Neuronal glutamate transporters control reciprocal inhibition and gain modulation in D1 medium spiny neurons
Figures
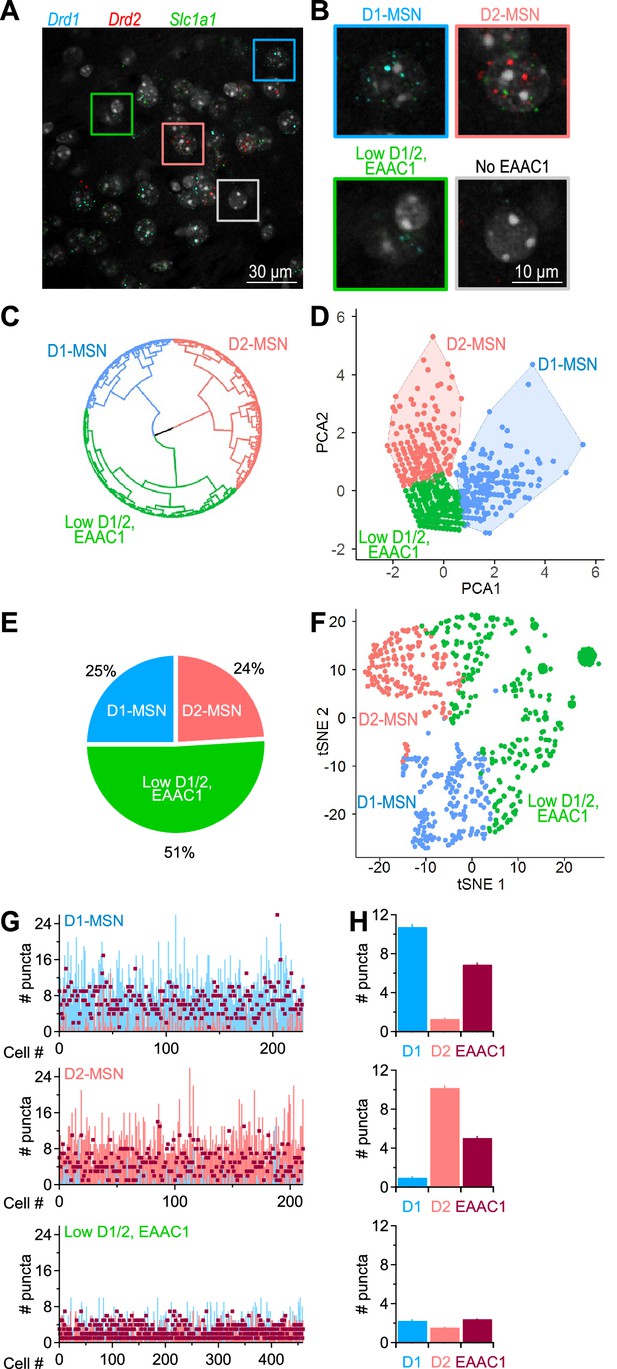
Distribution of EAAC1 mRNA in the DLS.
(A) RNAscope FISH images of Drd1 (blue), Drd2 (red), and Slc1a1 (green) mRNA expression in the DLS. These transcripts encode D1/2 receptors and EAAC1, respectively. (B) Annotated cell clusters identified with color-coded boxes in A, including cells expressing Drd1 (i.e. D1-MSNs; blue), cells expressing Drd2 (i.e. D2-MSNs; salmon), cells with low Drd1/Drd2 /Slc1a1 EAAC1 expression (green), and cells with no detectable Slc1a1 expression (which also had a very low expression of Drd1/Drd2; gray). (C) Circular dendrogram representation of RNAscope FISH data from EAAC1-expressing cells in the DLS. The dendrogram was obtained using the Ward’s minimum variance method for hierarchical cluster analysis, using three as the number of groups cutting the tree (i.e., the optimal number of clusters obtained using gap statistics). The three main groups represent D1-MSNs (blue), D2-MSNs (salmon) and cells that express low levels of D1, D2 and EAAC1 (green). (D) Principal component analysis obtained using an enhanced k-means clustering with the three populations of cells. (E) Pie chart describing the percentage of the cell types identified with the hierarchical clustering. (F) Annotated clusters in the t-SNE map showing three specific groups of cells identified by the k-means algorithm based on their differential expression of D1, D2 and EAAC1. (G) Analysis of mRNA puncta in D1-MSNs (n=229 puncta; blue), D2-MSNs (n=212 puncta, salmon) and low D1/2, EAAC1 (n=460 puncta, burgundy). (H) Summary graph showing the mean number of puncta and S.E.M. measured in each of the three identified cell populations described in C.
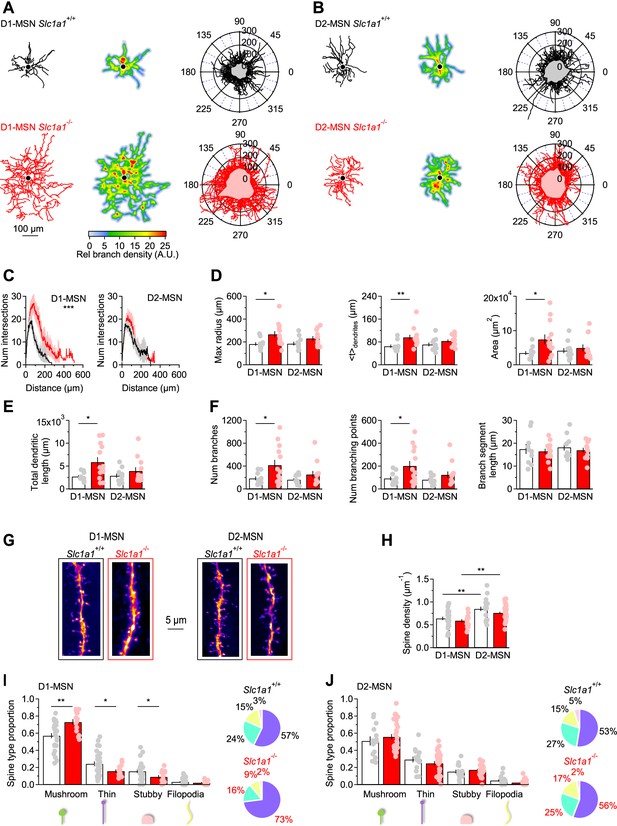
EAAC1 limits the branching pattern and spine size in D1-MSNs.
(A) Left, Example of biocytin-filled and reconstructed D1-MSNs in Slc1a1+/+ (top, black) and Slc1a1-/- mice (bottom, red). Center, heat maps of the reconstructions shown in the left panel. Regions highlighted in red are the areas with the highest branch density. Right, polar plots showing overlaid reconstructions of D1-MSNs in Slc1a1+/+ (n=10 neurons) and Slc1a1-/- mice (n=13 neurons). The gray and pink shaded areas represent the mean coverage area of D1-MSNs in each mouse genotype. (B) As in A, for D2-MSNs in Slc1a1+/+ (n=11 neurons) and Slc1a1-/- mice (n=12 neurons). (C) Left, Sholl plots showing the number of dendritic branch intersections formed by D1-MSNs at increasing distances from the center of the soma, for the D1-MSNs described in A. Right, As in left, for D2-MSNs. (D) Left, The maximum radius of dendritic branches, calculated as the maximum x-value in the Sholl plots in C, is increased in D1-MSNs of Slc1a1-/- mice. Center, The geometric centroid (<t > ) is the center of mass of the Sholl plots, which is the distance from the soma before and after which there is the same density of branches (i.e., number/weight). Right, Analysis of DLS neuropil coverage by MSNs, showing that D1-MSNs occupy a larger domain of the DLS neuropil in Slc1a1-/- mice. (E) The total length of all dendrites is larger in D1-MSNs of Slc1a1-/- mice. (F) Dendritic branch analysis of DLS MSNs. The total number of dendritic branches (left) and the number of branching points (center) are increased in D1-MSNs of Slc1a1-/- compared to Slc1a1+/+ mice. The mean branch length is similar among MSNs of Slc1a1+/+ and Slc1a1-/- mice (right). (G) 2D maximum intensity projections of confocal images of dendritic branches from D1-MSNs (left) and D2-MSNs (right) in Slc1a1+/+ (black frame) and Slc1a1-/- mice (red frame). (H) The density of dendritic spines is larger in D2-MSNs compared to D1-MSNs, in Slc1a1+/+ and Slc1a1-/- mice (Slc1a1+/+ D1-MSNs n=27 neurons, D2-MSNs n=18 neurons; Slc1a1-/- D1-MSN n=18 neurons, D2-MSN n=38 neurons). (I) Spine classification analysis, showing that there is an increase in the proportion of mushroom spines and a decrease in the proportion of thin and stubby spines in D1-MSNs from Slc1a1-/- mice. The pie charts on the right show the distribution of different spine types in D1-MSNs from Slc1a1+/+ (black) and Slc1a1-/- mice (red). Same n values as in H. (J) As in I, for D2-MSNs. Different types of spines are equally represented in D2-MSNs from Slc1a1+/+ and Slc1a1-/- mice. Same n values as in H. Data represent mean ± SEM.
