Manganese is a physiologically relevant TORC1 activator in yeast and mammals
Figures
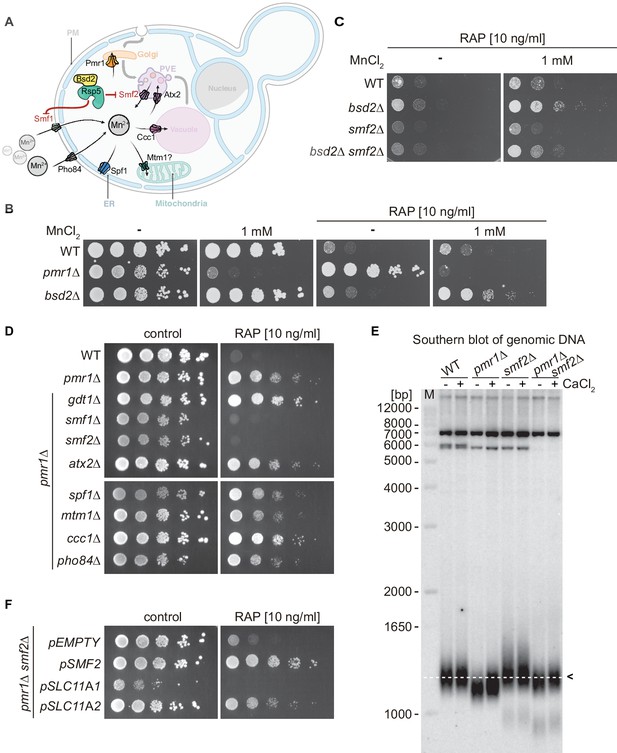
Natural resistance-associated macrophage protein (NRAMP) transporters link Mn2+-import to rapamycin resistance.
(A) Schematical outline of yeast Mn2+ transporters and their intracellular localization. PM, plasma membrane; PVE, pre-vacuolar endosomes; ER, endoplasmic reticulum. Note that Bsd2 is a specific adaptor protein for Rsp5-mediated Smf1 and Smf2 ubiquitination in response to Mn2+ overload. The Golgi Mn2+ transporter Gdt1 is omitted for clarity. (B–D) Growth on MnCl2 and/or rapamycin-containing medium (RAP). Ten-fold dilutions of exponentially growing cells are shown. Strains and compound concentrations are indicated. Note that the medium used in (D) was supplemented with 10 mM CaCl2. Data obtained in a medium without CaCl2 are shown in Figure 1—figure supplement 3. (E) Southern blot analysis of telomere length. Genomic DNA was derived from cells grown in a medium supplemented or not with CaCl2 and cleaved by XhoI before agarose gel electrophoresis. The 1.3 kb average length of telomeres from WT cells (dashed white line, black arrow) and size marker (M) are shown. (F) Growth of pmr1∆ smf2∆ double mutants transformed with plasmids expressing yeast Smf2, Mus musculus SLC11A1, or SLC11A2 on rapamycin-containing medium. Complementary data showing that pmr1∆ rapamycin resistance is not linked to Gap1 or Tor1 localization is provided in Figure 1—figure supplement 1. Rapamycin sensitivity of bsd2∆ cells overexpressing the Vcx1-M1 transporter are shown in Figure 1—figure supplement 2.
-
Figure 1—source data 1
Uncropped autoradiography image shown in Figure 1E.
- https://cdn.elifesciences.org/articles/80497/elife-80497-fig1-data1-v1.pdf
-
Figure 1—source data 2
Raw autoradiography image shown in Figure 1E.
- https://cdn.elifesciences.org/articles/80497/elife-80497-fig1-data2-v1.zip
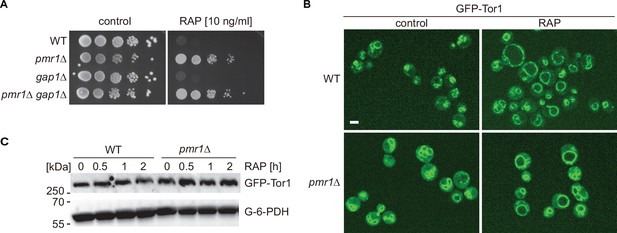
pmr1∆ rapamycin resistance is not linked to Gap1 or Tor1 localization.
