cAMP−EPAC−PKCε−RIM1α signaling regulates presynaptic long-term potentiation and motor learning
Figures
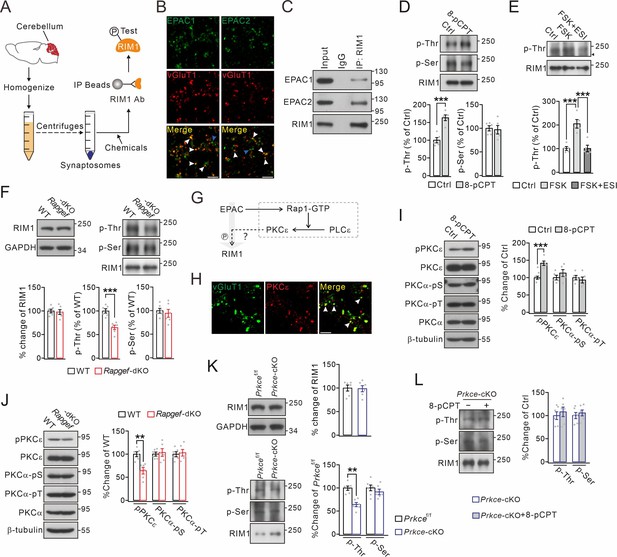
Threonine phosphorylation of RIM1 by EPAC and PKCε.
(A) Schematic showing purification of cerebellar synaptosomes and phophorylation assay of RIM1. (B) Immunostaining of EPAC1 or EPAC2 along with vGluT1 (white arrowheads) in cerebellar synaptosomes. Blue arrowheads show the synaptosomes marked by only EPAC1 or EPAC2. Scale bars, 5 μm. (C) Precleared synaptosomes (WT) were immunoprecipitated with anti-RIM1 antibody and probed with antibodies to EPAC1, EPAC2 and RIM1. Rabbit IgG was negative control. n=4. (D) WT synaptosomes were treated with control buffer (Ctrl) or 8-pCPT (20 μM, 30 min) and p-Thr and p-Ser of RIM1 were analyzed. p-Thr and p-Ser were normalized to corresponding RIM1 and percentage changes relative to Ctrl are plotted. p-Thr: 100 ± 8% (Ctrl) and 163 ± 8% (8-pCPT; p=0.00043). p-Ser: 100 ± 6% (Ctrl) and 97 ± 9% (8-pCPT; p=0.77). Unpaired t test. n=5 for all groups. ***p<0.001. (E) p-Thr and p-Ser of RIM1 in WT synaptosomes treated with control buffer, forskolin (FSK; 20 μM, 30 min), or FSK +ESI-09 (50 μM, 30 min) (FSK +ESI). Arrowhead marks nonspecific protein. p-Thr: 100 ± 8% (Ctrl), 205 ± 18% (FSK; p<0.001 vs. Ctrl), and 101 ± 14% (FSK +ESI; p=0.98 vs. Ctrl; p<0.001 vs. FSK). One-way ANOVA test. n=5 for all groups. ***p<0.001. (F) Phosphorylation of synaptosomal RIM1 from WT and Rapgef3/4-dKO mice. RIM1: 100 ± 4% (WT) and 98 ± 5% (Rapgef3/4-dKO; p=0.72). p-Thr: 100 ± 5% (WT) and 65 ± 5% (Rapgef3/4-dKO; p=0.00032). p-Ser: 100 ± 5% (WT) and 94 ± 8% (Rapgef3/4-dKO; p=0.57). Unpaired t test. n=6 for all groups. ***p<0.001. (G) Schematic depiction of proposed working model. The solid lines show known signaling pathways and the dashed line shows the hypothesis. (H) Immunostaining of PKCε and vGluT1 (arrowheads) in cerebellar synaptosomes. Scale bar, 5 μm. (I) WT synaptosomes were treated with control buffer or 8-pCPT (20 μM, 30 min). The phosphorylations of PKCε and PKCα were normalized to β-tubulin and percentage changes relative to control are plotted. pPKCε: 100 ± 5% (Ctrl) and 142 ± 7% (8-pCPT; p=0.0007). PKCα-pSer: 100 ± 8% (Ctrl) and 113 ± 11% (8-pCPT; p=0.31). PKCα-pThr: 100 ± 7% (Ctrl) and 93 ± 10% (8-pCPT; p=0.54). Unpaired t test. n=5 for all groups. ***p<0.001. (J) Phosphorylation of synaptosomal PKCε and PKCα in WT and Rapgef3/4-dKO mice. pPKCε, PKCα-pSer and PKCα-pThr were normalized to β-tubulin and their percentage changes relative to WT are plotted. pPKCε: 100 ± 5% (WT) and 64 ± 7% (Rapgef3/4-dKO; p=0.0013). PKCα-pSer: 100 ± 4% (WT) and 103 ± 8% (Rapgef3/4-dKO; p=0.70). PKCα-pThr: 100 ± 6% (WT) and 103 ± 7% (Rapgef3/4-dKO; p=0.73). Unpaired t test. n=6 for all groups. **p<0.01. (K) Phosphorylation of synaptosomal RIM1 in Prkcef/f and Prkce-cKO mice. RIM1: 100 ± 6% (WT) and 99 ± 6% (Rapgef3/4-dKO; p=0.88). p-Thr: 100 ± 3% (Prkcef/f) and 65 ± 6% (Prkce-cKO; p=0.0028). p-Ser: 100 ± 5% (Prkcef/f) and 95 ± 9% (Prkce-cKO; p=0.57). Unpaired t test. n=6 for all groups. **p<0.01. (L) Synaptosomes (Prkce-cKO) were treated wi/wo 8-pCPT (20 μM, 30 min) and RIM1 phosphorylation was analyzed. p-Thr: 100 ± 8%(Prkce-cKO) and 108 ± 10% (Prkce-cKO +8 pCPT; p=0.55). p-Ser: 100 ± 7% (Prkce-cKO) and 106 ± 6% (Prkce-cKO +8 pCPT; p=0.57). Unpaired t test. n=6 for all groups. See the online Figure 1—source data 1 file for source data of western blots in this figure.
-
Figure 1—source data 1
The uncut gel of western blots in Figure 1.
- https://cdn.elifesciences.org/articles/80875/elife-80875-fig1-data1-v2.pdf
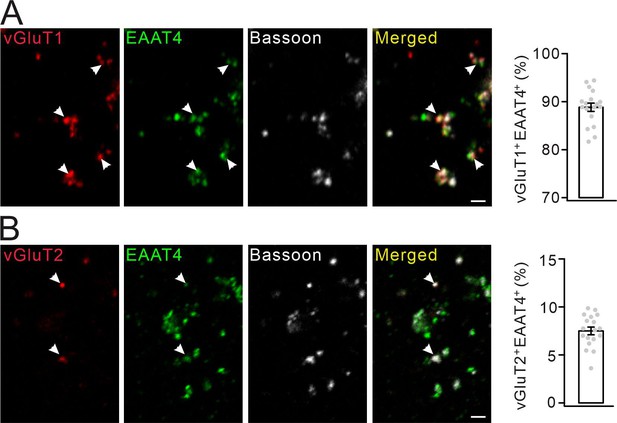
The percentages of PF and CF synapses among cerebellar synaptosomes.
(A) Immunostaining of vGluT1, EAAT4 and Bassoon in cerebellar synaptosomes. White arrowheads show PF synapse simultaneously marked by vGluT1 and EAAT4. Scale bar, 2 μm. Averaged percentage of PF synapses: 88.8 ± 0.8%. (B) Immunostaining of vGluT2, EAAT4 and Bassoon in cerebellar synaptosomes. White arrowheads show CF synapse simultaneously marked by vGluT2 and EAAT4. Scale bar, 2 μm. Averaged percentage of CF synapses: 7.5 ± 0.4%.
