Contrast polarity-specific mapping improves efficiency of neuronal computation for collision detection
Figures

Escape behavior to white and black looming stimuli.
(A) The locust eye (i) was stimulated using four simulated approaching squares (looming stimuli) with different contrast polarities (ii). (iii, iv): schematic of looming stimulus expansion on the retina (in the case of a black square) and time course of angular expansion for l/|v| = 80 ms, respectively. Inset illustrates the definition of the approach speed (v < 0), the square half-size (l) and the half-angle of the stimulus subtended at the retina (θ). Behaviorally, such stimuli lead to escape jumping (v). In (i), colored dots match those of Figure 2A. (B) Grasshoppers consistently jumped to white and black stimuli of different l/|v|. White stimuli had slightly lower but not significantly reduced escape responses (p=0.065, Fisher’s exact test). Error bars are 95% confidence intervals. (C) Comparison of jump response polarity preference for the seven animals tested shows reduced escape jumps for white stimuli (p=0.047, Wilcoxon signed-rank). (D) White stimuli produced jumps earlier relative to collision than did black stimuli for l/|v| of 80 ms; for l/|v| of 40, 80, and 120 ms, p=0.83, 8.9 • 10–4, and 0.26, respectively (Wilcoxon rank-sum test [WRS]). Points show individual trials. For (B) and (C), N = 263 trials from seven grasshoppers. See Methods, data analysis and statistics, for a description of box plot conventions.
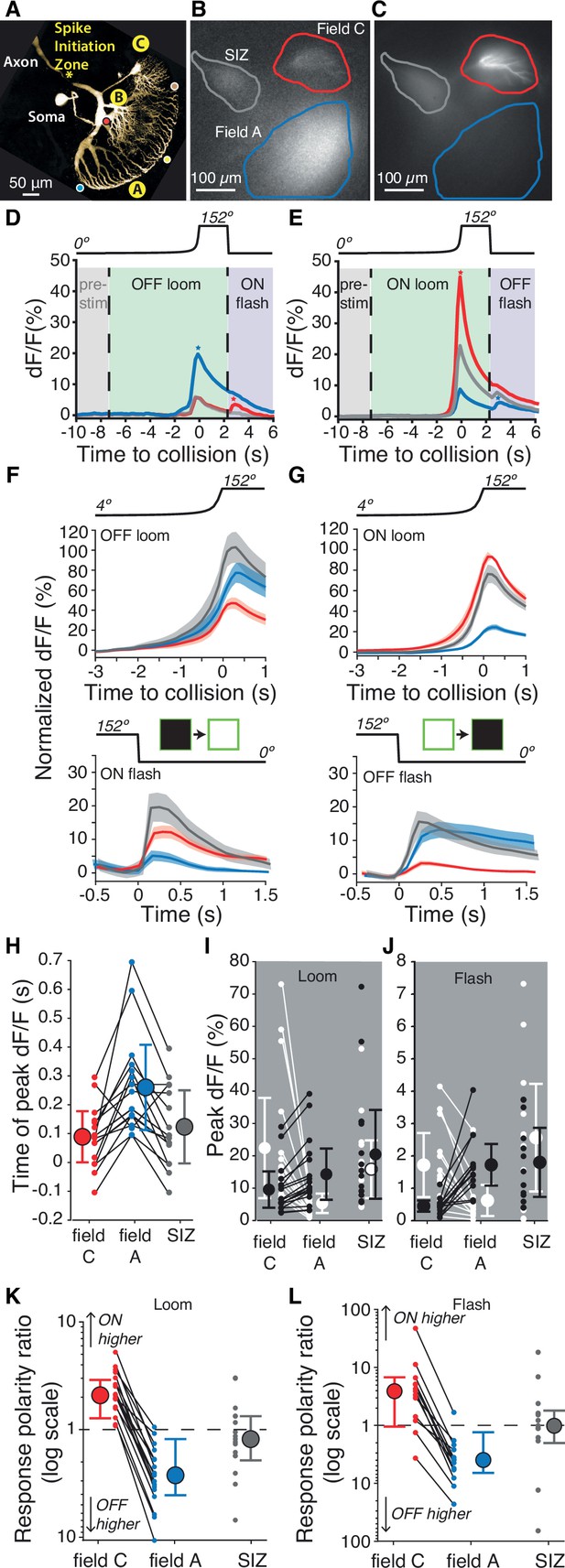
Luminance increases produce excitation in dendritic field C of the lobula giant movement detector (LGMD), unlike field A.
