Impaired iron recycling from erythrocytes is an early hallmark of aging
Figures
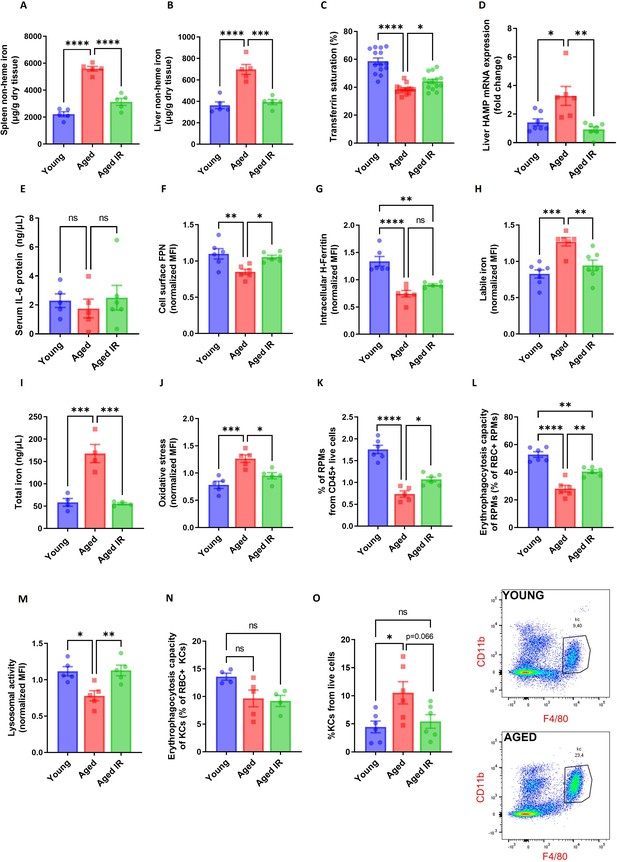
Iron-reduced diet normalizes body iron parameters during aging, diminishes iron retention in RPMs, and prevents oxidative stress.
(A) Splenic and (B) liver non-heme iron content was determined in young, aged, and aged IR mice. (C) Plasma transferrin saturation was determined in young, aged, and aged IR mice. (D) Relative mRNA expression of hepcidin (Hamp) in the liver of young, aged, and aged IR mice was determined by qPCR. (E) Serum IL-6 protein levels in young, aged, and aged IR mice were measured by Mouse IL-6 Quantikine ELISA Kit. (F) Expression of ferroportin (FPN) on the cell membrane of young, aged, and aged IR RPMs was assessed by flow cytometry. (G) Intracellular H-Ferritin protein levels in young, aged, and aged IR RPMs were quantified by flow cytometry. (H) Cytosolic ferrous iron (Fe2+) levels in young, aged, and aged IR RPMs were measured using FerroOrange with flow cytometry. (I) The total intracellular iron content in young, aged, and aged IR magnetically-sorted RPMs was assessed using the Iron Assay Kit. (J) The cytosolic ROS levels in young, aged, and aged IR RPMs were assessed by determining CellROX Deep Red fluorescence intensity with flow cytometry. (K) The percentage of RPMs from CD45 + live cells present in the spleen of young, aged, and aged IR mice was assessed by flow cytometry. (L) Erythrophagocytosis capacity in young, aged, and aged IR RPMs was determined using flow cytometry by measuring the percentage of RPMs that phagocytosed transfused PKH67-labeled temperature-stressed RBCs. (M) Lysosomal activity of young, aged, and aged IR RPMs was determined using Lysosomal Intracellular Activity Assay Kit with flow cytometry. (N) Erythrophagocytosis capacity in young, aged, and aged IR Kupffer cells (KCs) was determined using flow cytometry by measuring the percentage of KCs that phagocytosed transfused PKH67-labeled temperature-stressed RBCs. (O) Percentages of KCs in total live cells in the livers of young, aged, and aged IR mice and representative flow cytometry plots of KCs. Each dot represents one mouse. Data are represented as mean ± SEM. Statistical significance among the three groups was determined by One-Way ANOVA test with Tukey’s Multiple Comparison test. ns p>0.05, *p<0.05, **p<0.01, ***p<0.001 and ****p<0.0001.
-
Figure 1—source data 1
Related to Figure 1A–O.
- https://cdn.elifesciences.org/articles/79196/elife-79196-fig1-data1-v2.xlsx
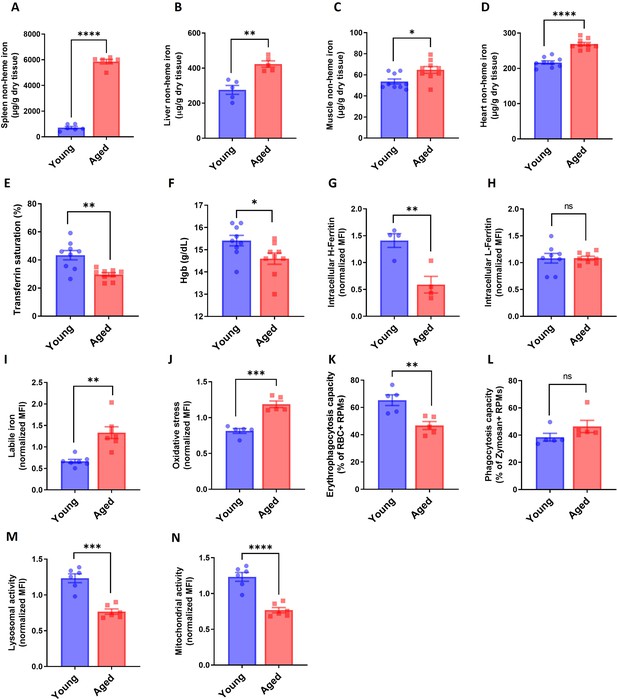
RPMs of aged mice show increased labile iron levels, oxidative stress and diminished iron-recycling functions.
(A–D) Spleen, muscle and heart non-heme iron content was determined in young and aged mice. (E) Plasma transferrin saturation and (F) blood hemoglobin (Hgb) concention were determined in young and aged mice. (G) Intracellular H-Ferritin and (H) L-Ferritin expression levels were quantified in young and aged RPMs with flow cytometry. (I) Cytosolic ferrous iron (Fe2+) and (J) ROS levels in young and aged RPMs were quantified using the FerroOrange and CellROX Deep Red probe, respectively, with flow cytometry. (K) The phagocytosis capacity for PKH67-labeled temperature-stressed RBCs and (L) zymosan A fluorescent particles by young and aged RPMs were analyzed ex vivo by flow cytometry. The percentage of cargo-positive RPMs is indicated. (M) Lysosomal activity in young and aged RPMs was determined using Lysosomal Intracellular Activity Assay Kit with flow cytometry. (N) Mitochondrial activity in young and aged RPMs was determined using the TMRE probe with flow cytometry. Each dot represents one mouse. Data are represented as mean ± SEM. Welch’s unpaired t-test determined statistical significance between the two groups. ns p>0.05, *p<0.05, **p<0.01, ***p<0.001 and ****p<0.0001.
-
Figure 1—figure supplement 1—source data 1
Related to Figure 1—figure supplement 1A–N.
- https://cdn.elifesciences.org/articles/79196/elife-79196-fig1-figsupp1-data1-v2.xlsx
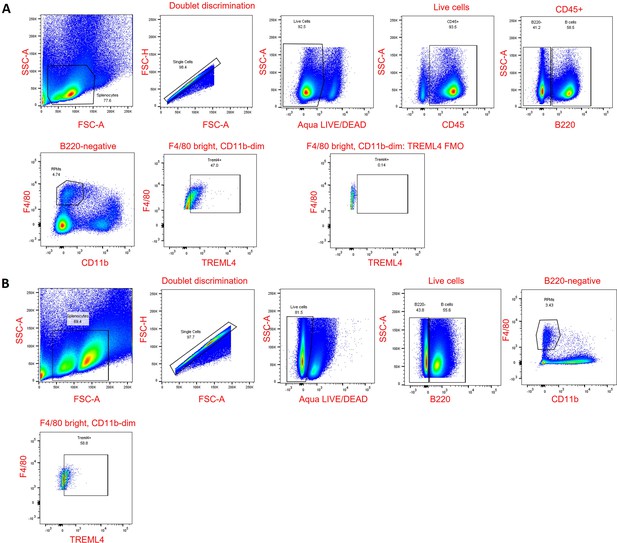
Gating strategy for RPMs.
(A) Non-permeabilized cells are shown, including an FMO control. (B) Permeabilized cells are shown.
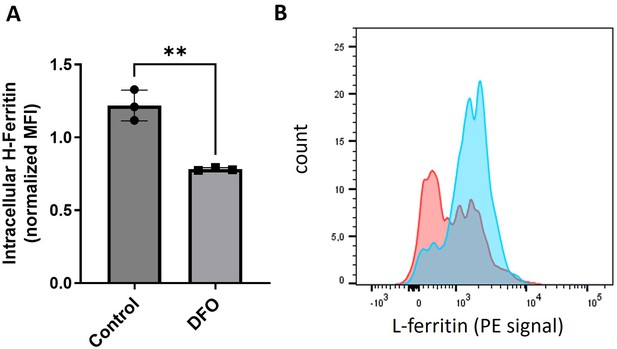
Validation of the antibodies against H and L ferritin.
