Neutral amino acid transporter SLC38A2 protects renal medulla from hyperosmolarity-induced ferroptosis
Figures
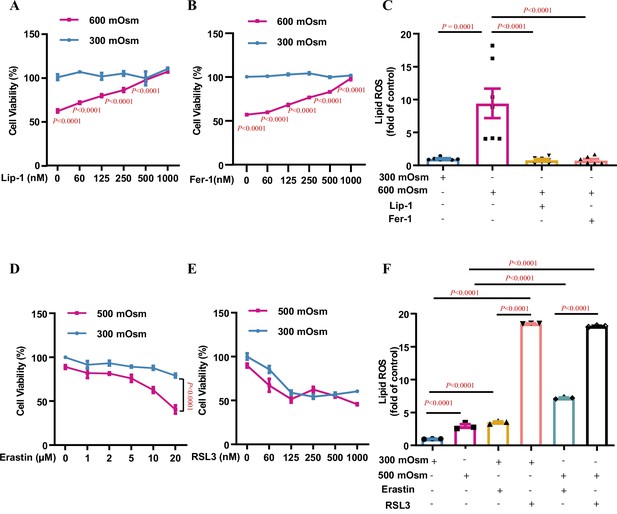
Ferroptosis is the major type of regulated cell death in inner medullary collecting duct (IMCD) cells under hyperosmotic stress.
mIMCD3 cells were treated with various doses of the ferroptosis inhibitors and inducers for 24 h, with or without hyperosmotic exposure in the final 12 h. (A and B) The 3-(4,5-dimethylthiazol-2-yl)-2,5-diphenyltetrazolium bromide (MTT) reduction assay showing the ferroptosis inhibitor liproxstatin-1 (Lip-1) (A) and ferrostatin-1 (Fer-1) (B) increased the cell viability under hyperosmotic condition (600 mOsm) in a dose-dependent manner. n=4. (C) Flow cytometry assay showing that Lip-1 (1 μM) and Fer-1 (1 μM) completely abolished hyperosmolarity-elicited lipid reactive oxygen species (ROS) production. n=7. (D) The system Xc− inhibitor erastin worsened hyperosmolarity-induced mIMCD3 cell death in a dose-dependent manner. Note: Erastin slightly reduced the viability of mIMCD3 cells exposed to isosmolarity. n=4. (E) The GPX4 inhibitor RSL3 induced mIMCD3 cell death under either isosmotic or hyperosmotic conditions. A slight difference in cell viability was found between the two groups. n=4. (F) Flow cytometry analysis results showing that RSL3 caused comparable production of lipid ROS in mIMCD3 cells treated with isosmolarity or hyperosmolarity. Compared to erastin, RSL3 was more potent in promoting lipid ROS production. n=3. Data are means ± SEM; two-tailed Student’s t-test for A and B; one-way ANOVA tests for C and F; two-way ANOVA tests for D and E. See numerical source data in Figure 1—source data 1.
-
Figure 1—source data 1
Numerical source data for Figure 1.
- https://cdn.elifesciences.org/articles/80647/elife-80647-fig1-data1-v2.zip
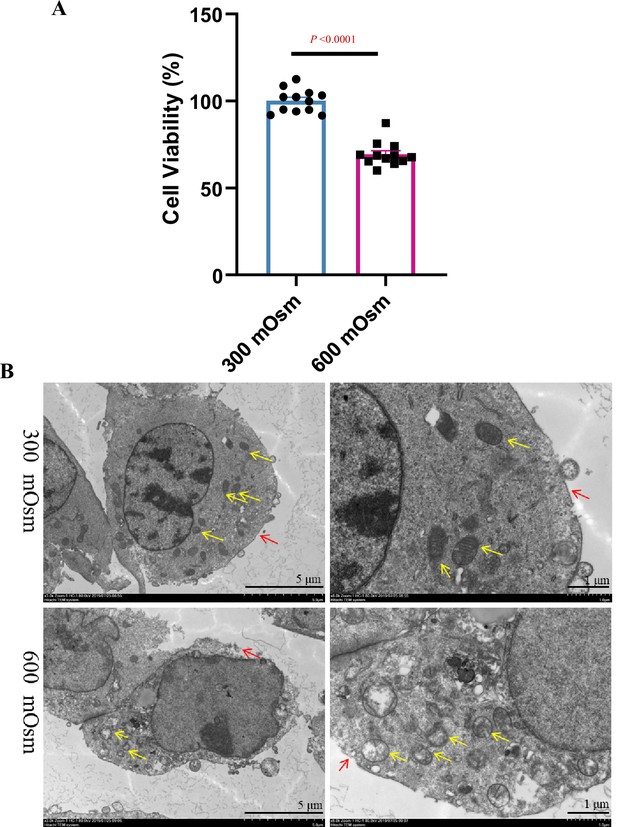
Hyperosmolarity treatment leads to reduced cell viability and severe mitochondrial damage.
(A) mIMCD3 cells were treated with isosmotic (300 mOsm) or hyperosmotic (600 mOsm) medium for 12 h. MTT assay showed that cell viability was significantly reduced under hyperosmotic conditions. (B) Effect of hyperosmolarity on the mitochondrial ultrastructure of mIMCD3 cells as assessed by transmission electron microscopy. The yellow arrows indicate the mitochondria, and red arrows indicate the cell membrane. After hyperosmotic treatment (600 mOsm) for 12 h, the mitochondrial cristae disappeared and were fragmented, and most mitochondria became smaller and shrunk. A broken cell membrane was frequently evident. Scale bars = 5 μm (left panels) and 1 μm (right panels). n=12. Data are means ± SEM; two-tailed Student’s t-test for A. See numerical source data in Figure 1—figure supplement 1—source data 1.
-
Figure 1—figure supplement 1—source data 1
Numerical source data for Figure 1—figure supplement 1.
- https://cdn.elifesciences.org/articles/80647/elife-80647-fig1-figsupp1-data1-v2.zip
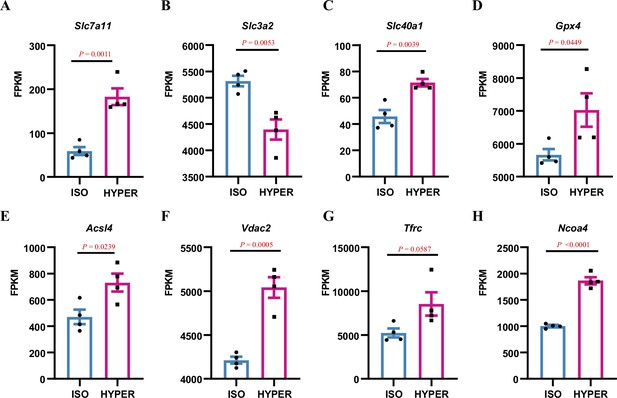
Ferroptosis is involved in the hyperosmolality-induced cell death of inner medullary collecting duct cells.
We analysed the RNA-Seq results and found that the expression of many genes associated with the ferroptotic process was significantly altered after hyperosmotic treatment. The expression of Slc7a11, Slc40a1, Gpx4, Acsl4, Vdac2, Tfrc, and Ncoa4 was significantly upregulated, whereas Slc3a2 expression was downregulated under hyperosmotic conditions (n=4). Slc7a11 and Slc3a2 form system Xc−, which transports glutamate, a raw material for glutathione synthesis, into cells. Slc40a1, also named ferroportin 1 (Fpn1), is an iron transporter involved in iron export from cells and also contributes to transferring iron between maternal and foetal circulations. Transferrin (serum transferrin or lactoferrin) mediates iron uptake through the transferrin receptor (Tfrc), which promotes ferroptosis. Acsl4 mediates the oxidation of polyunsaturated fatty acids, including arachidonic acid, which are required for the lipotoxicity of ferroptosis. Upregulation of Acsl4 expression is a hallmark of ferroptosis. Vdac2 is a voltage-dependent anion channel that transports ions and metabolites and is closely related to ferroptosis. Nuclear receptor coactivator 4 (Ncoa4) can result in the degradation of ferritin and a subsequent increase in the intracellular levels of ferrous iron, ultimately leading to ferroptosis. n=4. FPKM, fragments per kilobase of transcript sequence per million; ISO, isosmolarity; HYPER, hyperosmolarity. Data are means ± SEM; two-tailed Student’s t-test for A–H. See numerical source data in Figure 1—figure supplement 2—source data 1.
-
Figure 1—figure supplement 2—source data 1
Numerical source data for Figure 1—figure supplement 2.