(A) Micrograph (two-photon scan) of the LGMD neuron illustrating the neurites imaged: dendritic fields A and C, as well as the spike initiation zone (SIZ). Colored dots matching those of Figure 1Ai indicate retinotopic mapping of visual field onto LGMD dendritic field A. (B, C) Micrographs of maximum dF/F taken during presentation of OFF and ON, looming stimuli. Areas enclosed by solid lines are regions of interest (ROIs) used to compute dF/F time course. (D, E) Corresponding dF/F time course in response to an OFF, respectively ON, looming stimulus within the ROIs marked by matching colored lines in (B, C). Pre-stim, baseline prior to looming; ON flash, disappearance of full-size black square from screen. Field A showed a larger increase in dF/F during looming (blue star, D). Field C showed a larger rebound to the ON flash (red star, D). Conversely, the ON loom produced a larger dF/F in field C (red star, E). Its disappearance (OFF flash) produced a larger rebound dF/F in field A (blue star, E). (F) Population average normalized dF/F for the three ROIs during presentation of black stimuli (same color code as in B). Lines and shaded regions are mean ± SEM. In each animal, normalization is to the peak response for ON looms. (G) Similar average normalized dF/F for ON stimuli. (H) The peak dF/F in field A occurred later than in field C for both ON and OFF looms (p=2.7 • 10–4). (I) Peak dF/F response to ON and OFF looming stimuli for each animal. Responses to ON stimuli were higher in field C (p=1.5 • 10–5) and black looming responses were higher in field A (p=1.5 • 10–5). (J) Peak dF/F response to post-loom flash. Responses to ON flashes were higher in field C (p=2.4 • 10–4) while OFF flash responses were higher in field A (p=2.4 • 10–4). (K) For all animals, loom response polarity is biased towards ON stimuli in field C (p=1.5 • 10–5). In field A, it is biased towards OFF stimuli in 16 of 17 animals (p=2.7 • 10–4). (L) Similarly, response polarity is biased towards ON flashes in field C (p=2.3 • 10–3) and towards OFF flashes in field A (p=2.7 • 10–4). For (F–L), N=17, and colors in (D–H) and (K, L) match the ROIs shown in (B). SIZ is gray, field A is blue, and field C is red. The p-values for (H–L) are from two-sided sign tests.
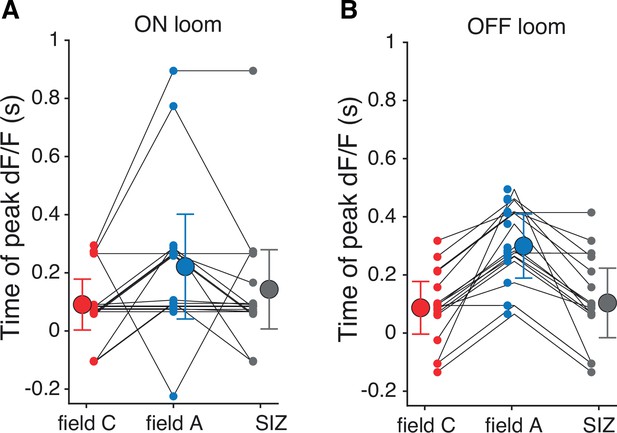
Timing of peak dF/F within each dendritic field as shown in Figure 2H with responses separated for ON (A) and OFF (B) looms.
Field A response timing for ON looms was highly variable, but the average peak time was consistent between stimulus polarities.