(A) Shown is the intracellular staining and flow cytometric analysis of H-ferritin in bone marrow-derived macrophages treated with an iron chelator DFO for 18 hr. An expected drop in fluorescence intensity was observed. (B) Shown is the intracellular staining and flow cytometric analysis of L-ferritin in endothelial cells treated with Holo-ferritin for 9 hr (blue) compared to untreated cells (red). The uptake of ferritin, which was independently confirmed with other methods, is observed. Each dot represents one independent cell-based experiment. Data are represented as mean ± SEM. Welch’s unpaired t-test determined statistical significance between the two groups. **p<0.01.
-
Figure 1—figure supplement 3—source data 1
Related to Figure 1—figure supplement 3A.
- https://cdn.elifesciences.org/articles/79196/elife-79196-fig1-figsupp3-data1-v2.xlsx
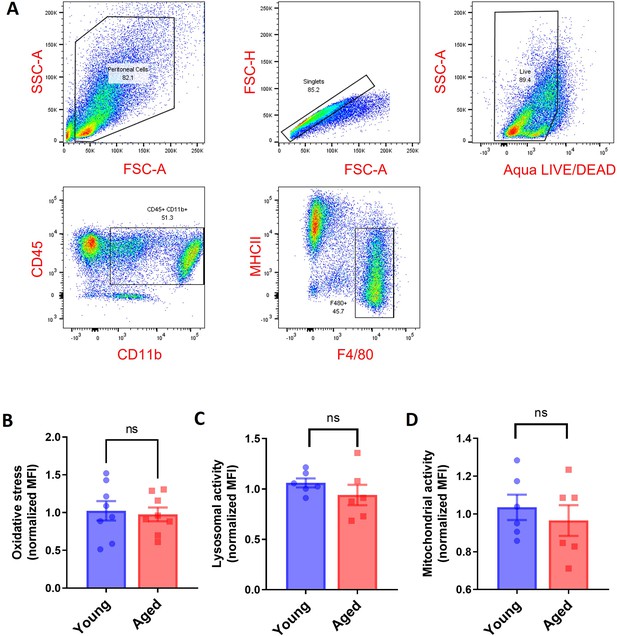
Peritoneal macrophages in aged mice do not show functional impairments.
(A) Gating strategy for peritoneal macrophages. (B) The cytosolic ROS levels in young and aged peritoneal macrophages were quantified using CellRox Deep Red with flow cytometry. (C) Lysosomal activity in young and aged peritoneal macrophages was determined using Lysosomal Intracellular Activity Assay Kit with flow cytometry. (D) Mitochondrial activity in young and aged peritoneal macrophages was determined using the TMRE probe with flow cytometry. Each dot represents one mouse. Data are represented as mean ± SEM. Welch’s unpaired t-test determined the statistical significance between the two groups. ns p>0.05.
-
Figure 1—figure supplement 4—source data 1
Related to Figure 1—figure supplement 4B–D.
- https://cdn.elifesciences.org/articles/79196/elife-79196-fig1-figsupp4-data1-v2.xlsx
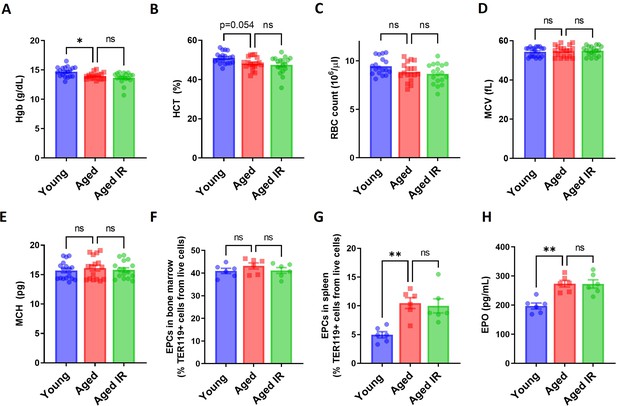
Hematological parameters and erythropoietic activity in aging mice.
(A) Blood hemoglobin (Hgb) concentration, (B) hematocrit (HCT), (C) RBC counts, (D) mean corpuscular volume (MCV) and (E) mean corpuscular hemoglobin (MCH) were determined in young, aged, and aged IR mice. (F) Shown is the percentage of erythroid progenitor cells (EPCs, TER119+ CD71+) present in the bone marrow and (G) the spleen of young, aged, and aged IR mice. (H) EPO concentration in the plasma of young, aged, and aged IR mice was measured by Mouse Erythropoietin/EPO Quantikine ELISA Kit. Each dot represents one mouse. Data are represented as mean ± SEM. Statistical significance among the three groups was determined by the One-Way ANOVA test with Tukey’s Multiple Comparison test. ns p>0.05, *p<0.05, **p<0.01.
-
Figure 1—figure supplement 5—source data 1
Related to Figure 1—figure supplement 5A–H.
- https://cdn.elifesciences.org/articles/79196/elife-79196-fig1-figsupp5-data1-v2.xlsx
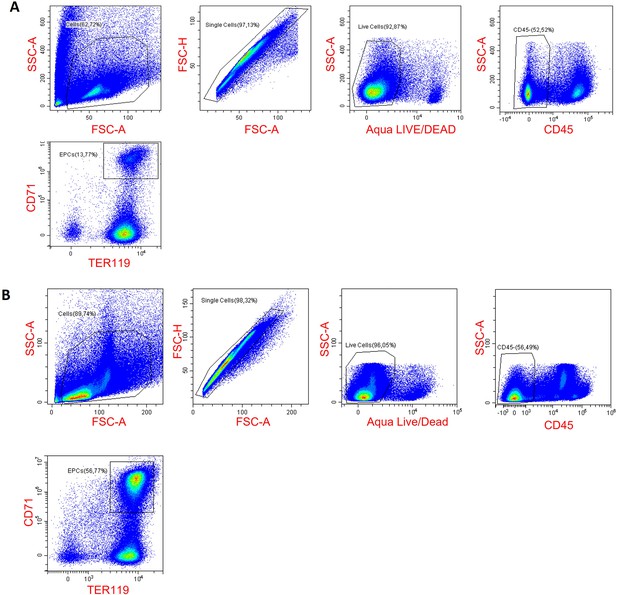
Gating strategy for erythroid progenitor cells in the spleen (A) and the bone marrow (B).
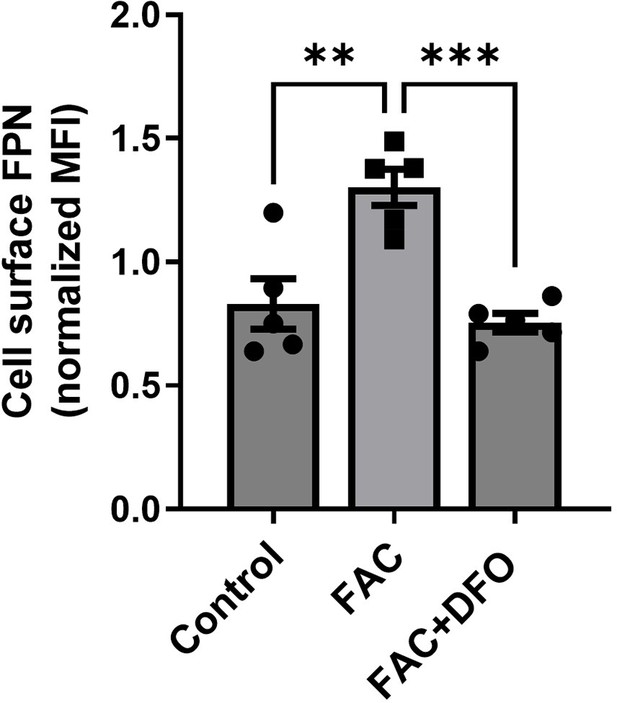
Validation of the ferroportin antibody for flow cytometry.
Shown is the cell membrane staining and flow cytometric analysis of ferroportin (FPN) in bone marrow-derived macrophages treated with ferric ammonium citrate (FAC, 50 µM, 24 hr) an iron chelator DFO (100 µM, 18 hr). Expected changes in fluorescence intensity were observed. Each dot represents one mouse. Data are represented as mean ± SEM. Statistical significance among the three groups was determined by the One-Way ANOVA test with Tukey’s Multiple Comparison test. **p<0.01 and ***p<0.001.
-
Figure 1—figure supplement 7—source data 1
Related to Figure 1—figure supplement 7.
