In-line swimming dynamics revealed by fish interacting with a robotic mechanism
Figures
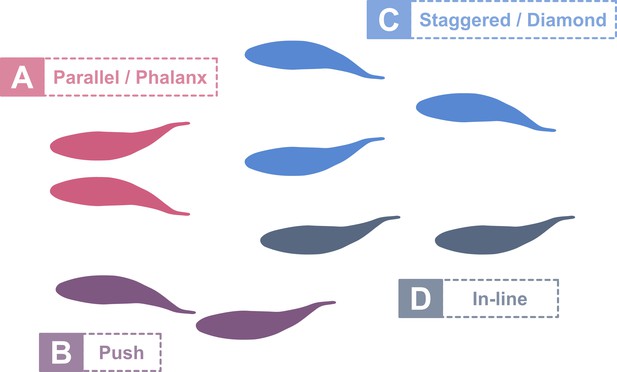
Schooling positions with hydrodynamic benefits.
(A) Swimming side-by-side can increase thrust and efficiency by making use of the channeling effect (Ashraf et al., 2017; Daghooghi and Borazjani, 2015). (B) Leading swimmers benefit from higher thrust production due to increased effective added mass at their trailing edge stemming from the blockage of the water in close proximity to trailing swimmers (Bao and Tao, 2014; Saadat et al., 2021). (C) Trailing fish face reduced oncoming flows between two leading fish when swimming in a diamond formation (4). (D) Leading-edge suction provides propulsive thrust for a fish in a trailing position (Kurt and Moored, 2018; Maertens et al., 2017; Saadat et al., 2021).
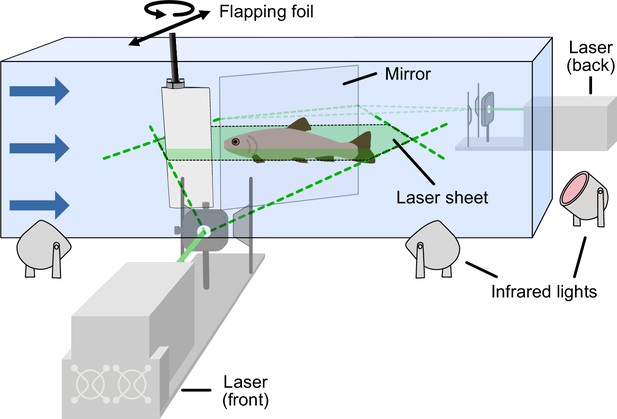
Experimental setup.
Flapping foil with 2 degrees of freedom (yaw and sway) generating a fish-like thrust wake in the flow tank. Trout swam in the dark while we captured the kinematics by means of high-speed cameras from a bottom and side view and using infrared lights for illumination. Low light in the tank upstream of the flapping foil allowed fish to orient. In separate experiments, we captured the flow dynamics using particle image velocimetry. We were able to record the entire flow field around the fish by using two lasers (in front and behind) simultaneously.
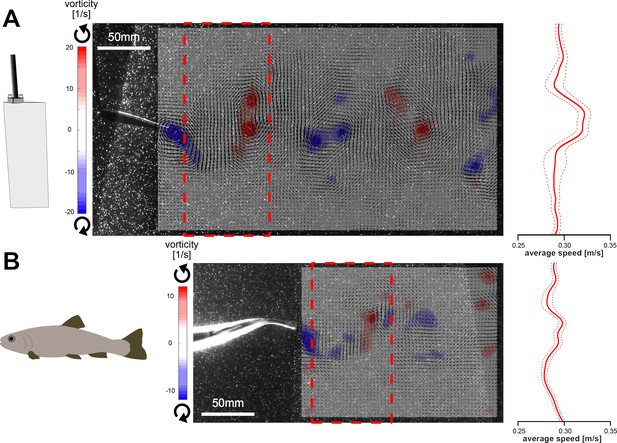
Comparison of flapping foil wake and fish wake.
(A) Velocity field of the wake behind the flapping foil: red and blue regions indicate counterclockwise and clockwise vorticity, respectively. The average speed profile of the region within the dashed rectangular region is shown on the right. (B) Corresponding plots of the wake behind a steadily swimming trout. Note that even though the flapping foil is rigid and the fish tail is flexible, it is possible to parametrize the motion of the robotic flapper to achieve similar tail tip excursions.