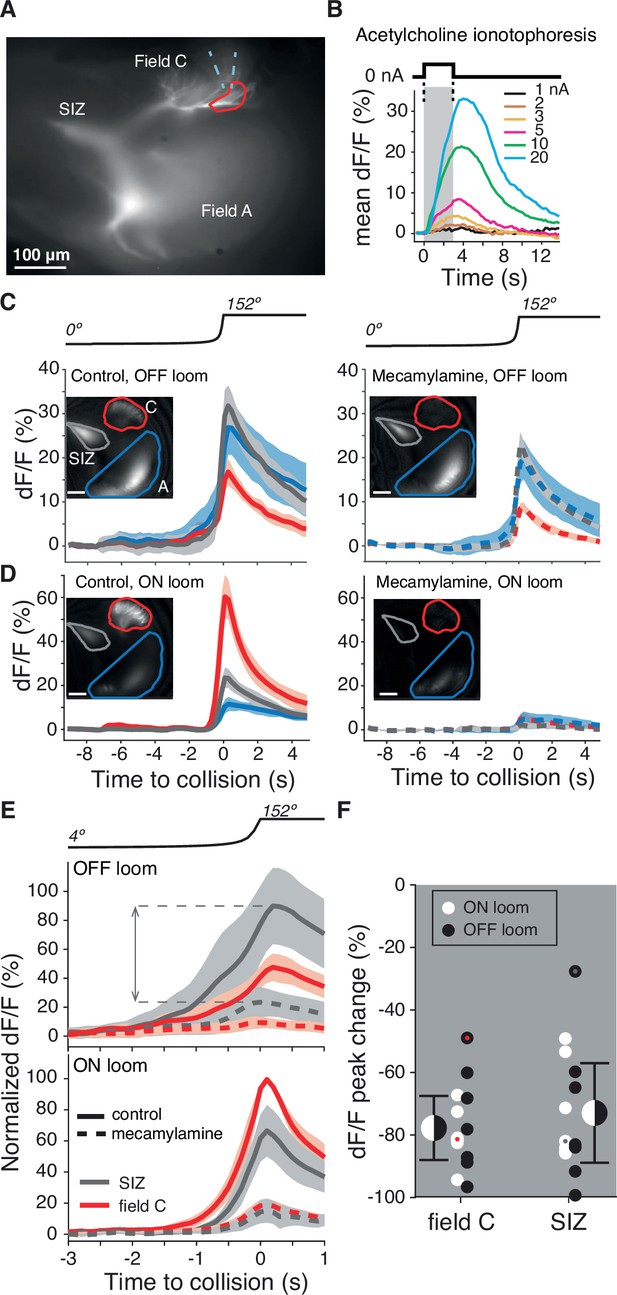
Dendritic field C receives excitatory synaptic inputs through nicotinic acetylcholine receptors (nAChRs).
(A) Micrograph of the lobula giant movement detector (LGMD) stained with OGB1 showing the locations of the spike initiation zone (SIZ), dendritic field A (both out of focus), and dendritic field C (in focus). Dashed blue wedge: location of iontophoresis electrode; closed red curve: boundary of area used to compute dF/F in (B). (B) Example dF/F to ACh iontophoresis for square current pulses (1–20 nA; top inset and gray shading). (C, D) Left: example responses from one animal showing the dF/F produced by Ca2+ influx in response to an OFF (C) or ON (D) looming stimulus in all three LGMD subregions (insets, as in A). Right: application of the nAChR blocker mecamylamine to field C reduced this influx (pipettes were placed as shown in A). Lines and shaded region are trial mean ± SD. (E) Average dF/F (± SEM) of seven animals before (solid lines) and after mecamylamine application (dashed lines) in field C (red) and at the SIZ (gray). Responses were normalized to each animal’s mean peak dF/F response in field C during an ON looming stimulus. Dashed horizontal lines and double arrow show how the peak change plotted in (F) was computed. (F) Looming responses were reduced in field C by 78% (p=0.015, sign test [ST]) and by 73% at the SIZ (p=0.015, ST) on average. Field A dF/F was reduced as well, 58%, but less than field C (p=0.013, ST). Black and white dots: individual animal responses. Half black and white discs: mean ± SD pooled across animals and stimuli. Dots marked red (field C) and gray (SIZ) correspond to the animal shown in (C, D).