(A) Sensitivity of indicated strains to rapamycin (RAP). Ten-fold dilutions of exponentially growing cells are shown. (B) Spinning disk microscopic analysis of GFP-Tor1 localization WT and pmr1∆ cells upon treatment with 200 ng/ml rapamycin (+RAP) for 30 min. Scale bar represents 5 µm. (C) Exponentially growing cells expressing GFP-Tor1 from the endogenous locus were treated for up to 2 hr with 200 ng/ml rapamycin (RAP). GFP-Tor1 protein levels were analyzed by immunoblotting with an anti-GFP antibody. G-6-PDH levels were used as a loading control.
-
Figure 1—figure supplement 1—source data 1
Uncropped blot shown in Figure 1—figure supplement 1C.
- https://cdn.elifesciences.org/articles/80497/elife-80497-fig1-figsupp1-data1-v1.pdf
-
Figure 1—figure supplement 1—source data 2
Raw blot shown in Figure 1—figure supplement 1C.
- https://cdn.elifesciences.org/articles/80497/elife-80497-fig1-figsupp1-data2-v1.zip

Growth of bsd2∆ mutants expressing Vcx1-M1 from plasmid pVCX1-M1 on MnCl2 and/or rapamycin (RAP) containing medium.
Ten-fold dilutions of exponentially growing cells are shown. Compound concentrations are indicated.
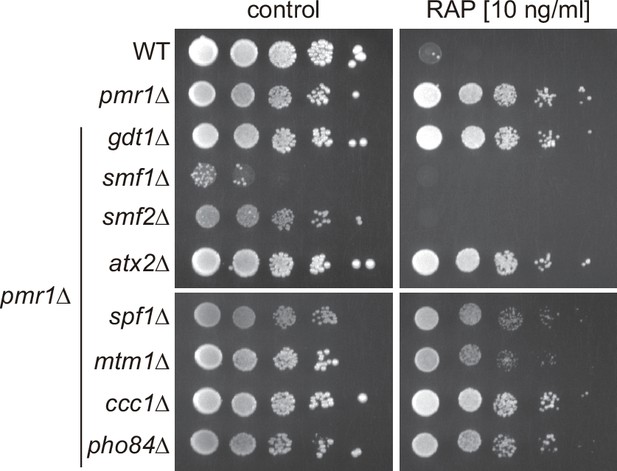
Natural resistance-associated macrophage protein (NRAMP) transporters mediate rapamycin resistance of pmr1∆ mutants.
Sensitivity of the indicated strains to rapamycin (RAP). Ten-fold dilution of exponentially growing cells are shown. Data obtained in medium supplemented with CaCl2 is shown in Figure 1D. Note that growth of the pmr1∆ smf1∆ double mutant is strongly impaired without CaCl2 addition.
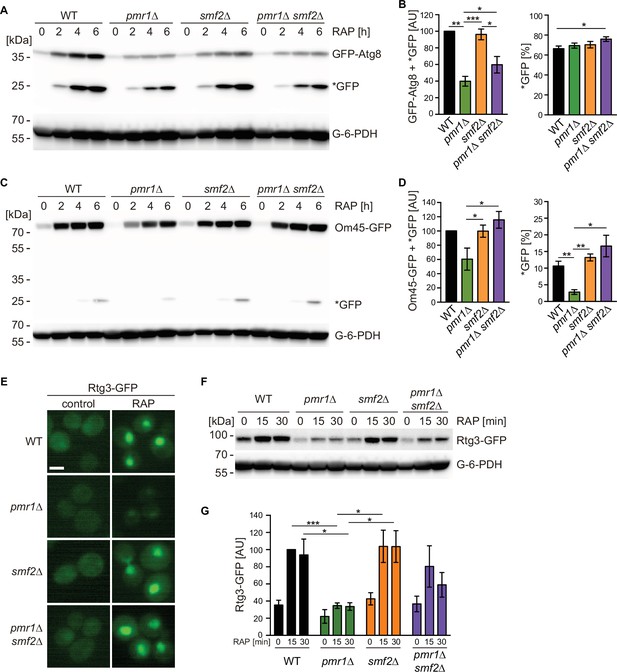
Intracellular manganese (Mn) excess antagonizes rapamycin-induced autophagy, mitophagy, and Rtg1-3 retrograde signaling.
