MLL3 regulates the CDKN2A tumor suppressor locus in liver cancer
Figures
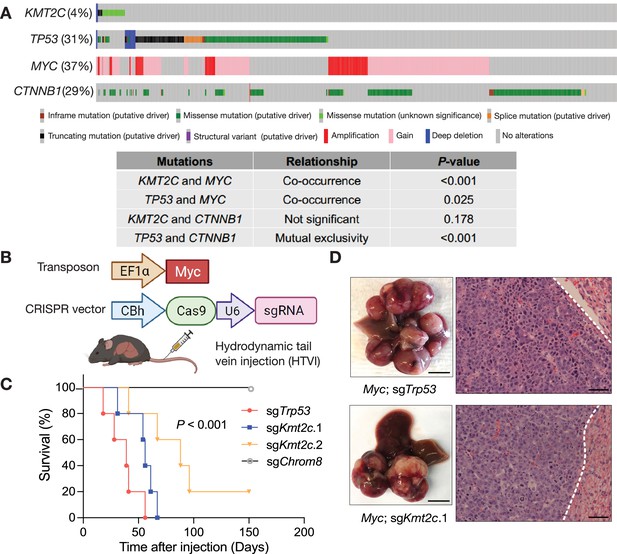
MLL3 constrains Myc-driven liver tumorigenesis.
(A) Oncoprints displaying genomic mutations and deletions of KMT2C and TP53, gains and amplifications of MYC, and activating CTNNB1 mutations in merged publicly available datasets (TCGA, MSK, INSERM, RIKEN, AMC, and MERCi) of 1280 sequenced hepatocellular carcinomas, and the table showing their relationships. p-Values were calculated by Fisher exact tests. (B) Schematic for hydrodynamic tail vein injection (HTVI) of gene delivery into murine livers. Vectors permitting stable expression of Myc transposon (top) and transient expression of Cas9 and single guide RNAs (sgRNAs) targeting putative tumor suppressors (bottom) via sleeping beauty transposase were introduced into hepatocytes by HTVI. (C) Survival curves of mice injected with Myc transposon and pX330 expressing two independent sgRNAs targeting Kmt2c after HTVI (Myc; sgKmt2c.1, n=5; Myc; sgKmt2c.2, n=5). Myc; sgTrp53 (n=5), and Myc; sgChrom8 (n=5) serve as controls. Survival curves were compared using log-rank tests. (D) Representative images (left, liver macro-dissection, scale bar: 0.5 cm; right, H&E staining, scale bar: 100 μm) of mouse liver tumors generated by HTVI delivery of Myc transposon and in vivo gene editing. The dashed lines indicate the boundaries between liver tumors and non-tumor liver tissues.
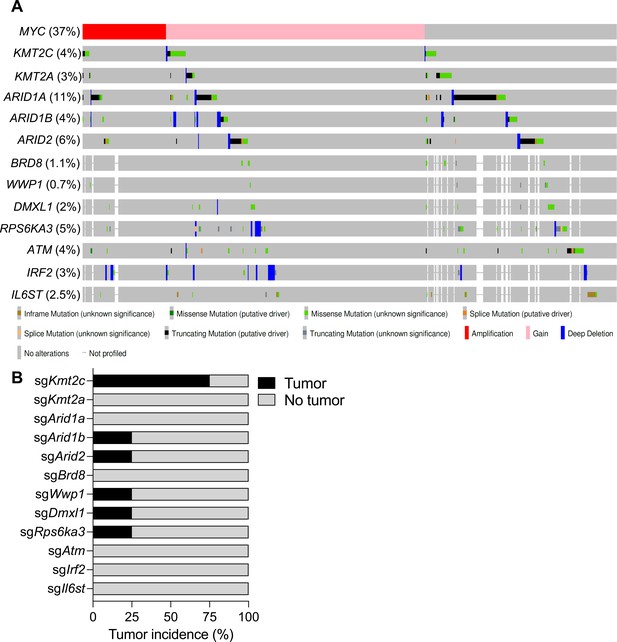
In vivo screen identifies MLL3 as a tumor suppressor in Myc-driven liver cancer.
(A) Oncoprints displaying genomic gains and amplifications of MYC oncogene, and mutations and deletions of 12 candidate tumor suppressor genes in merged publicly available datasets (TCGA, MSK, INSERM, RIKEN, AMC, and MERCi) of 1280 sequenced hepatocellular carcinomas. (B) Liver tumor incidence in mice after hydrodynamic tail vein injection (HTVI) of Myc transposon and single guide RNAs (sgRNAs) targeting each candidate gene (n=4 mice per group).