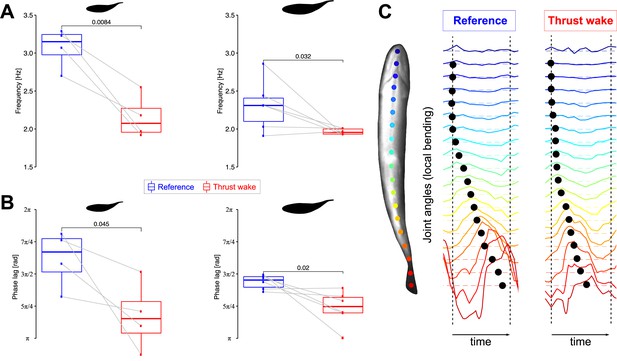
Body kinematics in thrust wakes.
(A) Reduced tail-beat frequencies and (B) reduced overall phase lags for small (n = 4) and large (n = 6) trout swimming in the thrust wake compared to steady swimming at the same flow tank speed. (C) Illustration of the bending pattern by means of joint angles (rainbow colored lines) along the body. Black markers indicate the bending phase.
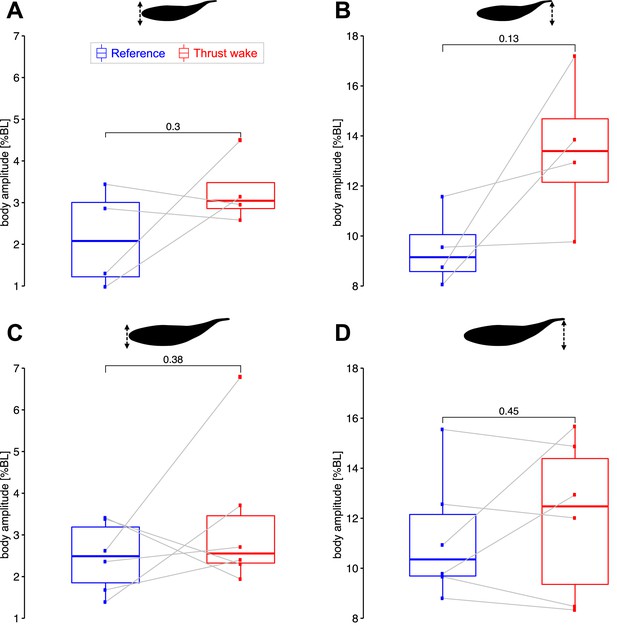
Head and tail amplitudes.
Body amplitudes represented as percentage of the body length (BL) for small (n = 4) (A, B) and large (n = 6) (C, D) trout. Head (A, C) and tail (B, D) amplitudes show a slight trend toward increased tail amplitude in the thrust wake but are not significantly different compared to steady swimming.
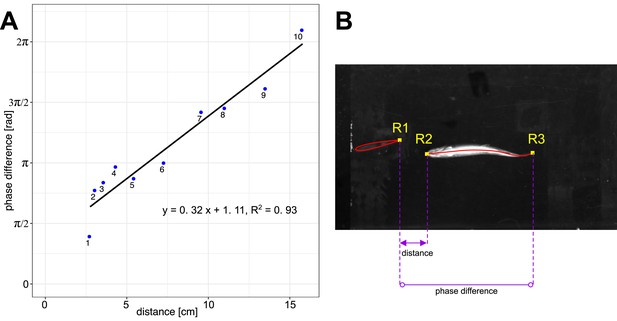
Phase difference between foil and fish.
(A) Linear relationship (n = 10) between phase difference and distance from the foil for fish swimming in-line in the thrust wake. Video 3 shows videos of the individual data points 1–10. (B) Distance is measured between the trailing edge of the foil (R1) and the leading edge of the fish (R2). The phase difference is measured between trailing edges of the foil (R1) and the fish (R3).
