann–Whitney test (D) (**p < 0.01).
-
Figure 5—source data 1
Individual data values for the graph in Figure 5B.
- https://cdn.elifesciences.org/articles/78601/elife-78601-fig5-data1-v2.xlsx
-
Figure 5—source data 2
Individual data values for the graph in Figure 5C.
- https://cdn.elifesciences.org/articles/78601/elife-78601-fig5-data2-v2.xlsx
-
Figure 5—source data 3
Individual data values for the graph in Figure 5D.
- https://cdn.elifesciences.org/articles/78601/elife-78601-fig5-data3-v2.xlsx
-
Figure 5—source data 4
Individual data values for the graph in Figure 5E.
- https://cdn.elifesciences.org/articles/78601/elife-78601-fig5-data4-v2.xlsx
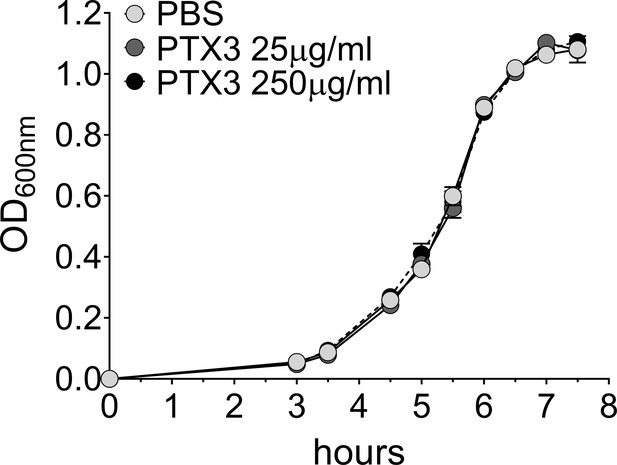
Effect of PTX3 on S. pneumoniae growth rate.
Growth rate of S. pneumoniae serotype 3 non-opsonized or pre-opsonized with recombinant PTX3 (25–250 µg/ml for 40 min) was measured in the culture condition reported in Materials and methods. Absorbance (600 nm) was measured at the indicated time points (n = 3) and is reported as mean ± SEM.
-
Figure 5—figure supplement 1—source data 1
Individual data values for the graph in Figure 5—figure supplement 1.
- https://cdn.elifesciences.org/articles/78601/elife-78601-fig5-figsupp1-data1-v2.xlsx
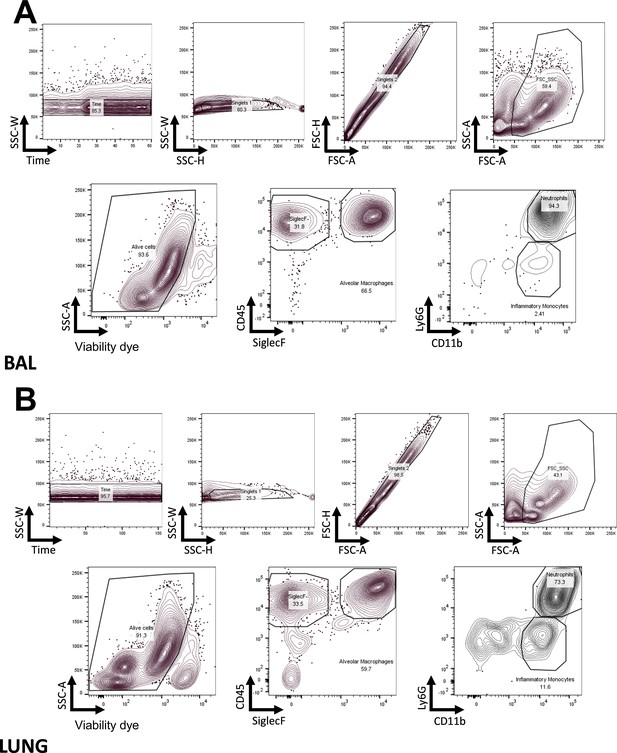
FACS gating strategy.
Gating strategy to identify myeloid subsets in the BAL (A) and in the lung (B), with the antibody panels described in Materials and methods.