- https://cdn.elifesciences.org/articles/79196/elife-79196-fig1-figsupp7-data1-v2.xlsx
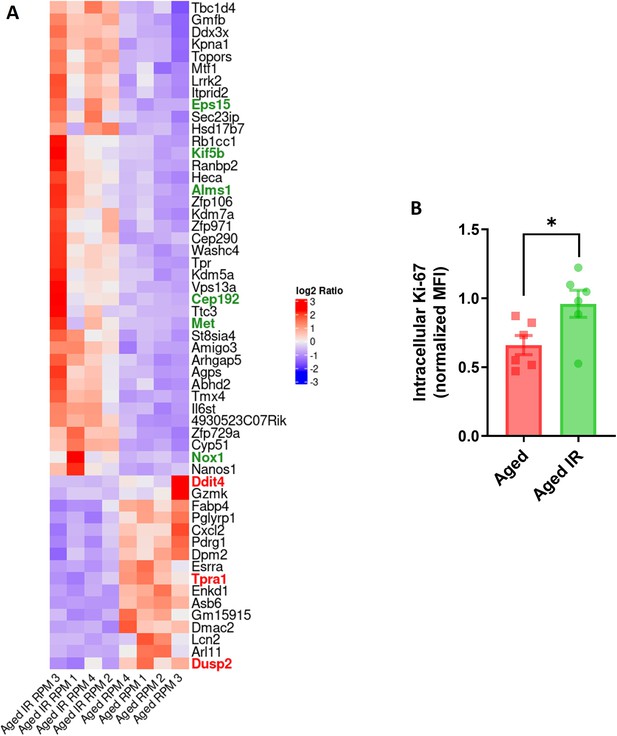
RPMs derived from aged IR versus aged mice show higher proliferation capacity.
(A) Heat map representing 55 differentially regulated genes between aged and aged IR RPMs. Genes positively involved in proliferation control are marked in green, and those that negatively regulate proliferation are highlighted in red. (B) Intracellular Ki-67 protein levels in RPMs derived from aged, and aged IR mice were assessed by flow cytometry. Each dot in (B) represents one mouse. Data are represented as mean ± SEM. Welch’s unpaired t-test determined the statistical significance between the two groups. *p<0.05.
-
Figure 1—figure supplement 8—source data 1
Related to Figure 1—figure supplement 8B.
- https://cdn.elifesciences.org/articles/79196/elife-79196-fig1-figsupp8-data1-v2.xlsx
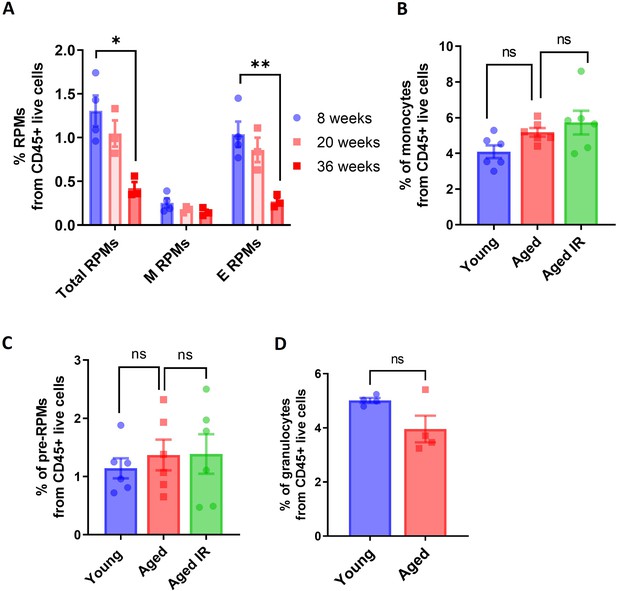
RPM depletion in aged mice affects embryonically-derived RPMs and is not a consequence of diminished recruitment from monocytes.
(A) Percentage of the total, monocyte-derived (M), and embryonically-derived (E) RPMs from CD45+ live cells present in the spleen of 8-, 20-, and 36-week-old mice. The results represent a re-analysis of the data from Liu et al., 2019. (B) Percentage of splenic monocytes (CD11b-high, F4/80-low) from CD45+ cells. (C) Percentage of splenic pre-RPMs (CD11b-high, F4/80 high) from CD45+ cells. (D) Percentage of splenic granulocytes (Gr1-high) from CD45+ cells. Each dot represents one mouse. Data are represented as mean ± SEM. Statistical significance among the three groups was determined by the One-Way ANOVA test with Tukey’s Multiple Comparison test; Welch’s unpaired t-test determined statistical significance between the two groups . ns p>0.05, *p<0.05, **p<0.01.
-
Figure 1—figure supplement 9—source data 1
Related to Figure 1—figure supplement 9A–D.
- https://cdn.elifesciences.org/articles/79196/elife-79196-fig1-figsupp9-data1-v2.xlsx
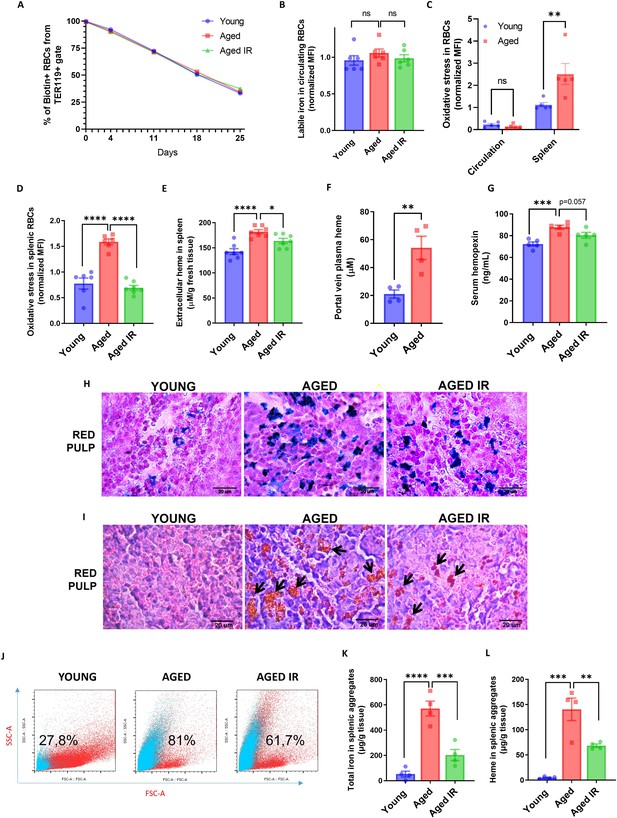
Aging triggers the retention of senescent RBCs, increased hemolysis, and the formation of non-degradable iron-rich aggregates in the spleen.
(A) The RBC biotinylation lifespan assay was performed on circulating RBCs from young, aged, and aged IR mice. (B) Cytosolic ferrous iron (Fe2+) levels in RBCs derived from the circulation of young, aged, and aged IR mice were measured using FerroOrange with flow cytometry. (C–D) The cytosolic ROS levels in RBCs derived from (C) circulation and the spleen of young and aged mice; (D) the spleen of young, aged, and aged IR mice. Levels of ROS were estimated by determining CellROX Deep Red fluorescence intensity with flow cytometry. (E) Extracellular heme levels were measured in the supernatant obtained after the dissociation of spleens from young, aged, and aged IR mice using the Heme Assay Kit. (F) Portal vein plasma heme levels were measured in young, aged, and aged IR mice using Heme Assay Kit. (G) Serum hemopexin levels were measured in young, aged, and aged IR mice with Mouse Hemopexin ELISA Kit. (H) Perls’ Prussian Blue staining of the splenic red pulp in young, aged, and aged IR mice. (I) Hematoxylin and eosin staining of the splenic red pulp in young, aged, and aged IR mice. Arrows indicate extracellular dark-colored aggregates. (J) Representative flow cytometry plots of magnetically-sorted splenocytes. In blue are events adverse for dead cell staining and cell surface markers CD45, TER119, CD11b, and F4/80. The percentage of these events in total acquired events is indicated. (K) The total iron content in magnetically-sorted, cell-free aggregates derived from spleens of young, aged, and aged IR mice was assessed using the Iron Assay Kit. (L) The heme content in magnetically-sorted, cell-free aggregates derived from spleens of young, aged, and aged IR mice was assessed using the Heme Assay Kit. Each dot in the graph (A) represents n=8. For the other panels, each dot represents one mouse. Data are represented as mean ± SEM. Welch’s unpaired t-test determined statistical significance between the two groups; for the three groups, One-Way ANOVA with Dunnett’s or Tukey’s Multiple Comparison test was applied. In (C) Two-Way ANOVA test was applied. *p<0.05, **p<0.01, ***p<0.001 and ****p<0.0001.
-
Figure 2—source data 1
Related to Figure 2A–G and K–L.
- https://cdn.elifesciences.org/articles/79196/elife-79196-fig2-data1-v2.xlsx
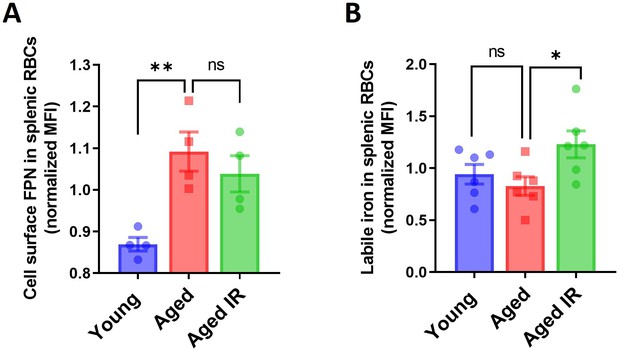
FPN levels and iron status in splenic RBCs in aging.