- https://cdn.elifesciences.org/articles/80647/elife-80647-fig1-figsupp2-data1-v2.zip
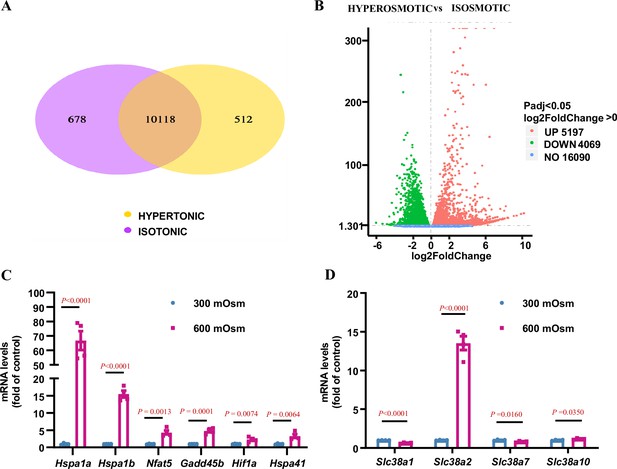
RNA-Seq analysis identifies Slc38a2 as an osmoregulated neutral amino acid transporter in mIMCD3 cells.
mIMCD3 cells were cultured under either isosmotic (300 mOsm) or hyperosmotic (600 mOsm) conditions for 6 h, and mRNA was then extracted for RNA-Seq analysis. (A) Venn diagram showing the overlap of different genes among the hyperosmotic and isosmotic groups. The co-expressed genes between the hyperosmotic and isosmotic groups are also shown. The sum of all the numbers in the circle of Venn diagram represents the total number of differential genes in the comparison combination, and the overlap area represents the number of common differential genes in the combination. (B) The volcano map visually displaying the differential gene distribution of the hyperosmotic and isosmotic groups. The abscissa in the figure represents the fold change of gene expression (log2FoldChange) in the groups, and the ordinate represents the significance level of the difference in gene expression between the treatment and control groups (−log10padj or −log10pvalue). Red dots represent the upregulated genes, and green dots indicate downregulated genes. The volcano map shows that 5197 genes were upregulated, and 4069 genes were downregulated after hyperosmotic treatment. (C) RNA-Seq analysis results showing that hyperosmolarity increased the mRNA levels of previously reported osmoregulatory genes. After hyperosmotic treatment, the expression of Hspa1a, Hspa1b, Hspa41, Nfat5, Gadd45b, and Hif1a was significantly increased. n=4. (D) RNA-Seq results demonstrating that hyperosmolarity significantly induced Slc38a2 mRNA expression in mIMCD3 cells. A slight change in Slc38a1, Slc38a7, and Slc38a10 expression was observed. n=4. Data are means ± SEM; two-tailed Student’s t-test for C–D. See numerical source data in Figure 2—source data 1.
-
Figure 2—source data 1
Numerical source data for Figure 2.
- https://cdn.elifesciences.org/articles/80647/elife-80647-fig2-data1-v2.zip
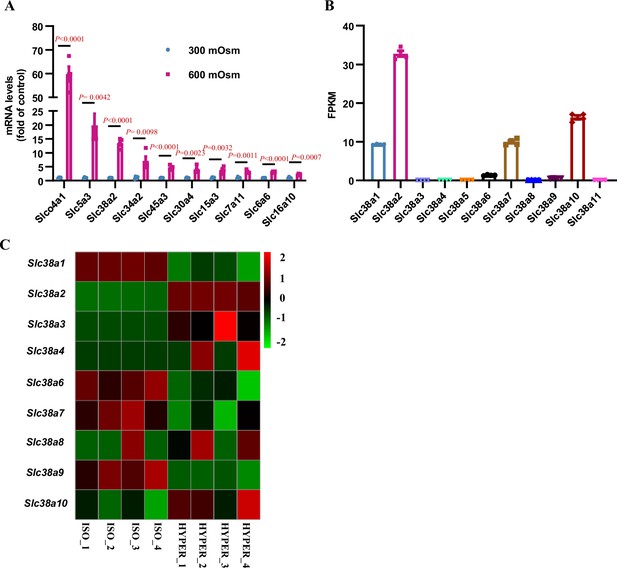
RNA-Seq analysis shows that hyperosmolarity induces Slc38a2 mRNA expression in mIMCD3 cells.
(A) Slc members whose mRNA expression was induced by hyperosmolarity. Hyperosmotic stress significantly upregulated the mRNA expression of Slco4a1, Slc5a3, Slc38a2, Slc34a2, Slc45a3, Slc30a4, Slc15a3, Slc7a11, Slc6a6, and Slc16a10. n=4. (B) RNA-Seq results showing the mRNA abundance of each member of the Slc38 subfamily in mIMCD3 cells under isosmotic conditions (300 mOsm). Slc38a2 represents the most abundant member in the Slc38 subfamily. n=4. (C) Gene heatmap of the Slc38 family showing that Slc38a2 was the major inducible member under hyperosmotic stress (600 mOsm). FPKM, fragments per kilobase of transcript sequence per million; ISO, isosmolarity; HYPER, hyperosmolarity. Data are means ± SEM; two-tailed Student’s t-test for A. See numerical source data in Figure 2—figure supplement 1—source data 1.
-
Figure 2—figure supplement 1—source data 1
Numerical source data for Figure 2—figure supplement 1.
- https://cdn.elifesciences.org/articles/80647/elife-80647-fig2-figsupp1-data1-v2.zip
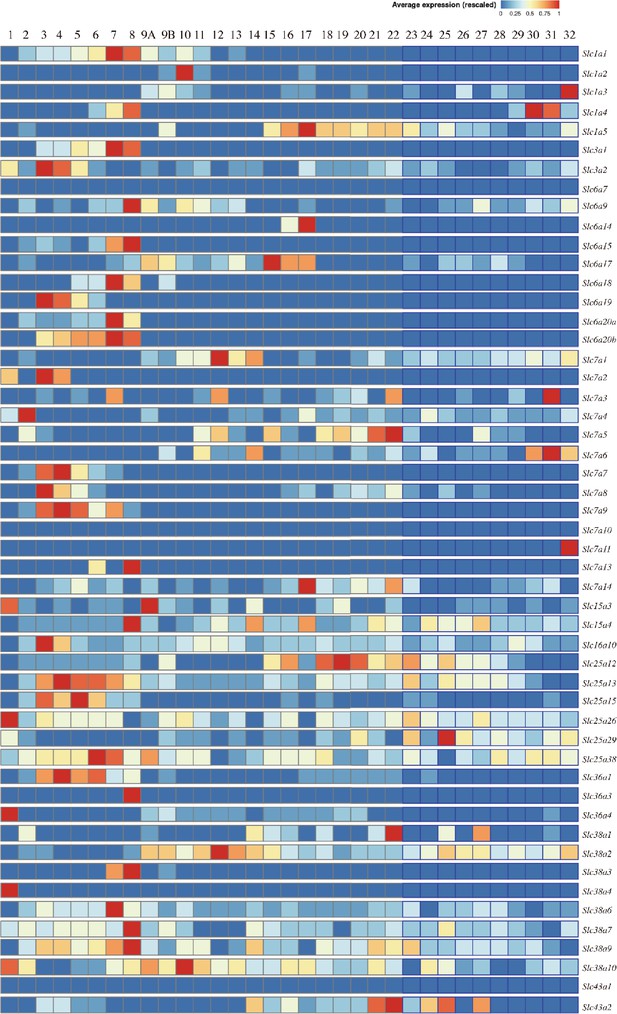
Expression and distribution of amino acid transporters in the juxtamedullary and cortical nephron and renal collecting duct.
The heat map shows the gene expression of 51 amino acid transporters in epithelial cells along the nephron and collecting duct. The nephrons and collecting ducts were subdivided into 32 segments (cell types) (data from KidneyCellExplorer, https://cello.shinyapps.io/kidneycellexplorer/; ). The numbers indicate the histological structure or cell type of the kidneys in both sexes as follows: (1) glomerular podocytes (visceral epithelium); (2) glomerular parietal epithelium; (3) segment 1 of the proximal tubule – female; (4) segment 1 of the proximal tubule – male; (5) segment 2 of the proximal tubule – female; (6) segment 2 of the proximal tubule – male; (7) segment 3 of the proximal tubule – female; (8) segment 3 of the proximal tubule – male; (9A) LOH, thin descending limb of the inner stripe of the outer medulla of the cortical nephron; (9B) LOH, thin descending limb of the inner stripe of the outer medulla of the juxtamedullary nephron; (10) upper LOH, thin descending limb of the inner medulla of the juxtamedullary nephron; (11) lower LOH, thin descending limb of the inner medulla of the juxtamedullary nephron; (12) lower LOH, thin limb of the inner medulla of the juxtamedullary nephron; (13) lower LOH, thin limb of the inner medulla of the juxtamedullary nephron; (14) upper LOH, thin ascending limb of the inner medulla of the juxtamedullary nephron; (15) distal straight tubule of the inner stripe of the outer medulla (syn: thick ascending limb of the LOH); (16) distal straight tubule of the outer stripe of the outer medulla and cortex (syn: thick ascending limb of LOH); (17) macula densa; (18) distal convoluted tubule; (19) nephron-connecting tubule; (20) principal-like cells of nephron-connecting tubules; (21) intercalated type non-A non-B cells of the nephron connecting tubule; (22) intercalated type A cells of the nephron connecting the tubule and the cortical collecting duct; (23) principal-like cell of the cortical collecting duct; (24) intercalated type B cells in the cortical collecting duct; (25) intercalated type A cells of the outer medullary collecting duct; (26) principal cell of the outer medullary collecting duct; (27) intercalated type A cells of the inner medullary collecting duct; (28) principal cell of the inner medullary collecting duct type 1; (29) principal cell of the inner medullary collecting duct type 2; (30) principal-like cells of the deep inner medullary collecting duct type 1; (31) cells of the deep inner medullary collecting duct type 2; and (32) deep medullary epithelium of the pelvis.