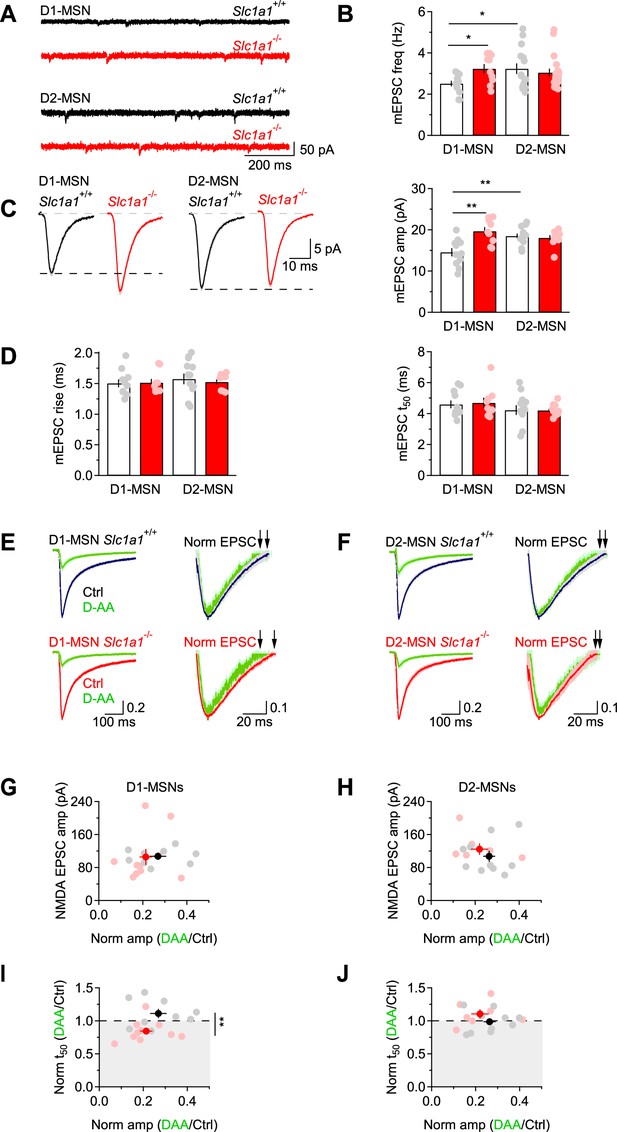
EAAC1 limits excitation in D1-MSNs.
(A) Sample traces of mEPSCs recordings from DLS MSNs in Slc1a1+/+ (black) and Slc1a1-/- mice (red), at Vhold=-70 mV. (B) Summary graph of the mEPSC frequency in DLS MSNs. In Slc1a1+/+ mice, the mEPSC frequency is higher in D2-MSNs (n=15 neurons) compared to D1-MSNs (n=12 neurons). In Slc1a1-/- mice, there is no significant difference in the mEPSC frequency between D1-MSNs (n=10 neurons) and D2-MSNs (n=19 neurons), but the mEPSC frequency is higher in D1-MSNs compared to Slc1a1+/+ mice. Data shown in B-D were collected from the same cells. (C) Left, Average mEPSCs from Slc1a1+/+ and Slc1a1-/- D1- and D2-MSNs. Right, Summary of the mEPSC amplitude. In Slc1a1+/+ mice, the mEPSC amplitude is larger in D2- compared to D1-MSNs. In Slc1a1-/- mice, there is no significant difference in the mEPSC amplitude between D1- and D2-MSNs, but the mEPSC amplitude is larger in D1-MSNs compared to Slc1a1+/+ mice. (D) Summary of the mEPSC rise (left) and 50% decay time (right) measured in D1- and D2-MSNs of Slc1a1+/+ and Slc1a1-/- mice. (E) Left, NMDA EPSCs from D1-MSNs, recorded in Mg2+-free extracellular solution at Vhold=-70 mV, in control conditions (Slc1a1+/+ n=9 neurons: black; Slc1a1-/- n=10 neurons: red) and in the presence of D-AA (100 µM; green). Right, peak normalized NMDA EPSCs, shown with a y-axis range of 0.5–1. This allows visualizing the time used for the calculations of the t50, highlighted by the black arrows. Each trace represents the average of 20 consecutive trials. (F) As in E, for D2-MSNs (Slc1a1+/+ n=10 neurons; Slc1a1-/- n=7 neurons). (G) Summary scatter plot showing the amplitude of NMDA EPSCs and its reduction by D-AA in D1-MSNs of Slc1a1+/+ and Slc1a1-/-mice. (H) As in G, for D2-MSNs. (I) Summary scatter plot showing that D-AA reduced the NMDA EPSC amplitude to the same extent in Slc1a1+/+ and Slc1a1-/- D1-MSNs but reduced the t50 only in D1-MSNs from Slc1a1-/- mice. (J) As in I, for D2-MSNs. Data represent mean ± SEM.
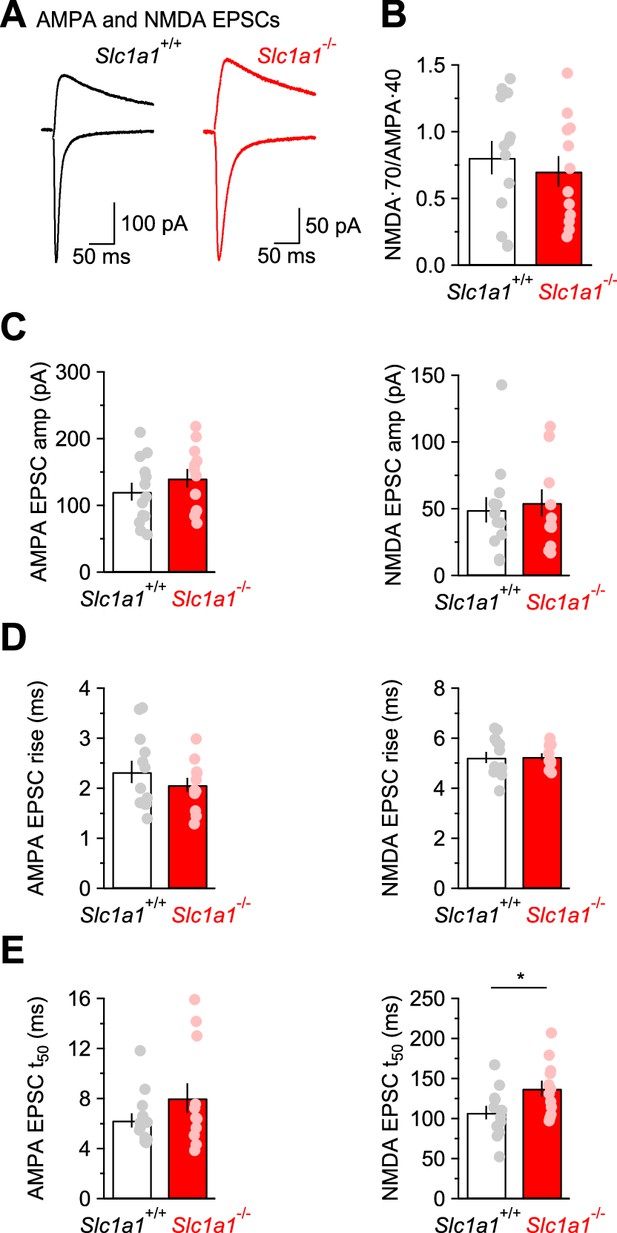
NMDA/AMPA ratio in striatal MSNs.
(A)Left, Example of AMPA EPSCs recorded at Vhold=-70 mV and NMDA EPSCs recorded at Vhold = 40 mV in a D1-MSN from an Slc1a1+/+ mouse. Each trace is the average of 20 consecutive trials. Right, as in the left panel, for an Slc1a1-/- mouse. (B–E) Summary of the NMDA/AMPA ratio weighted by the driving force of the currents mediated by these receptors (B), evoked AMPA and NMDA EPSC amplitude (C), 20–80% rise time (D) and 50% decay time (E) measured in D1-MSNs of Slc1a1+/+ (n=13 neurons) and Slc1a1-/- mice (n=12 neurons). Data represent mean ± SEM.
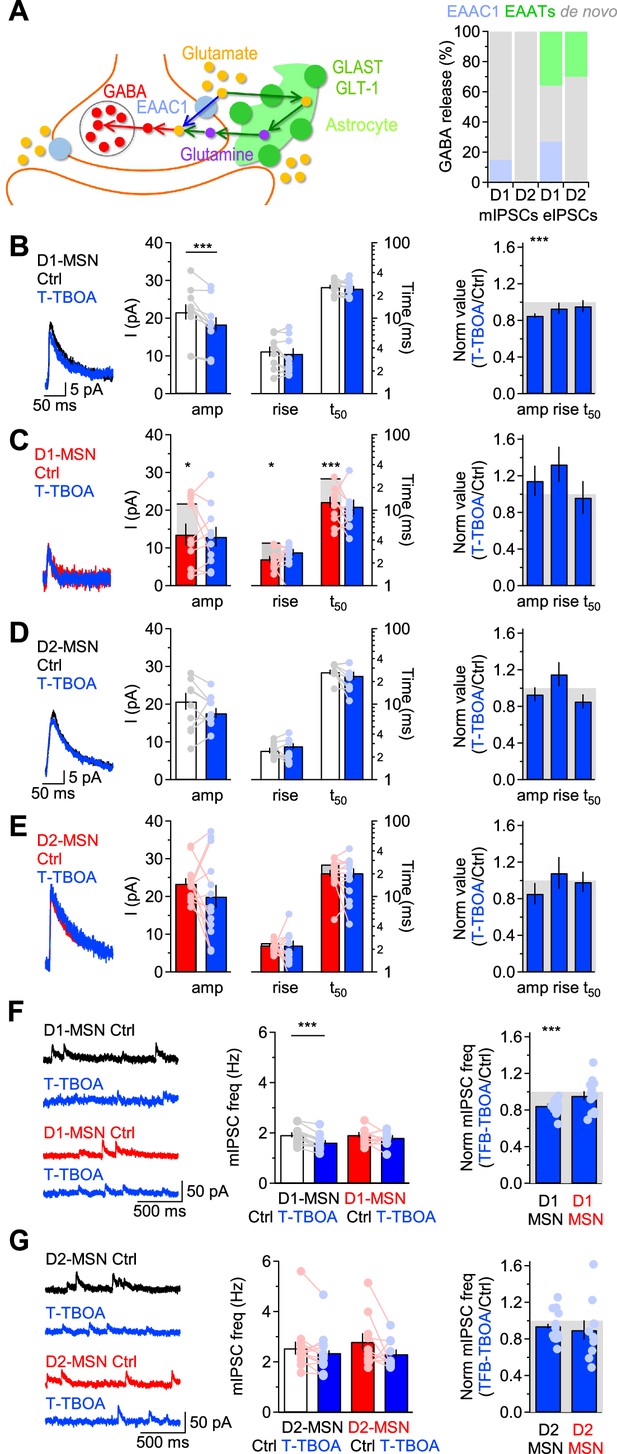
EAAC1 strengthens action potential-independent GABAergic inhibition onto D1-MSNs.