(A) Expression of ferroportin (FPN) on the surface of RBCs obtained from the spleen of young, aged, and aged IR mice were assessed by flow cytometry. (B) Cytosolic ferrous iron (Fe2+) levels in RBCs derived from the spleen of young, aged, and aged IR RPMs were measured using FerroOrange and flow cytometry. Each dot represents one mouse. Data are represented as mean ± SEM. Statistical significance among the three groups was determined by the One-Way ANOVA test with Tukey’s Multiple Comparison test. ns p>0.05, *p<0.05, **p<0.01.
-
Figure 2—figure supplement 2—source data 1
Related to Figure 2—figure supplement 2A–B.
- https://cdn.elifesciences.org/articles/79196/elife-79196-fig2-figsupp2-data1-v2.xlsx
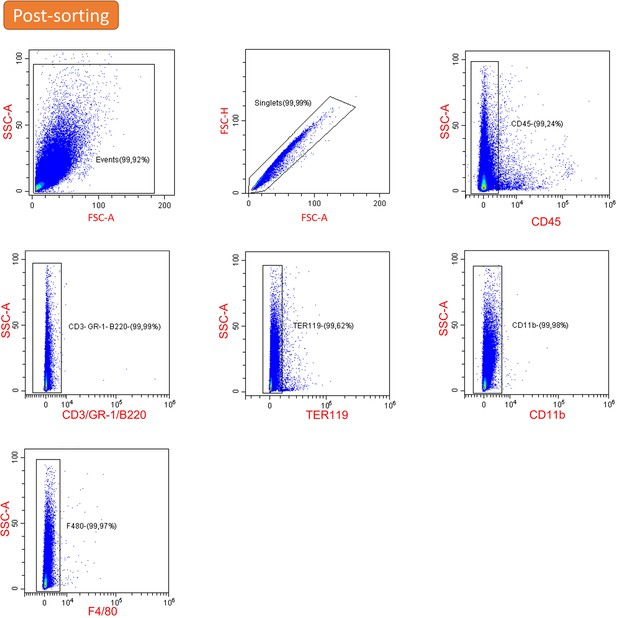
Post-sorting purity of extracellular aggregates isolated from the aged spleen.
Shown is the flow cytometry analysis of the isolated aggregates that are in >99% negative for the indicated splenocyte cell markers.
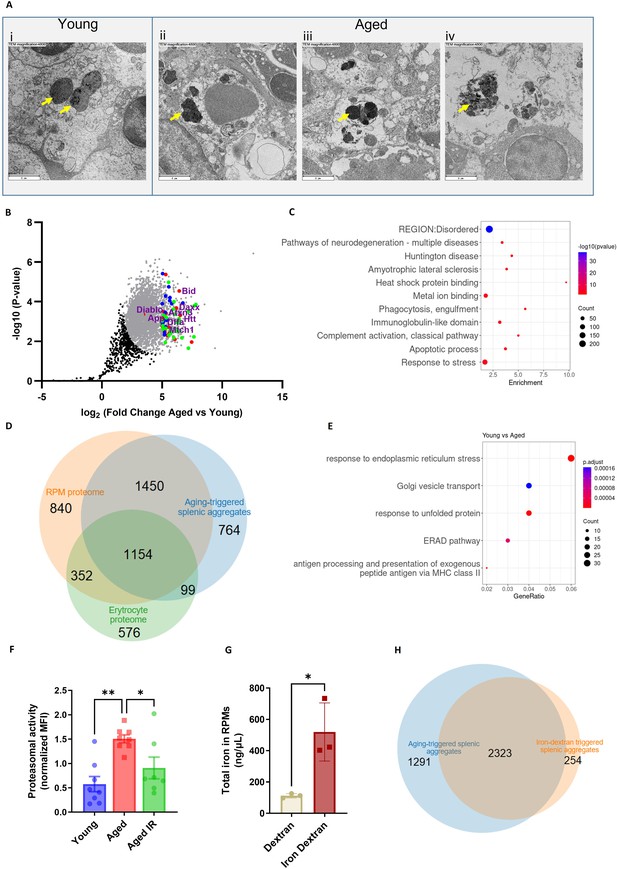
Splenic age-triggered iron deposits are rich in aggregation-prone proteins derived from damaged RPMs.
(A) Ultrastructural analyses of the spleen red pulp sections by transmission electron microscopy. Arrows indicate dark-colored dense deposits. (B) Volcano plot illustrating 3290 protein groups (in gray) that are significantly more abundant in magnetically-sorted, cell-free aggregates derived from aged versus young spleen. Based on the functional enrichment analyses, the red color denotes proteins linked with ‘pathways of neurodegeneration’, green - those associated with an ‘apoptotic process’ and blue - those related to the ‘immunoglobulin-like domain’ category. (C) Enriched functional categories among the top 387 protein hits that are more abundant in magnetically-sorted, cell-free aggregates derived from aged versus young spleen. (D) Venn diagram illustrating the number of common proteins identified in RPMs, erythrocytes (human), and aging-triggered splenic aggregates. (E) Enriched functional categories among differentially regulated genes in FACS-sorted RPMs derived from young versus aged mice (identified by RNA-seq). (F) Proteasomal activity was measured in RPMs derived from young, aged, and aged IR mice using a fluorescent proteasome activity probe with flow cytometry. (G) The total intracellular iron content in magnetically sorted RPMs derived from spleens of dextran- and iron dextran-injected mice (8 hr post-injection) was assessed using the Iron Assay Kit. (H) Venn diagram illustrating the number of common protein groups identified in aging-triggered and iron dextran-triggered splenic aggregates (log2 fold change >1.5 versus respective young and dextran-injected controls, respectively; n=2). The dextran-triggered aggregates were isolated 24 hr post-injection. Each dot represents one mouse; in (B) three biological replicates per group were analyzed. Data are represented as mean ± SEM. Welch’s unpaired t-test determined statistical significance between the two groups; statistical significance among the three groups was determined by One-Way ANOVA test with Tukey’s Multiple Comparison test. *p<0.05, **p<0.01.
-
Figure 3—source data 1
Related to Figure 3B.
- https://cdn.elifesciences.org/articles/79196/elife-79196-fig3-data1-v2.xlsx
-
Figure 3—source data 2
Related to Figure 3D.
- https://cdn.elifesciences.org/articles/79196/elife-79196-fig3-data2-v2.xlsx
-
Figure 3—source data 3
Related to Figure 3F–G.
- https://cdn.elifesciences.org/articles/79196/elife-79196-fig3-data3-v2.xlsx
-
Figure 3—source data 4
Related to Figure 3H.
- https://cdn.elifesciences.org/articles/79196/elife-79196-fig3-data4-v2.xlsx
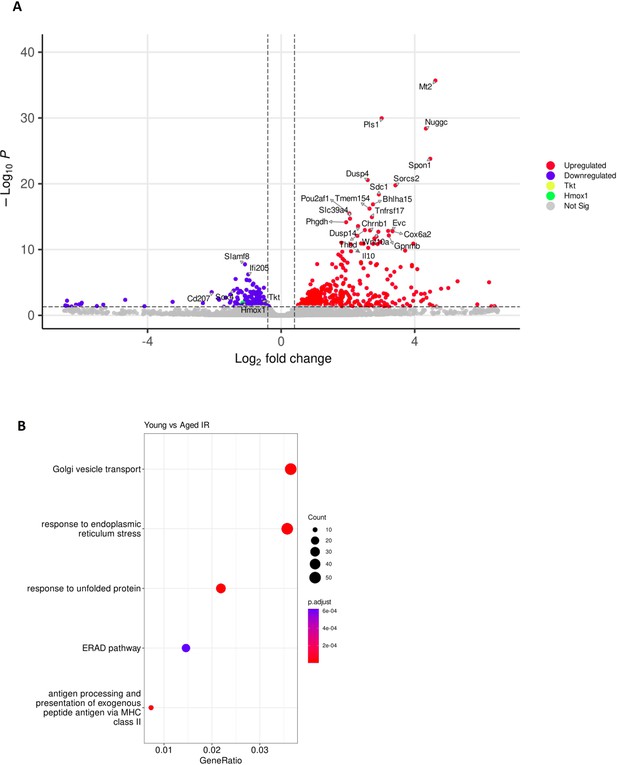
Supplementary data related to RNA-seq analysis of RPMs derived from young, aged, and aged IR mice.
(A) Volcano plot of differentially regulated genes in aged versus young FACS-sorted RPMs, with top hits indicated.(B) Enriched functional categories among differentially regulated genes in FACS-sorted RPMs derived from young versus aged IR mice. Four biological replicates of FACS-sorted RPMs per group were analyzed by RNA-seq.