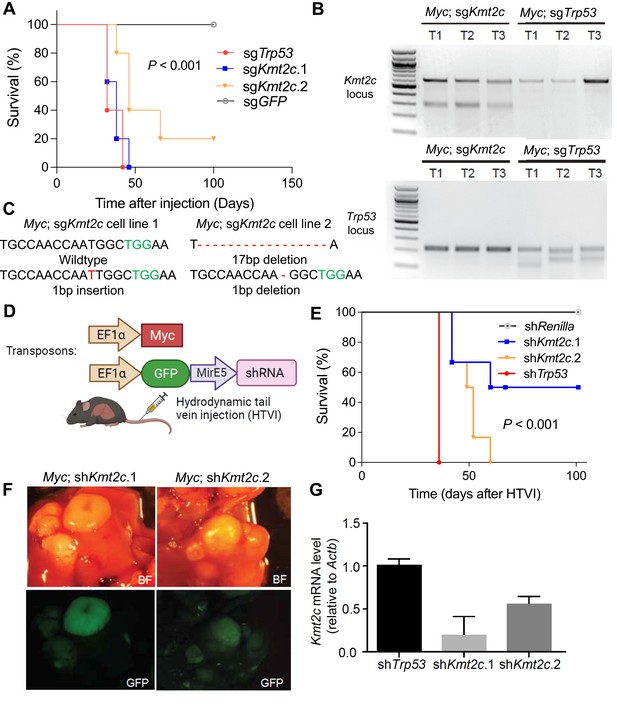
Suppression of Kmt2c by CRISPR or RNAi promotes Myc-driven liver cancer.
(A) Survival curve of a second cohort of mice injected with Myc transposon and pX330 expressing two independent single guide RNAs (sgRNAs) targeting Kmt2c (Myc; sgKmt2c.1, n=5; Myc; sgKmt2c.2, n=5). Myc; sgTrp53 (n=5), and Myc; sgGFP (n=5) served as controls. Survival curves were compared using log-rank tests. (B) Surveyor assay of PCR amplicons flanking predicted Kmt2c and Trp53 CRISPR cleavage sites in Myc; sgKmt2c and Myc; sgTrp53 cells. The ratio of intensity of the lower bands (containing indels) to that of the upper band (untargeted) provides an estimate of the efficiency of CRISPR-Cas9 cleavage at the Kmt2c and Tpr53 loci. (C) Sanger sequencing of the CRISPR-targeted Kmt2c locus from two independently derived cell lines using two different sgKmt2c. The (protospacer adjacent motif)PAM-sequence is indicated in green; indels are indicated in red. (D) Schematic for hydrodynamic tail vein injection (HTVI) for in vivo gene silencing in mouse livers. HTVI was used to introduce transposons enforcing stable expression of Myc and (green fluorescent protein)GFP-linked, constitutive shRNAs(small hairpin RNAs) targeting genes of interest. (E) Survival curve of mice injected with transposon vectors expressing Myc oncogene and two independent shRNAs targeting Kmt2c (Myc; shKmt2c.1, n=6; Myc; shKmt2c.2, n=6), Trp53 (Myc; shTrp53, n=3), or Renilla luciferase (Myc; shRen, n=3). Survival curves were compared using log-rank tests. (F) Representative images of tumor nodules expressing shRNA-linked GFP in mice transduced with Myc; shKmt2c. BF: bright field. (G) Kmt2c mRNA expression measured by qPCR in the indicated tumor tissues. Values are mean ± SD from experimental triplicates in three different tumors of each group.
-
Figure 1—figure supplement 2—source data 1
Original gel for surveyor assays in Figure 1—figure supplement 2B.
- https://cdn.elifesciences.org/articles/80854/elife-80854-fig1-figsupp2-data1-v2.pdf
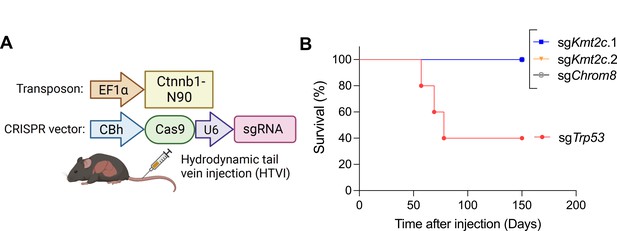
MLL3 loss does not cooperate with CTNNB1 oncogene to drive liver cancer.
(A) Schematic for hydrodynamic tail vein injection (HTVI) of gene delivery into murine livers. Vectors permitting stable expression of constitutively active β-catenin (Ctnnb1-N90) transposon (top) and transient expression of Cas9 and single guide RNAs (sgRNAs) targeting putative tumor suppressors (bottom) via sleeping beauty transposase were introduced into hepatocytes by HTVI. (B) Survival curves of mice injected with Ctnnb1-N90 transposon and pX330 expressing two independent sgRNAs targeting Kmt2c (Myc; sgKmt2c.1, n=5; Myc; sgKmt2c.2, n=5). Myc; sgTrp53 (n=5), and Myc; sgChrom8 (n=5) served as controls.