(A)Left, Schematic representation for routes of glutamate uptake via neuronal and glial glutamate transporters. Right, Summary of the contribution of glutamate uptake via EAAC1, EAATs and de novo GABA synthesis to action potential-independent GABA release. (B) Left, Example of GABA mIPSCs recorded from D1-MSNs of Slc1a1+/+ mice in control conditions (black) and in the presence of T-TBOA (1 µM; blue), at Vhold = 40 mV. Center, Summary of the mIPSC amplitude and kinetics recorded before and after T-TBOA (n=11 neurons). Right, Summary of the relative effect of T-TBOA on the mIPSC amplitude and kinetics. T-TBOA induced a significant reduction in the amplitude of mIPSCs recorded from D1-MSNs. (C–E) As in B, for DLS D1-MSNs of Slc1a1-/- mice (n=10 neurons), and D2-MSNs of Slc1a1+/+ mice (n=15 neurons), and Slc1a1-/- mice (n=13 neurons), respectively. In panels C, E we also included data collected from Slc1a1+/+ mice to show that loss of EAAC1 leads to smaller and faster mIPSCs in D1-MSNs, whereas no significant difference is detected between D2-MSNs of Slc1a1+/+ and Slc1a1-/- mice. (F) Summary of the effect of T-TBOA on the mIPSC frequency in D1-MSNs of Slc1a1+/+ and Slc1a1-/- mice, with representative traces (left), raw data (center) and normalized values (right). (G) As in F, for D2-MSNs. Data represent mean ± SEM.
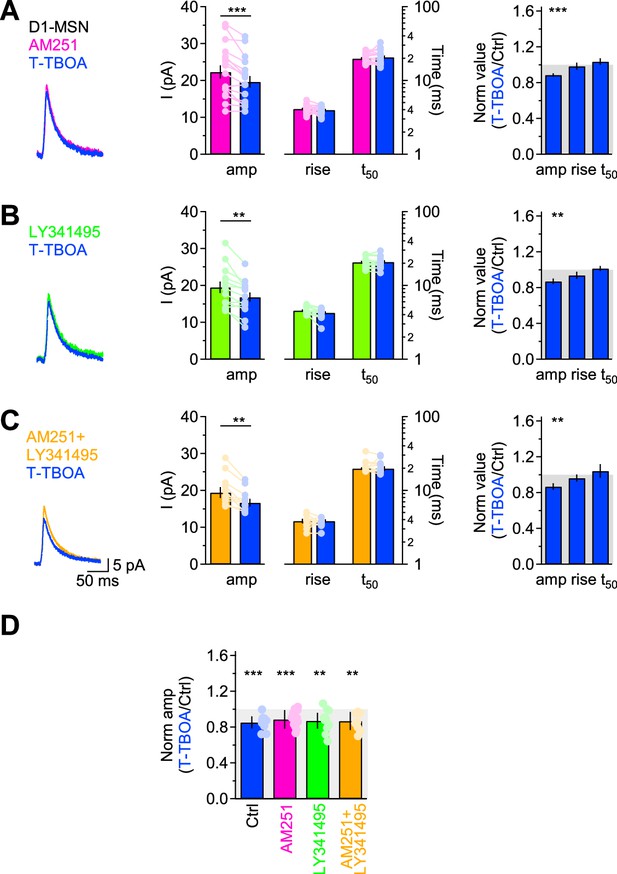
T-TBOA reduces action potential-independent GABAergic inhibition onto D1-MSNs in the presence of CB1 and mGluRI-III receptor antagonists.
(A)Left, Example of mIPSCs recorded from DLS D1-MSNs of Slc1a1+/+ mice in the presence of the CB1 receptor antagonist AM251 (2 µM), before (magenta) and after T-TBOA (1 µM; blue). Center and right, Summary of the mIPSC amplitude and kinetics recorded before and after T-TBOA (n=17 neurons). (B) As in A, for mIPSCs recorded in the presence of the mGluR antagonist LY341495 (100 µM), before (green) and after T-TBOA (n=12 neurons). T-TBOA reduced the mIPSC amplitude without changing the kinetics. (C) As in A, for mIPSCs recorded in the presence of both AM251 (2 µM) and LY341495 (100 µM) in the recording solution (orange, n=10 neurons). T-TBOA reduced the mIPSC amplitude without changing the kinetics. (D) Summary of the relative effect of T-TBOA on the mIPSC amplitude in control conditions (blue), and when applied in the presence of AM251 (magenta), LY341495 (green), and AM251 +LY341495 (orange), respectively. The effect of T-TBOA on the mIPSC amplitude was similar in these four experimental conditions. Data represent mean ± SEM.
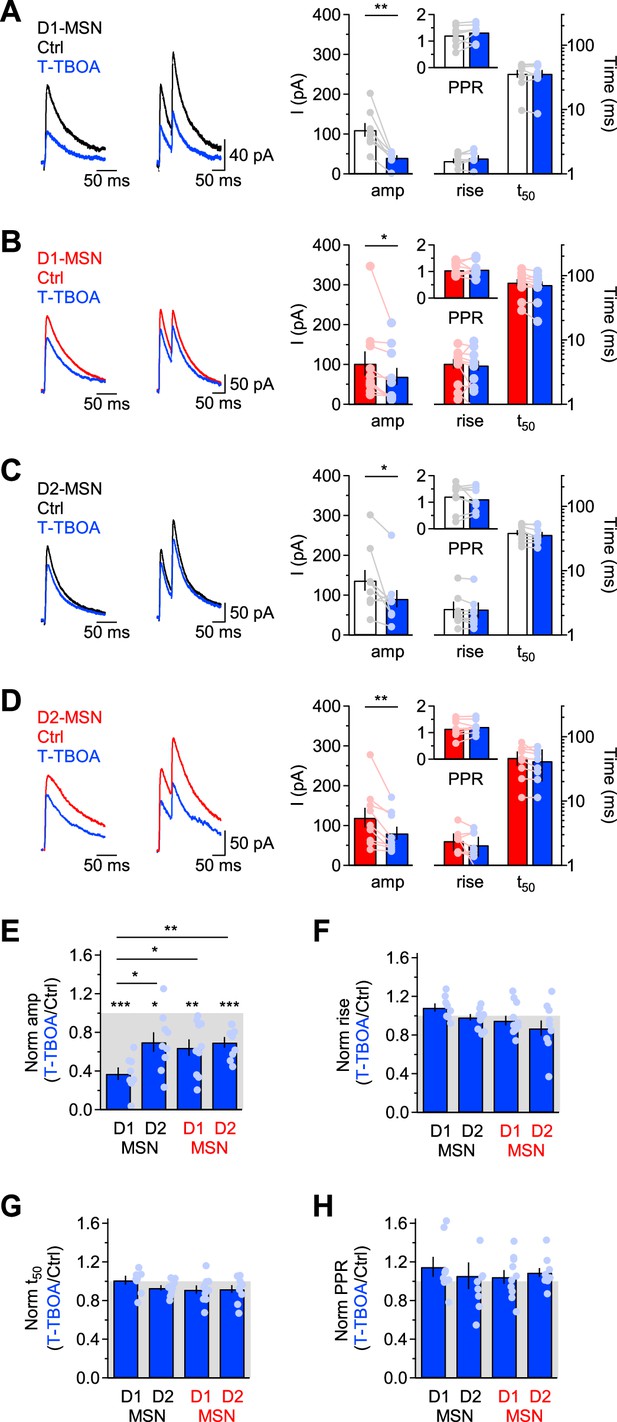
EAAC1 strengthens action potential-dependent GABAergic inhibition onto D1-MSNs.
(A)Left and center, Example of evoked single and paired IPSCs recorded from D1-MSNs of Slc1a1+/+ mice in control conditions (black) and in the presence of T-TBOA (1 µM; blue), at Vhold = 40 mV. Right, Summary of the IPSC amplitude and kinetics recorded before and after T-TBOA (n=8 neurons). The inset represents the summary values of the PPR. (B–D) As in A, for DLS D1-MSNs of Slc1a1-/- mice (n=10 neurons) and D2-MSNs of Slc1a1+/+ mice (n=9 neurons), and Slc1a1-/- mice (n=9 neurons), respectively. (E–H) Summary of the relative effect of T-TBOA on the IPSC amplitude and kinetics. T-TBOA induced a significant reduction on the IPSC amplitude, but not of the rise time, 50% decay time and PPR. T-TBOA reduced the IPSC amplitude in D1-MSNs of Slc1a1+/+ mice more than in any other type of MSNs tested in the experiments. Data represent mean ± SEM.