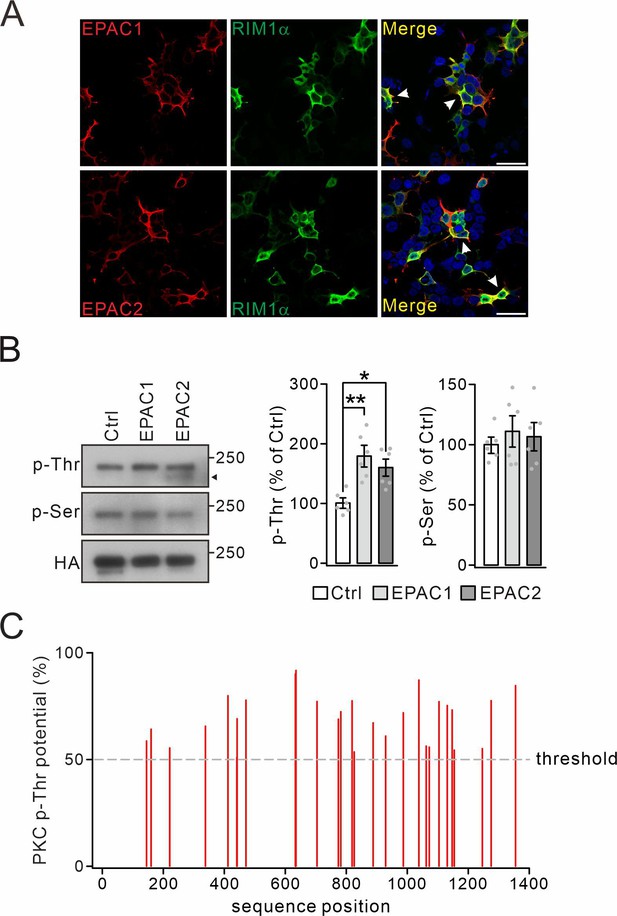
Threonine phosphorylation of RIM1 by EPAC in vitro.
(A) Co-transfection of HA-RIM1α with Flag-EPAC1 or Flag-EPAC2 in HEK cells. Arrowheads show the co-localization of RIM1α and EPACs. Scale bars: 100 μm. (B) HA-RIM1α (Ctrl) or HA-RIM1α with Flag-EPACs was transfected into HEK cells and p-Thr and p-Ser of RIM1α were measured. Arrowhead marks non-RIM1 protein. p-Thr: 100±7 (Ctrl), 179 ± 17% (EPAC1; p=0.0011 vs. Ctrl), and 160 ± 13% (EPAC2; p=0.010 vs. Ctrl). p-Ser: 100±5 (Ctrl), 111 ± 12% (EPAC1; p=0.69 vs. Ctrl), and 107 ± 10% (EPAC2; p=0.87 vs. Ctrl). One-way ANOVA test. n=6 for all groups. *p<0.05. **p<0.01. (C) Potential theronine phosphorylation sites by PKC in RIM1α were determined using the NetPhos 3.1 server (Technical University of Denmark; http://www.cbs.dtu.dk/services/NetPhos/). Dashed line: threshold for phosphorylation potential. See the online Figure 1—figure supplement 2—source data 1 or source data of western blots in this figure.
-
Figure 1—figure supplement 2—source data 1
The uncut gel of western blots in Figure 1—figure supplement 2.
- https://cdn.elifesciences.org/articles/80875/elife-80875-fig1-figsupp2-data1-v2.pdf
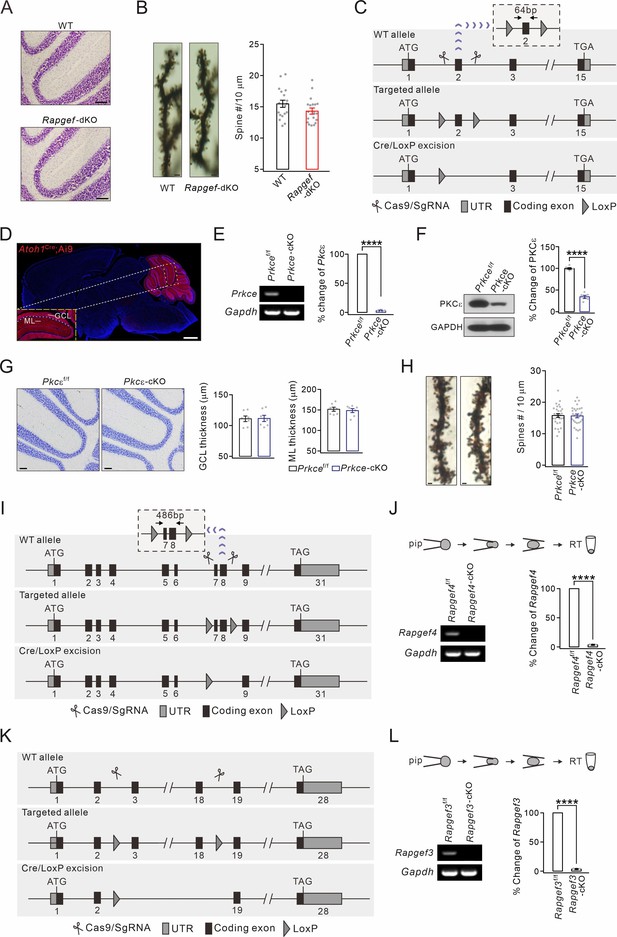
Generation of Prkce-cKO and Rapgef3;Rapgef4-cKO mice and cerebellar cytology.
(A) Nissl staining in the cerebellum from WT and Rapgef3/4-dKO mice. Scale bars, 100 μm. (B) Golgi staining showing apical PC spines. Scale bars, 2 μm. Average numbers of spines per 10 μm were 15.5±0.6 (WT; n=19 cells) and 14.3±0.5 (Rapgef3/4-dKO; n=19 cells). p=0.13. Unpaired t test. (C) The construction of Prkcef/f mice. LoxP sites were inserted before and after exon 2 and further excised by Atoh1Cre. (D) Native tdTomato fluorescence in the brain of Atoh1Cre;Ai9 mouse. Scale bars: 1 mm. (E) Granule cell contents of Prkcef/f and Prkce-cKO mice were harvested using glass micropipettes and subjected to RT-PCR. Prkce (165 bp) was absent in Prkce-cKO granule cells. ****p<0.0001. (F) PKCε expression in the cerebellum from Prkcef/f and Prkce-cKO mice. Percentage changes of PKCε: 100 ± 2% (Prkcef/f; n=6) and 34 ± 4% (Prkce-cKO; n=6). Unpaired t test. ****p<0.001. (G) Nissl staining in the cerebellum. Scale bars, 100 μm. GCL thickness: 111.0±4.9 μm (Prkcef/f) and 111.6±5.4 μm (Prkce-cKO), p=0.93, unpaired t test. ML thickness: 151.7±5.3 μm (Prkcef/f) and 148.4±5.3 μm (Prkce-cKO), p=0.64, unpaired t test. n=7 for all groups. (H) Golgi staining showing apical PC spines in Prkcef/f and Prkce-cKO mice. Scale bars, 2 μm. Average numbers of spines per 10 μm: 15.8±0.6 (Prkcef/f; n=29 cells) and 15.7±0.6 (Prkce-cKO; n=28 cells), p=0.88, unpaired t test. (I) The construction of Rapgef4f/f mice. LoxP sites were inserted between exons 6 and 9 and further excised by Atoh1Cre. (J) Granule cell contents of Rapgef4f/f and Rapgef4-cKO mice were harvested and subjected to RT-PCR. Rapgef4 (180 bp) was absent in Rapgef4-cKO granule cells. ****p<0.001. (K) The construction of Rapgef3f/f mice. LoxP sites were inserted between exons 3 and 18 and further excised by Atoh1Cre. (L) Granule cell contents of Rapgef3f/f and Rapgef3-cKO mice were harvested and subjected to RT-PCR. Rapgef3 (218 bp) was absent in Rapgef3-cKO granule cells. ****p<0.001. See the online Figure 1—figure supplement 3—source data 1 file for source data of western blots in this figure.
-
Figure 1—figure supplement 3—source data 1
The uncut gel of western blots and PCR in Figure 1—figure supplement 3.