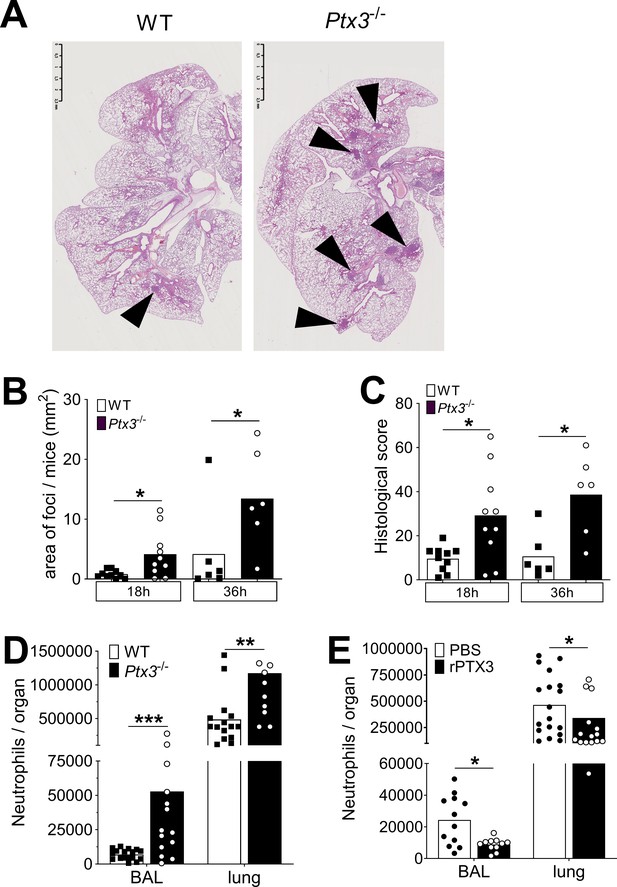
PTX3 regulates inflammation during pneumococcus infection.
Mice were infected intranasally with 5 × 104 CFU of S. pneumoniae serotype 3 and sacrificed at the indicated time points for tissue collection. (A) Hematoxylin and eosin (H&E) staining of formalin-fixed histological sections from the lungs of WT and Ptx3−/− mice at 4x magnification. One representative image from at least six biological replicates of WT and Ptx3−/− mice. Inflammatory cell foci are indicated by arrows. (B) Area of inflammatory cells foci measured in lungs collected 18 and 36 hr post-infection from WT and Ptx3−/− mice. Areas were measured on three H&E stained lung sections per mice at different depth separated by at least 100 µm each (n = 6–10). (C) Inflammatory histological score measured in lungs collected 18 and 36 hr post-infection from WT and Ptx3−/− mice. Scores (detailed in Materials and methods) were determined on three H&E stained lung sections per mice at different depth separated by at least 100 µm each (n = 6–10). (D) Neutrophil number determined by flow cytometry in BAL and lungs collected 18 hr post-infection from WT and Ptx3−/− mice (data pooled from two independent experiments, n = 11–18). (E) Neutrophil number determined by flow cytometry in the BAL and lung collected 18 hr post-infection from WT mice treated intranasally 12 hr post-infection with recombinant PTX3 or PBS (data pooled from two independent experiments, n = 11–18). Bars rapresent mean values. Statistical significance was determined using the Mann–Whitney test comparing results to uninfected mice (*p < 0.05, **p<0.01 and ***p < 0.001).
-
Figure 6—source data 1
Individual data values for the graph in Figure 6B.
- https://cdn.elifesciences.org/articles/78601/elife-78601-fig6-data1-v2.xlsx
-
Figure 6—source data 2
Individual data values for the graph in Figure 6C.
- https://cdn.elifesciences.org/articles/78601/elife-78601-fig6-data2-v2.xlsx
-
Figure 6—source data 3
Individual data values for the graph in Figure 6D.
- https://cdn.elifesciences.org/articles/78601/elife-78601-fig6-data3-v2.xlsx
-
Figure 6—source data 4
Individual data values for the graph in Figure 6E.