(A) Exponentially growing WT and indicated mutant strains expressing plasmid-encoded GFP-Atg8 were treated for up to 6 hr with 200 ng/ml rapamycin (RAP). GFP-Atg8 and cleaved GFP (*GFP) protein levels were analyzed by immunoblotting. Glucose-6-phosphate dehydrogenase (G-6-PDH) levels were used as a loading control. (B) Quantification of GFP-Atg8 and *GFP levels after a 6 hr rapamycin treatment. Total GFP signal (Atg8-GFP + *GFP) normalized to WT levels (left) and percentage of *GFP relative to the total GFP signal (right) are plotted. Data represent means ± SEM of independent experiments (n=4). Statistical analysis: two-tailed t-test (paired for normalized data, unpaired for GFP* percentage). *p<0.05; **p<0.01; ***p<0.001. (C) Exponentially growing WT and indicated mutant strains expressing Om45-GFP from the endogenous locus were treated and processed as in (A). (D) Quantification of Om45-GFP and *GFP levels after a 6 hr rapamycin treatment. Details as in (B) with n=3. (E) Representative fluorescence microscope images of WT, pmr1∆, smf2∆, and pmr1∆ smf2∆ mutants expressing an episomic Rtg3-GFP reporter construct. Exponentially growing cells were treated or not (control) for 30 min with 200 ng/ml rapamycin (RAP). Scale bar represents 5 µm. (F) Rtg3-GFP expressing cells were treated for up to 30 min with 200 ng/ml rapamycin. Protein levels were analyzed by immunoblotting. G-6-PDH levels were used as a loading control. (G) Quantification of Rtg3-GFP signals. Values normalized to WT levels after 15 min of rapamycin treatment (highest signal) are plotted. Data represent means ± SEM of independent experiments (n=4). Statistical analysis: paired two-tailed t-test.
-
Figure 2—source data 1
Quantification of blots for graphs shown in Figure 2B–D–G.
- https://cdn.elifesciences.org/articles/80497/elife-80497-fig2-data1-v1.xlsx
-
Figure 2—source data 2
Uncropped blots shown in Figure 2A–C–F and quantified in Figure 2B–D.
- https://cdn.elifesciences.org/articles/80497/elife-80497-fig2-data2-v1.pdf
-
Figure 2—source data 3
Raw blots shown in Figure 2A–C–F and quantified in Figure 2B–D.
- https://cdn.elifesciences.org/articles/80497/elife-80497-fig2-data3-v1.zip
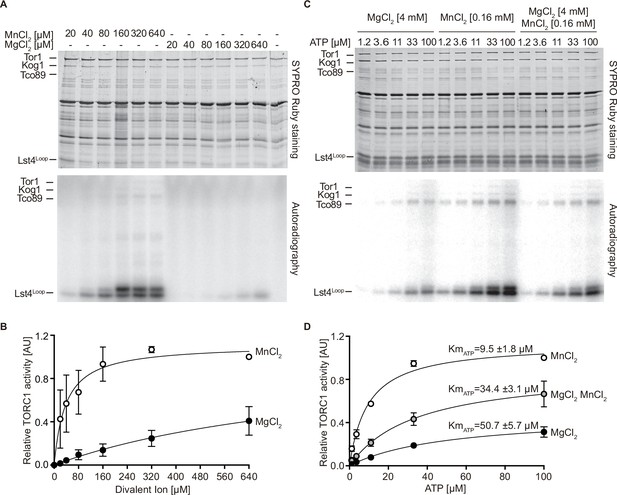
MnCl2 stimulates TORC1 kinase activity in vitro and in vivo.
(A) In vitro TORC1 kinase assays using [γ-32P]-ATP, recombinant Lst4Loop as substrate, and increasing concentrations (twofold dilutions) of MgCl2 or MnCl2. Substrate phosphorylation was detected by autoradiography (lower blot) and SYPRO Ruby staining is shown as loading control (upper blot). (B) Quantification of the assay shown in (A). Curve fitting and parameter calculations were performed with GraphPad Prism. Data shown are means (± SEM, n=3). (C) In vitro kinase assays (as in A) using the indicated concentrations of MgCl2 and/or MnCl2 and increasing concentrations of ATP. Substrate phosphorylation was detected by autoradiography (lower blot) and SYPRO Ruby staining is shown as loading control (upper blot). (D) Quantification of the assay shown in (C). Data shown are means (± SEM, n=3). Curve fitting and parameter calculations were performed with GraphPad Prism. KmATP are shown for each curve. VMAX [MnCl2]=1.13 ± 0.06, VMAX [MgCl2 MnCl2]=0.89 ± 0.03, VMAX [MgCl2]=0.47 ± 0.02.