-
Figure 3—figure supplement 1—source data 1
Related to Figure 3—figure supplement 1A.
- https://cdn.elifesciences.org/articles/79196/elife-79196-fig3-figsupp1-data1-v2.xlsx
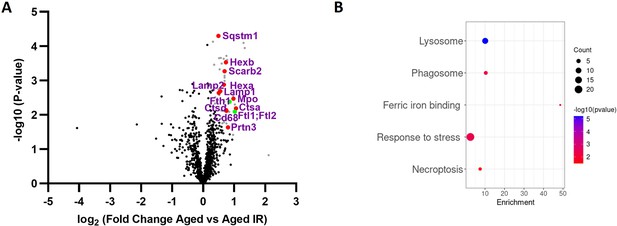
Components of lysosomes and ferritins are highly overrepresented in protein aggregates isolated from aged versus aged IR mice.
(A) Volcano plot illustrates protein groups (in gray) that are significantly more abundant in magnetically-sorted, cell-free aggregates derived from aged versus aged IR spleen. Based on the functional enrichment analyses, the red color denotes proteins linked with ‘lysosome’ and green – H and L ferritin. (B) Enriched functional categories among 50 protein hits that are more abundant in magnetically-sorted, cell-free aggregates derived from aged versus aged IR spleen. Three biological replicates per group were analyzed.
-
Figure 3—figure supplement 2—source data 1
Related to Figure 3—figure supplement 2A.
- https://cdn.elifesciences.org/articles/79196/elife-79196-fig3-figsupp2-data1-v2.xlsx
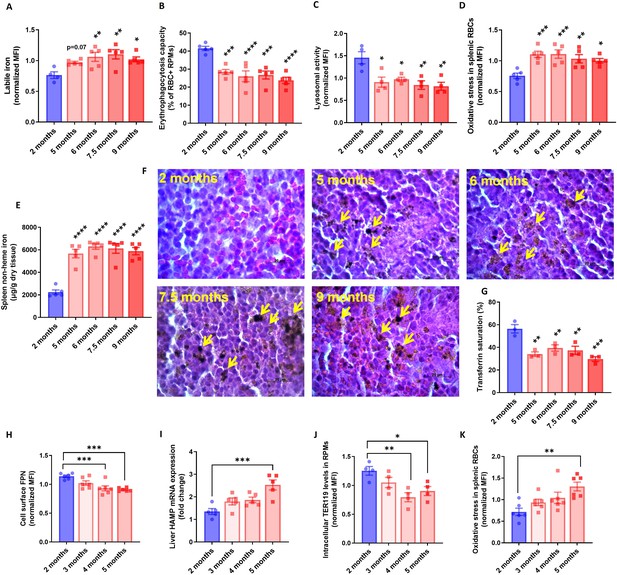
RPM dysfunction develops early in aging and is associated with ferroportin downregulation.
(A) Cytosolic ferrous iron (Fe2+) content in RPMs derived from mice at the indicated age was measured using FerroOrange with flow cytometry. (B) Erythrophagocytosis capacity of RPMs derived from mice at the indicated age was determined using flow cytometry by measuring the percentage of RPMs that phagocytosed transfused PKH67-labeled temperature-stressed RBCs. (C) Lysosomal activity in RPMs derived from mice at the indicated age was determined using Lysosomal Intracellular Activity Assay Kit by flow cytometry. (D) The cytosolic ROS levels in RBCs derived from the spleens of mice at the indicated age were estimated by determining CellROX Deep Red fluorescence intensity with flow cytometry. (E) Splenic non-heme iron content was determined in mice at the indicated age. (F) Hematoxylin and eosin staining of the splenic red pulp in young, aged, and aged IR mice. Arrows indicate extracellular dark-colored aggregates. (G) Plasma transferrin saturation was determined in mice at the indicated age. (H) Expression of ferroportin (FPN) on the cell membrane of RPMs derived from mice at the indicated age was assessed by flow cytometry. (I) Relative mRNA expression of hepcidin (Hamp) in the liver from mice at the indicated age was determined by qPCR. (J) Erythrophagocytosis capacity of RPMs derived from mice at the indicated age was determined by intracellular staining of the erythrocytic marker TER119 in RPMs and flow cytometry. (K) The cytosolic ROS levels in RBCs derived from the spleens of mice at the indicated age were estimated by determining CellROX Deep Red fluorescence intensity with flow cytometry. Each dot represents one mouse. Data are represented as mean ± SEM. Statistical significance versus young controls (2 months) was determined by One-Way ANOVA test with Dunnett’s Multiple Comparison test. *p<0.05, **p<0.01, ***p<0.001 and ****p<0.0001.
-
Figure 4—source data 1
Related to Figure 4A–E and G–K.
- https://cdn.elifesciences.org/articles/79196/elife-79196-fig4-data1-v2.xlsx
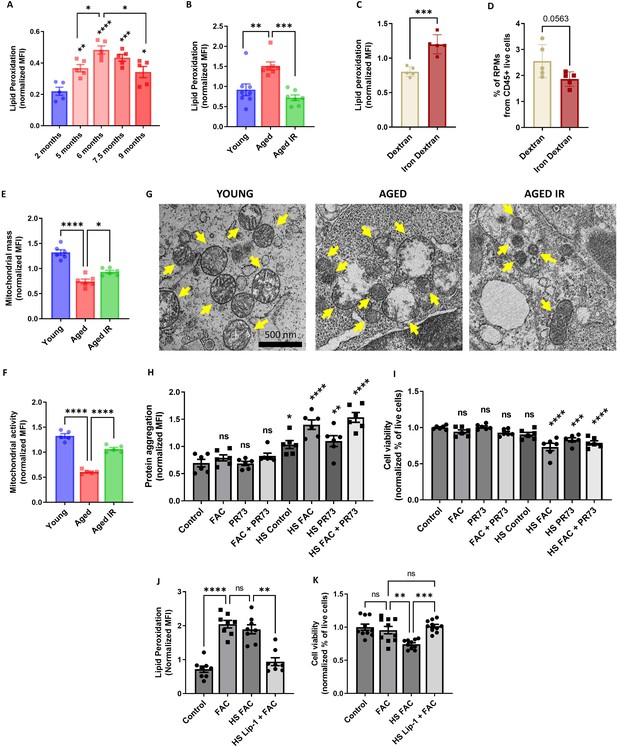
Aging triggers RPM loss via mechanisms that resemble ferroptosis and involve proteotoxicity.
(A) Lipid peroxidation was determined in RPMs derived from mice at the indicated age using the Lipid Peroxidation Assay Kit with flow cytometry. (B) Lipid peroxidation was determined in RPMs derived from young, aged, and aged IR mice using the Lipid Peroxidation Assay Kit with flow cytometry. (C) Lipid peroxidation was determined in RPMs derived from dextran- and iron-dextran-injected mice (8 hr post-injection) using the Lipid Peroxidation Assay Kit with flow cytometry. (D) The percentage of RPMs from CD45+ live cells present in the spleen of dextran- and iron dextran-injected mice (8 hr post-injection) was assessed by flow cytometry. (E) Mitochondrial mass and (F) mitochondrial activity were determined in RPMs derived from young, aged, and aged IR mice using MitoTracker Green and TMRE probes, respectively, with flow cytometry. (G) Ultrastructural analyses of mitochondrial morphology in spleen red pulp sections obtained from young, aged, and aged IR mice. Yellow arrows indicate mitochondria. (H) Protein aggregation and (I) cell viability in cultured iRPMs were determined using PROTEOSTAT Aggresome detection kit and a fluorescent Aqua Live/Dead probe, respectively, with flow cytometry. Cells were treated with FAC (150 µM, 24 hr), PR73 mini-hepcidin (2 µg/mL, 24 hr), or exposed to heat shock (HS) stress (42 °C, 4 hr) as indicated. (J) Lipid peroxidation and (K) cell viability of cultured iRPMs were determined using the Lipid Peroxidation Assay Kit and fluorescent Aqua Live/Dead probe, respectively, with flow cytometry. Cells were treated with FAC (150 µM, 24 hr), Liproxstatin-1 (Lip-1; 2 µM, 25 hr) or exposed to heat shock (HS) stress (42 °C, 4 hr) as indicated. Each dot represents one mouse or independent cell-based experiment. Data are represented as mean ± SEM. Welch’s unpaired t-test determined statistical significance between the two groups; statistical significance among the three or more groups was determined by One-Way ANOVA test with Dunnett’s or Tukey’s Multiple Comparison test. *p<0.05, **p<0.01, ***p<0.001 and ****p<0.0001.
-
Figure 5—source data 1
Related to Figure 5A–F and H–K.