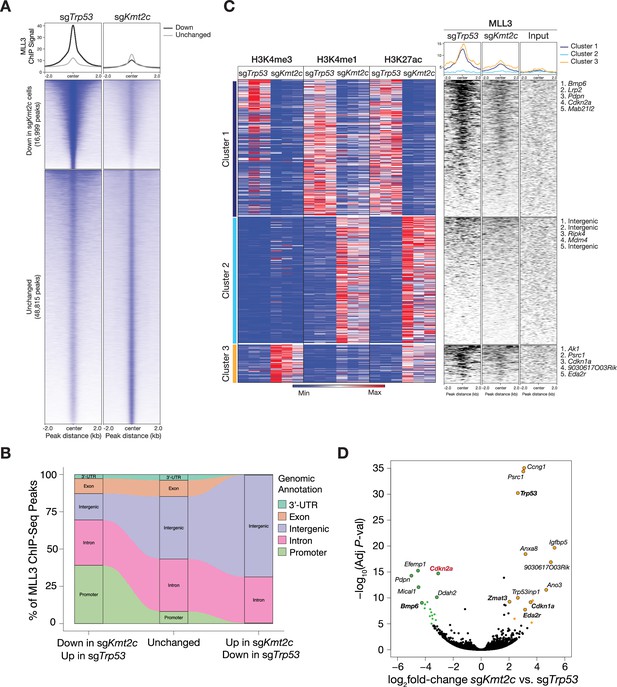
MLL3 disruption alters the chromatin and transcriptional landscape of liver cancer cells.
(A) Tornado plots showing MLL3 chromatin immunoprecipitation-sequencing (ChIP-Seq) signal (peaks) that were down or remained unchanged in Myc; sgKmt2c cells relative to Myc; sgTrp53 cells. Center: transcriptional start site (TSS). (B) Alluvial plot showing the percentages of MLL3 ChIP-Seq peaks in different genomic elements in Myc; sgKmt2c vs Myc; sgTrp53 cells, including 16,999 peaks down, 48,815 peaks unchanged, and 265 peaks up in sgKmt2c cells. Promoter regions were defined as TSS±2 kb. (C) Heatmaps of histone modification ChIP-Seq signals (H3K4me3, H3K4me1, H3K27ac, left panel) and MLL3 ChIP-Seq signal (right panel) at promoter or intergenic regions in three independent Myc; sgKmt2c and Myc; sgTrp53 liver tumor-derived cell lines. Cluster 1: loss of promoter and enhancer activity (loss of H3K4me3, H3K4me1, and H3K27ac); cluster 2: gain of enhancer activity (gain of H3K4me1 and H3K27ac); and cluster 3: gain of promoter activity (increase of H3K4me3). Representative top five loci for each cluster were listed on the right. (D) Volcano plot of differentially expressed genes revealed by RNA-sequencing of three independent Myc; sgKmt2c and Myc; sgTrp53 hepatocellular carcinoma (HCC) cell lines. Genes in sgKmt2c cells with more than twofold expression change and exceeding adjusted p-value<10–5 are color-labeled (orange: upregulated; green: downregulated). Some differentially expressed genes are labeled with gene symbols, and p53 targets are bolded.
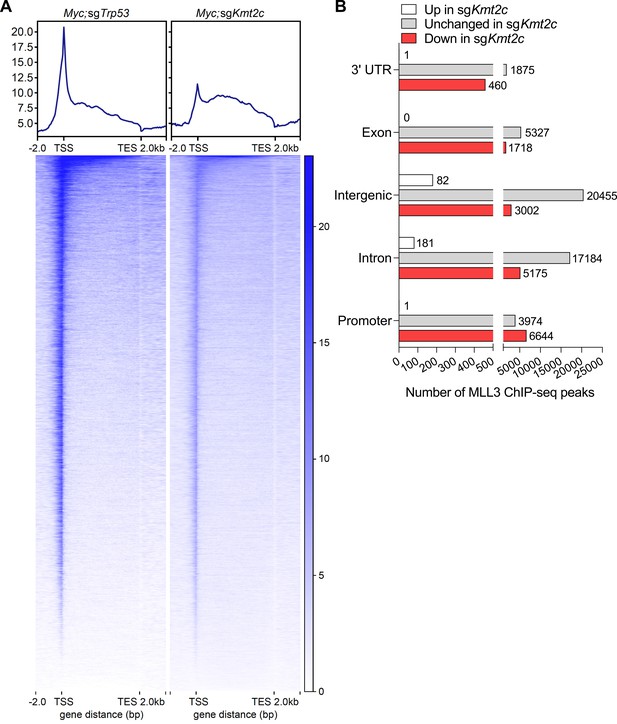
MLL3 deficiency disrupts its binding at promoters in liver cancer cells.
(A) Tornado plots showing MLL3 chromatin immunoprecipitation-sequencing (ChIP-Seq) signals at promoter regions in Myc; sgTrp53 and Myc; sgKmt2c cells. Promoter regions were defined as transcriptional start sites (TSS)±2 kb. TES, transcription end sites. (B) Distribution graph of MLL3 binding peaks in different genomic regions and the changes based on the liver cancer genotypes.