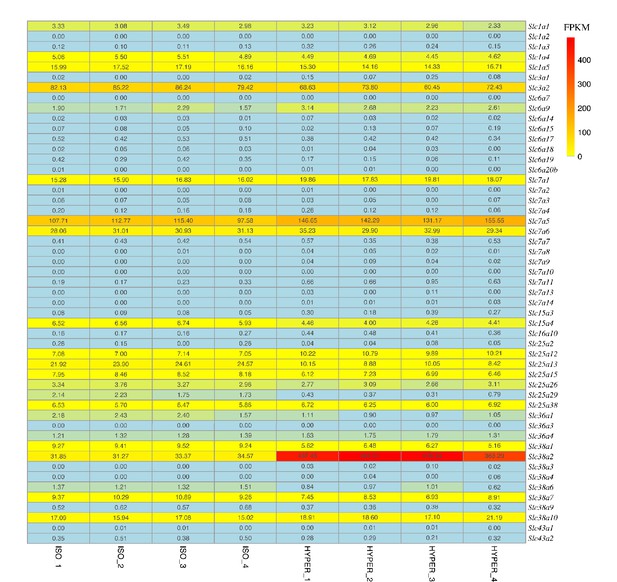
RNA-Seq analysis identifies Slc38a2 as an osmoregulated amino acid transporter in mIMCD3 cells.
The 51 amino acid transporters in mIMCD3 cells were further screened and analysed using our RNA-Seq dataset. The heat map shows the expression changes of fragments per kilobase of transcript sequence per million (FPKM) values of 51 amino acid transporter genes under normal osmotic (300 mOsm) and hyperosmotic (600 mOsm) conditions. Among them, Slc38a2 was the amino acid transporter most significantly induced by hyperosmolarity. ISO, isosmolarity; HYPER, hyperosmolarity.
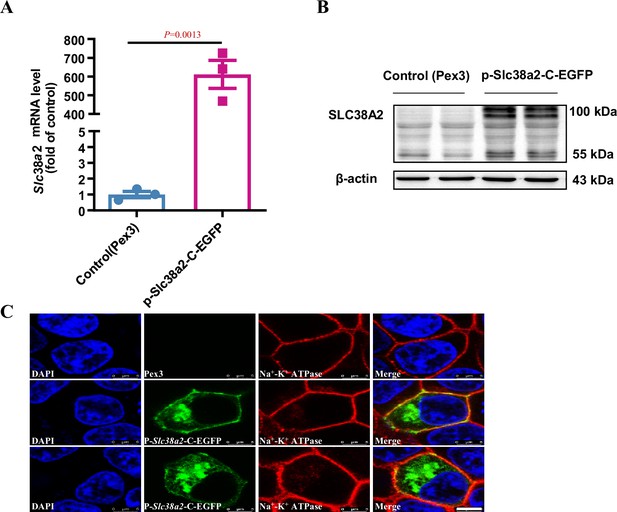
SLC38A2 protein is expressed in the cell membrane and cytoplasm.
The Slc38a2-C-EGFP plasmid was transfected into 293T cells. (A) Real-time PCR analysis results showing a marked increase in Slc38a2 mRNA in the cells transfected with the Slc38a2-C-EGFP expression vector. n=3. (B) Western blotting analysis results demonstrating a significant increase in SLC38A2 protein expression in the cells transfected with the Slc38a2-C-EGFP expression vector. (C) Immunofluorescence analysis results showing that SLC38A2 was mainly expressed in the cell membrane and cytoplasm. The nucleus was stained blue with DAPI. The green colour represents EGFP fluorescence, indicating the subcellular location of SLC38A2. Immunofluorescence staining of Na+-K+-ATPase (red) indicates its location in the cell membrane. Scale bar = 5 μm. Data are means ± SEM; two-tailed Student’s t-test for A. DAPI, 4',6-diamidino-2-phenylindole. See numerical source data and uncropped western blot images in Figure 2—figure supplement 4—source data 1.
-
Figure 2—figure supplement 4—source data 1
Numerical and uncropped western blot source data for Figure 2—figure supplement 4.
- https://cdn.elifesciences.org/articles/80647/elife-80647-fig2-figsupp4-data1-v2.zip
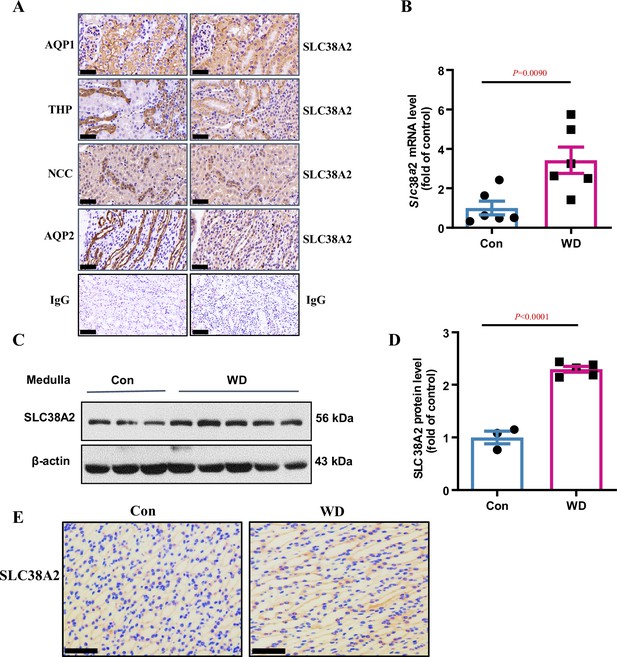
Intrarenal localisation and osmoregulation of SLC38A2 protein in the kidney.
(A) Immunohistochemical analysis results indicating intrarenal localisation of SLC38A2 protein in mice. The results showed that SLC38A2 was expressed at relatively high level in the proximal tubule, thick ascending limb, and collecting duct, with low expression in the distal tubule. AQP1, THP, NCC, and AQP2 are markers for the proximal tubule, distal tubule, thick ascending limb, and collecting duct, respectively. (B–D) Water deprivation increased medullary Slc38a2 mRNA (B) and protein (C) expression in mice. Male C57BL/6 mice were deprived of water for 24 h. Real-time PCR and western blotting analyses were used to measure Slc38a2 mRNA and protein expression in renal medullas, respectively. SLC38A2 protein levels were semi-quantitated (D). B, n=6; D, n=3–5. (E) Immunohistochemical analysis results showing that SLC38A2 protein expression was induced in renal medullary collecting duct cells. Scale bar = 50 μm. WD, water deprivation. Data are means ± SEM. Two-tailed Student’s t-test for B and D. See numerical source data and uncropped western blot images in Figure 3—source data 1.
-
Figure 3—source data 1
Numerical and uncropped western blot source data for Figure 3.
- https://cdn.elifesciences.org/articles/80647/elife-80647-fig3-data1-v2.zip
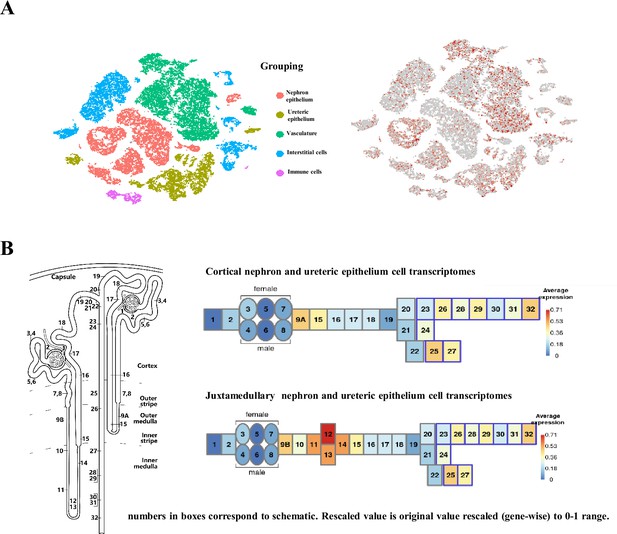
Intrarenal localisation and osmoregulation of Slc38a2 mRNA in the kidney.