- https://cdn.elifesciences.org/articles/78601/elife-78601-fig6-data4-v2.xlsx
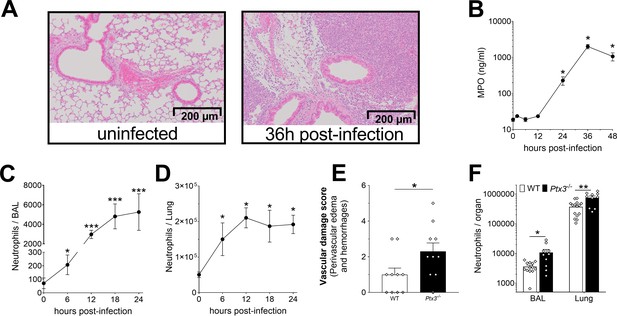
Neutrophil recruitment during invasive pneumococcus infection.
Mice were infected intranasally with 5 × 104 CFU of S. pneumoniae serotype 3 (A–E) or with 106 CFU of serotype 1 (F) and sacrificed at the indicated time points for tissue collection. (A) Hematoxylin and eosin (H&E) staining of formalin-fixed lung sections from WT mice uninfected and 36 hr after infection at 10 x magnification. (B) MPO levels determined by ELISA in lung homogenates collected at the indicated time points (n = 4). Neutrophil number determined by flow cytometry in BAL (C) and lung (D) collected at the indicated time points from WT mice (n = 4–8). (E) Vascular damage histological score measured in lungs collected 18 hr post-infection from WT and Ptx3−/− mice. Scores (detailed in Materials and methods) were determined on three H&E stained lung sections per mice at different depth separated by at least 100 µm each (n = 6–10). (F) Neutrophil number determined by flow cytometry in the BAL and lung collected 18 hr post-infection from WT and Ptx3−/− mice (data pooled from two independent experiments, n = 10–13). Results are reported as mean ± SEM. Statistical significance was determined using the Mann–Whitney test comparing results to uninfected mice (*p < 0.05, **p < 0.01, and ***p < 0.001).
-
Figure 6—figure supplement 1—source data 1
Individual data values for the graph in Figure 6—figure supplement 1B.
- https://cdn.elifesciences.org/articles/78601/elife-78601-fig6-figsupp1-data1-v2.xlsx
-
Figure 6—figure supplement 1—source data 2
Individual data values for the graph in Figure 6—figure supplement 1C.
- https://cdn.elifesciences.org/articles/78601/elife-78601-fig6-figsupp1-data2-v2.xlsx
-
Figure 6—figure supplement 1—source data 3
Individual data values for the graph in Figure 6—figure supplement 1D.
- https://cdn.elifesciences.org/articles/78601/elife-78601-fig6-figsupp1-data3-v2.xlsx
-
Figure 6—figure supplement 1—source data 4
Individual data values for the graph in Figure 6—figure supplement 1E.
- https://cdn.elifesciences.org/articles/78601/elife-78601-fig6-figsupp1-data4-v2.xlsx
-
Figure 6—figure supplement 1—source data 5
Individual data values for the graph in Figure 6—figure supplement 1F.
- https://cdn.elifesciences.org/articles/78601/elife-78601-fig6-figsupp1-data5-v2.xlsx
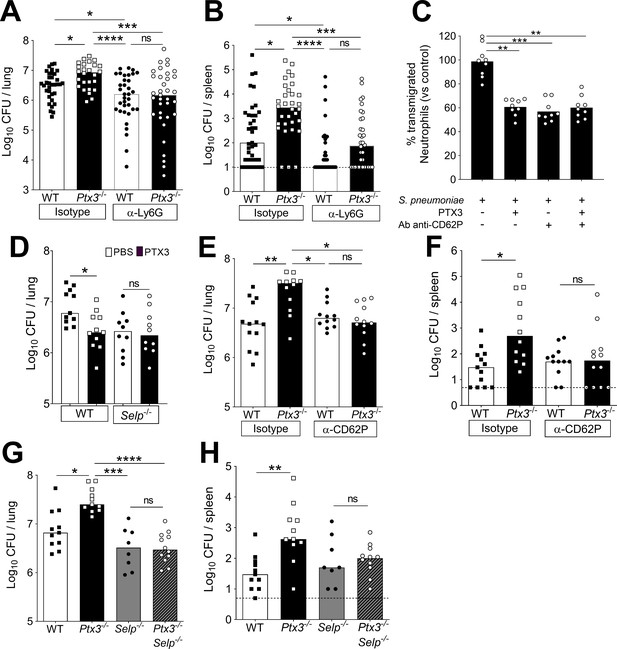
Involvement of P-selectin in PTX3-mediated regulation of neutrophil recruitment.