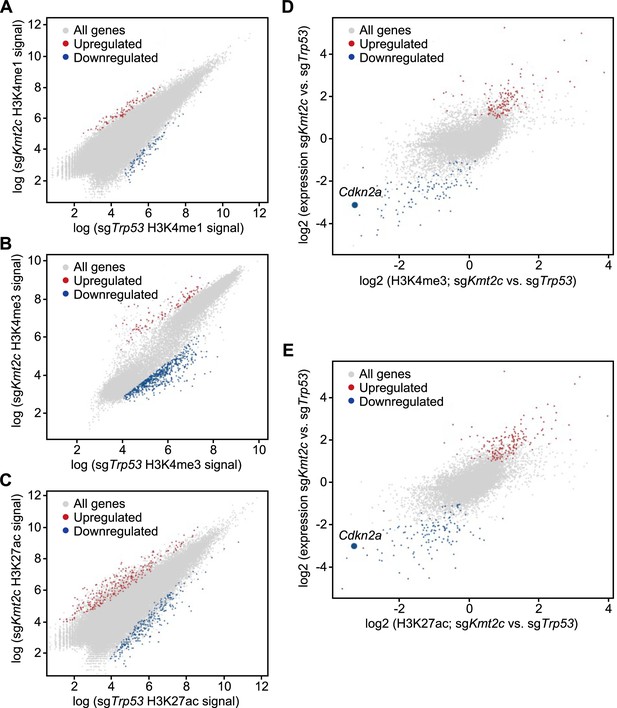
MLL3 disruption impacts transcriptional and histone modification profiles in liver tumors.
(A–C) Scatter plots comparing chromatin immunoprecipitation-sequencing (ChIP-Seq) signals for (A) H3K4me1, (B) H3K4me3, and (C) H3K27ac in Myc; sgKmt2c (y-axis) and Myc; sgTrp53 (x-axis) liver tumor-derived cell lines. (D and E) Scatter plots comparing RNA-seq (y-axis) and ChIP-Seq signals (x-axis) for (D) H3K4me3 and (E) H3K27ac in Myc; sgKmt2c hepatocellular carcinoma (HCC) cell lines.
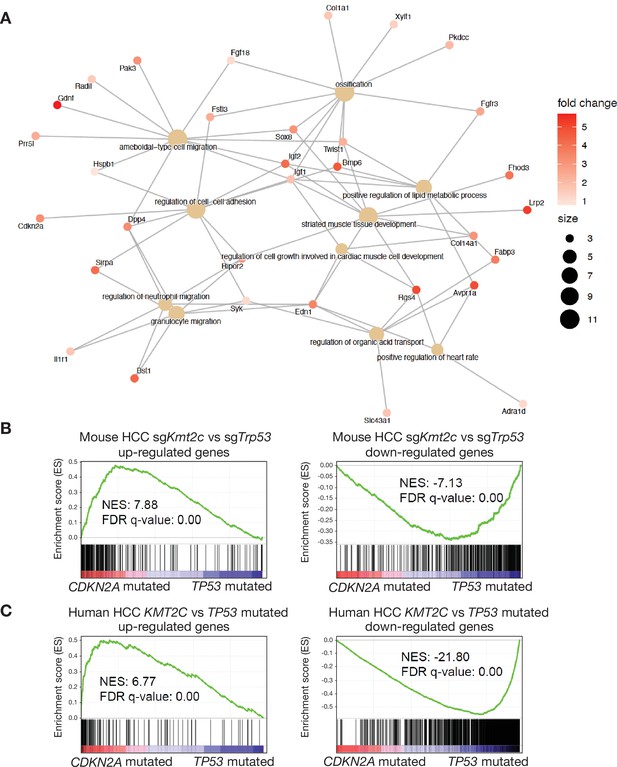
MLL3 regulates specific transcription programs including tumor suppressor CDKN2A.
(A) Network plot showing the major biological processes and related genes directly regulated by MLL3 binding. p-Values and cluster sizes were calculated by the integrative analyses of RNA-seq and MLL3 chromatin immunoprecipitation-sequencing (ChIP-Seq), as detailed in the Materials and methods. (B) Gene set enrichment analysis (GSEA) plots of transcriptional signatures derived from mouse hepatocellular carcinomas (HCCs; Myc; sgKmt2c vs Myc; sgTrp53) against transcriptomics of HCCs with CDKN2A vs TP53 mutations. (C) GSEA plots of transcriptional signatures derived from KMT2C mutated/deleted human HCCs against the ones with CDKN2A mutations or homozygous deletions. HCCs with TP53 mutations were used as the controls for both comparisons. Normalized enrichment scores (NES) and false discovery rate (FDR) q-values were calculated by GSEA.
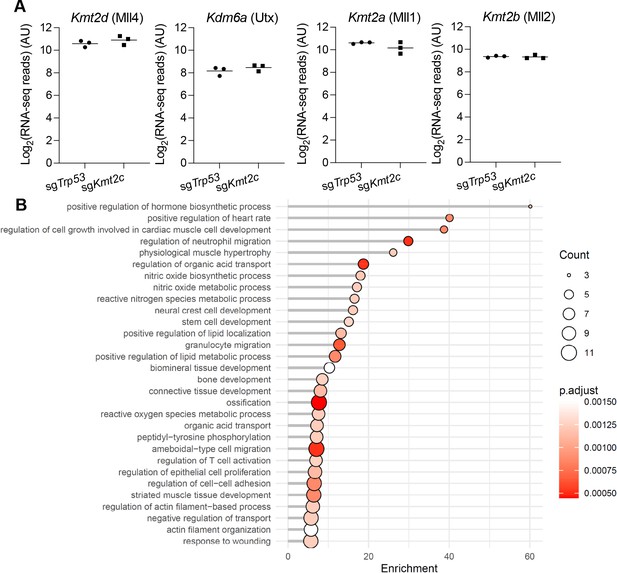
MLL3 loss impacts specific transcription programs.