(A) Single-cell sequencing results showing that Slc38a2 mRNA was wildly expressed in the renal nephron epithelium, ureteric epithelium, vasculature, immune cells, and interstitial cells. (B) Segmental expression of Slc38a2 in the cortical and juxtamedullary nephron and ureteric epithelium. Slc38a2 was highly expressed in the ascending and descending branches of the loop of Henle and the collecting ducts. The nephron and collecting duct were subdivided into 32 segments as described in detail in Figure 2—figure supplement 2. Data were extracted from KidneyCellExplorer, https://cello.shinyapps.io/kidneycellexplorer/.
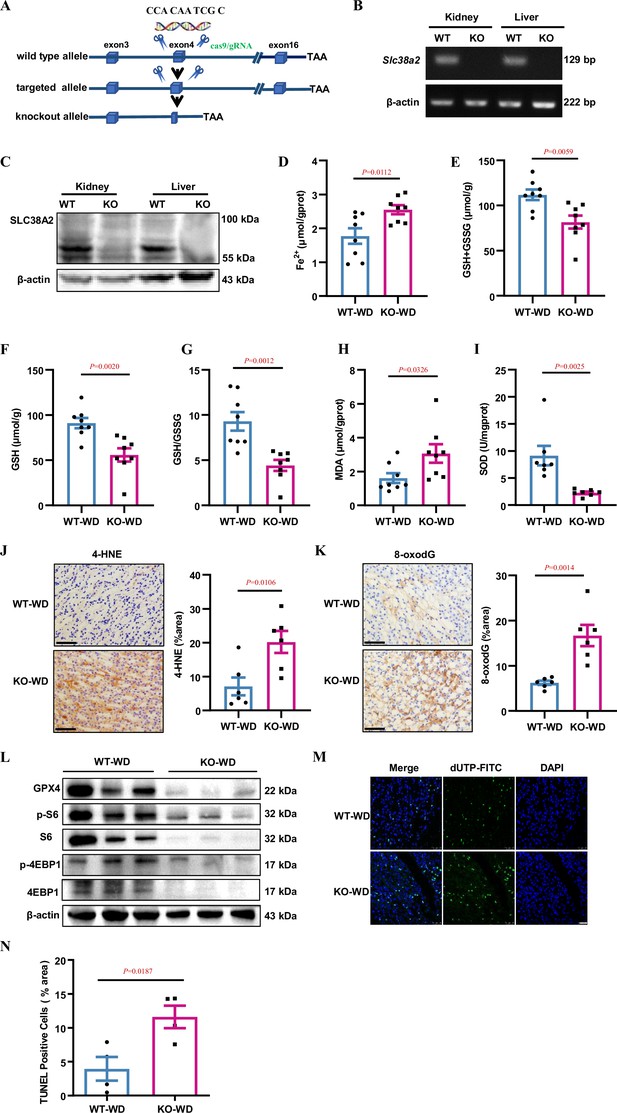
Slc38a2-gene deficiency accelerates renal medullary cell ferroptosis in dehydrated mice.
(A) Strategy for generating global Slc38a2-gene knockout mice using the CRISP/cas9 technique to delete a 10 bp of DNA sequence (5′-CCA CAA TCG C-3′) in the fourth exon of the Slc38a2 gene. (B and C) RT-PCR (B) and western blotting (C) assays showing the absence of Slc38a2 mRNA and protein in the kidney and liver of Slc38a2−/− mice, respectively. (D) Slc38a2−/− mice exhibited significantly increased renal medullary Fe2+ concentration compared to wild-type mice after water deprivation. n=8. (E–G) Water deprivation resulted in a significant decrease in total (E) and reduced (F) GSH levels and GSH/GSSG ratios in the renal medulla of Slc38a2-KO mice. n=8. (H and I) Water deprivation resulted in a significant increase in MDA (H) and a marked reduction in SOD activity (I) in the medulla of Slc38a2−/− mice. n=7–8. (J and K) Immunohistochemical analysis results showing that 4-HNE and 8-oxo-dG levels were significantly increased in the renal medulla of Slc38a2−/− mice compared with that in wild-type mice after water deprivation. n=6. Scale bar = 50 μm. (L) Western blotting assay results demonstrating that water deprivation led to a significant decrease in the levels of total and phosphorylated S6 and 4EBP1 protein expression in the medulla of Slc38a2−/− mice compared with that in wild-type mice. (M) Immunofluorescence analysis results showing that water restriction resulted in a marked increase in cell death (green) in the medulla of Slc38a2−/− mice as assessed by the TUNEL assay. Scale bar = 25 μm. (N) Water restriction resulted in a marked increase in cell death in the medulla of the Slc38a2−/− mice as assessed by the TUNEL assay. n=4. WT, wild-type; KO, Slc38a2−/−; WD, water deprivation; GSH, reductive glutathione; GSSG, oxidised glutathione; MDA, malondialdehyde; SOD, superoxide dismutase. Data are means ± SEM; two-tailed Student’s t-test for D, E, F, G, H, I, J, K, and N. See numerical source data and uncropped western blot images in Figure 4—source data 1.
-
Figure 4—source data 1
Numerical and uncropped western blot source data for Figure 4.
- https://cdn.elifesciences.org/articles/80647/elife-80647-fig4-data1-v2.zip
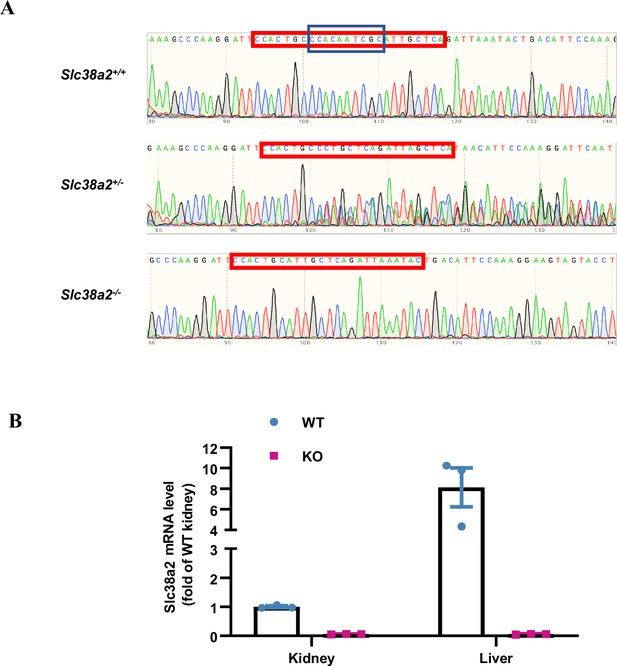
Generation and validation of global Slc38a2-gene knockout mice.
(A) Global Slc38a2-gene knockout mice were generated on C57BL/6 background by deleting a 10-bp sequence (5′-CCA CAA TCG C-3′) in exon 4 using the CRISPR/Cas9 technique, followed by backcrossing to a 129 background for five generations. The genotype was confirmed by DNA sequencing. (B) Real-time PCR analysis results reveal extremely low Slc38a2 mRNA levels in the liver and kidney of Slc38a2−/− mice. n=3. WT, wild type; KO, knockout. See numerical source data in Figure 4—figure supplement 1—source data 1.
-
Figure 4—figure supplement 1—source data 1
Numerical source data for Figure 4—figure supplement 1.
- https://cdn.elifesciences.org/articles/80647/elife-80647-fig4-figsupp1-data1-v2.zip
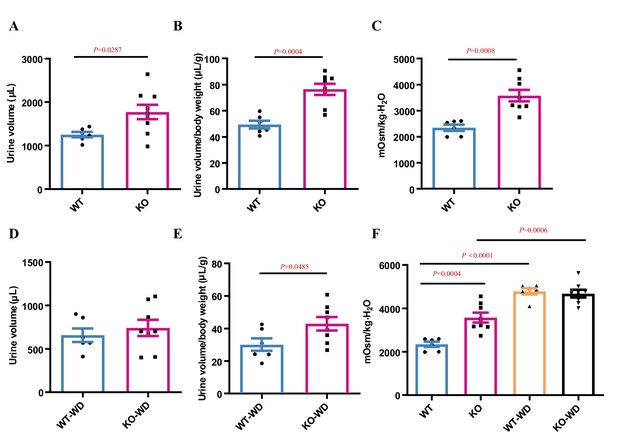
Effect of Slc38a2-gene deficiency on urine output and osmolality in mice.