- https://cdn.elifesciences.org/articles/80875/elife-80875-fig1-figsupp3-data1-v2.pdf
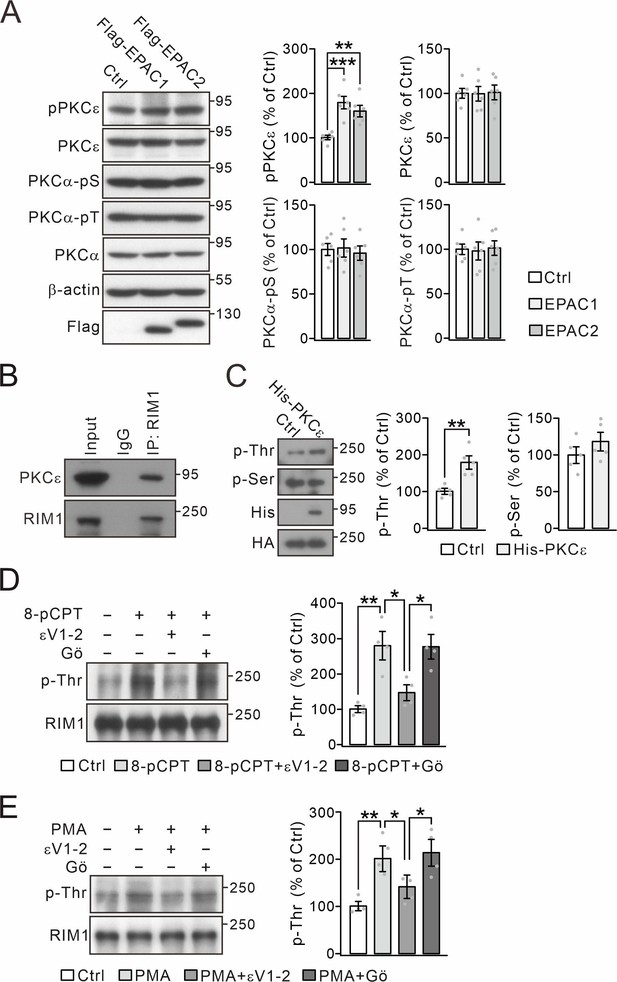
8-pCPT-induced RIM1 phosphorylation is blocked by PKCε inhibitor.
(A) Flag-RIM1α (Ctrl) or Flag-RIM1α with HA-EPACs was transfected into HEK cells, and the phosphorylation and expression of PKCε and PKCα were normalized to β-actin. For percentage change, pPKCε was 100 ± 5% (Ctrl), 179 ± 13% (EPAC1; p=0.0003 vs. Ctrl), and 160 ± 12% (EPAC2; p=0.003 vs. Ctrl); PKCε was 100 ± 6% (Ctrl), 99 ± 8% (EPAC1; p=0.96 vs. Ctrl), and 101 ± 8% (EPAC2; p=0.92 vs. Ctrl); PKCα-pS was 100 ± 7% (Ctrl), 101 ± 10% (EPAC1; p=0.94 vs. Ctrl), and 96 ± 8% (EPAC2; p=0.67 vs. Ctrl); and PKCα-pT was 100 ± 6% (Ctrl), 97 ± 10% (EPAC1; p=0.81 vs. Ctrl), and 101 ± 8% (EPAC2; p=0.90 vs. Ctrl). One-way ANOVA test. n=6 for all groups. **p<0.01. ***p<0.001. (B) Precleared synaptosomes from WT mice were immunoprecipitated with anti-RIM1 antibody and probed with antibodies to PKCε and RIM1. n=4. (C) HA-RIM1α (Ctrl) or HA-RIM1α with His-PKCε was transfected into HEK cells and p-Thr and p-Ser of RIM1α were analyzed. p-Thr and p-Ser were normalized to HA and percentage changes relative to Ctrl are plotted. p-Thr was 100 ± 8% (Ctrl) and 179 ± 18% (PKCε; p=0.0021). p-Ser was 100 ± 11% (Ctrl) and 118 ± 13% (PKCε; p=0.26). n=5 for all groups. Unpaired t test. **p<0.01. (D) Synaptosomes (WT) were treated with control buffer, 8-pCPT (20 μM, 30 min), 8-pCPT+εV1-2 (5 µM, 30 min) and 8-pCPT+Gӧ (10 μM, 30 min). p-Thr was normalized to RIM1 and percentage changes relative to Ctrl are plotted. Ctrl: 100 ± 8%. 8-pCPT: 280 ± 35% (p=0.0016 vs. Ctrl). 8-pCPT+εV1-2: 147 ± 19% (p=0.58 vs. Ctrl; p=0.015 vs 8-pCPT). 8-pCPT+Gӧ: 276±31 (p=0.0019 vs. Ctrl; p=0.99 vs 8-pCPT; p=0.017 vs 8-pCPT+εV1-2). One-way ANOVA test. n=4 for all groups. *p<0.05. **p<0.01. (E) Synaptosomes (WT) were treated with control buffer, PMA (1 μM, 30 min), PMA+εV1-2 (5 µM, 30 min) and PMA +Gӧ (10 μM, 30 min). RIM1 p-Thr was normalized to RIM1, and percentage changes relative to Ctrl are plotted. Ctrl: 100 ± 8%. PMA: 208 ± 19% (p=0.0041 vs. Ctrl). PMA+εV1-2: 134 ± 13% (p=0.55 vs. Ctrl; p=0.043 vs. PMA). PMA +Gӧ: 214 ± 24% (p=0.0029 vs. Ctrl; p=0.99 vs. PMA; p=0.030 vs. PMA+εV1-2). One-way ANOVA test. n=4 for all groups. *p<0.05. **p<0.01. See the online Figure 1—figure supplement 4—source data 1 file for source data of western blots in this figure.
-
Figure 1—figure supplement 4—source data 1
The uncut gel of western blots in Figure 1—figure supplement 4.
- https://cdn.elifesciences.org/articles/80875/elife-80875-fig1-figsupp4-data1-v2.pdf
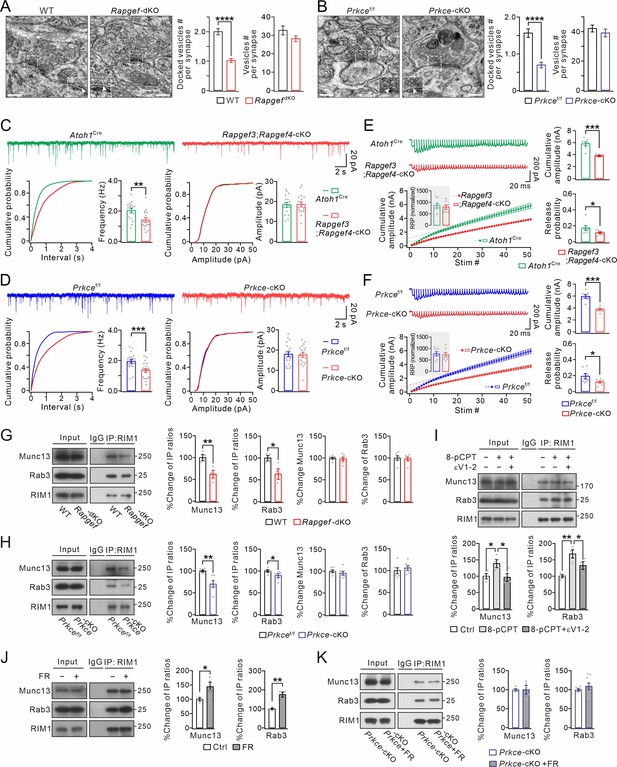
EPAC and PKCε act on vesicle docking, synaptic release, and Rab3-RIM1-Munc13 complex.