(A) Log2-transformed RNA-seq reads of Kmt2d, Kdm6a, Kmt2a, and Kmt2b in Myc; sgTrp53 and Myc; sgKmt2c liver cancer cells. (B) Gene ontology enrichment plot listing the biological processes regulated by direct MLL3 binding. Adjusted p-values and cluster sizes were calculated by the integrative analyses of RNA-seq and MLL3 chromatin immunoprecipitation-sequencing (ChIP-Seq), as detailed in Materials and methods.
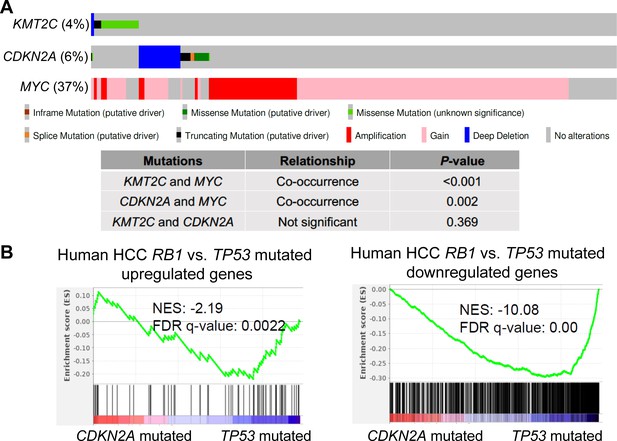
CDKN2A and KMT2C mutations cause similar transcriptional changes in human hepatocellular carcinoma (HCC).
(A) Oncoprints displaying the genomic alterations of MYC (gains and amplifications) and KMT2C and CDKN2A (mutations and deletions) in 1280 human HCCs, and a table showing their relationships. p-Values were calculated by Fisher Exact tests. (B) Gene set enrichment analysis (GSEA) plots of transcriptional signatures from RB1 mutated/deleted human HCCs against the ones with CDKN2A mutations or homozygous deletions. HCCs with TP53 mutations were used as the controls for both comparisons. Normalized enrichment scores (NES) and False discovery rate (FDR) q-values were calculated by GSEA.
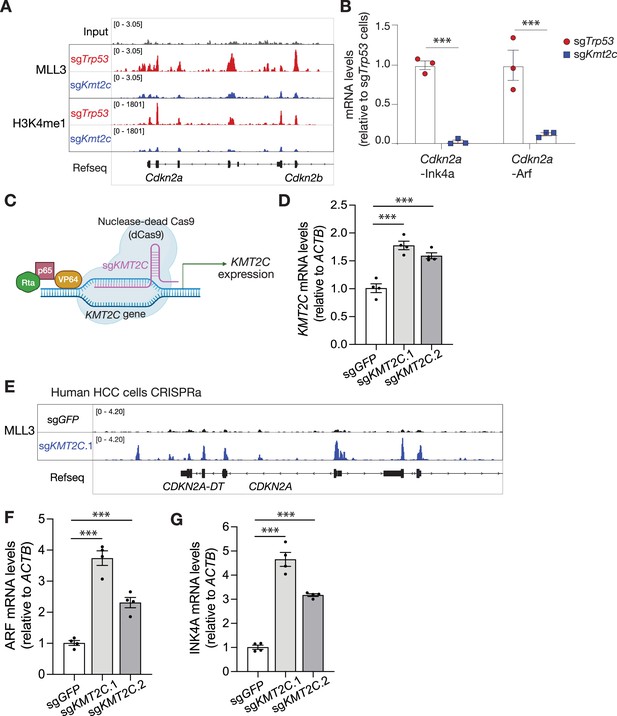
CDKN2A locus is a genomic and transcriptional target of MLL3 in liver cancer.
(A) Genome browser tracks for MLL3 and H3K4me1 chromatin immunoprecipitation-sequencing (ChIP-Seq) in Myc; sgTrp53 (red) and Myc; sgKmt2c (blue) hepatocellular carcinoma (HCC) cell lines at the Cdkn2a locus. (B) qPCR analysis for mRNA expression of Ink4a and Arf from three independent Myc; sgKmt2c and Myc; sgTrp53 HCC lines (n=3 cell lines each genotype). Values are shown as mean ± SD. ***=p<0.001 (unpaired two-tailed t-test). (C) Schematic for CRISPR activation (CRISPRa) system of nuclease-dead Cas9 (dCas9) and VP64-p65-Rta (VPR) guided by sgKMT2C to activate KMT2C expression in human HLE HCC cell line. (D) qPCR analysis for mRNA expression of KMT2C in HLE cells with sgGFP (control) or two different CRISPRa single guide RNAs (sgRNAs) targeting KMT2C (n=4 cell lines each genotype). Each data point represents the average of technical duplicates. Data are shown as mean ± SEM. ***=p<0.001 (one-way ANOVA followed by post-hoc t-tests). (E) Genome browser tracks for MLL3 ChIP-Seq at the CDKN2A locus in HLE cells with sgGFP (control, black) or sgKmt2c.1 (blue). (F and G) qPCR analysis for mRNA expression of (F) INK4A and (G) ARF in HLE cells with sgGFP (control) or sgKMT2C (n=4). Each data point represents the average of technical duplicates. Data are shown as mean ± SEM. ***=p<0.001 (one-way ANOVA followed by post-hoc t-tests).