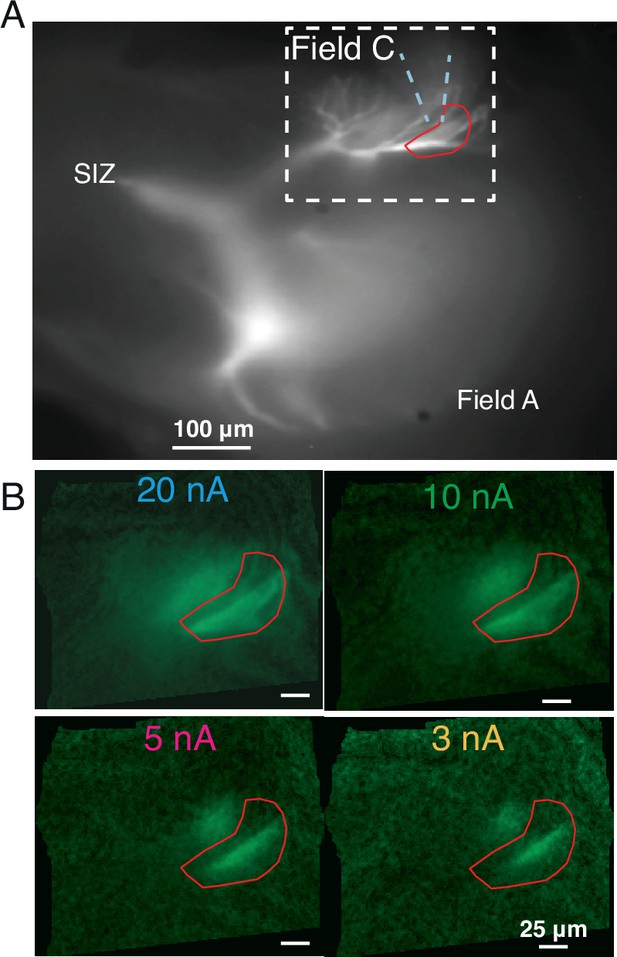
Micrographs of OGB1 fluorescence during iontophoresis of acetylcholine (ACh).
(A) Same image as in Figure 3A showing pipette location and the region of interest (ROI) used for the measurements depicted in Figure 3B. The white dashed box delimits the area shown in panel (B). (B) Larger currents caused increased dF/F due to a wider spread of ACh. For all current levels, responses were limited to field C branches directly exposed to ACh. Brightness of each image is normalized to peak dF/F.
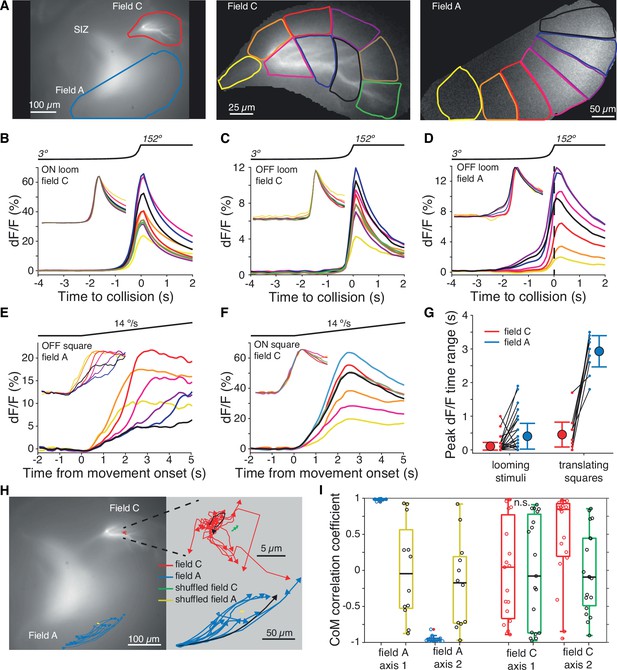
Within-field comparisons show lack of retinotopy in field C.
(A) Left: example dF micrograph in response to an ON loom with dendritic fields marked. Middle: close-up of field C indicating the color-coded subregions used for dF/F calculations. Right: similar close-up of field A in response to a black loom. (B) Time course of mean dF/F in field C subregions shown in (A) in response to ON looms. The traces are rescaled to have the same peak value in the inset. (C) Black looms elicited similarly timed responses across field C subregions. (D) Black looms produced earlier and larger responses in field A subregions receiving inputs from the center of the loom. (E) Responses to black translating squares show sequential activation of field A subregions (inset, as in B). (F) ON translating squares produced a large synchronous dF/F signal across field C (inset, as in B). (G) The range in peak dF/F loom response times between subregions was higher in field A than C (p=3.2 • 10–4, t-test, N = 21). For translating squares, the range of dF/F peak times was larger for field A (p=0.002, t-test, N = 8). (H) Micrograph shown in (A) with superimposed trajectories of the dF/F center of mass in dendritic fields A and C for each trial of a translating bar (blue and red lines). For each dendritic field, the center of mass trajectories of randomized data are shown with yellow and green lines, respectively. At right, the same trajectories are shown zoomed in with each trajectory aligned to their initial position to illustrate the direction of spread. For each field, one example trajectory is shown in black. (I) The correlations between dF/F center of mass and bar position of each animal. Data from fields A and C are shown in blue and red, respectively, and randomized data for each is shown in yellow and green. Circles indicate data from individual trials. For (H) and (I), data is taken from 19 trials of five animals. See Methods, data analysis and statistics for a detailed description of box plots.