(A) Representative EM (23,000×) of PF-PC synapses of WT and Rapgef3/4-dKO mice. Scale bars: 200 nm. The inserts show docked vesicles. Unpaired t test. ****p<0.0001. (B) Representative EM of PF-PC synapses of Prkcef/f and Prkce-cKO mice. Scale bars: 200 nm. Unpaired t test. ****p<0.0001. (C) Example PC mEPSCs in Atoh1Cre and Rapgef3;Rapgef4-cKO mice. Lower: statistics of inter-event interval and amplitude. Grey dots indicate individual data points. Frequency: 2.0±0.2 Hz (Atoh1Cre) and 1.4±0.2 Hz (Rapgef3;Rapgef4-cKO; p=0.0036). Amplitude: 18.3±1.3 pA (Atoh1Cre) and 18.5±1.3 pA (Rapgef3;Rapgef4-cKO; p=0.46). Unpaired t test. n=for all groups. **p<0.01. (D) Example PC mEPSCs from Prkcef/f and Prkce-cKO mice. Frequency: 1.9±0.1 Hz (Prkcef/f; n=19) and 1.3±0.1 Hz (Prkce-cKO; n=20; p=0.00059). Amplitude: 17.9±1.2 pA (Prkcef/f; n=19) and 17.5±1.1 pA (Prkce-cKO; n=20; p=0.39). Unpaired t test. ***p<0.001. (E) Representative responses of Atoh1Cre and Rapgef3;Rapgef4-cKO PCs to 100 Hz PF stimulation. The artifacts were truncated and each EPSC were aligned to its initial rising point. RRP was defined as the y-intercept of linear portion of cumulative amplitude curve. For RRP (inset), Atoh1Cre: 861±113; Rapgef3;Rapgef4-cKO: 790±101; p=0.31, unpaired t test. For cumulative amplitude, Atoh1Cre: 5815±360 pA; Rapgef3;Rapgef4-cKO: 3848±66 pA; p<0.001, unpaired t test. For Pr, Atoh1Cre: 0.17±0.03; Rapgef3;Rapgef4-cKO: 0.11±0.01; p=0.043, unpaired t test. n=7 for both groups. *p<0.05. ***p<0.001. (F) Representative responses of Prkcef/f and Prkce-cKO PCs to 100 Hz PF stimulation. The artifacts were truncated and each EPSC was aligned to its initial rising point. For RRP, Prkcef/f, 764±100; Prkce-cKO, 728±106, p=0.40, unpaired t test. For cumulative amplitude, Prkcef/f, 5940±337 pA; Prkce-cKO, 3755±181 pA; p<0.001, unpaired t test. For Pr, Prkcef/f, 0.19±0.04; Prkce-cKO, 0.12±0.01; p=0.034, unpaired t test. n=7 for both groups. *p<0.05. ***p<0.001. (G) Cerebellar synaptosomes from WT and Rapgef3/4-dKO mice were immunoprecipitated by anti-RIM1 antibody, and the immunoprecipitates were probed with antibodies to Munc13-1, Rab3A, and RIM1. Rabbit IgG was negative control. Ratios of immunoprecipitated Munc13-1 or Rab3A vs. RIM1 were normalized to WT. Munc13-1: 100 ± 6% (WT) and 62 ± 8% (Rapgef3/4-dKO; p=0.0081, n=4). Rab3A: 100 ± 5% (WT) and 63 ± 10% (Rapgef3/4-dKO; p=0.019, n=4). Total Rab3A and RIM1 were normalized to WT. Munc13-1: 100 ± 2% (WT) and 98 ± 4% (Rapgef3/4-dKO; p=0.73, n=6). Rab3A: 100 ± 5% (WT) and 98 ± 4% (Rapgef3/4-dKO; p=0.77, n=6). Unpaired t test. *p<0.05. **p<0.01. (H) Immunoprecipitation of Munc13-1 and Rab3A with RIM1 in cerebellar synaptosomes from Prkcef/f and Prkce-cKO mice. Ratios of immunoprecipitated Munc13-1 or Rab3A vs. RIM1 were normalized to WT. Munc13-1: 100 ± 2% (Prkcef/f) and 70 ± 8% (Prkce-cKO; p=0.0030). Rab3A: 100 ± 2% (Prkcef/f) and 89 ± 4% (Prkce-cKO; p=0.019). Total Rab3A and RIM1 were normalized to Prkcef/f. Munc13-1: 100 ± 3% (Prkcef/f) and 96 ± 5% (Prkce-cKO; p=0.46). Rab3A: 100 ± 7% (Prkcef/f) and 106 ± 5% (Prkce-cKO; p=0.52). n=6 for all groups. Unpaired t test. *p<0.05. **p<0.01. (I) Cerebellar synaptosomes (WT) mice were incubated in control buffer or 8-pCPT (20 μM, 30 min) and εV1-2 (5 µM, 30 min) and immunoprecipitated. Ratios of immunoprecipitated Munc13-1 or Rab3A vs. RIM1 were normalized to control. Munc13-1: 100 ± 8% (Ctrl); 138 ± 12% (8-pCPT; p=0.041 vs. Ctrl); 96 ± 12% (8-pCPT+εV1-2; p=0.97 vs. Ctrl; p=0.029 vs 8-pCPT). Rab3A: 100 ± 5% (Ctrl); 168 ± 12% (8-pCPT; p=0.0011 vs. Ctrl); 133 ± 12% (8-pCPT+εV1-2; p=0.069 vs. Ctrl; p=0.046 vs 8-pCPT). One-way ANOVA test. n=4 for all groups. *p<0.05. **p<0.01. (J) Cerebellar synaptosomes (WT) were treated with control buffer or FR236924 (FR) (200 nM, 30 min) and immunoprecipitated. Ratios of immunoprecipitated Munc13-1 or Rab3A vs. RIM1 were normalized to Ctrl. Munc13-1: 100 ± 4% (Ctrl) and 144 ± 16% (FR; p=0.041). Rab3A: 100 ± 4% (Ctrl) and 175 ± 13% (FR; p=0.0016). Unpaired t test. n=4 for all groups. *p<0.05. **p<0.01. (K) Cerebellar synaptosomes (Prkce-cKO) were treated with control buffer or FR236924 and immunoprecipitated. Ratios of immunoprecipitated Munc13-1 or Rab3A vs. RIM1 were normalized to Prkce-cKO. Munc13-1: 100 ± 3% (Prkce-cKO; n=4) and 100 ± 12% (Prkce-cKO +FR; p=0.99; n=4). Rab3A: 100 ± 2% (Prkce-cKO; n=8) and 108 ± 9% (Prkce-cKO +FR; p=0.37; n=8). Unpaired t test. See the online Figure 2—source data 1 file for source data of western blots in this figure.
-
Figure 2—source data 1
The uncut gel of western blots in Figure 2.
- https://cdn.elifesciences.org/articles/80875/elife-80875-fig2-data1-v2.pdf
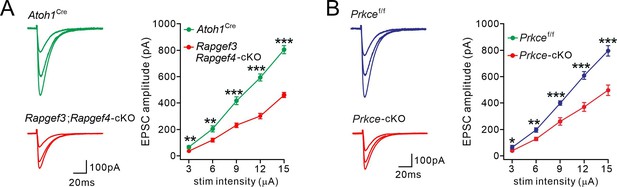
Input-output relationship of evoked PF-EPSCs in control and mutant mice.
(A) Example PF-EPSCs obtained from Atoh1Cre and Rapgef3;Rapgef4-cKO PCs with stimulation intensities of 6, 9, and 12 μA. For Atoh1Cre PCs, 66±11 pA (3 μA), 204±23 pA (6 μA); 417±29 pA (9 μA); 594±26 pA (12 μA); and 804±32 pA (15 μA); n=9. For Rapgef3;Rapgef4-cKO PCs, 36±4 pA (3 μA; p=0.0034), 119±15 pA (6 μA; p=0.0022); 231±17 pA (9 μA; p<0.001); 302±20 pA (12 μA; p<0.001); and 461±19 pA (15 μA; p<0.001); n=10. Unpaired t test. (B) Example PF-EPSCs obtained from Prkcef/f and Prkce-cKO PCs with stimulation intensities of 6, 9, and 12 μA. For Prkcef/f PCs, 64±14 pA (3 μA), 196±17 pA (6 μA); 400±17 pA (9 μA); 608±30 pA (12 μA); and 796±40 pA (15 μA); n=9. For Prkce-cKO PCs, 39±4 pA (3 μA; P=0.028), 127±12 pA (6 μA; p=0.001); 260±28 pA (9 μA; p=0.00011); 370±34 pA (12 μA; p<0.001); and 497±40 pA (15 μA; p<0.001); n=10. Unpaired t test.