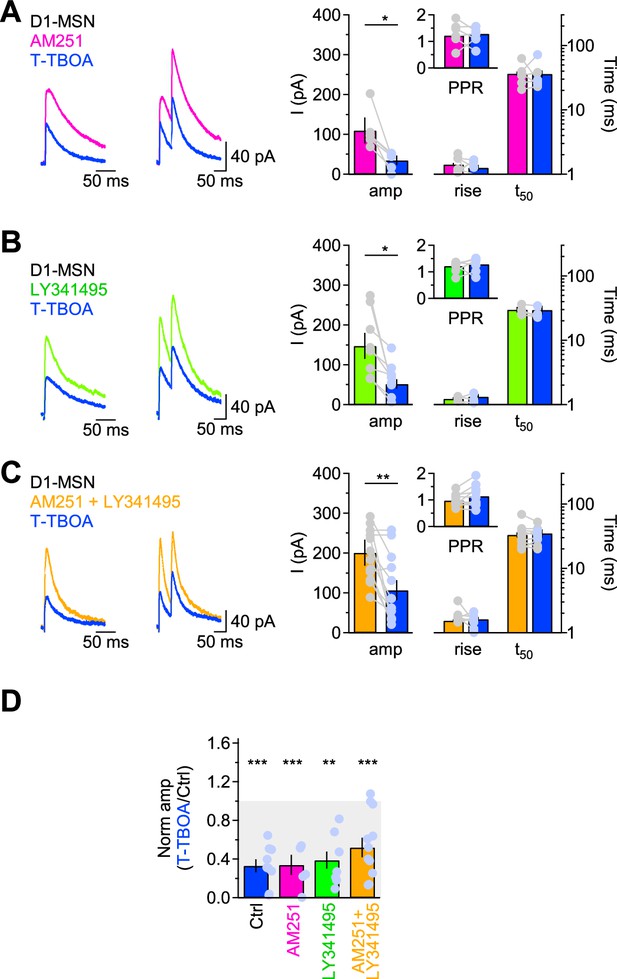
T-TBOA reduces action potential-dependent GABAergic inhibition onto D1-MSNs in the presence of CB1 and mGluRI-III receptor antagonists.
(A) Left, Example of evoked single and paired IPSCs recorded from DLS D1-MSNs of Slc1a1+/+ mice in the presence of the CB1 receptor antagonist AM251 (2 µM), before (magenta) and after T-TBOA (1 µM; blue). Center and right, Summary of the IPSC amplitude and kinetics recorded before and after T-TBOA (n=6 neurons). The inset represents the summary values of the PPR. T-TBOA reduced the IPSC amplitude without changing the kinetics and PPR. (B) As in A, for IPSCs recorded in the presence of the mGluR antagonist LY341495 (100 µM), before (green) and after T-TBOA (n=7 neurons). T-TBOA reduced the IPSC amplitude without changing the kinetics and PPR. (C) As in A, for IPSCs recorded in the presence of both AM251 (2 µM) and LY341495 (100 µM) in the recording solution (orange, n=12 neurons). T-TBOA reduced the IPSC amplitude without changing the kinetics and PPR. (D) Summary of the relative effect of T-TBOA on the IPSC amplitude in control conditions (blue), and when applied in the presence of AM251 (magenta), LY341495 (green), and AM251 +LY341495 (orange), respectively. The effect of T-TBOA on the IPSC amplitude was similar in these four experimental conditions. Data represent mean ± SEM.
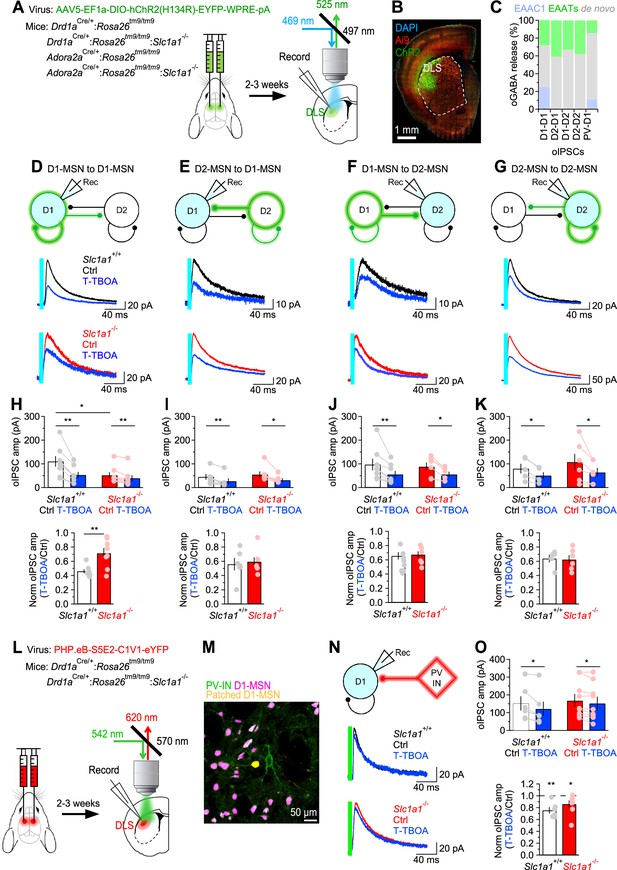
EAAC1 strengthens GABAergic inhibition at D1-D1 synapses.
(A) Schematics of viral transduction in the DLS and light evoked stimulation of ChR2-transfected MSNs in slices. (B) Confocal image of mouse coronal slice transfected with ChR2. (C) Right, Summary of the contribution of glutamate uptake via EAAC1, EAATs and de novo GABA synthesis to action potential-dependent GABA release evoked by light activation of ChR2 at different sets of synapses. (C) Summary of the contribution of different types of glutamate transporters and de novo GABA synthesis to GABA released during oIPSCs. (D) Top, Schematic representation of the experimental design. The patched cell was voltage clamped at Vhold = 20 mV, the reversal potential of ChR2 photocurrents. Middle, Example of oIPSCs recorded from D1-MSNs in response to optogenetic stimulation of GABA release from other D1-MSNs, in mice expressing EAAC1. Each trace represents the average of 20 consecutive trials. Bottom, As in the middle panel, for Slc1a1-/- mice. (E–G) As in D, for D2-D1 (E), D1-D2 (F) and D2-D2 synapses (G). (H) Top, In-cell comparison of D1-D1 oIPSCs in Slc1a1+/+ (n=8 neurons) and Slc1a1-/- mice (n=8 neurons), before and after T-TBOA (1 µM; blue). Bottom, Summary of the peak normalized oIPSC amplitude. (I) As in H, for D2-D1 oIPSCs in Slc1a1+/+ (n=6 neurons) and Slc1a1-/- mice (n=7 neurons). (J) As in H, for D1-D2 oIPSCs in Slc1a1+/+ (n=8 neurons) and Slc1a1-/- mice (n=6 neurons). (K) As in H, for D2-D2 oIPSCs in Slc1a1+/+ (n=5 neurons) and Slc1a1-/- mice (n=6 neurons). (L) Schematics of viral transduction in the DLS and light evoked stimulation of C1V1-transfected PV-INs in slices. (M) Confocal image of tdTomato expressing D1-MSNs (magenta) and C1V1 transfected PV-INs (green) in a slice from which we recorded oIPSCs from a D1-MSN (yellow). (N) As in D, for oIPSCs recorded from D1-MSNs in response to green light activation of C1V1 expressed in PV-INs. (O) As in H, for oIPSCs recorded in response to C1V1 activation of GABA release from PV-INs to D1-MSNs (Slc1a1+/+ n=6 neurons; Slc1a1-/- n=9 neurons). Data represent mean ± SEM.
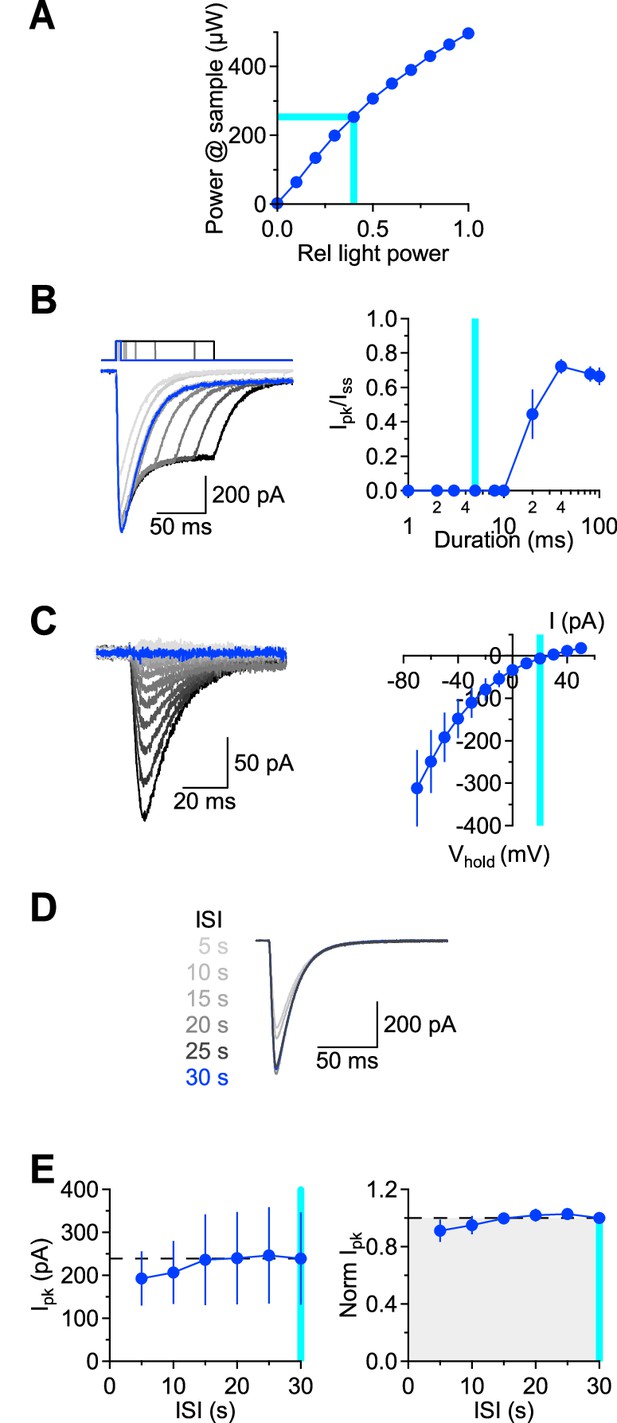
Optimization of stimulation parameters for optogenetic stimulation.