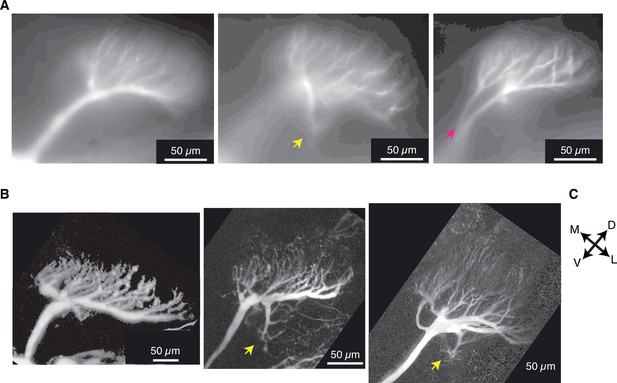
Micrographs of field C dendrites showing variability of branching patterns.
(A) CCD images of OGB1 fluorescence for three animals included in this study. (B) Maximum intensity projections of two-photon stacks showing field C stained with Alexa 594 in three different animals. The images show common field C morphologies including: field C forming a single fan (two left images), a single field with an additional branch projecting postero-ventrally (yellow arrows), and splitting into separate dendritic fans (red arrow shows origin of split). (C) All images are in the same orientation, with the dorsal edge of the lobula in the top, right corners. M, medial; D, dorsal; V, ventral; L, lateral. The anterior surface is toward and posterior is away from the viewer.
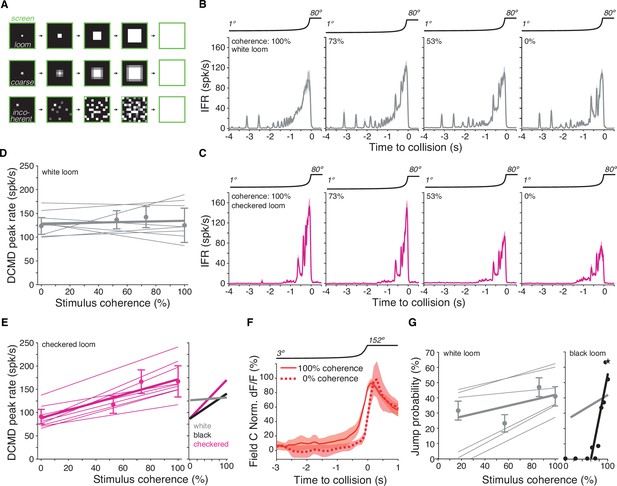
Lack of spatial coherence preference for white stimuli.
(A) Top: schematic of looming stimulus. Middle: coarse looming stimulus. Bottom: stimulus with reduced spatial coherence. (B, C) Firing frequency of the lobula giant movement detector (LGMD) in response to white and checkerboard looming stimuli, respectively. Lines and shaded region are mean ± SEM, N = 9. As the spatial coherence was decreased, the response to checkered looms decreased (magenta). Responses to white looming stimuli did not decrease with reduction in spatial coherence (gray). (D, E) Linear fits of the peak firing rate to the spatial coherence of the stimulus. Thin lines are fits to individual animals, thick lines are fits to the population. Points and error bars are population median ± mad. Checkered stimuli had a mean ρ of 0.91, while for white stimuli, mean ρ was 0.15. Right inset: firing rate as a function of stimulus coherence for black looms (from Dewell and Gabbiani, 2018b) compared to data for white and checkered looms at left and in (D). (F) Time course of field C normalized dF/F in response to 100 and 0% coherent white looming stimuli shows no change in peak value (N = 6). (G) Left: jump probability as a function of stimulus coherence for white looms (mean ± SD, N = 6). Thick gray line: linear fit; thin gray lines: fits on individual animals. Right inset: jump probability as a function of stimulus coherence for black looms (from Dewell and Gabbiani, 2018a). Thick gray line is reproduced from left plot for comparison, but note these data are from different animals so the absolute jump probabilities cannot be directly compared.