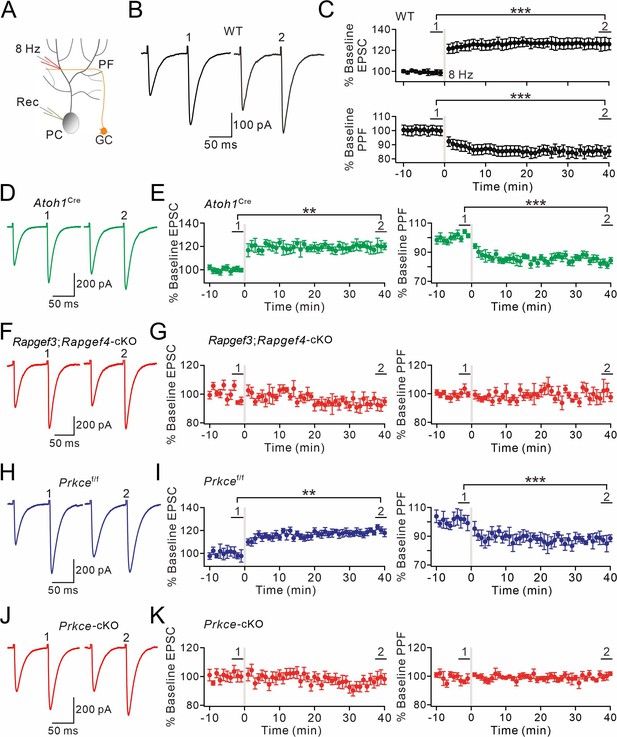
EPAC and PKCε are required for presynaptic PF-PC LTP.
(A) Schematic showing the induction of presynaptic LTP. (B, D, F, H, J) Example PF-EPSCs for baseline (1) and after LTP induction (2) in WT (B), Atoh1Cre (D), Rapgef3;Rapgef4-cKO (F), Prkcef/f (H), and Prkce-cKO (J) mice. (C) Percentage changes of PF-EPSC amplitudes (WT). (1): 101 ± 4%; (2): 131 ± 6%; n=13; p<0.001. Percentage changes of PPF ratios from cells shown above. (1): 101 ± 3%; (2): 84 ± 4%; n=13; p<0.001. Paired t test. ***p<0.001. (E) Left: percentage changes of PF-EPSC amplitudes (Atoh1Cre). (1): 100 ± 2%; (2): 120 ± 5%; n=10; p=0.004. Right: percentage changes of PPF ratios. (1): 102 ± 2%; (2): 83 ± 2%; n=10; p<0.001. Unpaired t test. **p<0.01. ***p<0.001. (G) Left: percentage changes of PF-EPSC amplitudes (Rapgef3;Rapgef4-cKO). (1): 99 ± 2%; (2): 93 ± 4%; n=9; p=0.059. Right: percentage changes of PPF ratios. (1): 101 ± 3%; (2): 101 ± 5%; n=9; p=0.07. Paired t test. (I) Left: percentage changes of PF-EPSC amplitudes (Prkcef/f). (1): 99 ± 4%; (2): 120 ± 3%; n=7; p=0.004. Right: percentage changes of PPF ratios. (1): 100 ± 5%; (2): 86 ± 4%; n=7; p<0.001. Paired t test. **p<0.01. ***p<0.001. (K) Left: percentage changes of PF-EPSC amplitudes (Prkce-cKO). (1): 101 ± 4%; (2): 99 ± 5%; n=10; p=0.065. Right: percentage changes of PPF ratios. (1): 101 ± 3%; (2): 100 ± 2%; n=10; p=0.77. Paired t test.
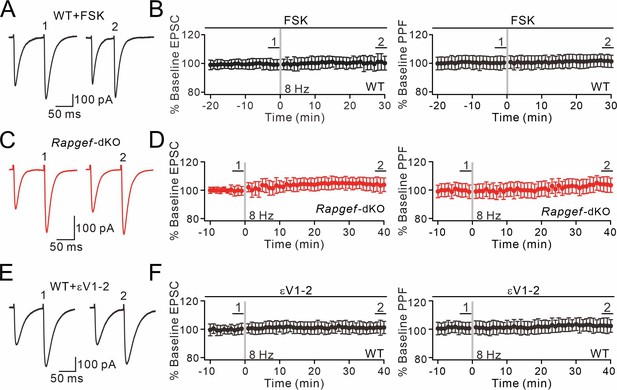
Presynaptic PF-PC LTP is blocked by forskolin incubation, EPAC ablation, or εV1-2 application.
(A) Example PF-EPSCs for baseline (1) and after LTP induction (2) in a WT PC perfused with forskolin (20 μM). (B) Percentage changes of PF-EPSC amplitude with forskolin treatment in WT mice: 99 ± 3% (1) and 101 ± 5% (2); n=11; p=0.63. PPF ratios from cells shown in the left panel: 100 ± 5% (1) and 102 ± 4% (2); n=11; p=0.72. Paired t test. (C) Example PF-EPSCs for baseline and after LTP induction in an Rapgef3/4-dKO PC. (D) Percentage changes of PF-EPSC amplitude in Rapgef3/4-dKO mice: 100 ± 3% (1) and 104 ± 5% (2); n=11; p=0.66. PPF ratios from cells shown in the left panel: 99 ± 5% (1) and 104 ± 5% (2); n=11; p=0.58. Paired t test. (E) Example PF-EPSCs for baseline and after LTP induction in a WT PC perfused with εV1-2 (5 µM). (F) Percentage changes of PF-EPSC amplitude in WT mice: 100 ± 3% (1) and 101 ± 4% (2); n=9; p=0.59. PPF ratios from cells shown in the left panel: 101 ± 4% (1) and 102 ± 5% (2); n=9; p=0.22. Paired t test.
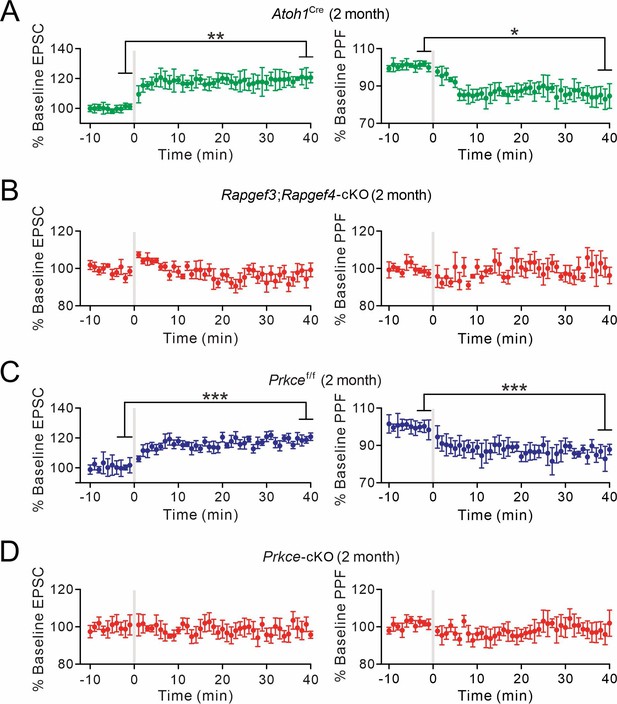
The induction of presynaptic PF-PC LTP in 2-month-old mice.
(A) Left: percentage changes of PF-EPSC amplitudes (Atoh1Cre). Right: percentage changes of PPF ratios. 84 ± 4% at t=38–40 min; n=7; p=0.011. Paired t test. *p<0.05. **p<0.01. (B) Left: percentage changes of PF-EPSC amplitudes (Rapgef3;Rapgef4-cKO). Right: percentage changes of PPF ratios. 100 ± 4% at t=38–40 min; n=6; p=0.97. Paired t test. (C) Left: percentage changes of PF-EPSC amplitudes (Prkcef/f). Right: percentage changes of PPF ratios. 86 ± 2% at t=38–40 min; n=6; p=0.0008. Paired t test. ***p<0.001. (D) Left: percentage changes of PF-EPSC amplitudes (Prkce-cKO). Right: percentage changes of PPF ratios. 99 ± 5% at t=38–40 min; n=7; p=0.78. Paired t test.