(A–C) Slc38a2−/− mice exhibited increased urine volume and osmolality compared to wild-type mice. Male mice aged 8–10 wks were used. Urine volume, urine volume to body weight ratio, and urine osmolality were significantly increased in Slc38a2−/− mice. n=6–8. (D–G) The effect of Slc38a2-gene deficiency on urine output and osmolality in mice subjected to water restriction for 24 h. WT, wild type; KO, knockout; WD, water deprivation. n=6–8. Data are means ± SEM; two-tailed Student’s t-test for A–E. One-way ANOVA tests for F. See numerical source data in Figure 4—figure supplement 2—source data 1.
-
Figure 4—figure supplement 2—source data 1
Numerical source data for Figure 4—figure supplement 2.
- https://cdn.elifesciences.org/articles/80647/elife-80647-fig4-figsupp2-data1-v2.zip
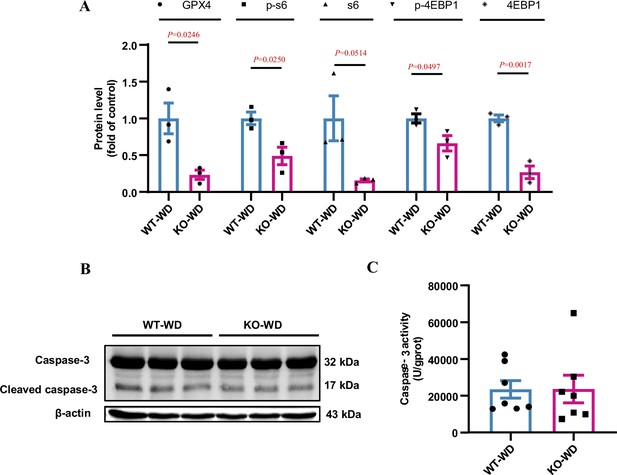
Effect of water deprivation on mTORC1 activity and ferroptosis in the renal medulla of Slc38a2−/− mice.
(A) Water deprivation led to a significant decrease in the levels of total and phosphorylated S6 and 4EBP1 protein expression in the medulla of Slc38a2−/− mice. n=3. (B) The expression of total and cleaved caspase-3 expression in the renal medulla of wild-type and Slc38a2−/− mice under water deprivation. No difference was observed between the two genotypes. (C) Caspase-3 activity in the renal medulla of wild-type and Slc38a2−/− mice under water deprivation. No difference was observed between the two genotypes. n=7. WT, wild-type; KO, Slc38a2−/−; WD, water deprivation. Data are means ± SEM; two-tailed Student’s t-test for A and C. See numerical source data and uncropped western blot images in Figure 4—figure supplement 3—source data 1.
-
Figure 4—figure supplement 3—source data 1
Numerical and uncropped western blot source data for Figure 4—figure supplement 3.
- https://cdn.elifesciences.org/articles/80647/elife-80647-fig4-figsupp3-data1-v2.zip
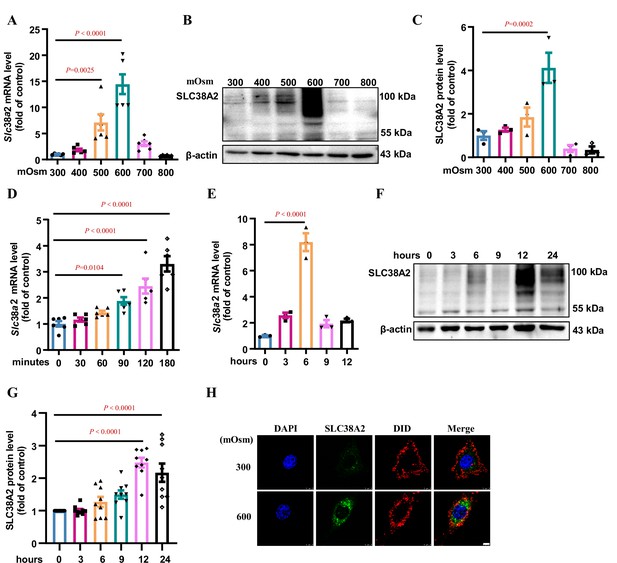
Hyperosmolarity induces SLC38A2 expression in mIMCD3 cells.
(A) Real-time PCR assay showing that hyperosmolarity induced Slc38a2 mRNA expression in mIMCD3 cells in a dose-dependent manner. The cells were incubated with hyperosmotic solutions (400–800 mOsm) for 6 h. n=6. (B–C) Western blotting analysis demonstrating that hyperosmolarity induced SLC38A2 protein expression in mIMCD3 cells in a dose-dependent manner. The cells were exposed to various degrees of hyperosmotic stress (400, 500, 600, 700, and 800 mOsm) for 12 h. SLC38A2 protein expression levels were quantitated (C). n=3. (D–E) Time-dependent effect of hyperosmolarity on Slc38a2 mRNA expression. The cells were treated with a hyperosmotic solution (600 mOsm) at various time intervals. Slc38a2 mRNA expression began to increase at 1.5 h (D) and reached the highest levels at 6 h after hyperosmolarity treatment (E). n=3–6. (F–G) Time-dependent induction of SLC38A2 protein expression by hyperosmolarity. SLC38A2 protein expression began to increase at 6 h (F) and reached the highest level at 12 h after the treatment (G). n=9. (H) Immunofluorescence studies showing that hyperosmolarity markedly induced SLC38A2 expression. DAPI stains the nucleus in blue. DID stains the cell membrane in red. SLC38A2 protein was stained in green. Scale bar = 5 μm. Data are means ± SEM; one-way ANOVA tests for A, C, D, E, and G. DAPI, 4',6-diamidino-2-phenylindole; DID, 1,1'-dioctadecyl-3,3,3',3'-tetramethylindodicarbocyanine,4-chlorobenzenesulfonate salt. See numerical source data and uncropped western blot images in Figure 5—source data 1.
-
Figure 5—source data 1
Numerical and uncropped western blot source data for Figure 5.
- https://cdn.elifesciences.org/articles/80647/elife-80647-fig5-data1-v2.zip
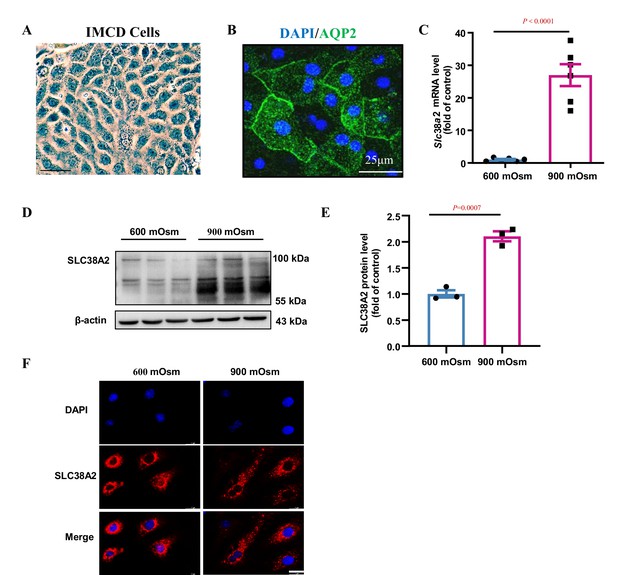
Hyperosmolarity induces SLC38A2 expression in mouse primary inner medullary collecting duct (IMCD) cells.
(A) Morphological examination of mouse primary IMCD cells showing a typical oval shape under the light microscope. Scale bar = 50 μm. (B) Immunofluorescence analysis demonstrating the expression of aquaporin 2 (AQP2) protein (shown in green) on the cell membrane. The nucleus was visualised by staining with DAPI (blue). Scale bar = 25 μm. (C) Real-time PCR indicating hyperosmolarity-induced Slc38a2 mRNA expression. Cultured primary IMCD cells were incubated with hyperosmotic solutions (900 mOsm) for 6 h. n=6. (D–E) Western blot assay showing that hyperosmolarity significantly induced SLC38A2 protein expression in IMCD cells. The IMCD cells were exposed to hyperosmotic stress (900 mOsm) for 12 h. n=3. (F) Immunofluorescence analysis showing that hyperosmolarity upregulated SLC38A2 expression and cell membrane translocation (red). The nucleus was visualised by staining with DAPI (blue). Scale bar = 25 μm. Data are means ± SEM; two-tailed Student’s t-test for C and E. DAPI, 4',6-diamidino-2-phenylindole. See numerical source data and uncropped western blot images in Figure 5—figure supplement 1—source data 1.