(A) Power output of the light source used for the slice optogenetics experiments. Our experiments were performed using stimulation intensities leading ~250 µW power at the sample plane, measured using a power meter tuned to 488 nm. (B) Left, ChR2-mediated photocurrents recorded from MSNs in Slc1a1+/+ mice at Vhold=-70 mV, in response to light pulses of increasing duration (1–100ms). Each trace represents the average of 20 consecutive trials, acquired at 30 s inter-pulse intervals. The current evoked by a 5 ms-long stimulation, which we used in the rest of our experiments, is highlighted in blue. Right, Quantification of the amplitude ratio between the peak and steady state values of the ChR2-mediated photocurrents. Light pulses of 5ms duration (cyan band) did not evoke a steady-state current (n=9 neurons). (C) Left, Example of ChR2-mediated photocurrents recorded at different holding potentials. Right, I/V profile of ChR2-mediated photocurrents, with an estimated reversal potential of 20 mV (cyan band; n=7 neurons). (D) Left, Example of ChR2 photocurrents recorded using different inter-stimulus intervals (ISIs). (E) Left, Peak amplitude of ChR2-mediated photocurrents recorded at different ISIs. No rundown was detected using 30 s ISIs, which is the value that we used in all other experiments presented here (cyan band; n=7 neurons). Right, As in the left panel, with results normalized by peak amplitude of ChR2 photocurrents recorded with ISI = 30 s. Data represent mean ± SEM.
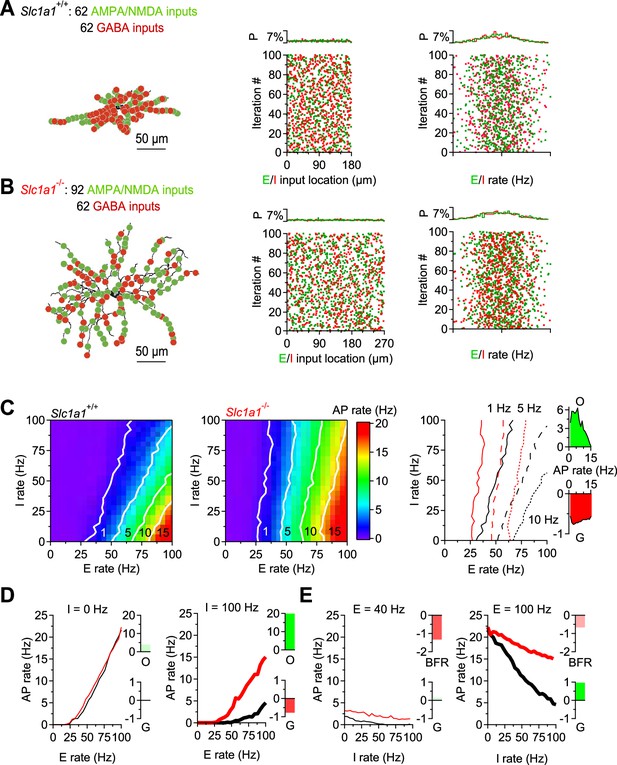
EAAC1 reduces the firing output of D1-MSNs in a realistic compartmental model of D1-MSNs.
(A)Left, Representation of a biocytin-filled D1-MSN in Slc1a1+/+ mice, with excitatory (green) and inhibitory inputs (red) randomly distributed along all dendrites. Center, Spatial distribution of excitatory and inhibitory inputs along the length of the dendrites, Right, Schematic representation of the instantaneous frequency of activation of excitatory and inhibitory inputs in each of the 100 simulation iterations. The activation frequency for these inputs was set to have a Gaussian distribution. (B) As in A, for a biocytin-filled D1-MSN in Slc1a1-/- mice. (C) Left, Heat map showing the firing output of D1-MSNs in Slc1a1+/+ mice for different combinations of activity for excitatory (x-axis) and inhibitory inputs (y-axis). Center, as in the right panel, for Slc1a1-/- mice. Right, Overlaid contour lines for action potential firing at 1, 5, and 10 Hz in D1-MSNs from Slc1a1+/+ and Slc1a1-/- mice. (D) Left, Output firing frequency-input frequency relationships for synaptic excitation for D1-MSNs of Slc1a1+/+ (black) and Slc1a1-/- mice (red), in the absence (thin curves) of synaptic inhibition. Right, As in the left panel for high inhibition levels (i.e. 100 Hz; thick curves). (E) As in D, to compare the effect of inhibition at varying levels of excitation in D1-MSNs of Slc1a1+/+ and Slc1a1-/- mice. Data represent means from 100 simulations.
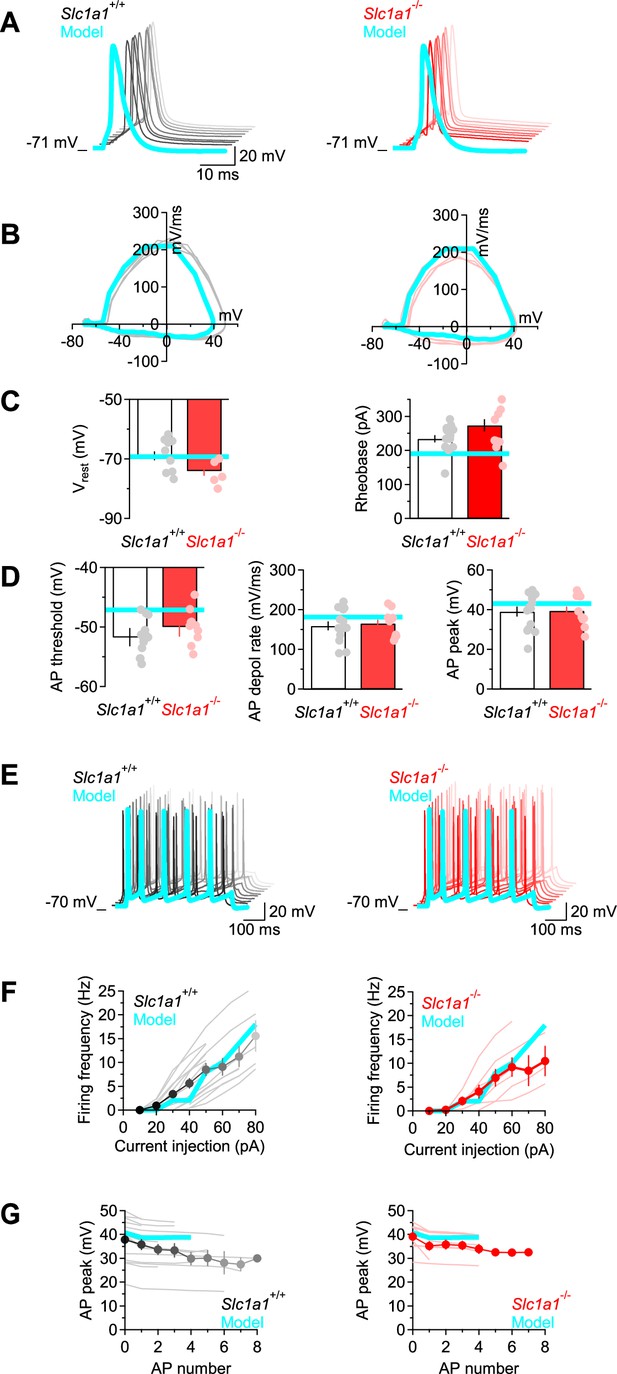
Optimization of ball-and-stick NEURON model of D1-MSN excitability.
(A)Left, Example of action potentials evoked by brief somatic depolarizing steps delivered to a D1-MSN from Slc1a1+/+ mice (150 pA · 5ms). The action potential evoked by the NEURON model is superimposed to the traces (cyan). Right, As in the left panel, for a D1-MSN of Slc1a1-/- mice (150 pA · 5ms). (B) Phase plots of the action potentials recorded in the experiments shown in A (gray and pink for Slc1a1+/+ and Slc1a1-/- mice, respectively) and in the NEURON model (cyan). (C) Left, Values of the resting membrane potential measured experimentally in D1-MSNs of Slc1a1+/+ (n=11 neurons) and Slc1a1-/- mice (n=10 neurons) and used in the NEURON model (cyan line). Right, As in the left panel, for the rheobase levels in D1-MSNs of Slc1a1+/+ (n=14 neurons) and Slc1a1-/- (n=10 neurons). (D) As in C, for the action potential threshold (left), peak (center) and depolarization rate (right). (E) Left, Example of trains of action potentials evoked by prolonged somatic depolarizing steps delivered to a D1-MSN from Slc1a1+/+ mice (50 pA · 500ms). The train of action potentials evoked by the NEURON model is superimposed to the traces (cyan). Right, As in the left panel, for a D1-MSN from Slc1a1-/- mice (50 pA · 500ms). (F) Left, Frequency/current (f/I) plots obtained using 500ms somatic current step injections in D1-MSNs from Slc1a1+/+ mice (n=14 neurons). Right, As in the left panel, for D1-MSNs from Slc1a1-/- mice (n=10 neurons). (G) Average peak of each action potential in a train evoked using 50 pA · 500ms somatic current injections. Data represent mean ± SEM.