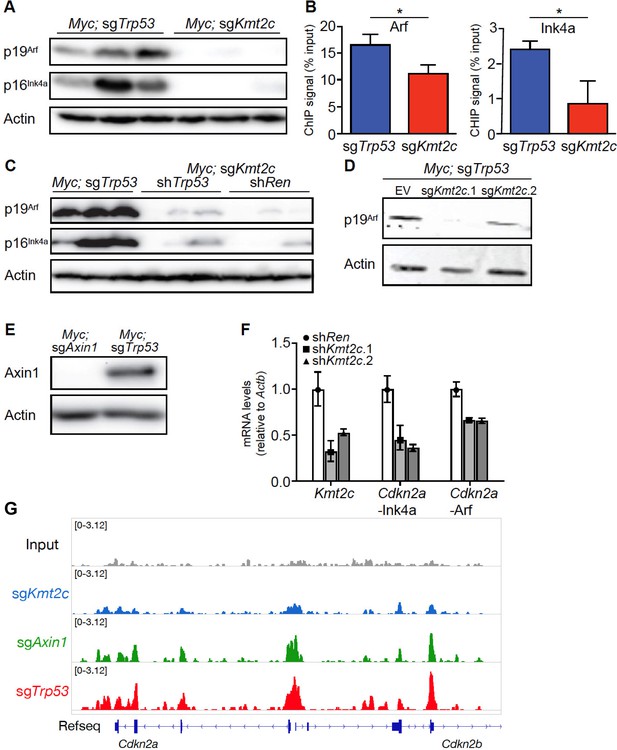
MLL3 directly regulates Cdkn2a expression in liver cancer cells.
(A) Immunoblots for p16Ink4a and p19Arf proteins in three independent Myc; sgKmt2c and Myc; sgTrp53 HCC lines. β-actin was used as loading control. (B) Chromatin immunoprecipitation (ChIP)-qPCR validation of H3K4me3 signals at Arf and Ink4a promoters. Values are mean ± SD from three independent Myc; sgKmt2c; and Myc; sgTrp53 liver tumor-derived cell lines. *=p<0.05 (unpaired two-tailed t-test). (C) Western blot analysis of p16Ink4a and p19Arf proteins in three independent Myc; sgTrp53 and Myc; sgKmt2c cell lines expressing either a p53 shRNA (shTrp53) or a control shRNA (shRen). (D) Western blot analysis of p19Arf in Myc; sgTrp53 cell lines infected with two independent Kmt2c single guide RNAs (sgRNAs; sgKmt2c.1, sgKmt2c.2) or an empty vector (EV) control. (E) Western blot analysis of Axin1 in Myc; sgAxin1 and Myc; sgTrp53 cell lines. (F) qPCR analysis of Kmt2c and Cdkn2a transcripts in Myc; sgAxin1 cell lines expressing two independent Kmt2c shRNAs or a control shRNA. Values are mean ± SD from experimental triplicates. For all western blots, β-actin was used as loading control. (G) Genome browser tracks for MLL3 ChIP-sequencing (ChIP-Seq) in Myc; sgKmt2c (blue), Myc; sgAxin1 (green), and Myc; sgTrp53 (red) HCC cell lines at the Cdkn2a locus.
-
Figure 4—figure supplement 1—source data 1
Original western blots for Figure 4—figure supplement 1A,C,D,E.
- https://cdn.elifesciences.org/articles/80854/elife-80854-fig4-figsupp1-data1-v2.pdf
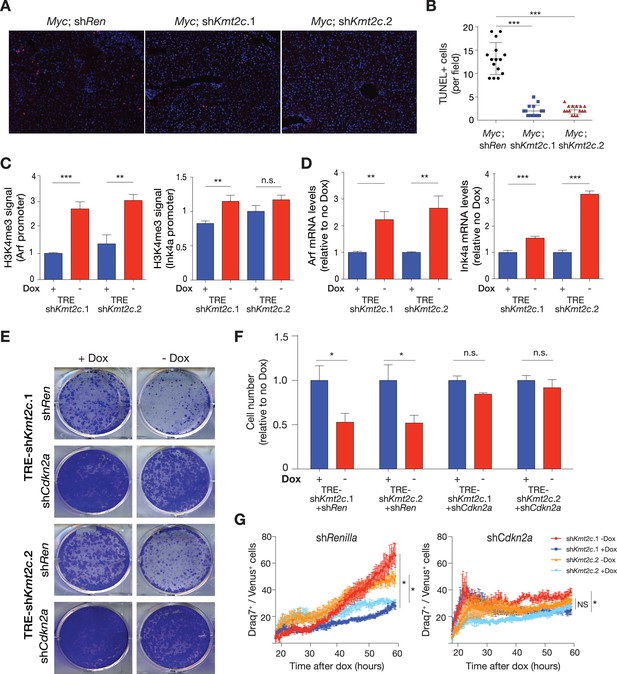
MLL3 mediates oncogene-induced apoptosis in a Cdkn2a-dependent manner.