- https://cdn.elifesciences.org/articles/79196/elife-79196-fig5-data1-v2.xlsx
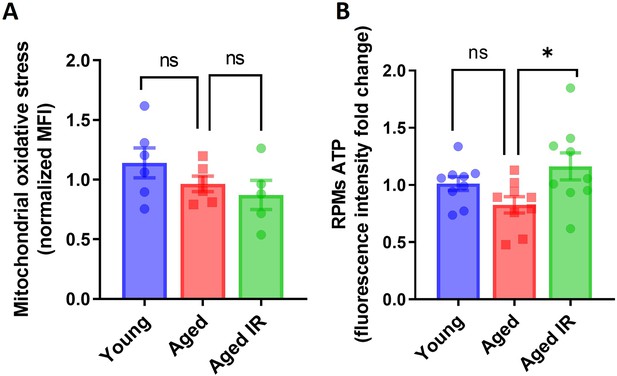
Mitochondrial oxidative stress and ATP levels in young, aged and aged IR RPMs.
(A) The mitochondria-associated ROS levels in young, aged and aged IR RPMs were determined using the MitoSOX Red probe with flow cytometry. (B) Levels of ATP in FACS-sorted RPMs isolated from young, aged, and aged IR mice were measured using ATP Fluorometric Assay Kit. Each dot represents one mouse. Data are represented as mean ± SEM. Statistical significance among the three groups was determined by the One-Way ANOVA test with Tukey’s Multiple Comparison test. ns p>0.05, *p<0.05.
-
Figure 5—figure supplement 1—source data 1
Related to Figure 5—figure supplement 1A–B.
- https://cdn.elifesciences.org/articles/79196/elife-79196-fig5-figsupp1-data1-v2.xlsx
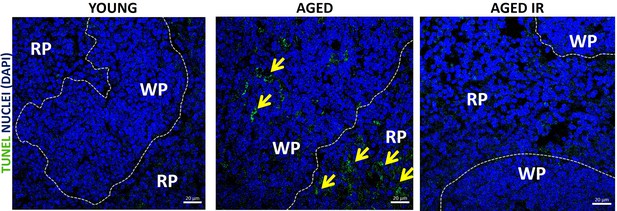
Apoptotic cell death was detected using In Situ Cell Death Detection Kit in spleen sections of young, aged and aged IR mice spleens.
The red pulp (RP) and white pulp (WP) are indicated. Yellow arrows indicate apoptotic cell death. The micrographs are representative of two or three mice per group.
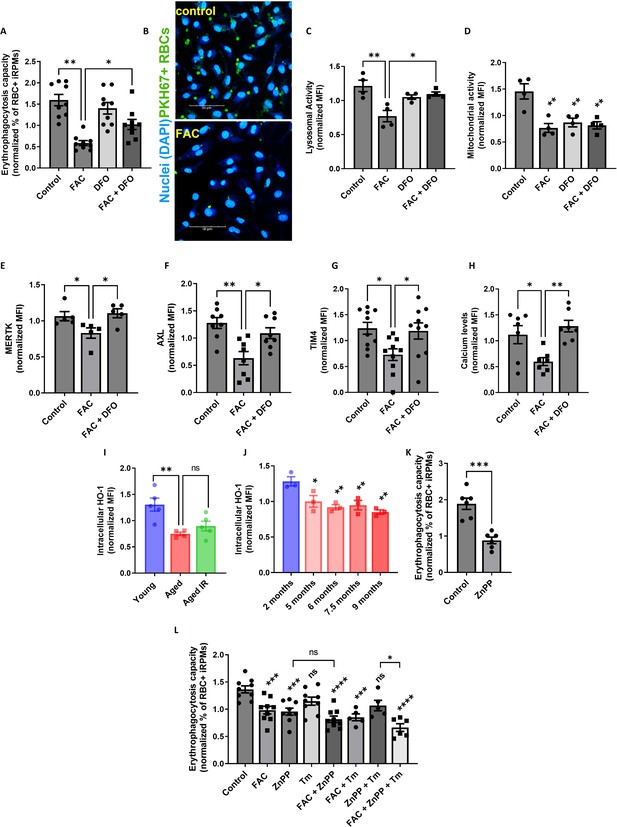
Iron loading undermines the phagocytic activity of iRPMs in concert with impaired heme metabolism and ER stress.
(A) Normalized erythrophagocytosis capacity of PKH67-labeled temperature-stressed RBCs by cultured iRPMs. Cells were treated with FAC (50 µM, 24 hr) and DFO (100 µM, 18 hr) as indicated. (B) Representative confocal microscopy images of erythrophagocytosis in FAC-treated iRPMs compared with control cells. (C) Lysosomal and (D) mitochondrial activity of cultured iRPMs were determined using Lysosomal Intracellular Activity Assay Kit and TMRE probe, respectively, with flow cytometry. Cells were treated with FAC (50 µM, 24 hr) and DFO (100 µM, 18 hr) as indicated. (E) Cell membrane expression levels of MERTK, (F) AXL and (G) TIM4 of cultured iRPMs were determined by using fluorescently labeled antibodies and flow cytometry. Cells were treated with FAC (50 µM, 24 hr) and DFO (100 µM, 18 hr) as indicated. (H) Cytosolic calcium levels of cultured iRPMs were determined using Cal-520 fluorescent probe with flow cytometry. Cells were treated with FAC (50 µM, 24 hr) and DFO (100 µM, 18 hr) as indicated. (I) Intracellular HO-1 protein levels in RPMs isolated from young, aged, and aged IR mice were measured by flow cytometry. (J) Intracellular HO-1 protein levels in RPMs isolated from mice at the indicated age were measured by flow cytometry. (K) Normalized erythrophagocytosis capacity of PKH67-labeled temperature-stressed RBCs by cultured iRPMs. Cells were treated with ZnPP (0.5 µM, 24 hr). (L) Normalized erythrophagocytosis capacity of PKH67-labeled temperature-stressed RBCs by cultured iRPMs. Cells were treated with indicated concentrations of ZnPP (0.5 µM) and FAC (10 µM) and with ER stress inducer Tunicamycin (Tm; 2.5 µM) for 24 hr. Each dot represents one mouse or independent cell-based experiment. Data are represented as mean ± SEM. Statistical significance among the three or more groups was determined by One-Way ANOVA test with Dunnett’s or Tukey’s Multiple Comparison test. *p<0.05, **p<0.01, ***p<0.001 and ****p<0.0001.
-
Figure 6—source data 1
Related to Figure 6A and C–L.
- https://cdn.elifesciences.org/articles/79196/elife-79196-fig6-data1-v2.xlsx
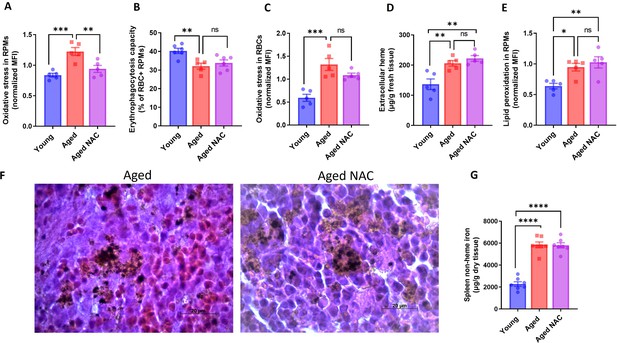
Iron loading, but not oxidative stress leads to RPM decline during aging.
(A) The cytosolic ROS levels in RPMs derived from young, aged, and aged NAC were assessed by determining CellROX Deep Red fluorescence intensity with flow cytometry. (B) RBC clearance capacity of RPMs derived from young, aged, and aged NAC mice was determined by measuring the percentage of RPMs that phagocytosed transfused PKH67-labeled temperature-stressed RBCs. (C) The cytosolic ROS levels in RBCs derived from the spleen of young, aged, and aged NAC mice were assessed by determining CellROX Deep Red fluorescence intensity with flow cytometry. (D) Extracellular heme levels were measured in the supernatant obtained after the dissociation of spleens from young, aged, and aged NAC mice using the Heme Assay Kit. (E) Lipid peroxidation was determined in RPMs derived from young, aged, and aged NAC mice using the Lipid Peroxidation Assay Kit with flow cytometry. (F) Hematoxylin and eosin staining of the splenic red pulp in young, aged, and aged IR mice. (G) Spleen non-heme iron content was determined in young, aged, and aged NAC mice. Each dot represents one mouse. Data are represented as mean ± SEM. Statistical significance among the three groups was determined by the One-Way ANOVA test with Tukey’s Multiple Comparison test. ns p>0.05, *p<0.05, **p<0.01, ***p<0.001 and ****p<0.0001.
-
Figure 6—figure supplement 1—source data 1
Related to Figure 6—figure supplement 1A–E and G.
- https://cdn.elifesciences.org/articles/79196/elife-79196-fig6-figsupp1-data1-v2.xlsx
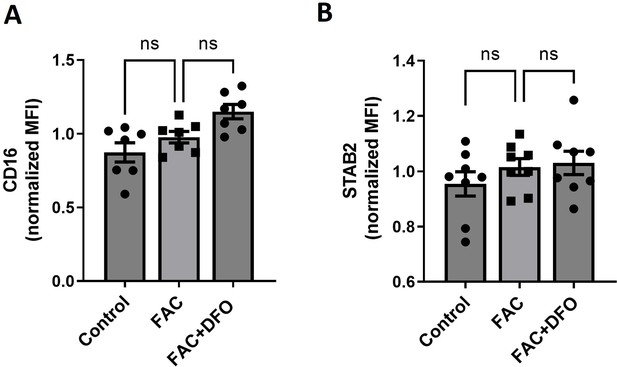
The Fc receptor CD16 and the apoptotic cell receptor STAB2 are not regulated by iron-loading.