-
Figure 3—source data 1
Quantification of autoradiographies for graphs shown in Figure 3B–D.
- https://cdn.elifesciences.org/articles/80497/elife-80497-fig3-data1-v1.xlsx
-
Figure 3—source data 2
Uncropped gels and autoradiographies shown in Figure 3A–C and quantified in Figure 3B–D.
- https://cdn.elifesciences.org/articles/80497/elife-80497-fig3-data2-v1.pdf
-
Figure 3—source data 3
Raw gels and autoradiographies shown in Figure 3A–C and quantified in Figure 3B–D.
- https://cdn.elifesciences.org/articles/80497/elife-80497-fig3-data3-v1.zip
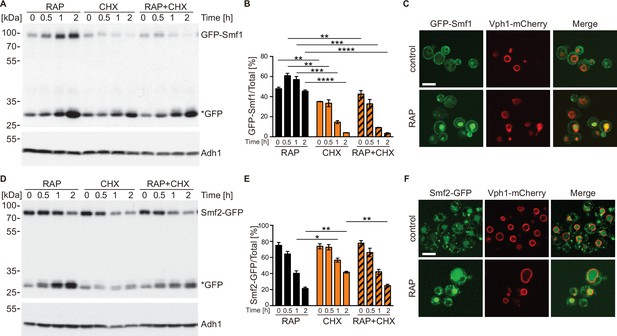
TORC1 regulates natural resistance-associated macrophage protein (NRAMP) transporter levels.
(A) GFP-Smf1 expressing cells were cultivated for 5–6 hr in a synthetic medium devoid of manganese sulfate before being treated with 200 ng/ml rapamycin (RAP), 25 µg/ml cycloheximide (CHX), or both compounds (RAP + CHX) for the indicated times. GFP-Smf1 and cleaved GFP (*GFP) protein levels were analyzed by immunoblotting using an anti-GFP antibody. Alcohol dehydrogenase (Adh1) protein levels, probed with anti-Adh1 antibodies, served as a loading control. (B) Quantification of GFP-Smf1. Percentages of GFP-Smf1 relative to the total GFP signal (GFP-Smf1 + GFP) are plotted. Data represent means ± SEM of independent experiments (n=3). Statistical analysis: unpaired two-tailed t-test. *p<0.05; **p<0.01, ***p<0.001, ****p<0.0001. (C) Microscopic analysis of GFP-Smf1 localization. Cells co-expressing GFP-Smf1 and the vacuolar marker Vph1-mCherry were grown exponentially in a manganese-free medium for 5–6 hr, then treated with 200 ng/ml rapamycin for 2 hr. Scale bar represents 5 µm. (D–E) Cells expressing Smf2-GFP from its endogenous locus were grown, treated, and processed as in (A). (F) Microscopic analysis of Smf2-GFP localization. Cells co-expressing Smf2-GFP and the vacuolar marker Vph1-mCherry were cultivated, treated, and examined as in (C).
-
Figure 4—source data 1
Quantification of blots for graphs shown in Figure 4B–E.
- https://cdn.elifesciences.org/articles/80497/elife-80497-fig4-data1-v1.xlsx
-
Figure 4—source data 2
Uncropped blots shown in Figure 4A–D and quantified in Figure 4B–E.
- https://cdn.elifesciences.org/articles/80497/elife-80497-fig4-data2-v1.pdf
-
Figure 4—source data 3
Raw blots shown in Figure 4A–D and quantified in Figure 4B–E.