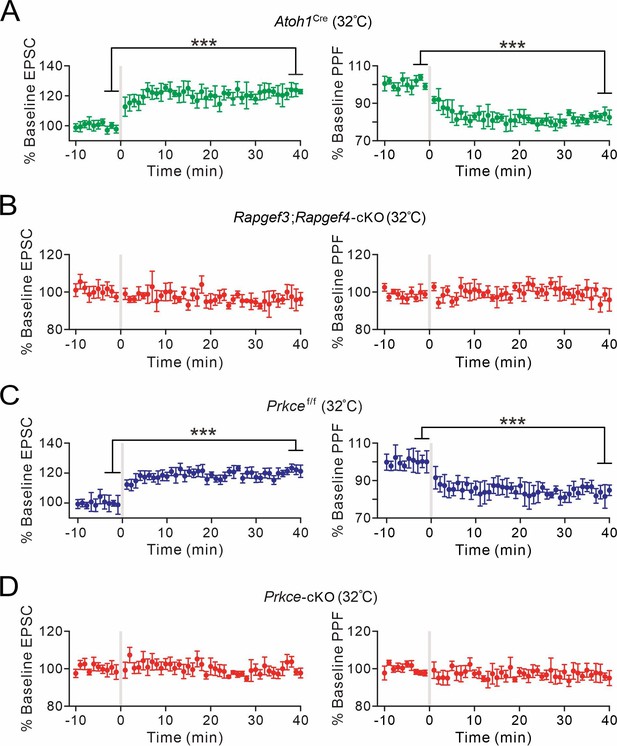
The induction of presynaptic PF-PC LTP in elevated temperature.
(A) Left: percentage changes of PF-EPSC amplitudes (Atoh1Cre). Right: percentage changes of PPF ratios. 83 ± 2% at t=38–40 min; n=6; p=0.00036. Paired t test. ***p<0.001. (B) Left: percentage changes of PF-EPSC amplitudes (Rapgef3;Rapgef4-cKO). Right: percentage changes of PPF ratios. 96 ± 3% at t=38–40 min; n=6; p=0.27. Paired t test. (C) Left: percentage changes of PF-EPSC amplitudes (Prkcef/f). Right: percentage changes of PPF ratios. 83 ± 2% at t=38–40 min; n=6; p=0.00039. Paired t test. ***p<0.001. (D) Left: percentage changes of PF-EPSC amplitudes (Prkce-cKO). Right: percentage changes of PPF ratios. 96 ± 3% at t=38–40 min; n=6; p=0.22. Paired t test.
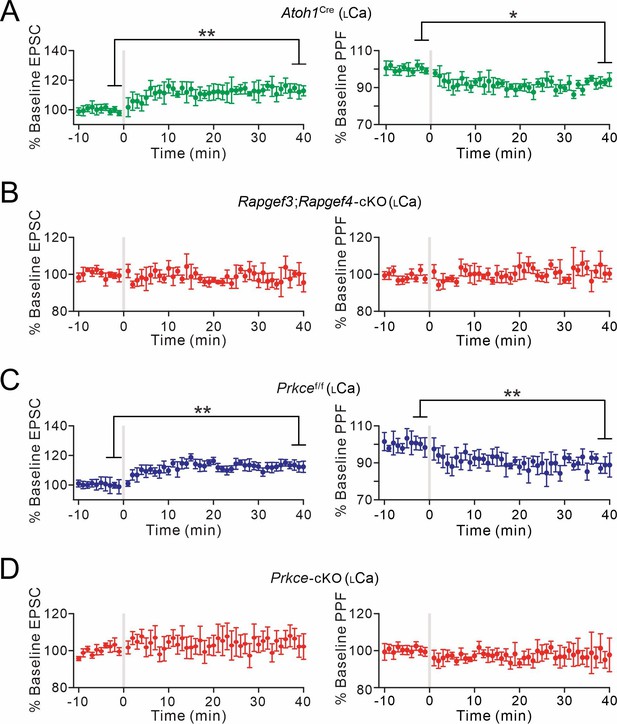
The induction of presynaptic PF-PC LTP in lower Ca2+ concentration.
(A) Left: percentage changes of PF-EPSC amplitudes (Atoh1Cre). Right: percentage changes of PPF ratios. 93 ± 2% at t=38–40 min; n=6; p=0.017. Paired t test. *p<0.05. **p<0.01. (B) Left: percentage changes of PF-EPSC amplitudes (Rapgef3;Rapgef4-cKO). Right: percentage changes of PPF ratios. 102 ± 2% at t=38–40 min; n=6; p=0.42. Paired t test. (C) Left: percentage changes of PF-EPSC amplitudes (Prkcef/f). Right: percentage changes of PPF ratios. 88 ± 6% at t=38–40 min; n=6; p=0.005. Paired t test. **p<0.01. (D) Left: percentage changes of PF-EPSC amplitudes (Prkce-cKO). Right: percentage changes of PPF ratios. 98 ± 2% at t=38–40 min; n=6; p=0.76. Paired t test.
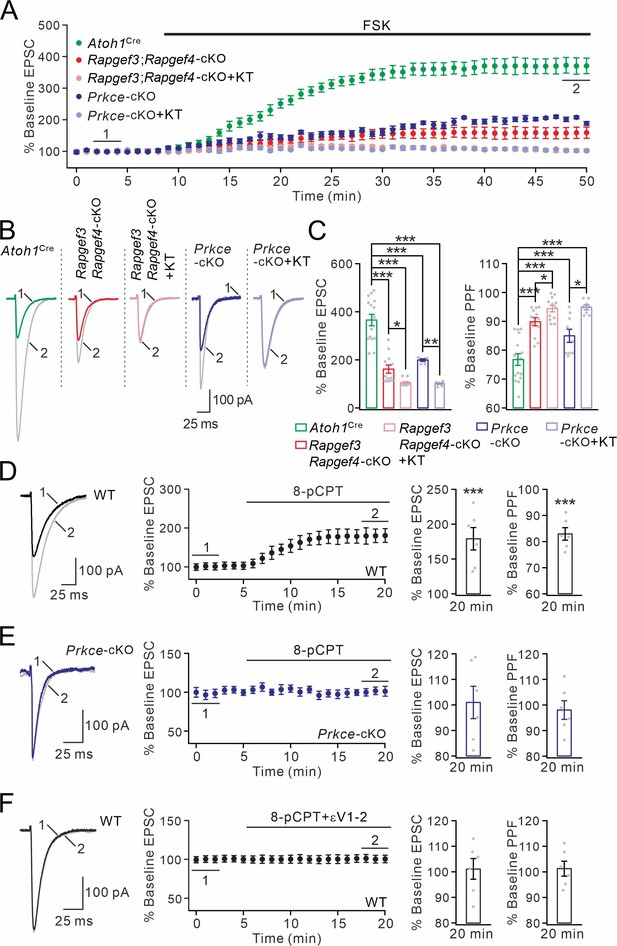
cAMP-triggered PF facilitation is dependent on EPAC and PKCε.
(A) The facilitation of PF-EPSCs by forskolin (FSK) (20 μM) in Atoh1Cre, Rapgef3;Rapgef4-cKO and Prkce-cKO mice. (B) Example traces for baseline (1) and after potentiation (2) shown in (A). (C) Left: percent changes of EPSC amplitude. Atoh1Cre: 366 ± 25% (n=15); Rapgef3;Rapgef4-cKO: 162 ± 18% (n=12; p<0.001 vs. Atoh1Cre); Rapgef3;Rapgef4-cKO+KT: 106 ± 4% (n=12; p<0.001 vs. Atoh1Cre; p=0.046 vs. Rapgef3;Rapgef4-cKO); Prkce-cKO: 198 ± 5% (n=12; p<0.001 vs. Atoh1Cre); Prkce-cKO +KT: 101 ± 3% (n=12; p<0.001 vs. Atoh1Cre; p=0.0034 vs. Prkce-cKO). Right: percent changes of PPF. Atoh1Cre: 77 ± 2% (n=15); Rapgef3;Rapgef4-cKO: 90 ± 1% (n=12; p<0.001 vs. Atoh1Cre); Rapgef3;Rapgef4-cKO+KT: 94 ± 1% (n=12; p<0.001 vs. Atoh1Cre; p=0.049 vs. Rapgef3;Rapgef4-cKO); Prkce-cKO: 85 ± 2% (n=12; p<0.001 vs. Atoh1Cre); Prkce-cKO +KT: 95 ± 1% (n=12; p<0.001 vs. Atoh1Cre; p=0.025 vs. Prkce-cKO). One-way ANOVA test. *p<0.05. ***p<0.001. (D) Bath application of 8-pCPT (20 μM) caused PF-EPSC potentiation in WT mice. Left: example traces before (1) and after potentiation (2). Middle: time course of PF facilitation. Right: percent changes of EPSC amplitude (179 ± 18%; n=6; p<0.001) and PPF (83 ± 3%; n=6; p<0.001) at 18–20 min vs. baseline (0–2 min). Paired t test. ***p<0.001. (E) 8-pCPT failed to induce PF-EPSC potentiation in Prkce-cKO mice. Left: example traces for baseline (1) and after potentiation (2). Middle: time course of PF facilitation. Right: percent changes of EPSC amplitude (101 ± 6%; n=6; p=0.35) and PPF (98 ± 4%; n=6; p=0.45) at 18–20 min vs. baseline (0–2 min). Paired t test. (F) Co-application of 8-pCPT and εV1-2 (5 μM) failed to produce PF potentiation in WT mice. Left: example traces for baseline (1) and after potentiation (2). Middle: time course of PF-EPSCs. Right: percent changes of EPSC amplitude (101 ± 4%; n=6; p=0.78) and PPF (101 ± 3%; n=6; p=0.67) at 18–20 min vs. baseline (0–2 min). Paired t test.