(A) Representative images of TUNEL-positive nuclei (red staining) in murine livers 5 days after hydrodynamic injection of the indicated vector combinations. DAPI(4′,6-diamidino-2-phenylindole) was used to visualize nuclei. (B) Quantification of TUNEL-positive nuclei in mouse livers 5 days after hydrodynamic tail vein injection (HTVI) of the indicated vector combinations. Data points represent the number of TUNEL-positive cells in five different high-power fields in three independent murine livers per group. ***=p<0.001 (one-way ANOVA followed by post hoc t-tests). (C) Chromatin immunoprecipitation (ChIP)-qPCR analysis for H3K4me3 signals at Arf and Ink4a promoters 4 days after doxycycline (dox) withdrawal in Myc-rtTA3; TRE-shKmt2c cells. Values are mean ± SD from technical replicates (n=3), and the experiments were conducted in two independent liver progenitor cell (LPC) lines with different shKmt2c. (D) qPCR analysis for mRNA expression of Arf and Ink4a 4 days after dox withdrawal in two independent LPC lines with different shKmt2c. Values are mean ± SD from technical replicates (n=3). ***=p<0.001 and **=p<0.01 (unpaired two-tailed t-test). (E) Representative images of colony formation assay of the indicated cell lines 5 days after dox withdrawal. (F) Quantification of colony formation assay. Values are mean ± SD of three independent experiments with two independent LPC lines. *=p<0.05 (unpaired two-tailed t-test). (G) Time course analysis of Draq7-positive (dead or permeabilized) cells as a fraction of Venus-positive, Myc-rtTA3; TRE-shKmt2c cells expressing constitutive shRNAs targeting both Ink4a and Arf (shCdkn2a) or Renilla luciferase (shRen) off and on dox. Values represent mean ± SEM of triplicate wells of each genotype at each timepoint of two independently derived LPC lines, infected with either shRen or shCdkn2a. *=p<0.05 (unpaired two-tailed t-test of final average percentage Draq7+/GFP+). NS, not significant (p>0.05).
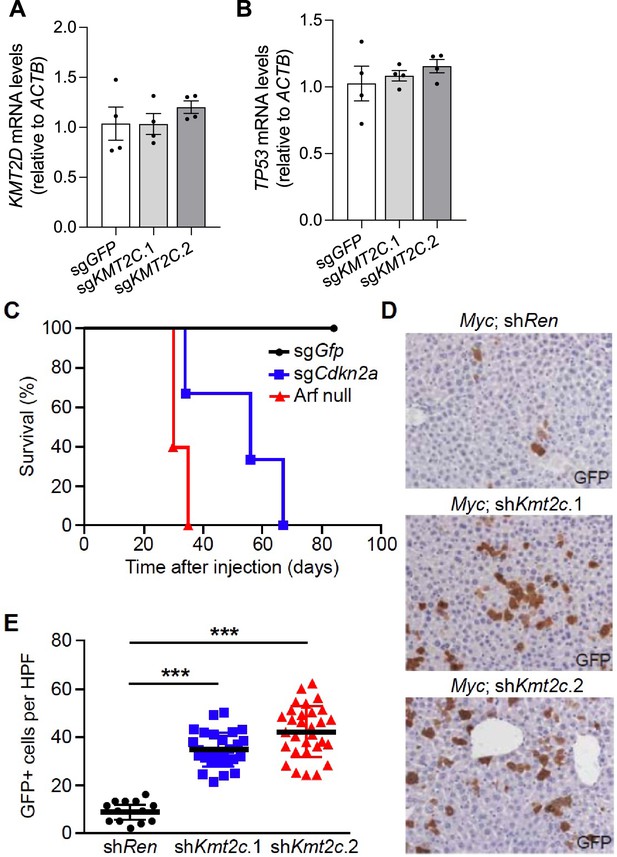
Kmt2c suppression reduces cell clearance upon enforced Myc expression in vivo.
(A–B) qPCR analysis for mRNA expression of KMT2D and TP53 in human HLE cells with sgGFP (control) or CRISPRa sgKMT2C (n=4). Each data point represents the average of technical duplicates. Data are shown as mean ± SEM. (C) Survival curve of wild-type mice injected with Myc transposon and pX330 expressing single guide RNAs (sgRNAs) targeting Cdkn2a (Myc; sgCdkn2a, n=5), or Arf-null mice injected with Myc vectors (Myc; Arf-null, n=5). Wild-type mice injected with Myc; sgGFP (n=3) served as a control. (D) Representative images of GFP-expressing cells detected by immunohistochemistry in murine livers 10 days after hydrodynamic injection of vectors enforcing stable expression of Myc and GFP-linked, constitutive shRNAs targeting Kmt2c or Renilla luciferase. (E) Quantification of GFP-positive cells in murine livers 10 days after hydrodynamic injection of the indicated vector combinations. Each data point represents GFP-positive cells in one of 10 different high-power fields (HPFs) in one of three independent murine livers.