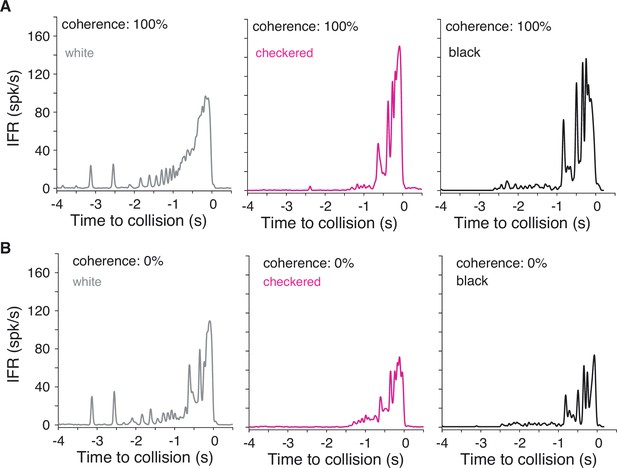
Instantaneous firing rates for white and checkered stimuli from Figure 5 compared to previously recorded responses to black stimuli.
(A) For 100% coherent coarse looms, white stimuli produced slightly lower peak firing rates than checkered or black stimuli. (B) For 0% coherence scrambled looms, however, white stimuli produced higher firing rates than checkered or black stimuli. Note that the white and checkered data were recorded from different animals than the black responses, so comparisons with responses to black stimuli are qualitative only; see Figure 1G of Dewell and Gabbiani, 2018a for illustration of variability of responses across animals to these stimuli.
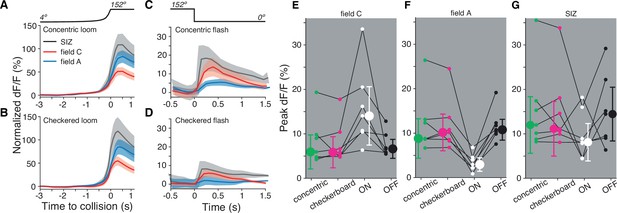
Both dendritic fields A and C respond similarly to looming stimuli of mixed luminance and to black looms.
(A) Looming stimuli composed of five concentric ON and OFF squares produce slightly higher responses in field A (p=0.016, sign test [ST]). (B) Checkered looming stimuli similarly produced higher field A responses (p=0.016, ST). (C, D) Post-loom flash responses were higher in field C after concentric looming stimuli (p=0.026, ST) but not for post-checkered flashes (p=0.093, ST). (E) Field C peak dF/F for each stimulus. ON looms elicited the highest response in all animals (p=0.015, ST), and responses to the other three stimuli were not significantly different (p=0.82, Kruskal–Wallis test [KW]). (F) Field A peak dF/F for each stimulus. ON looms elicited the lowest response in all animals (p=0.015, ST), and responses to the other three stimuli were not different (p=0.89, KW). (G) All stimuli produced similar peak dF/F at the spike initiation zone (SIZ) (p=0.26, KW). In (A–G), N = 7 animals.
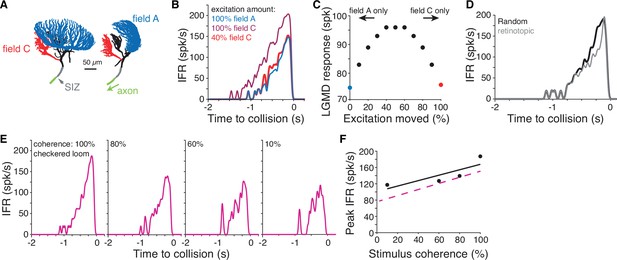
Simulations of a biophysical lobula giant movement detector (LGMD) model reveal energetic savings of segregated inputs.