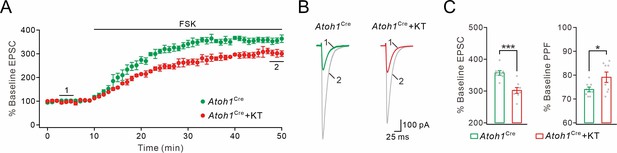
PKA inhibition has a modest effect in blocking cAMP-triggered facilitation.
(A) The facilitation of PF-EPSCs by forskolin (FSK) (20 μM) and its inhibition by KT5720 (3 μM) in Atoh1Cre mice. (B) Example traces for baseline (1) and after potentiation (2) shown in (A). (C) Left: percent changes of EPSC amplitude. Atoh1Cre: 357 ± 8% (n=8); Atoh1Cre +KT5720: 302 ± 10% (n=8; p=0.00015). Right: percent changes of PPF. Atoh1Cre: 74 ± 1% (n=8); Atoh1Cre+KT5720: 79 ± 2% (n=8; p=0.026). Paired t test. *p<0.05. ***p<0.001.
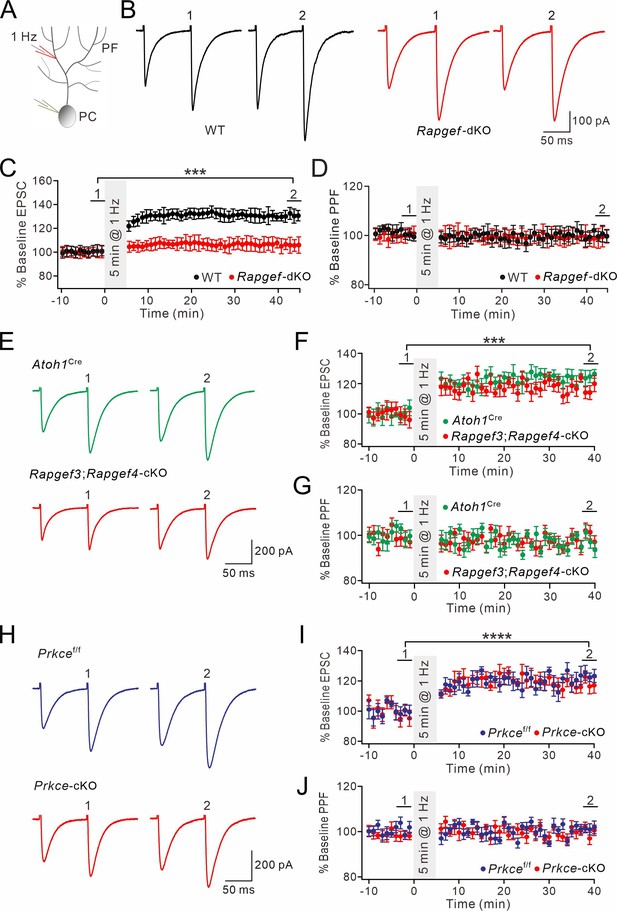
Postsynaptic PF-PC LTP is intact upon presynaptic deletion of EPAC or PKCε.
(A) Schematic showing the induction of postsynaptic LTP. (B, E, H) Example PF-EPSCs for baseline (1) and after induction (2) in WT and Rapgef3/4-dKO PCs (B), Atoh1Cre and Rapgef3;Rapgef4-cKO PCs (E), and Prkcef/f and Prkce-cKO PCs (H). (C) Percentage changes of PF-EPSC amplitude. In WT, 101 ± 5% for (1) and 131 ± 5% for (2) (p<0.001). In Rapgef3/4-dKO, 100 ± 5% for (1) and 106 ± 6% for (2) (p=0.26). Paired t test. n=13 for both groups. ***p<0.001. (D) Percentage changes of PPF ratios of cells shown in (C). In WT, 100 ± 2% for (1) and 100 ± 3% for (2) (p=0.63). In Rapgef3/4-dKO, 101 ± 3% for (1) and 99 ± 4% for (2) (p=0.74). Paired t test. n=13 for both groups. (F) Percentage changes of PF-EPSC amplitude. In Atoh1Cre, 100 ± 5% for (1) and 123 ± 3% for (2) (p<0.001). In Rapgef3;Rapgef4-cKO, 98 ± 5% for (1) and 119 ± 4% for (2) (p<0.001). Paired t test. n=7 for both groups. ***p<0.001. (G) Percentage changes of PPF ratios of cells shown in (C). In Atoh1Cre: 100 ± 2% for (1) and 96 ± 3% for (2) (p=0.26). In Rapgef3;Rapgef4-cKO: 98 ± 3% for (1) and 95 ± 3% for (2) (p=0.28). Paired t test. n=7 for both groups. (I) Percentage changes of PF-EPSC amplitude. In Prkcef/f, 99 ± 4% for (1) and 121 ± 4% for (2) (p<0.0001). In Prkce-cKO: 97 ± 5% for (1) and 118 ± 5% for (2) (p<0.0001). Paired t test. n=7 for both groups. ****p<0.0001. (J) Percentage changes of PPF ratios from cells shown in (I). In Prkcef/f, 102 ± 2% for (1) and 101 ± 2% for (2) (p=0.73). In Prkce-cKO, 98 ± 2% and 100 ± 2% for (2) (p=0.78). Paired t test. n=7 for both groups.
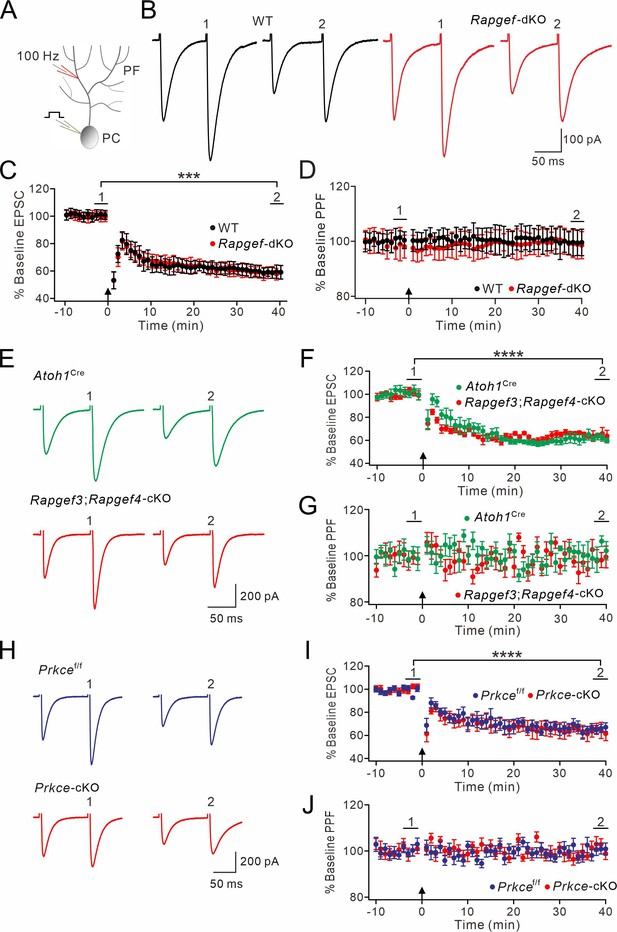
PF-LTD is unaltered by presynaptic deletion of EPAC or PKCε.