(A) Cell membrane expression levels of CD16 and (B) STAB2 in iRPMs were determined by using fluorescently labeled antibodies and flow cytometry. Cells were treated with FAC (50 µM, 24 hr) with or without the addition of DFO (100 µM, 18 hr) as indicated. Each dot represents one independent cell-based experiment. Data are represented as mean ± SEM. Statistical significance among the three groups was determined by the One-Way ANOVA test with Tukey’s Multiple Comparison test. ns p>0.05.
-
Figure 6—figure supplement 2—source data 1
Related to Figure 6—figure supplement 2A–B.
- https://cdn.elifesciences.org/articles/79196/elife-79196-fig6-figsupp2-data1-v2.xlsx
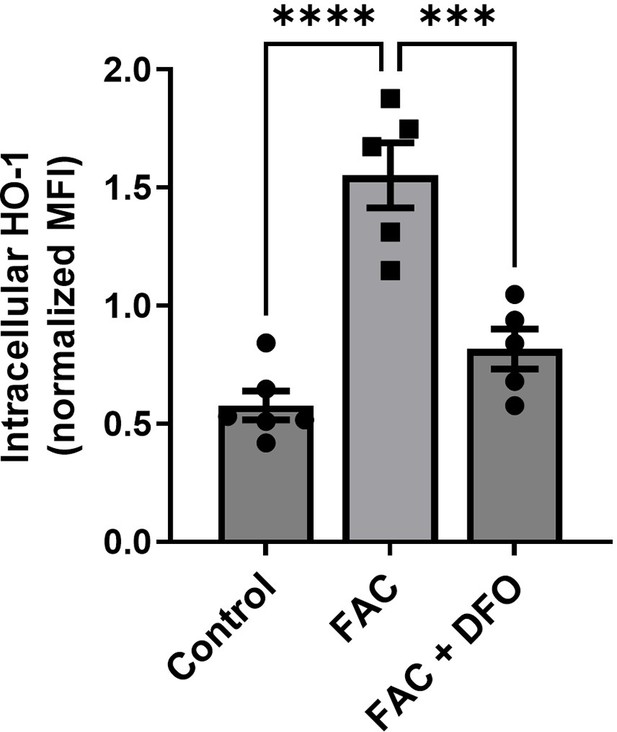
Validation of the antibody against HO-1 in flow cytometry.
Shown is the intracellular staining and flow cytometric analysis of HO-1 in bone-marrow-derived macrophages treated with ferric ammonium citrate (FAC, 50 µM, 24 hr) and iron chelator DFO (100 µM, 18 hr). Expected changes in fluorescence intensity were observed. Each dot represents one independent cell-based experiment. Data are represented as mean ± SEM. Statistical significance among the three groups was determined by the One-Way ANOVA test with Tukey’s Multiple Comparison test. ***p<0.001 and ****p<0.0001.
-
Figure 6—figure supplement 3—source data 1
Related to Figure 6—figure supplement 3.
- https://cdn.elifesciences.org/articles/79196/elife-79196-fig6-figsupp3-data1-v2.xlsx
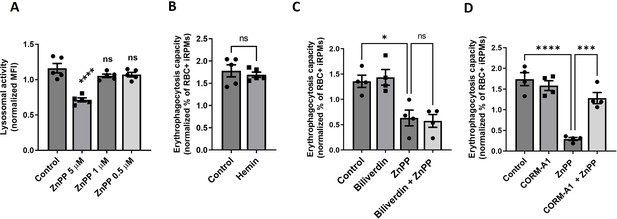
The effects of HO-1 inhibition on lysosomal activity and HO-1 co-products on the erythrophagocytosis capacity of iRPMs.
(A) Lysosomal activity of cultured iRPMs was determined using Lysosomal Intracellular Activity Assay Kit with flow cytometry. Cells were treated with ZnPP in 5 µM, 1 µM, and 0.5 µM concentrations for 24 hr. (B–D) Normalized erythrophagocytosis capacity of PKH67-labeled temperature-stressed RBCs by cultured iRPMs. Cells were treated with (B) hemin (50 µM, 24 hr), (C–D) ZnPP (5 µM, 24 hr) and (C) Biliverdin (50 µM, 24 hr) or (D) the CO donor CORM-A1 (50 µM, 24 hr) as indicated. Each dot represents one independent cell-based experiment. Data are represented as mean ± SEM. Statistical significance among the four groups was determined by One-Way ANOVA test with Dunnett’s or Tukey’s Multiple Comparison test; Welch’s unpaired t-test determined statistical significance between the two groups. ns p>0.05, *p<0.05, ***p<0.001 and ****p<0.0001.
-
Figure 6—figure supplement 4—source data 1
Related to Figure 6—figure supplement 4A–D.
- https://cdn.elifesciences.org/articles/79196/elife-79196-fig6-figsupp4-data1-v2.xlsx
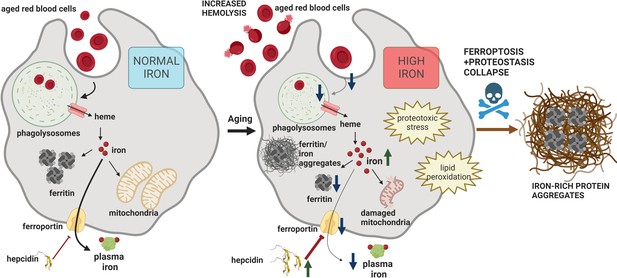
Model of RPM dysfunction and collapse during aging.
Iron-dependent functional defects of RPMs are initiated early during aging. Intracellular iron loading of RPMs, in concert with proteostasis defects, precedes the deposition of iron in a form of extracellular protein-rich aggregates, likely emerging from damaged RPMs. The build-up of un-degradable iron-rich deposits, in concert with increased hepcidin levels, and reduced erythrophagocytic and lysosomal activity of the remaining RPMs limit plasma iron availability during aging. A drop in RBC clearance capacity leads to enhanced splenic hemolysis.