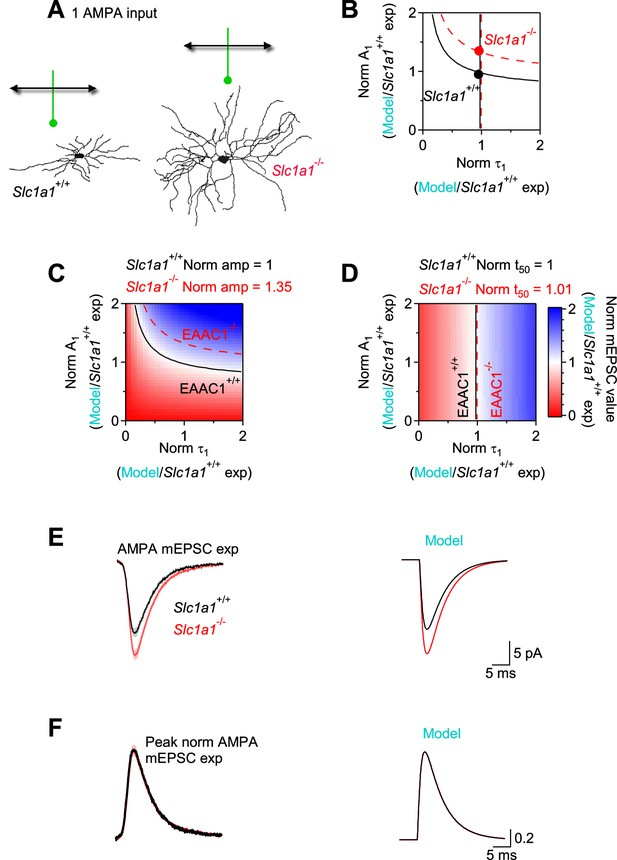
Optimization of synaptic weight for excitatory synapses containing AMPA receptors, using a NEURON model of D1-MSNs with realistic morphology.
(A) Schematic representation of the ball-and-stick NEURON compartmental model of D1-MSNs, with one AMPA input (green) located at varying distances from the soma. The location of the input was selected randomly and results of 100 simulations were averaged to obtain the data shown in panels B-D. (B) The contour plots represent an overlay of those described in panels C,D. The dots represent the only combination of A1 and τ1 values (where ), which allow our model to capture the experimentally detected differences in AMPA mEPSC amplitude and decay time. (C) The colored matrix represents the peak normalized AMPA mEPSC amplitude obtained using different combinations of A1 and τ1. The contour plots describe the infinite number of possible solutions for currents recorded from our ball-and-stick model of D1-MSNs in Slc1a1+/+ (black curve) and Slc1a1-/- mice (red dashed curve). The red dashed contour plot is obtained setting the normalized mEPSC amplitude to 1.35, as indicated above the plot. (D) The colored matrix represents the normalized AMPA mEPSC 50% decay time obtained using different combinations of A1 and τ1. The contour plots describe the infinite number of possible solutions for currents recorded from our ball-and-stick model of D1-MSNs in Slc1a1+/+ (black line) and Slc1a1-/- mice (red dashed line). The red dashed contour plot is obtained setting the normalized mEPSC t50 to 1.01, as indicated above the plot. (E) Left, overlay of AMPA mEPSCs recorded from D1-MSNs in Slc1a1+/+ (black) and Slc1a1-/- mice (red). Each trace represents the average of mEPSCs recorded from Slc1a1+/+ (n=12 neurons) and Slc1a1-/- D1-MSNs (n=10 neurons). Right, overlay of modeled AMPA mEPSCs in D1-MSNs from Slc1a1+/+ (black) and Slc1a1-/- mice (red). (F) As in E, after peak normalization.
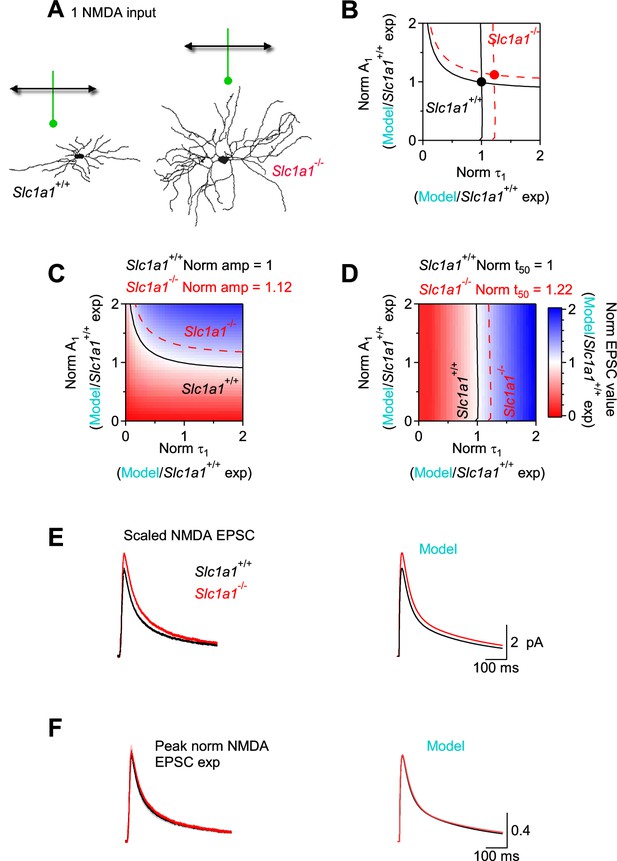
Optimization of synaptic weight for excitatory synapses containing NMDA receptors, using a NEURON model of D1-MSNs with realistic morphology.
(A) Schematic representation of the ball-and-stick NEURON compartmental model of D1-MSNs, with one NMDA input (green) located at varying distances from the soma. The location of the input was selected randomly and results of 100 simulations were averaged to obtain the results shown in panels B-D. (B) The contour plots represent an overlay of those described in panels C, D. The dots represent the only combination of A1 and τ1 values that allow our model to capture the experimentally detected differences in NMDA EPSC amplitude and decay time, after their amplitude was scaled based on the amplitude of AMPA mEPSCs and the NMDA/AMPA ratio. (C) The colored matrix represents the peak normalized NMDA EPSC amplitude obtained using different combinations of A1 and τ1. The contour plots describe the infinite number of possible solutions for currents recorded from our ball-and-stick model of D1-MSNs in Slc1a1+/+ (black curve) and Slc1a1-/- mice (red dashed curve). The red dashed contour plot is obtained setting the normalized EPSC amplitude to 1.12, as indicated above the plot. (D) The colored matrix represents the estimated normalized NMDA mEPSC 50% decay time obtained using different combinations of A1 and τ1. The contour plots describe the infinite number of possible solutions for currents recorded from our ball-and-stick model of D1-MSNs in Slc1a1+/+ (black line) and Slc1a1-/- mice (red dashed line). The red dashed contour plot is obtained setting the normalized mEPSC t50 to 1.01, as indicated above the plot. (E) Left, overlay of NMDA mEPSCs estimated from recordings of evoked NMDA EPSC currents and AMPA/NMDA ratios in D1-MSNs in Slc1a1+/+ (black) and Slc1a1-/- mice (red). Each trace represents the average of scaled NMDA EPSCs recorded from Slc1a1+/+ (n=11 neurons) and Slc1a1-/- D1-MSNs (n=11 neurons). Right, overlay of modeled NMDA mEPSCs in D1-MSNs from Slc1a1AC1+/+ (black) and Slc1a1-/- mice (red). (F) As in E, after peak normalization.
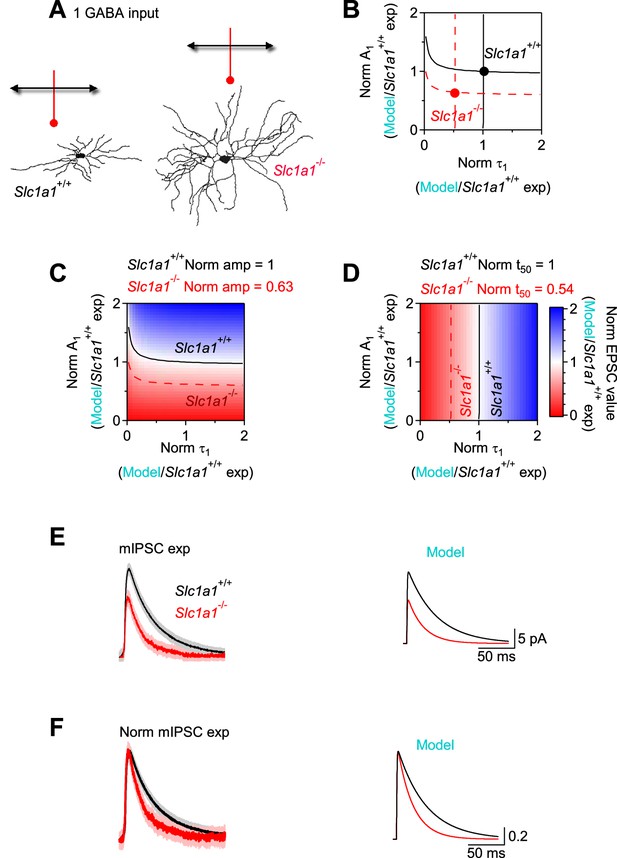
Optimization of synaptic weight for inhibitory synapses containing GABAA receptors, using a NEURON model of D1-MSNs with realistic morphology.