(A) A scheme showing the induction of postsynaptic LTD. (B, E, H) Example PF-EPSCs for baseline (1) and after LTD induction (2) in WT and Rapgef3/4-dKO PCs (B), Atoh1Cre and Rapgef3;Rapgef4-cKO PCs (E), and Prkcef/f and Prkce-cKO PCs (H). (C) Percentage changes of PF-EPSC amplitude. In WT, 101 ± 3% for (1) and 59 ± 5% for (2) (p<0.001). In Rapgef3/4-dKO, 100 ± 3% for (1) and 59 ± 4% for (2) (<0.001). Paired t test. n=13 for both groups. ***p<0.001. (D) Percentage changes of PPF ratios of cells shown in (C). In WT, 100 ± 3% for (1) and 100 ± 5% for (2) (p=0.49). In Rapgef3/4-dKO, 100 ± 5% for (1) and 100 ± 5% for (2) (p=0.26). Paired t test. n=13 for both groups. (F) Percentage changes of PF-EPSC amplitude. In Atoh1Cre, 100 ± 4% for (1) and 61 ± 3% for (2) (p<0.0001). In Rapgef3;Rapgef4-cKO, 101 ± 3% for (1) and 65 ± 4% for (2) (p<0.0001). Paired t test. n=7 for both groups. ****p<0.0001. (G) Percentage changes of PPF ratios of cells shown in (F). In Atoh1Cre, 100 ± 2% for (2) and 100 ± 3% for (2) (p=0.40). In Rapgef3;Rapgef4-cKO, 101 ± 3% for (2) and 99 ± 4% for (2) (p=0.61). Paired t test. n=7 for both groups. (I) Percentage changes of PF-EPSC amplitude. In Prkcef/f, 99 ± 2% for (1) and 66 ± 4% for (2) (p<0.0001). In Prkce-cKO, baseline: 101 ± 2% for (1) and 64 ± 6% for (2) (p<0.0001). Paired t test. n=7 for both groups. ****p<0.0001. (J) Percentage changes of PPF ratios of cells shown in (I). In Prkcef/f, 101 ± 2% for (1) and 101 ± 2% for (2) (p=0.56). In Prkce-cKO, 100 ± 2% for (1) and 102 ± 2% for (2) (p=0.54). Paired t test. n=7 for both groups.
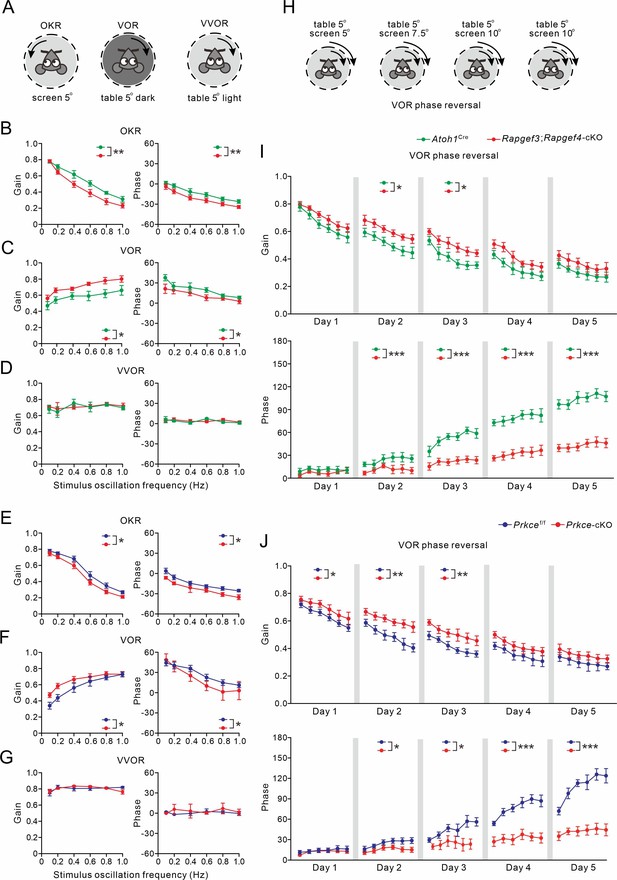
VOR baseline and adaptation in Atoh1Cre, Rapgef3;Rapgef4-cKO, Prkcef/f and Prkce-cKO mice.
(A) Pictograms depicted compensatory eye movements driven by visual stimulus (OKR), vestibular stimulus (VOR) or both (VVOR). (B) OKR gain (measure of eye movement amplitude) and phase (measure of timing) were smaller in Rapgef3;Rapgef4-cKO (n=16) mice compared to Atoh1Cre (n=10) mice. (C) VOR was affected in Rapgef3;Rapgef4-cKO mice. (D) The combination of vestibular and visual input by rotation of the mouse in the light evoked the VVOR in Atoh1Cre and Rapgef3;Rapgef4-cKO mice. (E) OKR gain and phase were smaller in Prkce-cKO (n=11) mice compared to Prkcef/f (n=10) mice. (F) VOR was affected in Prkce-cKO mice. (G) VVOR gain and phase in Prkcef/f and Prkce-cKO mice. (H) Mismatched visual and vestibular input was used to trigger adaptation of the eye movements in order to test motor learning ability. This training induced a reversal of VOR phase probed by VOR recordings in the dark. (I) Both gain-decrease learning and phase learning of Rapgef3;Rapgef4-cKO were impaired. *p<0.05. ***p<0.001. (J) Both gain-decrease learning and phase learning of Prkce-cKO were impaired. *p<0.05. **p<0.01. ***p<0.001.
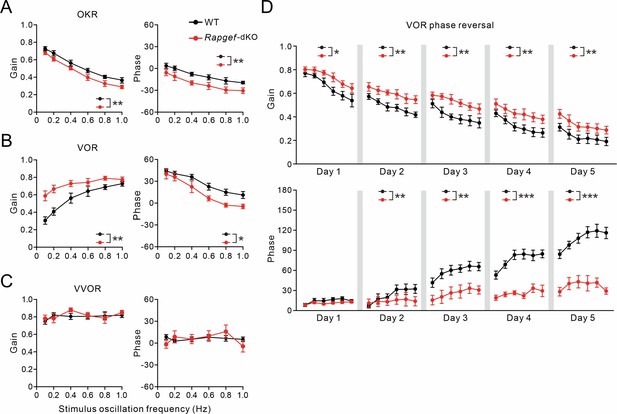
Impaired VOR learning in Rapgef3/4-dKO mice.
(A) Gain and phase values of WT (n=10) and Rapgef3/4dKO (n=10) mice during OKR evoked by visual stimulation. **p<0.01. (B) Gain and phase values of WT and Rapgef3/4dKO mice evoked by vestibular stimulation in the dark. *p<0.05. **p<0.01. (C) Gain and phase values of WT and Rapgef3/4-dKO mice during VVOR in the light. With visual input, the VOR deficits in Rapgef3/4-dKO were no longer present. (D) VOR recordings in the dark revealed a reversal of VOR phase in WT and Rapgef3/4-dKO mice. *p<0.05. **p<0.01. ***p<0.001.
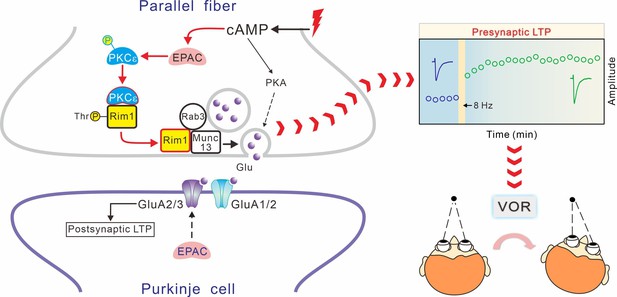
Proposed schematic model for the function of EPAC-PKCε module in presynaptic LTP and motor learning.
In this model, EPAC activation by forskolin or presynaptic tetanus stimulation promotes PKCε activation and phosphorylation threonine of RIM1, which further facilitates the assembly of Rab3A-RIM1-Munc13-1 tripartite complex and the docking of vesicles at active zones. All these events are required for the induction of presynaptic LTP. Importantly, presynaptic LTP is essential to VOR phase adaptation learning.