Mice were infected intranasally with 5 × 104 CFU of S. pneumoniae serotype 3 and sacrificed at the indicated time points for tissue collection. Bacterial load in lung (A) and spleen (B) collected 36 hr post-infection from WT and Ptx3−/− mice treated intraperitoneally 12 hr post-infection with 200 µg/100 µl of anti-Ly6G or isotype control antibodies (data pooled from three independent experiments, n = 26–37). (C) Transmigration of human purified neutrophils toward S. pneumoniae (data pooled from two independent experiments, n = 11). Results are reported as percentage of transmigrated neutrophils considering as 100% the number of transmigrated neutrophils in the control condition (i.e. S. pneumoniae in the lower chamber and no treatment in the upper chamber). (D) Bacterial load in lungs collected 36 hr post-infection from WT and Selp−/− mice treated intranasally 12 hr post-infection with 1 µg/30 µl of recombinant PTX3 or phosphate-buffered saline (PBS) (data pooled from two independent experiments, n = 10–11). Bacterial load in lungs (E) and spleens (F) collected 36 hr post-infection from WT and Ptx3−/− mice treated intravenously 12 hr post-infection with 50 µg/100 µl of anti-CD62P or isotype control antibodies (data pooled from two independent experiments, n = 12–13). Bacterial load in lungs (G) and spleens (H) collected 36 hr post-infection from WT, Ptx3−/−, Selp−/−, and Ptx3−/−Selp−/− mice (data pooled from two independent experiments, n = 8–12). Results are reported as median (A, B, D–H) and mean. Detection limits in the spleen is 5 CFU (dotted line in panels B, F, H). Statistical significance was determined using the non-parametric Kruskal–Wallis test with post hoc corrected Dunn’s test comparing every means (A–H) (*p < 0.05, **p < 0.01, ***p < 0.001, and ****p < 0.0001; ns: not significant).
-
Figure 7—source data 1
Individual data values for the graph in Figure 7A.
- https://cdn.elifesciences.org/articles/78601/elife-78601-fig7-data1-v2.xlsx
-
Figure 7—source data 2
Individual data values for the graph in Figure 7B.
- https://cdn.elifesciences.org/articles/78601/elife-78601-fig7-data2-v2.xlsx
-
Figure 7—source data 3
Individual data values for the graph in Figure 7C.
- https://cdn.elifesciences.org/articles/78601/elife-78601-fig7-data3-v2.xlsx
-
Figure 7—source data 4
Individual data values for the graph in Figure 7D.
- https://cdn.elifesciences.org/articles/78601/elife-78601-fig7-data4-v2.xlsx
-
Figure 7—source data 5
Individual data values for the graph in Figure 7E.
- https://cdn.elifesciences.org/articles/78601/elife-78601-fig7-data5-v2.xlsx
-
Figure 7—source data 6
Individual data values for the graph in Figure 7F.
- https://cdn.elifesciences.org/articles/78601/elife-78601-fig7-data6-v2.xlsx
-
Figure 7—source data 7
Individual data values for the graph in Figure 7G.
- https://cdn.elifesciences.org/articles/78601/elife-78601-fig7-data7-v2.xlsx
-
Figure 7—source data 8
Individual data values for the graph in Figure 7H.
- https://cdn.elifesciences.org/articles/78601/elife-78601-fig7-data8-v2.xlsx
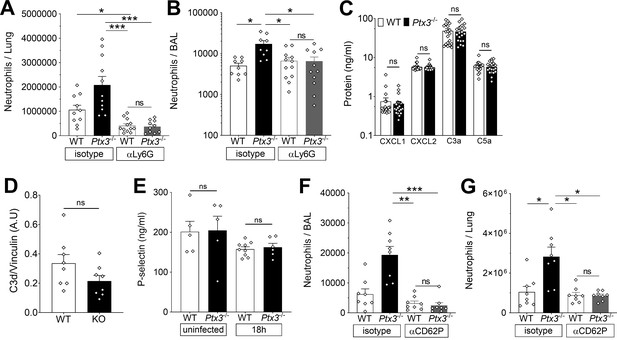
PTX3 modulates neutrophil recruitment in lungs of S. pneumoniae infected mice.