(A) Schematic representation of the ball-and-stick NEURON compartmental model of D1-MSNs, with one GABA input (red) located at varying distances from the soma. The location of the input was selected randomly and results of 100 simulations were averaged to obtain the results shown in panels B-D. (B) The contour plots represent an overlay of those described in panels C, D. The dots represent the only combination of A1 and τ1 values that allow our model to capture the experimentally detected differences in GABA mIPSC amplitude and decay time. (C) The colored matrix represents the peak normalized GABA mIPSC amplitude obtained using different combinations of A1 and τ1. The contour plots describe the infinite number of possible solutions for currents recorded from our ball-and-stick model of D1-MSNs in Slc1a1+/+ (black curve) and Slc1a1-/- mice (red dashed curve). The red dashed contour plot is obtained setting the normalized EPSC amplitude to 0.63, as indicated above the plot. (D) The colored matrix represents the estimated normalized NMDA mEPSC 50% decay time obtained using different combinations of A1 and τ1. The contour plots describe the infinite number of possible solutions for currents recorded from our ball-and-stick model of D1-MSNs in Slc1a1+/+ (black line) and Slc1a1-/- mice (red dashed line). The red dashed contour plot is obtained setting the normalized mIPSC t50 to 0.54, as indicated above the plot. (E) Left, overlay of GABA mIPSCs from recordings in D1-MSNs of Slc1a1+/+ (black) and Slc1a1-/- mice (red). Each trace represents the average of GABA mIPSCs recorded from Slc1a1+/+ (n=11 neurons) and Slc1a1-/- D1-MSNs (n=10 neurons). Right, overlay of modeled GABA mIPSCs in D1-MSNs from Slc1a1+/+ (black) and Slc1a1-/- mice (red). (F) As in E, after peak normalization.
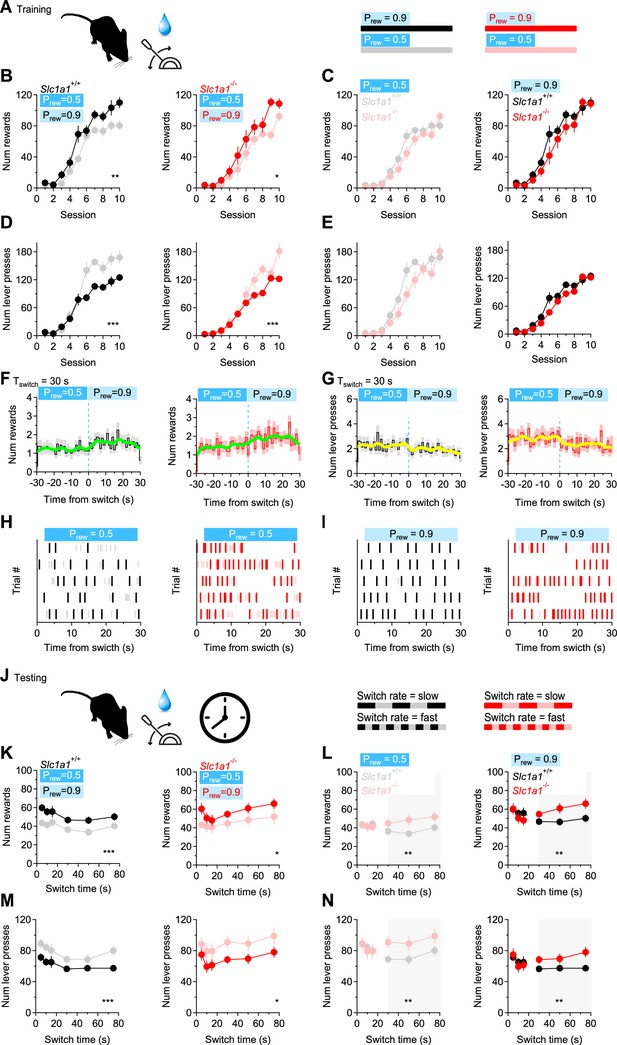
EAAC1 shapes slow-switching behaviors.
(A) Schematic representation of the training sessions, with water rewards delivered at two different reward probabilities (Prew), during each 5 min session. (B) Number of rewards collected by Slc1a1+/+ (n=16 mice) and Slc1a1-/- mice (n=14 mice), at Prew = 0.5 and Prew = 0.9 over the course of 10 training sessions. (C) Comparison between Slc1a1+/+ and Slc1a1-/- mice for the data shown in B. (D, E) As in B, C, showing the total number of lever presses performed by the mice. (F) Temporal distribution of the number of rewards collected by Slc1a1+/+ (left) and Slc1a1-/- mice (right) when the reward probability was switched from Prew = 0.5 to Prew = 0.9. The thick green line represents a binomial smoothing of the mean. (G) As in F, for the number of lever presses. (H) Raster plot showing the temporal distribution of lever presses in a 5 min long trial, in which the reward probability was switched from Prew = 0.5 to Prew = 0.9 every 30 s. The raster plots in this panel were collected at Prew = 0.5. Color-coded tick marks (gray/pink) represent the times when the lever was pressed. Other tick marks (black/red) represent the times when the water rewards were collected. (I) As in H, for Prew = 0.9. (J) Schematic representation of the test session, with water rewards delivered at two different values of Prew, switching at different time intervals (5–75 s). (K–N) As in B-E, for the test session. Data represent mean ± SEM.
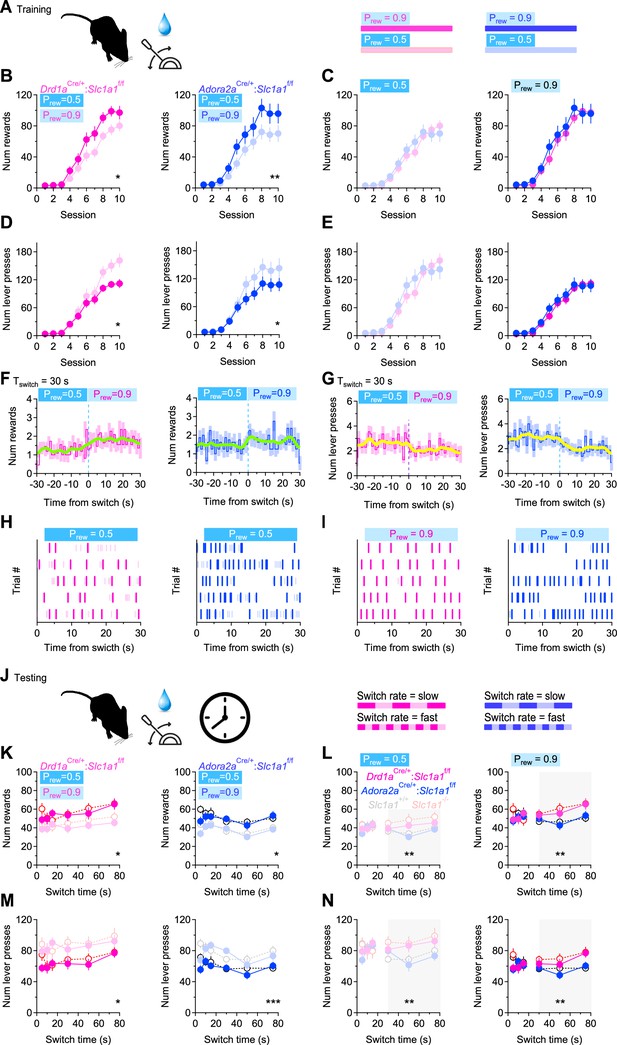
EAAC1 expression in D1-MSNs shapes slow-switching behaviors.
(A) Schematic representation of the training sessions, with water rewards delivered at two different reward probabilities (Prew), during each 5 min session. (B) Number of rewards collected by Drd1aCre/+:Slc1a1f/f (n=15 mice) and Adora2aCre/+:Slc1a1f/f mice (n=17 mice), at Prew = 0.5 and Prew = 0.9 over the course of 10 training sessions. (C) Comparison between D1Cre/+:Slc1a1f/f and Adora2aCre/+:Slc1a1f/f mice for the data shown in B. (D, E) As in B, C, showing the total number of lever presses performed by the mice. (F) Temporal distribution of the number of rewards collected by Drd1aCre/+:Slc1a1f/f (left) and Adora2aCre/+:Slc1a1f/f mice (right) when the reward probability was switched from Prew = 0.5 to Prew = 0.9. The thick green line represents a binomial smoothing of the mean. (G) As in F, for the number of lever presses. (H) Raster plot showing the temporal distribution of lever presses in a 5 min long trial, in which the reward probability was switched from Prew = 0.5 to Prew = 0.9 every 30 s. The raster plots in this panel were collected at Prew = 0.5. Pink/light blue ticks represent the times when the lever was pressed. Magenta/blue ticks represent the times when the water rewards were collected. (I) As in H, for Prew = 0.9. (J) Schematic representation of the test session, with water rewards delivered at two different values of Prew, switching at different time intervals (5–75 s). (K–N) As in B-E, for the test session. Data represent mean ± SEM.