(A) Images of the model morphology showing the dendritic fields; the right image shows the model neuron rotated 90° from the left image. (B) Instantaneous firing rate (IFR) of the model’s response to a black loom with excitation impinging on field A (blue line; same synapse locations as in Dewell and Gabbiani, 2018b). Moving all excitation to random field C locations (dark red) increased firing. Removal of 60% of excitatory synapses still produced a response as high as the field A inputs (red). (C) If excitatory synapses of simulated looms were distributed between the dendritic fields (with removal of 60% of synapses moved to field C), responses were highest with inputs split evenly between fields. Red and blue points are same as simulations shown in (B). (D) If all excitation impinged on field C with excitatory inputs clustered and spreading from a central point to approximate a retinotopic mapping, responses were reduced relative to inputs with random mapping. (E) Simulations reproduced firing patterns of response to checkered looming stimuli of different spatial coherences (Figure 5C). (F) Spatial coherence preference for checkered looms for the model (black) and experimental data (dashed magenta).
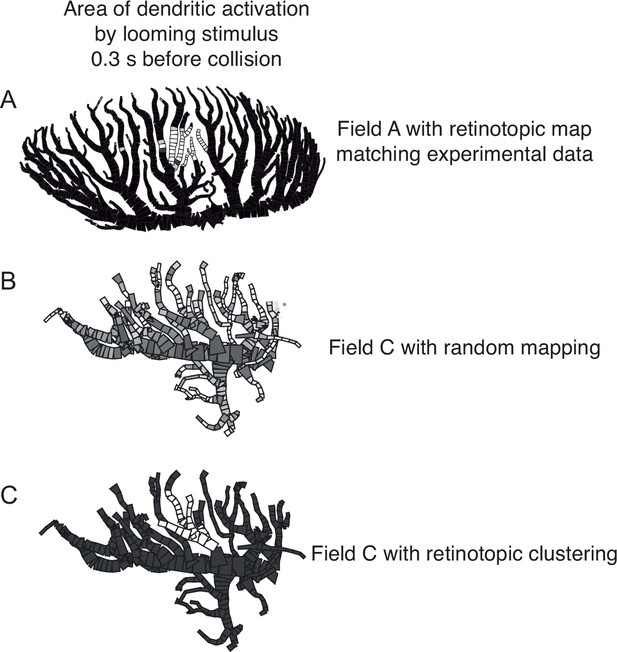
Illustration of retinotopic clustering used in simulations for Figure 7D.
(A) Area of field A dendrites activated by an OFF loom, 300 ms before projected collision. Black compartments indicate unexcited compartments, lighter compartments have greater activation. (B) The activation pattern of field C with randomly mapped inputs, 300 ms before collision of an ON loom. (C) Activation pattern of same field C inputs but clustered retinotopically. Panel (A) adapted from Figure 7D of Dewell and Gabbiani, 2018a.
Videos
Example jump escape response of a grasshopper to a white looming stimulus.
As the stimulus expands, the animal jumps and flies away before the time of projected collision. Frame rate: 200 Hz; slowed to 30 Hz. Compare to a similar response for a black looming stimulus in Video 3 of Dewell and Gabbiani, 2018a; DOI: 10.7554/eLife.34238.008.
Example of raw fluorescence signal within the lobula giant movement detector (LGMD) in response to a white looming stimulus (inset upper left).
As the looming stimulus expands, note the fluorescence increase in field C (top right, in focus). Baseline field A fluorescence is also visible at the center. Frame rate for Videos 2–7: 5 Hz; real speed. The visual stimuli in Videos 2–7 have been downsampled from 240 to 5 Hz to match fluorescence data. Matches data of Figure 2C and E. Image size for Videos 2–7: 630 × 470 µm. Scale bar: 100 µm.
The calculated fluorescence dF/F of the same trial shown in Video 2.
Note the disappearance of field A baseline fluorescence through this manipulation.
An example of the fluorescence dF/F during a black looming stimulus (inset upper left).
Data is from the same lobula giant movement detector (LGMD) as shown in Videos 2 and 3. Matches data of Figure 2B, D. Scale bar: 100 µm.
Example of fluorescence dF/F within the lobula giant movement detector (LGMD) in response to a black moving dot stimulus (inset upper left). Scale bar: 100 µm.
Note strong activation in eld A sweeping along a crescent starting from bottom center (out of focus). In addition, some uniform activation is also seen in eld C (top right, in focus). Matches data in Figure 4E.