-
Figure 5—figure supplement 1—source data 1
Numerical and uncropped western blot source data for Figure 5—figure supplement 1.
- https://cdn.elifesciences.org/articles/80647/elife-80647-fig5-figsupp1-data1-v2.zip
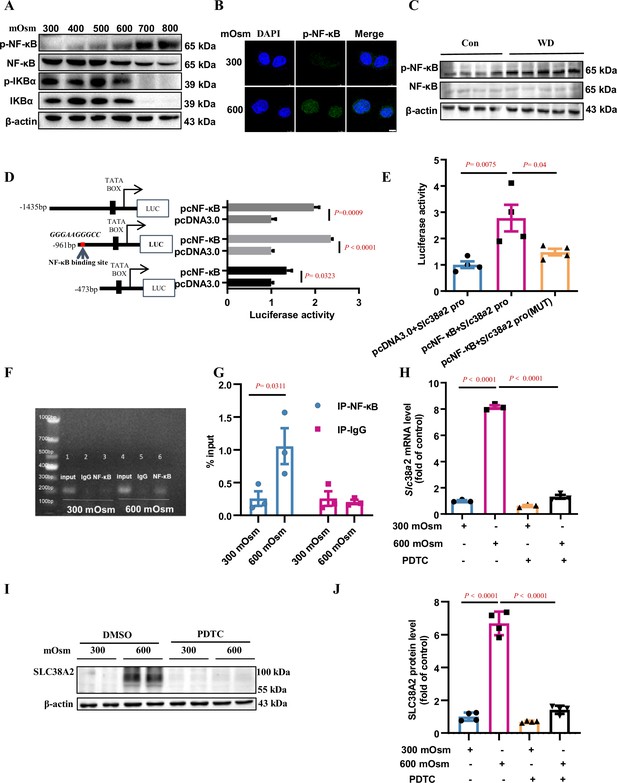
Hyperosmolarity induces SLC38A2 expression by activating the nuclear factor kappa B (NF-κB) pathway.
(A) Hyperosmolarity increased NF-κB activity in mIMCD3 cells in a dose-dependent manner. mIMCD3 cells were treated with hyperosmotic solutions for 12 h. The protein levels of total and phosphorylated NF-κB and IκBα were measured by western blotting. (B) Immunofluorescence assay demonstrated that hyperosmolarity promoted nuclear translocation of NF-κB in mIMCD3 cells. The cells were exposed to hyperosmotic stress (600 mOsm) for 12 h. Scale bar = 8 μm. (C) Water deprivation significantly increased p-NF-κB protein levels and the ratio of p-NF-κB to total NF-κB in the renal medulla of WT C57BL/6 mice. The mice were subjected to water deprivation for 24 h or free access to water. (D) Luciferase reporter assay showed that NF-κB promoted mouse Slc38a2 gene promoter activity. Three promoter fragments (−1435 bp~+124 bp, −961 bp~+124 bp, −473 bp~+124 bp) were obtained by PCR and subcloned into the pGL3-basic vector. n=4. (E) Point mutations defined a consensus sequence containing an NF-κB binding site in mouse Slc38a2 gene promoter. A potential NF-κB binding site between −867 nt and −876 nt was predicted, and the sequence was mutated from 5′-GGG AAG GGC C-3′ to 5′-TCA AGT CTC C-3′. n=4. (F and G) Chromatin immunoprecipitation assay confirmed the binding of NF-κB to the Slc38a2 promoter region. n=3. (H) Real-time PCR analysis showed that inhibition of NF-κB abolished hyperosmolarity-induced Slc38a2 mRNA expression. mIMCD3 cells were treated with the NF-κB inhibitor PDTC (10 μM) for 24 h, with hyperosmotic exposure for the last 12 h. n=3. (I and J) Western blots showed that inhibition of NF-κB blocked hyperosmolarity-induced SLC38A2 protein expression. n=4. Data are means ± SEM; two-tailed Student’s t-test for D; one-way ANOVA tests for E, G, H, and J. Con, control mice with free access to water; WT, wild-type; KO, knockout; WD, water deprivation; PDTC, ammonium pyrrolidinedithiocarbamate. See numerical source data and uncropped western blot images in Figure 6—source data 1.
-
Figure 6—source data 1
Numerical and uncropped western blot source data for Figure 6.
- https://cdn.elifesciences.org/articles/80647/elife-80647-fig6-data1-v2.zip
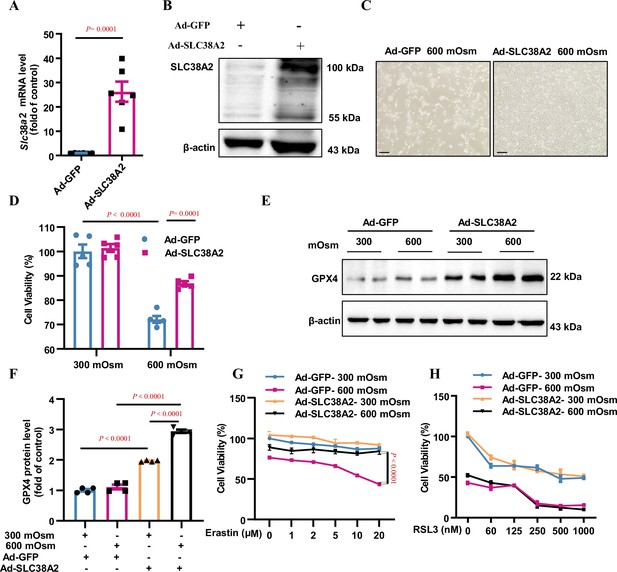
Overexpression of SLC38A2 protects mIMCD3 cells from hyperosmolarity-induced ferroptosis.
(A and B) Adenovirus-mediated SLC38A2 overexpression at both the mRNA (A) and protein (B) levels. mIMCD3 cells were infected with the Ad-SLC38A2 or Ad-GFP (multiplicity of infection [MOI] = 20) for 24 h. Real-time PCR and western blot assays showed that Ad-SLC38A2 infection significantly increased Slc38a2 mRNA (A) and protein (B) expression. n=6. (C) Morphological examination showing the protective effect of SLC38A2 overexpression on hyperosmolarity-induced mIMCD3 cell death. The cells were infected with Ad-SLC38A2 or Ad-GFP for 36 h (MOI = 20), followed by exposure to hyperosmotic stress (600 mOsm) for 12 h. Scale bar = 100 μm. (D) MTT assay demonstrating that SLC38A2 overexpression protects mIMCD3 cells from hyperosmolarity-induced cell death. n=5. (E and F) Western blotting analysis results showing that SLC38A2 overexpression increased GPX4 protein expression under both isosmotic and hyperosmotic conditions. The cells were treated as described in (C). n=4. (G) SLC38A2 protects erastin-induced ferroptosis of mIMCD3 cells under hyperosmotic stress. The cells were infected with Ad-SLC38A2 or Ad-GFP (MOI = 20) for 36 h, followed by treatment with erastin at various doses under hyperosmotic stress (600 mOsm) for 12 h. n=8. (H) The GPX4 inhibitor, RSL3, abolished the protective effect of SLC38A2 overexpression in hyperosmolarity-treated mIMCD3 cells. The cells were treated as described in (G). MTT assay was used to determine cell viability. RSL3 reduced cell viability in the hyperosmotic group to a greater extent than in the isosmotic group. n=3. Data are means ± SEM; two-tailed Student’s t-test for A; one-way ANOVA tests for D and F; two-way ANOVA tests for G and H. See numerical source data and uncropped western blot images in Figure 7—source data 1.
-
Figure 7—source data 1
Numerical and uncropped western blot source data for Figure 7.
- https://cdn.elifesciences.org/articles/80647/elife-80647-fig7-data1-v2.zip
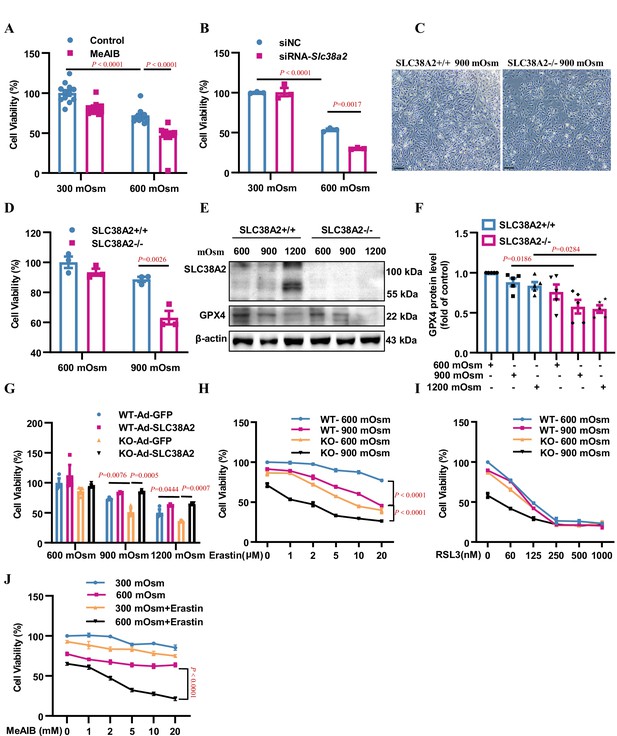
Inhibition or downregulation of SLC38A2 aggravates hyperosmolarity-induced ferroptosis in mIMCD3 and primary medullary collecting duct (MCD) cells.