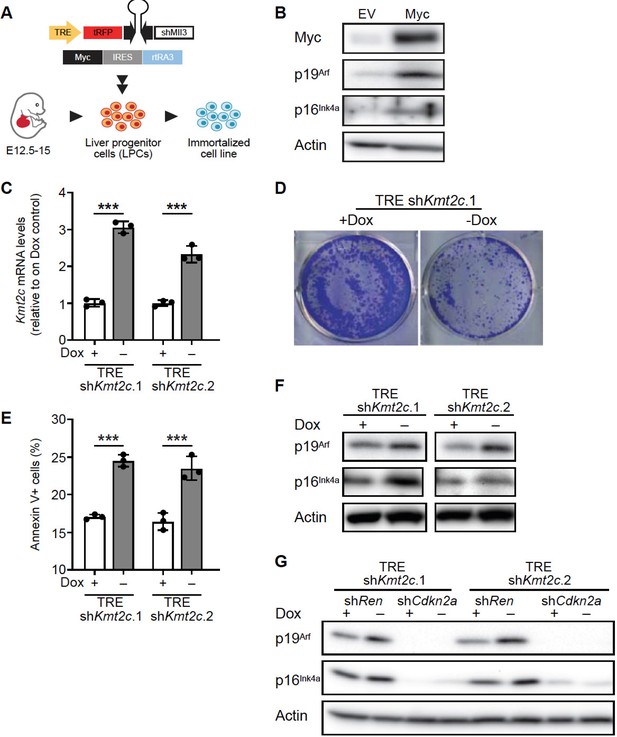
Endogenous Kmt2c restoration triggers apoptosis and is accompanied by increased Cdkn2a expression.
(A) Schematic for generating immortalized liver progenitor cell (LPC) lines from ED13 liver progenitor cells. (B) Western blot analysis of p16Ink4a, p19Arf, and MYC in wild-type LPCs 2 days after Myc transduction. (C) Kmt2c mRNA expression before (on) and after (off) Kmt2c restoration in LPCs expressing with two independent tet-regulated Kmt2c shRNAs. Values are mean ± SD from technical replicates (n=3). ***=p<0.001 (unpaired two-tailed t-test). (D) Representative images of colony formation assay of LPCs 4 days after Kmt2c restoration. (E) Annexin-V labeling in Myc-rtTA3; TRE-shKmt2c cells grown for 3 days with or without doxycycline (dox). Data are shown as mean ± SD of wells analyzed in triplicate. ***=p<0.001 (unpaired two-tailed t-test). (F) Western blot analysis of p16Ink4a and p19Arf 4 days after dox withdrawal in Myc-rtTA3; TRE-shKmt2c cells. (G) Western blot analysis of p16Ink4a and p19Arf proteins in Kmt2c -restored LPCs (two independent shRNAs targeting Kmt2c) with constitutively expressed control shRNAs (shRen) or Cdkn2a-targeting shRNAs (shCdkn2a). For all western blots, β-actin was used as loading control.
-
Figure 5—figure supplement 2—source data 1
Original western blots for Figure 5—figure supplement 2B,F,G.
- https://cdn.elifesciences.org/articles/80854/elife-80854-fig5-figsupp2-data1-v2.pdf
Tables
Reagent type (species) or resource | Designation | Source or reference | Identifiers | Additional information |
---|---|---|---|---|
Strain and strain background (M. musculus) | Wild-type C57BL/6 J | The Jackson Laboratory | Stock #000664 | |
Cell line (Homo-sapiens) | HLE HCC cell line | JCRB Cell Bank | JCRB0404 | |
Cell line (M. musculus) | Myc; sgTrp53 HCC cell lines | This paper | NA | Three independent cell lines derived from different mice were used as biological replicates |
Cell line (M. musculus) | Myc; sgKmt2c HCC cell lines | This paper | NA | Three independent cell lines derived from different mice were used as biological replicates |
Cell line (M. musculus) | Myc; sgAxin1 HCC cell lines | This paper | NA | Three independent cell lines derived from different mice were used as biological replicates |
Cell line (M. musculus) | TRE-shKmt2c.1 liver progenitor line | This paper | NA | |
Cell line (M. musculus) | TRE-shKmt2c.2 liver progenitor line | This paper | NA | |
Antibody | Anti-MLL3/4 (Rabbit polyclonal) | Dorighi et al., 2017; PMID:28483418 | NA | ChIP-seq (1:500) |
Recombinant DNA reagent | pT3-Myc | Addgene | #92046 | |
Recombinant DNA reagent | pT3-Ctnnb1 N90 | Addgene | #31785 | |
Recombinant DNA reagent | PX330-Cas9-U6-sgRNA | Addgene | #42230 | |
Recombinant DNA reagent | CMV-SB13 | Huang et al., 2014; PMID:25128497 | NA | |
Recombinant DNA reagent | pT3-EF1a-GFP-miRE | Huang et al., 2014; PMID:25128497 | NA |
Additional files
-
Supplementary file 1
Tables displaying the sequences of single guide RNA (sgRNA), shRNA, qPCR primers, and chromatin immunoprecipitation (ChIP)-qPCR primers used in the studies of this manuscript.
- https://cdn.elifesciences.org/articles/80854/elife-80854-supp1-v2.pdf
-
MDAR checklist
- https://cdn.elifesciences.org/articles/80854/elife-80854-mdarchecklist1-v2.pdf