Tables
Reagent type (species) or resource | Designation | Source or reference | Identifiers | Additional information |
---|---|---|---|---|
Antibody | Anti-mouse CD45 APC/Cyanine7 (Rat monoclonal) | BioLegend | cat.# 103115 | 1:200 |
Antibody | Anti-mouse/human CD45R/B220 Pacific Blue (Rat monoclonal) | BioLegend | cat.# 103230 | 1:200 |
Antibody | Anti-mouse F4/80 APC (Rat monoclonal) | BioLegend | cat.# 123115 | 1:100 |
Antibody | Anti-mouse F4/80 FITC (Rat monoclonal) | BioLegend | cat.# 123107 | 1:100 |
Antibody | Anti-mouse/human CD11b PE/Cyanine7 (Rat monoclonal) | BioLegend | cat.# 101215 | 1:200 |
Antibody | Anti-mouse Treml4 PE (Rat monoclonal) | BioLegend | cat.# 143303 | 1:100 |
Antibody | Anti-mouse TER-119 Pacifin Blue (Rat monoclonal) | BioLegend | cat.# 116231 | 1:300 |
Antibody | Anti-mouse TER-119 FITC (Rat monoclonal) | BioLegend | cat.# 116205 | 1:300 |
Antibody | Anti-mouse CD71 APC (Rat monoclonal) | BioLegend | cat.# 113819 | 1:100 |
Antibody | Anti-mouse CD71 PE (Rat monoclonal) | BioLegend | cat.# 113807 | 1:100 |
Antibody | Anti-mouse TER-119 PE (Rat monoclonal) | BioLegend | cat.# 116207 | 1:300 |
Antibody | Anti-mouse CD45 PE/Cyanine7 (Rat monoclonal) | BioLegend | cat.# 147703 | 1:200 |
Antibody | Anti-mouse FPN (Rat monoclonal) | Amgen | DOI:10.1172/JCI127341 | 1:100 |
Antibody | Anti-mouse Gr-1 Pacific Blue (Rat monoclonal) | BioLegend | cat.# 108429 | 1:200 |
Antibody | Anti-mouse MHCII Pacific Blue (Rat monoclonal) | BioLegend | cat.# 107619 | 1:200 |
Antibody | Anti-human/mouse Ferritin Heavy Chain (FTH1) (Rabbit polyclonal) | Cell Signaling Technology | cat.# 3998 | 1:100 |
Antibody | Anti-mouse AXL APC (Rat monoclonal) | Invitrogen | cat.# 17-1084-82 | 1:100 |
Antibody | Anti-mouse MERTK FITC (Rat monoclonal) | BioLegend | cat.# 151503 | 1:100 |
Antibody | Anti-mouse Tim-4 Alexa Fluor 647 (Rat monoclonal) | BioLegend | cat.# 130007 | 1:100 |
Antibody | Anti-mouse Stab2 Alexa Fluor 488 (Rabbit polyclonal) | G-Biosciences | cat.# ITN2255 | 1:100 |
Antibody | Anti-mouse/human Ferritin Light Chain (FTL) (Rabbit polyclonal) | Abcam | cat.# ab69090 | 1:100 |
Antibody | Anti-mouse HO-1 (Rabbit polyclonal) | Enzo Life Sciences | cat.# ADI-OSA-150 | 1:400 |
Antibody | Anti-mouse Ki-67 Alexa Fluor 488 (Rat monoclonal) | BioLegend | cat.# 652417 | 1:100 |
Antibody | Anti-Rabbit IgG (H+L) Alexa Fluor 488 (Donkey polyclonal) | Thermo Fisher Scientific | cat.# A-21206 | 1:200 |
Antibody | Anti-Rabbit IgG (H+L) Alexa Fluor 647 (Donkey polyclonal) | ThermoFisher Scientific | cat.# A-31573 | 1:200 |
Antibody | Anti-mouse CD16/32 TruStain FcX (Rat monoclonal) | BioLegend | cat.# 101319 | 1:100 |
Antibody | Anti-mouse Gr-1 Biotin (Rat monoclonal) | BioLegend | cat.# 108403 | 1:100 |
Antibody | Anti-mouse/human CD45R/B220 (Rat monoclonal) | BioLegend | cat.# 103204 | 1:100 |
Antibody | Anti-mouse CD3 (Rat monoclonal) | BioLegend | cat.# 100243 | 1:100 |
Antibody | Anti-mouse Ly-6C (Rat monoclonal) | BioLegend | cat.# 128003 | 1:100 |
Chemical compound, drug | N-Acetyl-L-cysteine (NAC) | Sigma-Aldrich | cat.# A7250 | 2 g/L in drinking water |
Chemical compound, drug | Iron-Dextran Solution | Sigma-Aldrich | cat.# D8517 | 8 mg |
Chemical compound, drug | Hemin | Sigma-Aldrich | cat.# 51280 | 20 µM |
Chemical compound, drug | Ferric Ammonium Citrate (FAC) | Sigma-Aldrich | cat.# F5879 | 150, 50 and 10 µM |
chemical compound, drug | CORM-A1 | Sigma-Aldrich | cat.# SML0315 | 50 µM |
Chemical compound, drug | Deferoxamine (DFO) | Sigma-Aldrich | cat.# D9533 | 100 µM |
Chemical compound, drug | Zinc (II) Protoporphyrin IX (ZnPP) | Sigma-Aldrich | cat.# 691550 | 5, 1 and 0.5 µM |
Chemical compound, drug | Tunicamycin (Tm) | Sigma-Aldrich | cat.# T7765 | 2.5 µM |
Chemical compound, drug | Liproxstatin-1 (Lip-1) | Sigma-Aldrich | cat.# SML1414 | 2 µM |
Chemical compound, drug | EZ-Link Sulfo-NHS Biotin | Thermo Fisher Scientific | cat.# 21217 | 1 mg / 100 µL |
Commercial assay, kit | LIVE/DEAD Fixable Aqua | Invitrogen | cat.# L34966 | |
Commercial assay, kit | LIVE/DEAD Fixable Violet | Invitrogen | cat.# L34964 | |
Commercial assay, kit | CellROX Deep Red Reagent | Invitrogen | cat.# C10422 | |
Commercial assay, kit | Lipid Peroxidation Assay Kit | Abcam | cat.# ab243377 | |
Commercial assay, kit | MitoSOX Red | Invitrogen | cat.# M36008 | |
Commercial assay, kit | Lysosomal Intracellular Activity Assay Kit | Biovision | cat.# K448 | |
Commercial assay, kit | Me4BodipyFL-Ahx3Leu3VS (Fluorescent Proteasome Activity) | R&D Systems | cat.# I-190 | |
Commercial assay, kit | tetramethylrhodamine ethyl ester - TMRE (Fluorescent Probe) | Sigma-Aldrich | cat.# 87917 | |
Commercial assay, kit | MitoTracker Green | Invitrogen | cat.# M7514 | |
Commercial assay, kit | Cal-520 AM Probe | Abcam | cat.# ab171868 | |
Commercial assay, kit | FerroOrange | DojinD | cat.# F374 | |
Commercial assay, kit | PROTEOSTAT Aggresome Detection Kit | Enzo Life Sciences | cat.# ENZ-51035–0025 | |
Commercial assay, kit | ATP Fluorometric Assay Kit | Sigma-Aldrich | cat.# MAK190 | |
Commercial assay, kit | Iron Assay Kit | Sigma-Aldrich | cat.# MAK025 | |
Commercial assay, kit | PKH-67 | Sigma-Aldrich | cat.# MIDI67-1KT | |
Commercial assay, kit | Heme Assay Kit | Sigma-Aldrich | cat.# MAK316 | |
Commercial assay, kit | SFBC | Biolabo | cat.# 80008 | |
Commercial assay, kit | UIBC | Biolabo | cat.# 97408 | |
Commercial assay, kit | Mouse Erythropoietin/EPO Quantikine ELISA Kit | R&D Systems | cat.# MEP00B | |
Commercial assay, kit | IL-6 Quantikine ELISA Kit | R&D Systems | cat.# M600B | |
Commercial assay, kit | Mouse Hemopexin ELISA Kit | Abcam | cat.# ab157716 | |
Commercial assay, kit | Direct-zol RNA Microprep Kit | Zymo Research | cat.# R2062 | |
Commercial assay, kit | Alexa Fluor 488 Conjugation Kit (Fast) - Lightning-Link | Abcam | cat.# ab236553 | |
Commercial assay, kit | Alexa Fluor 647 Conjugation Kit (Fast) - Lightning-Link | Abcam | cat.# ab269823 | |
Commercial assay, kit | RevertAid H Minus Reverse Transcriptase | Thermo Fisher Scientific | cat.# EP0452 | |
Commercial assay, kit | SG qPCR Master Mix | EURx | cat.# E0401 | |
Commercial assay, kit | Iron Stain Kit | Sigma-Aldrich | cat.# HT20-1KT | |
Commercial assay, kit | Iron Stain Kit | Abcam | cat.# ab150647 | |
Commercial assay, kit | In Situ Cell Death Detextion Kit | Roche | cat.# 11684795910 | |
Other | Standard iron content diet for mice | SAFE | cat.# U8958v0177 | Diet for young and aged mice |
Other | Reduced iron content diet for mice | SAFE | cat.# U8958v0294 | Diet for aged IR mice |
Other | Collagenase D | Roche | cat.# 11088882001 | Digestion of spleen for sorting |
Other | Trizol-LS | Invitrogen | cat.# 10296010 | RNA isolation reagent for RNA-seq |
Other | Normal Rat Serum | Thermo Fisher Scientific | cat.# 10,710 C | Blocking reagent for flow cytometry |
Other | MojoSort Streptavidin Nanobeads | BioLegend | cat.# 480016 | Beads for magnetic sorting of RPMs and aggreagtes |
Other | MojoSort Streptavidin Nanobeads | BioLegend | cat.# 480072 | Beads for magnetic sorting of RPMs and aggreagtes |
Other | EasyEights EasySep Magnet | STEMCELL | cat.# 18103 | Magnet used for magnetic sorting of RPMs and aggregates |
Other | LS Separation Column | Milyenyi Biotec | cat.# 130-042-401 | Magnet used for magnetic sorting of aggregates |
Other | CPDA-1 | Sigma-Aldrich | cat.# C4431 | Anticoagulant used for blood collection for transfusions |
Other | Accutase | BioLegend | cat.# 423201 | Reagent used for cells detachment in vitro |
Other | Zymosan A BioParticles Alexa Fluor 488 | Invitrogen | cat.# Z23373 | Cargo for ex vivo phgocytosis |
Other | TRIzol Reagent | Invitrogen | cat.# 15596018 | RNA isolation reagent |
Peptide, recombinant protein | IL-33 | BioLegend | cat.# 580506 | 10 ng/mL |
Peptide, recombinant protein | Mini-hepcidin (PR73) | Gift from Elizabeta Nemeth, UCLA, USA | https://doi.org/10.1128/IAI.00253-18 | 2 µg/mL |
Peptide, recombinant protein | Macrophage Colony-Stimulating Factor (M-CSF) | BioLegend | cat.# 576406 | 20 ng/mL |
Sequence-based reagent | HAMP Forward | This paper | Real-time PCR primers | 5’ ATACCAATGCAGAAGAGAAGG-3’ |
Sequence-based reagent | HAMP Reverse | This paper | Real-time PCR primers | 5’-AACAGATACCACACTGGGAA-3’ |
Software, algorithm | FlowJo | FlowJo | v10.8.1 | |
Software, algorithm | CytExpert | Beckman Coulter | v2.4 | |
Software, algorithm | GraphPad Prism | GraphPad Software | v9 |