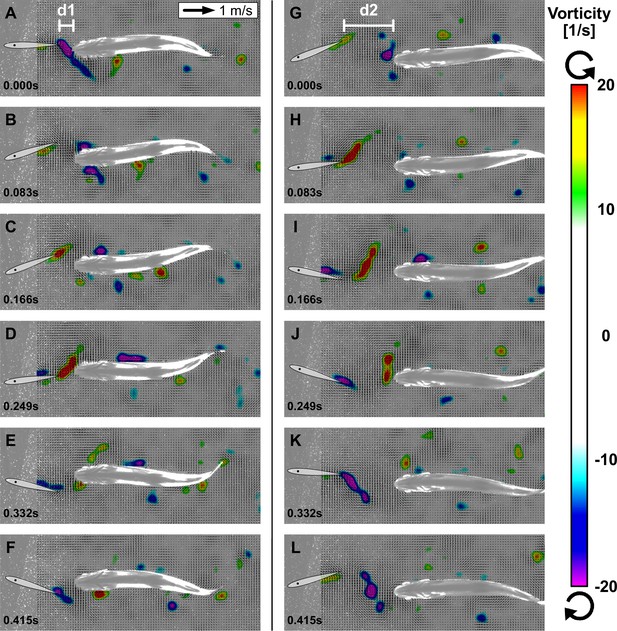
Interactions between fish and vortices.
Two representative sequences over one swimming cycle with ventral view of trout station-holding in the thrust wake near the foil at distance d1 with double-sided vortex interactions (A–F) and located more downstream at d2 with single-sided vortex interactions (G–L). Oncoming vortices from the flapping foil are intercepted by trout in the wake. The vortices stay attached on one side depending on their orientation and ‘roll’ downstream along the body (velocity fields shown after subtraction of mean flow speed).
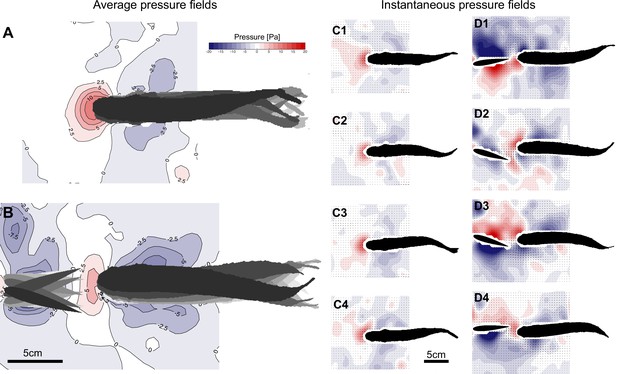
Reduced head pressure in the thrust wake.
Average pressure fields of a trout swimming in free-stream flow (A) and in the thrust wake of a flapping foil (B) show reduced positive pressures (46% decrease) around the head despite increased oncoming flow. Consistent instantaneous positive pressures over time are present under free-stream flow conditions (C1–C4). Corresponding instantaneous pressure fields display alternating positive and negative pressures around the head in the thrust wake over time (D1–D4).
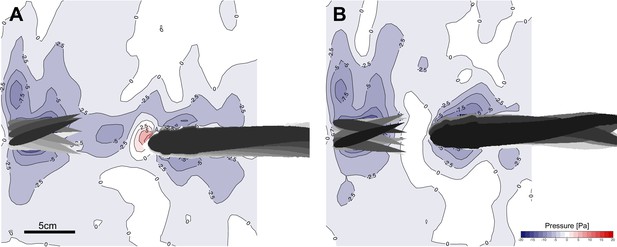
Reduced average head pressures in thrust wakes.
(A) Trout swimming in the thrust wake exploiting single-sided vortex interactions and 45% decrease in head pressure compared to free-stream swimming. (B) Second example of trout exploiting double-sided vortex interactions with 86% decrease in head pressure found.
Videos
Trout swimming in the thrust wake of a flapping foil (bottom and side views).
Time lapse of a trout exploring the flow tank with a thrust wake present (bottom view).
Over time trout position themselves in the thrust wake and synchronize with the flapping foil.
Analysis of phase difference between flapping foil and fish swimming in the thrust wake.
Panels 1-10 show the individual data points and illustrate how the phasing of the tail-beat changes linearly with the distance from the foil. Numbers on the panels correspond to the point numbers in the graph in the lower right, and to those in Figure 3.