Mice were infected intranasally with 5 × 104 CFU of S. pneumoniae serotype 3 and sacrificed at the indicated time points for tissue collection. Neutrophil number was determined by flow cytometry in the lung (A) and BAL (B) collected 18 hr post-infection from WT and Ptx3−/− mice treated intraperitoneally 12 hr post-infection with 200 µg/100 µl of anti-Ly6G or isotype control antibodies (data pooled from two independent experiments, n = 9–12). (C) Chemokines (CXCL1/CXCL2) and anaphylatoxins (C3a/C5a) levels measured by ELISA in lung homogenates collected 18 hr post-infection from WT and Ptx3−/− mice (data pooled from two independent experiments, n = 20). (D) C3d level in lung homogenates collected 36 hr post-infection from WT and Ptx3−/− (KO) mice, detected by western blot and normalized with vinculin expression (n = 8). (E) P-selectin levels measured by ELISA in lung homogenates collected from uninfected or S pneumoniae infected mice sacrificed 18 hr post-infection (n = 5–9). Neutrophil number determined by flow cytometry in the BAL (F) and lung (G) collected 18 hr post-infection from WT and Ptx3−/− mice treated intraperitoneally 12 hr post-infection with 50 µg/100 µl of anti-CD62P or isotype control antibodies (n = 8). Results are reported as mean ± SEM. Statistical significance was determined using the non-parametric Kruskal–Wallis test with post hoc corrected Dunn’s test comparing means to WT mice treated with isotype antibody (A, B, E–G) and the Mann–Whitney test (C, D) (*p < 0.05, **p < 0.01, and ***p < 0.001; ns: not significant).
-
Figure 7—figure supplement 1—source data 1
Individual data values for the graph in Figure 7—figure supplement 1A.
- https://cdn.elifesciences.org/articles/78601/elife-78601-fig7-figsupp1-data1-v2.xlsx
-
Figure 7—figure supplement 1—source data 2
Individual data values for the graph in Figure 7—figure supplement 1B.
- https://cdn.elifesciences.org/articles/78601/elife-78601-fig7-figsupp1-data2-v2.xlsx
-
Figure 7—figure supplement 1—source data 3
Individual data values for the graph in Figure 7—figure supplement 1C.
- https://cdn.elifesciences.org/articles/78601/elife-78601-fig7-figsupp1-data3-v2.xlsx
-
Figure 7—figure supplement 1—source data 4
Individual data values for the graph in Figure 7—figure supplement 1D.
- https://cdn.elifesciences.org/articles/78601/elife-78601-fig7-figsupp1-data4-v2.xlsx
-
Figure 7—figure supplement 1—source data 5
Individual data values for the graph in Figure 7—figure supplement 1E.
- https://cdn.elifesciences.org/articles/78601/elife-78601-fig7-figsupp1-data5-v2.xlsx
-
Figure 7—figure supplement 1—source data 6
Individual data values for the graph in Figure 7—figure supplement 1F.
- https://cdn.elifesciences.org/articles/78601/elife-78601-fig7-figsupp1-data6-v2.xlsx
-
Figure 7—figure supplement 1—source data 7
Individual data values for the graph in Figure 7—figure supplement 1G.