(A) The System A amino acid transporter inhibitor 2-(methylamino) isobutyric acid (MeAIB) worsened hyperosmolarity-induced cell death. mIMCD3 cells were treated with 5 mM MeAIB for 24 h, with or without hyperosmotic exposure (600 mOsm) in the final 12 h. MTT assay was used to determine cell viability. n=12. (B) SLC38A2 knockdown sensitised mIMCD3 cells to hyperosmolarity-elicited cell death. The cells were transfected with Slc38a2 small interfering RNA (siRNA) for 24 h, followed by exposure to hyperosmotic stress (600 mOsm) for 12 h. n=3. (C) Slc38a2-gene deficiency promoted hyperosmolarity-induced cell death. The primary inner MCD (IMCD) cells of the wild-type (Slc38a2+/+, WT) and knockout (Slc38a2−/−, KO) mice were challenged with hyperosmotic stress (900 mOsm) for 12 h. The cell numbers were examined by light microscopy. Scale bar = 100 μm. (D) MTT assay of primary MCD cells of WT and KO mice. The cells were treated with hypertonicity (900 mOsm) for 12 h. n=3. (E and F) Western blotting analysis results showing that hyperosmolarity caused a more significant decrease in GPX4 levels in SLC38A2−/− IMCD cells than in SLC38A2+/+ IMCD cells under hyperosmotic conditions. n=5. (G) MTT experiment results demonstrating that adenovirus-mediated SLC38A2 overexpression completely reversed hyperosmotic stress-induced cell injury of primary SLC38A2−/− IMCD cells. n=3. (H) The System Xc- inhibitor erastin sensitised hyperosmolality-induced ferroptosis in SLC38A2−/− IMCD cells in a dose-dependent manner. Erastin also enhanced hyperosmotic stress-elicited ferroptosis in SLC38A2+/+ IMCD cells to a lower extent than that in SLC38A2−/− MCD cells. n=3. (I) The GXP4 inhibitor RSL3 abolished the difference in cell viability under hyperosmotic stress between primary SLC38A2+/+ and SLC38A2−/− IMCD cells in a dose-dependent manner. n=3. (J) MTT assay results demonstrating that the SLC38A2 blocker MeAIB sensitised mIMCD3 cells to erastin-induced ferroptosis under hyperosmolarity. mIMCD3 cells were treated with MeAIB at various doses for 24 h, with or without erastin (10 μM) treatment in the presence or absence of hyperosmolarity (600 mOsm) for the last 12 h. n=4. siNC, scramble siRNA control. Data are means ± SEM; one-way ANOVA tests for A, B, D, F, and G; two-way ANOVA tests for H, I, and J. See numerical source data and uncropped western blot images in Figure 8—source data 1.
-
Figure 8—source data 1
Numerical and uncropped western blot source data for Figure 8.
- https://cdn.elifesciences.org/articles/80647/elife-80647-fig8-data1-v2.zip
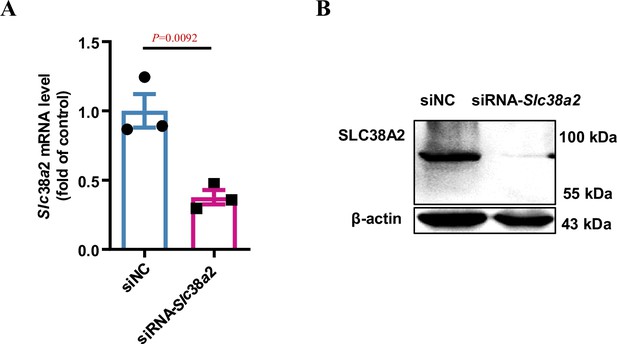
Knockdown of Slc38a2 expression in mIMCD3 cells using a small interfering RNA (siRNA)-mediated approach.
(A) mIMCD3 cells were transfected with an Slc38a2 siRNA. Slc38a2 mRNA expression was significantly decreased by Slc38a2 siRNA. (B) SLC38A2 protein expression was decreased after transfection with Slc38a2 siRNA. n=3. siNC, scramble siRNA control. Data are means ± SEM; two-tailed Student’s t-test for A. See numerical source data and uncropped western blot images in Figure 8—figure supplement 1—source data 1.
-
Figure 8—figure supplement 1—source data 1
Numerical and uncropped western blot source data for Figure 8—figure supplement 1.
- https://cdn.elifesciences.org/articles/80647/elife-80647-fig8-figsupp1-data1-v2.zip
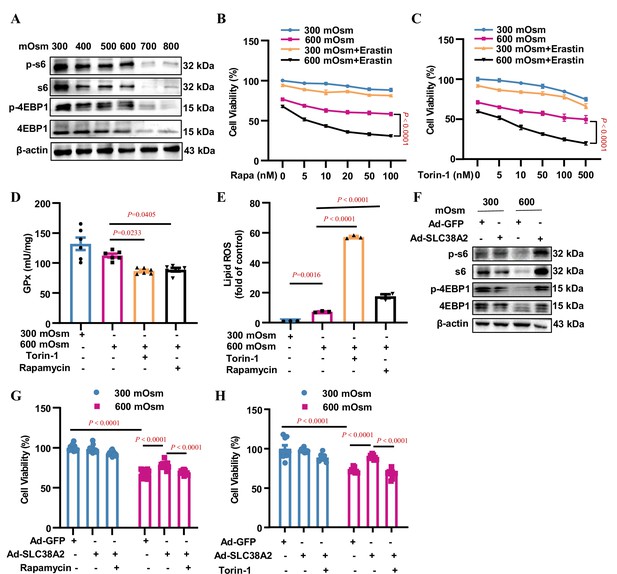
SLC38A2 protects mIMCD3 cells from ferroptosis under hyperosmotic stress by activating mTORC1.
(A) Inhibitory effect of hyperosmolarity on the mTORC1 signalling pathway. mIMCD3 cells were incubated with hyperosmotic solutions for 12 h. Western blotting analysis showed that hyperosmolarity suppressed the total and phosphorylated S6 and 4E-BP1 expression in a dose-dependent manner. (B and C) MTT assay results showing that rapamycin (B) or torin-1 (C) sensitised mIMCD3 cells to erastin-induced ferroptosis under hyperosmolality. mIMCD3 cells were treated with rapamycin or torin-1 at various doses for 24 h. During the last 12 h, the cells were treated with or without erastin (10 μM) in the presence or absence of hyperosmolarity (600 mOsm). n=8. (D) The mTORC1 inhibitors, rapamycin and torin-1, reduced the activity of glutathione peroxidase (GPx) activity. The cells were treated with rapamycin (10 nM) or torin-1 (10 nM) for 24 h, with or without hyperosmotic treatment in the final 12 h. n=6. (E) Cytometry assay showing that rapamycin and torin-1 significantly increased the levels of lipid reactive oxygen species (ROS). n=3. (F) Western blotting assay results demonstrating that SLC38A2 overexpression reversed hyperosmolarity-suppressed expression of total and phosphorylated S6 and 4E-BP1. The cells were infected with Ad-SLC38A2 or Ad-GFP for 36 h followed by hyperosmotic stress for 12 h. (G–H) MTT assay results showing that rapamycin (G) and torin-1 (H) completely abolished the protective effect of SLC38A2 in mIMCD3 cells under hyperosmolarity. After being infected with the adenoviruses, the cells were treated with rapamycin (10 nM) or torin-1 (10 nM) for 24 h with or without hyperosmolarity in the last 12 h. n=9–12. Data are means ± SEM; two-way ANOVA tests for B and C; one-way ANOVA tests for D, E, G, and H. See numerical source data and uncropped western blot images in Figure 9—source data 1.
-
Figure 9—source data 1
Numerical and uncropped western blot source data for Figure 9.