- https://cdn.elifesciences.org/articles/80497/elife-80497-fig4-data3-v1.zip
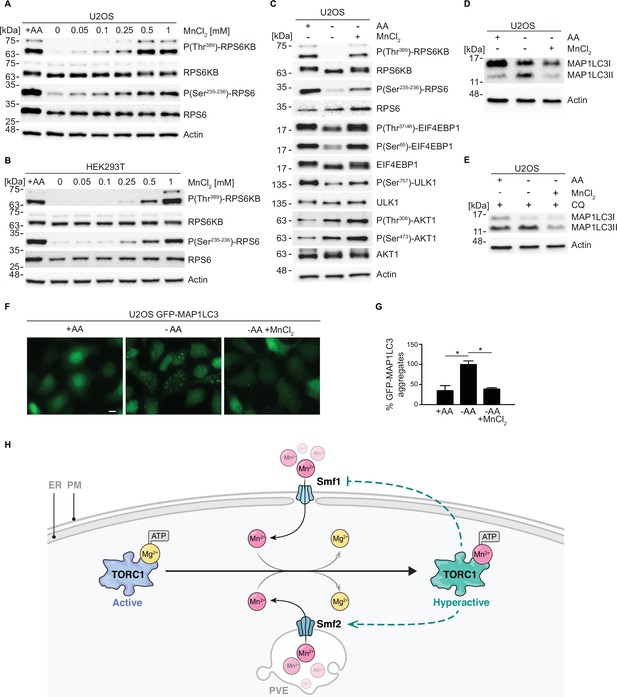
MnCl2 maintains mTORC1 activity during starvation in human cells.
(A–B) Human U2OS (A) and HEK293T (B) cells were starved for all amino acids and supplemented with increasing concentrations of MnCl2 as indicated for 2 hr. Phosphorylation of the mTORC1 downstream targets RPS6KB (ribosomal protein S6 kinase B) and RPS6 (ribosomal protein S6) was assessed by immunoblot analysis. A control with cells incubated in the presence of all proteinogenic amino acids (+AA) was included as a positive control. (C) Human U2OS were starved for all amino acids (-AA) and supplemented with 0.35 mM of MnCl2 for 2 hr. Phosphorylation of RPS6KB, RPS6, EIF4EBP1, ULK1, and AKT1 was assessed by immunoblot analysis. A control with cells incubated in the presence of all proteinogenic amino acids (+AA) was included as a positive control. (D–E) Human U2OS cells were treated as in (C) for 4 hr either in the absence (D) or the presence (E) of chloroquine (CQ). Autophagic marker MAP1LC3I/II was then analyzed by immunoblot. (F–G) GFP-MAP1LC3 expressing U2OS cells were incubated as in (D). GFP-MAP1LC3 aggregation was assessed by confocal microscopy (F) and quantified using ImageJ software (G). Scale bar indicates 10 µm. Values were normalized to -AA condition. Graphs represent mean ± SD, with n=3 (*p<0.05, ANOVA followed by Tukey’s test). (H) Model of Mn2+-driven TORC1 activation. The Smf1/2 natural resistance-associated macrophage protein (NRAMP) transporter-dependent increase in cytoplasmatic Mn2+ levels favors TORC1-Mn2+ binding and ATP coordination, leading to TORC1 hyperactivation. NRAMP transporters are part of a feedback control mechanism impinged by TPRC1 (dashed lines).
-
Figure 5—source data 1
Quantification of microscopy images for graph shown in Figure 5G.
- https://cdn.elifesciences.org/articles/80497/elife-80497-fig5-data1-v1.xlsx
-
Figure 5—source data 2
Uncropped blots shown in Figure 5A–E.
- https://cdn.elifesciences.org/articles/80497/elife-80497-fig5-data2-v1.pdf
-
Figure 5—source data 3
Raw blots shown in Figure 5A–E.
- https://cdn.elifesciences.org/articles/80497/elife-80497-fig5-data3-v1.zip
Additional files
-
Supplementary file 1
RTG1-3 target genes down-regulated in pmr1∆ cells.
- https://cdn.elifesciences.org/articles/80497/elife-80497-supp1-v1.docx
-
Supplementary file 2
Plasmids used in this study.
- https://cdn.elifesciences.org/articles/80497/elife-80497-supp2-v1.docx
-
Supplementary file 3
Yeast strains used in this study.
- https://cdn.elifesciences.org/articles/80497/elife-80497-supp3-v1.docx
-
MDAR checklist
- https://cdn.elifesciences.org/articles/80497/elife-80497-mdarchecklist1-v1.pdf