- https://cdn.elifesciences.org/articles/78601/elife-78601-fig7-figsupp1-data7-v2.xlsx
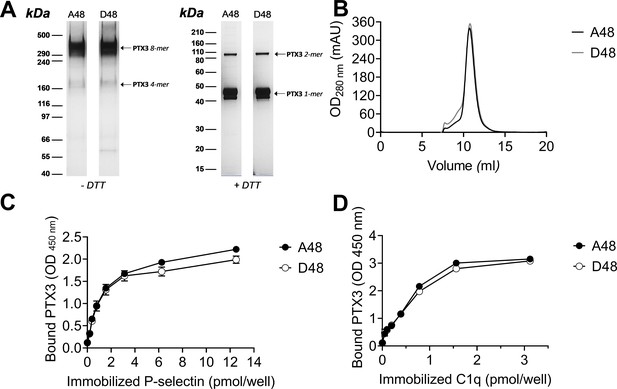
Biochemical characterization of the D48 and A48 allelic variants of PTX3 and their binding to P-selectin. (A) 500 ng/lane of purified recombinant PTX3 (A48 and D48 from HEK293 cells) were run under denaturing conditions on Tris-Acetate 3–8% (wt/vol) and Bis-Tris 10% (wt/vol) protein gels, in the absence (−) and presence (+), respectively, of dithiothreitol (DTT). Gels are shown with molecular weight markers on the left, and position of the PTX3 monomers, dimers, tetramers, and octamers (1-, 2-, 4-, and 8-mers, respectively) on the right. (B) 200 µg aliquots of either one of the two allelic variants were separated on a Superose 6 10/300 GL size exclusion chromatography column in non-denaturing conditions, with elution monitoring by UV absorbance at 280 nm. An overlay of individual chromatograms is presented. (C, D) The effect of the +734A/C polymorphism on the interaction of PTX3 with P-selectin was investigated by a solid phase binding assay using microtiter plates coated with the indicated amounts of P-selectin or C1q (here, used as a control) that were incubated with either of the A48 and D48 variants (both at 3 nM). Bound proteins were revealed with an anti-human PTX3 polyclonal antibody, and results are expressed as optical density at 450 nm (OD 450 nm), following background subtraction (n = 3, mean ± standard deviation [SD]). Data shown in panels A to D are representative of three independent experiments with similar results.
-
Figure 8—source data 1
Raw images of blot in Figure 8A.
- https://cdn.elifesciences.org/articles/78601/elife-78601-fig8-data1-v2.zip
-
Figure 8—source data 2
Individual data values for the graph in Figure 8B.
- https://cdn.elifesciences.org/articles/78601/elife-78601-fig8-data2-v2.xlsx
-
Figure 8—source data 3
Individual data values for the graph in Figure 8C.
- https://cdn.elifesciences.org/articles/78601/elife-78601-fig8-data3-v2.xlsx
-
Figure 8—source data 4
Individual data values for the graph in Figure 8D.
- https://cdn.elifesciences.org/articles/78601/elife-78601-fig8-data4-v2.xlsx
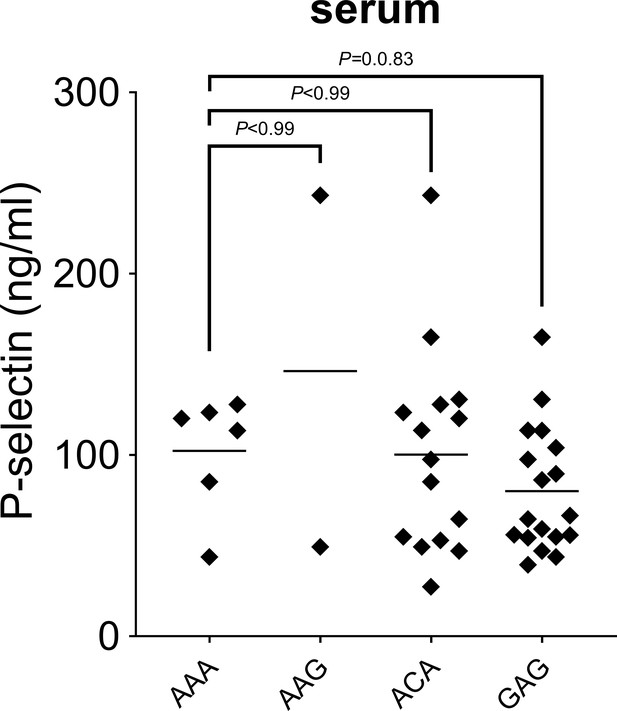
P-selectin levels in sera from patients with invasive pulmonary disease (IPD).