- https://cdn.elifesciences.org/articles/80647/elife-80647-fig9-data1-v2.zip
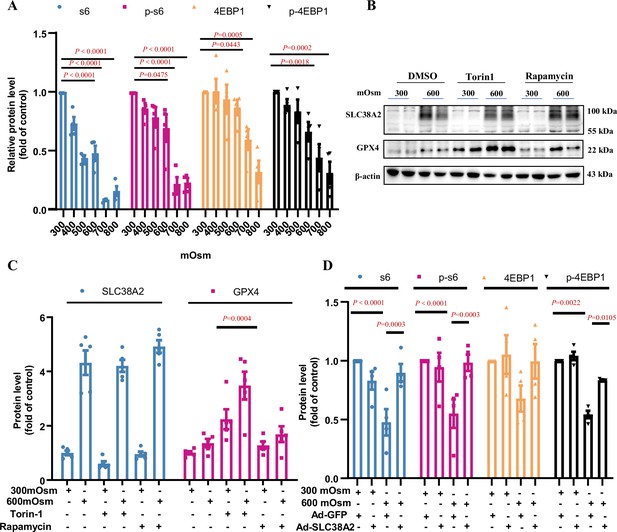
SLC38A2 protects mIMCD3 cells from ferroptosis under hyperosmotic stress by activating mTORC1.
(A) The effect of hyperosmolarity on the expression of mTORC1 downstream effector S6 and 4EBP1. The total and phosphorylated S6 and 4EBP1 expression were inhibited by hyperosmolarity in a dose-dependent manner. n=4. (B and C) Effect of the mTORC1 inhibitors, rapamycin and torin-1, on glutathione peroxidase 4 (GPX4) and SLC38A2 expression in mIMCD3 cells under isosmotic and hyperosmotic conditions. mIMCD3 cells were treated with rapamycin (10 nM) or torin-1 (10 nM) for 24 h, with exposure to isosmotic or hyperosmotic conditions during the final 12 h. The results showed that under hyperosmotic conditions, rapamycin and torin-1 treatment increased GPX4 expression, with little effect on SLC38A2 expression. The induction of GPX4 by rapamycin and torin-1 may reflect a positive feedback regulation of reduced GPX4 activity. n=5. (D) Adenovirus-mediated SLC38A2 overexpression reversed hyperosmolarity-induced suppression of the mTORC1 downstream effector S6 and 4EBP1. n=4. Data are means ± SEM; one-way ANOVA tests for A, C, and D. DMSO, dimethyl sulfoxide. See numerical source data and uncropped western blot images in Figure 9—figure supplement 1—source data 1.
-
Figure 9—figure supplement 1—source data 1
Numerical and uncropped western blot source data for Figure 9—figure supplement 1.
- https://cdn.elifesciences.org/articles/80647/elife-80647-fig9-figsupp1-data1-v2.zip
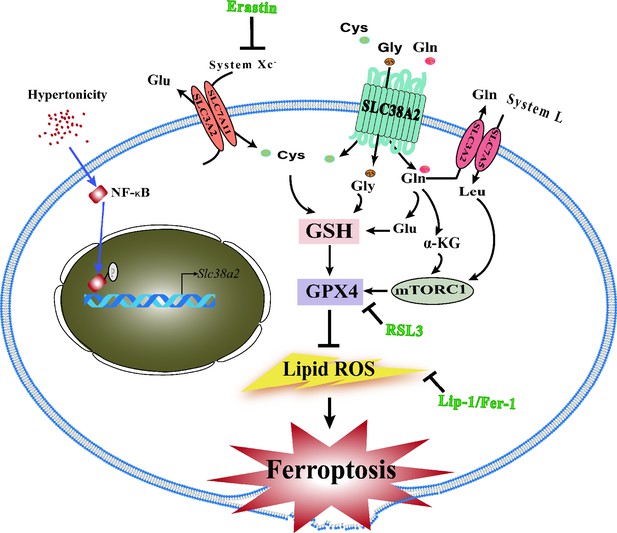
Schematic showing the underlying mechanism by which SLC38A2 promotes the survival of renal medullary collecting duct (MCD) cells under hyperosmotic stress by inhibiting ferroptosis.
(1) Hyperosmotic stress increases lipid reactive oxygen species (ROS) production, which promotes ferroptosis of MCD cells; (2) hyperosmolarity upregulates Slc38a2 mRNA expression by activating the nuclear factor kappa B (NF-κB) pathway; (3) SLC38A2 mediates the uptake of Cys, Gly, and Gln, which may help maintain cellular redox homeostasis and intracellular glutathione peroxidase 4 (GPX4) activity to exert its anti-ferroptotic effect; (4) SLC38A2-mediated uptake of glutamine can strongly activate mTORC1 by facilitating cellular uptake of leucine via the System L amino acid transporter LAT1; (5) SLC38A2 promotes Gln uptake, which is converted into α-ketoglutarate (α-KG) to activate mTORC1.

Effect of water restriction on renal medullary mTOC1 activity.
Water deprivation significantly decreased p-S6 and p-4E-BP1 protein levels in renal medullas of wild-type C57BL/6 mice. The mice were subjected to water deprivation (WD) for 24 h or free access to water (Control).

Intrarenal localisation of SLC38A2 protein in the kidney.
Immunohistochemical study indicating intrarenal localisation of SLC38A2 protein in mouse. The results showed that SLC38A2 was expressed at relatively high level in the proximal tubule, thick ascending limb, and collecting duct, with low expression in the distal tubule. AQP1, THP, NCC, AQP2 are markers for the proximal tubule, distal tubule, thick ascending limb and collecting duct, respectively. IgG was used as a negative control.

Daily urinary excretion of osmotic solutes and amino acids was increased in the Slc38a2- KO mice.
(A) Urine was collected from wild type (WT) and the Slc38a2-knockout (KO) mice (8-10 weeks old, male) for 24 h in the urine metabolic cage. The Slc38a2-KO mice exhibited more urine osmolyte excretion than WT mice. Urine osmotic pressure and urine volume were used to detect urine osmotic solutes (urine volume multiplied by urine osmotic pressure). (B) The Slc38a2-KO mice excreted more total amino acids in the urine than WT mice. Total Amino Acids (T-AA) Colorimetric Assay Kit was used to measure total amino acids in mouse urine (Elabscience, K055-M). Data are means ± SEM; two-tailed Student’s t test was used. P<0.01, n=6-9.
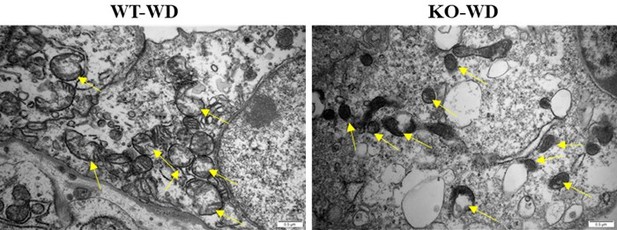
Electron microscope examination showed that ferroptosis of renal medullary cells was aggravated in the Slc38a2-KO mice under water deprivation condition.
The renal medullas of mice were collected and analyzed by electron microscope after 24-h water deprivation. Representative images showed that more severe medullary cell ferroptosis as reflected by the reduction of mitochondria volume and loss and rupture of mitochondrial crest (arrows) was found in the KO mice than that in WT mice under water deprivation. Scale bar = 0.5μm. WD: water deprivation.
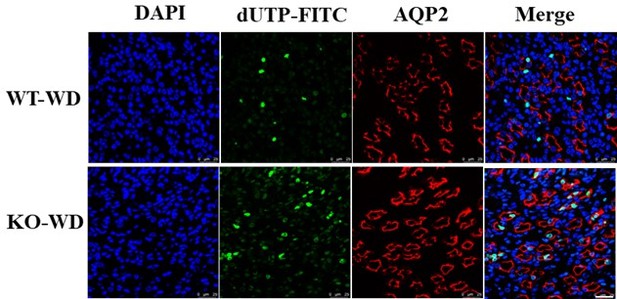
Increased TUNEL-positive cells in renal medullary collecting duct cells of the Slc38a2-KO mice after water deprivation.
Immunofluorescence analysis showing that water restriction resulted in a marked increase in cell death (green) in the medullas of the Slc38a2-/- mice as assessed by the TUNEL assay. TUNEL staining (green) and AQP2 (red) staining showed that the TUNEL-positive cells were mainly renal medullary collecting ducts. AQP2: Aquaporin 2, a marker of renal collecting duct cells. WT: wild-type; KO: Slc38a2-/-; WD: water deprivation. Scale bar=25μm.
Additional files
-
MDAR checklist
- https://cdn.elifesciences.org/articles/80647/elife-80647-mdarchecklist1-v2.pdf
-
Supplementary file 1
Primer pairs used for real-time PCR to determine the mRNA levels of Slc38a2.
- https://cdn.elifesciences.org/articles/80647/elife-80647-supp1-v2.xlsx