P-selectin levels were measured by ELISA in serum obtained from patients with IPD. Statistical significance was determined using the non-parametric Kruskal–Wallis test with post hoc corrected Dunn’s test comparing P-selectin levels in AAG, ACA, and GAG haplotype carrying patients with those measured in subjects with AAA haplotype.
-
Figure 8—figure supplement 1—source data 1
Individual data values for the graph in Figure 8—figure supplement 1.
- https://cdn.elifesciences.org/articles/78601/elife-78601-fig8-figsupp1-data1-v2.xlsx
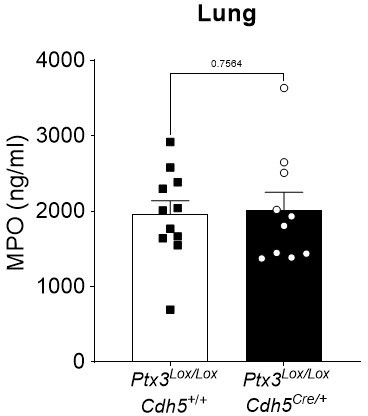
Ptx3Lox/LoxCdh5+/+ and Ptx3Lox/LoxCdh5Cre/- mice were infected intranasally with 5x104 CFU of S.
pneumoniae serotype 3 and sacrificed at 36h post-infection for lung collection. MPO levels were determined by ELISA in lung homogenates. Statistical significance was determined using the non-parametric Mann-Whitney test.
Tables
Frequency distribution of PTX3 gene single-nucleotide polymorphisms (SNPs) in invasive pulmonary disease (IPD) patients and controls.
SNP | Alleles | Amino acid change | Associated allele | Frequency (%) | χ2 | OR (95%) | p value | |
---|---|---|---|---|---|---|---|---|
IPD patient(n = 57) | Control (n = 521) | |||||||
rs2305619 | +281A/G | A | 43.86 | 43.44 | 0.007 | 1.02 (0.69–1.50) | 0.931 | |
G | 56.14 | 56.56 | ||||||
rs3816527 | +734C/A | Ala→Asp | C | 32.46 | 38.42 | 1.552 | 0.77 (0.51–1.16) | 0.213 |
A | 67.54 | 61.58 | ||||||
rs1840680 | +1149A/G | A | 42.11 | 42.49 | 0.006 | 0.98 (0.67–1.46) | 0.938 | |
G | 57.89 | 57.51 |
Haplotype analysis for PTX3 gene in invasive pulmonary disease (IPD) patients and controls.
rs2305619 | rs3816527 | rs1840680 | Frequency (%) | χ2 | p value* | |
---|---|---|---|---|---|---|
IPD patients(n = 57) | Controls (n = 521) | |||||
G | A | G | 56.15 | 56.51 | 0.005 | 0.9409 |
G | A | 56.14 | 56.59 | 0.008 | 0.9269 | |
A | G | 57.89 | 57.41 | 0.010 | 0.9202 | |
A | C | A | 32.44 | 38.28 | 1.488 | 0.2226 |
A | C | 32.46 | 38.47 | 1.577 | 0.2091 | |
C | A | 32.46 | 38.33 | 1.51 | 0.2191 | |
A | A | A | 9.67 | 4.26 | 6.604 | 0.0102 |
A | A | 11.4 | 4.94 | 8.129 | 0.0044 | |
A | A | 9.65 | 4.26 | 6.533 | 0.0106 | |
A | A | G | 1.73 | 0.95 | 0.610 | 0.4348 |
-
*
values in bold are statistically significant
Invasive pulmonary disease (IPD) severity developed by patients and association with PTX3 haplotypes.
AAA | ACA | GAG | AAG | |
---|---|---|---|---|
Bacteremia | 7/9 (77.78%) | 17/27 (62.96%) | 27/43 (65.91%) | 1/1 (100%) |
Sepsis | 5/9 (55.56%) | 13/27 (48.15%) | 17/42 (37.21%) | 1/1 (100%) |
Septic shock | 0/7 (0%) | 2/23 (8.7%) | 1/37 (2.70%) | 0/1 (0%) |
Intensive care | 1/8 (12.5%) | 7/25 (28%) | 7/36 (18.42%) | 0/1 (0%) |