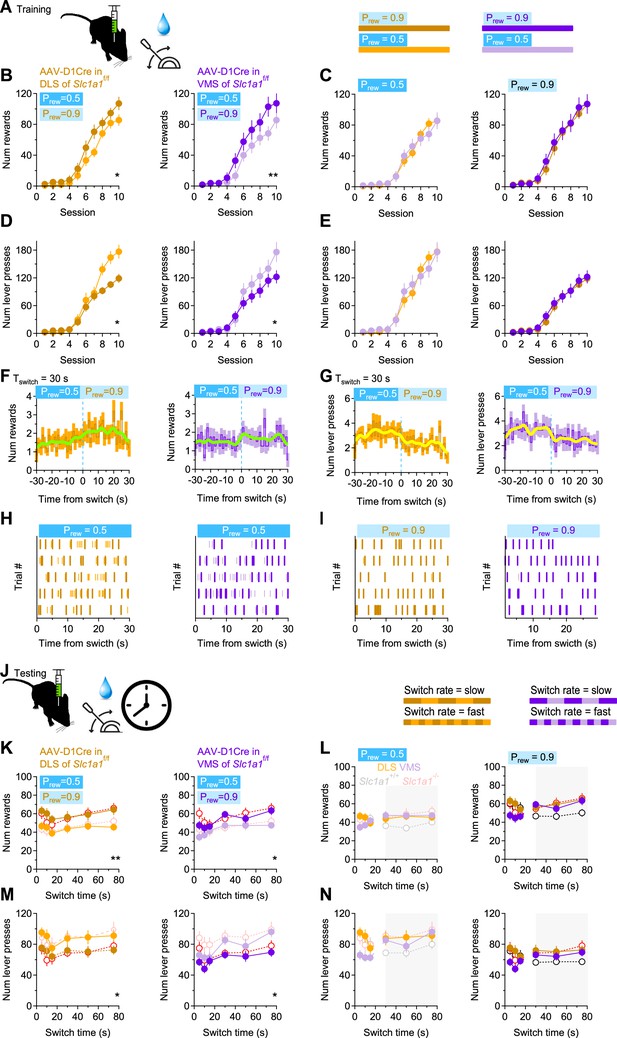
EAAC1 expression in D1-MSNs of the DLS and VMS shapes slow-switching behaviors.
(A) Schematic representation of the training sessions, with water rewards delivered at two different reward probabilities (Prew), during each 5 min session. (B) Number of rewards collected by Slc1a1f/f mice transfected with AAV-D1Cre in the DLS (n=11 mice) and VMS (n=12 mice), at Prew = 0.5 and Prew = 0.9 over the course of 10 training sessions. (C) Comparison between Slc1a1f/f mice injected with AAV-D1Cre in the DLS and VMS for the data shown in B. (D, E) As in B, C, showing the total number of lever presses performed by the mice. (F) Temporal distribution of the number of rewards collected by Slc1a1f/f mice injected with AAV-D1Cre in the DLS a (left) and VMS (right) when the reward probability was switched from Prew = 0.5 to Prew = 0.9. The thick green line represents a binomial smoothing of the mean. (G) As in F, for the number of lever presses. (H) Raster plot showing the temporal distribution of lever presses in a 5 min long trial, in which the reward probability was switched from Prew = 0.5 to Prew = 0.9 every 30 s. The raster plots in this panel were collected at Prew = 0.5. Gold/light purple ticks represent the times when the lever was pressed. Brown/purple ticks represent the times when the water rewards were collected. (I) As in H, for Prew = 0.9. (J) Schematic representation of the test session, with water rewards delivered at two different values of Prew, switching at different time intervals (5–75 s). (K–N) As in B-E, for the test session. Data represent mean ± SEM.
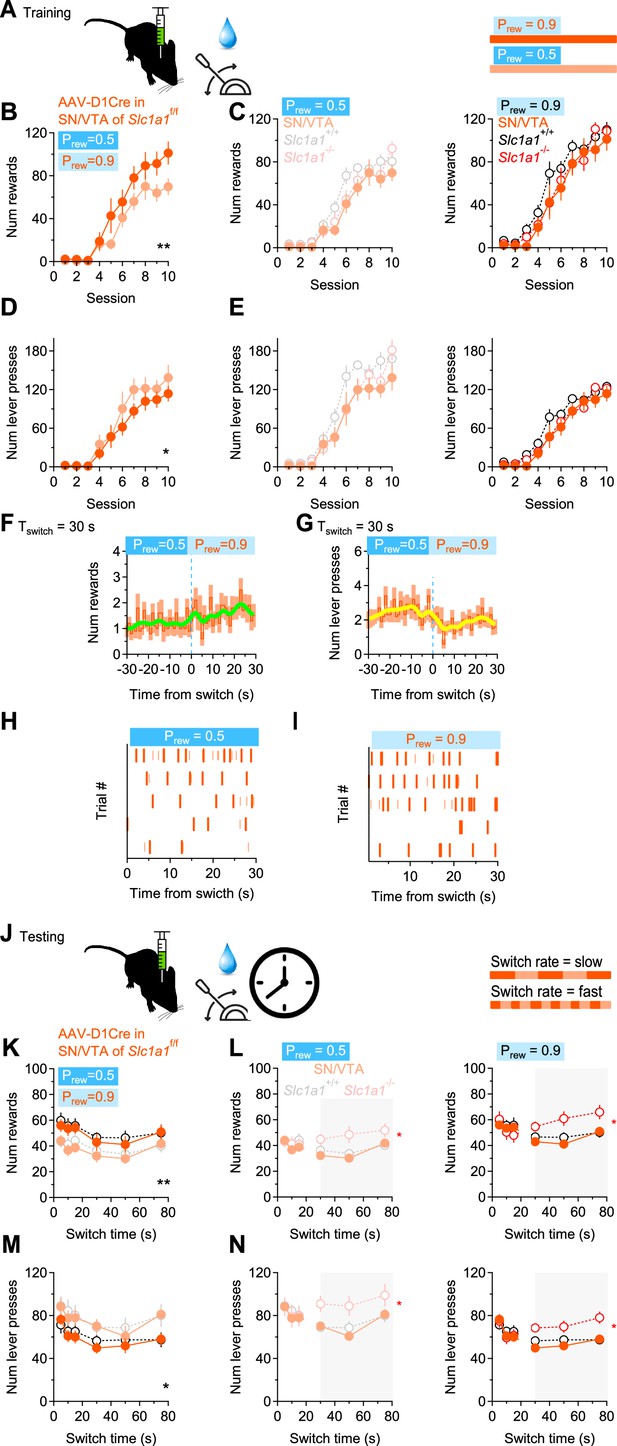
EAAC1 expression in the SN/VTA does not alter slow-switching behaviors.
(A) Schematic representation of the training sessions, with water rewards delivered at two different reward probabilities (Prew), during each 5 min session. (B) Number of rewards collected by Slc1a1f/f mice transfected with AAV-D1Cre in the SN/VTA (n=10 mice), at Prew = 0.5 and Prew = 0.9 over the course of 10 training sessions. (C) Comparison between Slc1a1f/f mice transfected with AAV-D1Cre in the SN/VTA and Slc1a1+/+ and Slc1a1-/- mice for the data shown in B. (D, E) As in B, C, showing the total number of lever presses performed by the mice. (F) Temporal distribution of the number of rewards collected by Slc1a1f/f mice transfected with AAV-D1Cre in the SN/VTA when the reward probability was switched from Prew = 0.5 to Prew = 0.9. The thick green line represents a binomial smoothing of the mean. (G) As in F, for the number of lever presses. (H) Raster plot showing the temporal distribution of lever presses in a 5 min long trial, in which the reward probability was switched from Prew = 0.5 to Prew = 0.9 every 30 s. The raster plots in this panel were collected at Prew = 0.5. Light orange ticks represent the times when the lever was pressed. Orange ticks represent the times when the water rewards were collected. (I) As in H, for Prew = 0.9. (J) Schematic representation of the test session, with water rewards delivered at two different values of Prew, switching at different time intervals (5–75 s). (K–N) As in B-E, for the test session. Data represent mean ± SEM.
Tables
Reagent type (species) or resource | Designation | Source or reference | Identifiers | Additional information |
---|---|---|---|---|
Strain, strain background (Mus musculus) | Slc1a1-/- | PMID:19923291 | ||
Strain, strain background (Mus musculus) | Slc1atm1c/tm1c | This paper | Here referred to as Slc1a1f/f | |
Strain, strain background (Mus musculus) | Gt(ROSA)26S or tm9(CAG-tdTomato)Hze | JAX | IMSR_JAX:007909 | Here referred to as Rosa26tm9/tm9 |
Strain, strain background (Mus musculus) | Drd1atdT/+ | JAX | IMSR_JAX:016204 | |
Strain, strain background (Mus musculus) | Drd1aCre/+ | MMRRC | MMRRC_030778-UCD | |
Strain, strain background (Mus musculus) | Adora2aCre/+ | MMRRC | MMRRC_036158-UCD | |
Transfected construct (M. musculus) | AAV2/5-EF1a-DIO-hChR2(H134R)-EYFP-WPRE-pA | University of North Carolina | Refers to viral construct used for stereotaxic injections | |
Transfected construct (M. musculus) | AAV2/9-D1-Cre-EGFP-WPRE-hGH-pA | Biohippo | Cat# PT-0812/BC-2111 | Refers to viral construct used for stereotaxic injections |
Transfected construct (M. musculus) | PHP.eB-S5E2-C1V1-eYFP | Addgene | Cat# 135633 | Refers to viral construct used for stereotaxic injections |
Other | Mm-Drd1-C1 | Advanced Cell Diagnostics | RNAscope FISH probe | |
Other | Mm-Slc1a1-C2 | Advanced Cell Diagnostics | RNAscope FISH probe | |
Other | Mm-Drd2-C3 | Advanced Cell Diagnostics | RNAscope FISH probe | |
Other | DAPI stain | Southern Biotech | Cat# 0100–02 | Immunohistochemistry |
Other | Streptavidin-Alexa Fluor 488 | Jackson Immuno Research | Cat# 016-540-084 | 1:1,000 |
Other | Streptavidin-Alexa Fluor 647 | Jackson Immuno Research | Cat# 016-600-084 | 1:1,000 |
Software, algorithm | SPSS v28 | SPSS | ||
Software, algorithm | IgorPro v6.37 | IgorPro | ||
Software, algorithm | RStudio 2023.03.1+446 | RStudio |
Sequence of genotyping primers.
Gene | Forward primer | Reverse primer | Band size (bp) |
---|---|---|---|
Slc1a1+/+ | 5’ ACT CAT CGC AAA CGT CAG TG 3’ | 5’ GAG AGC AGC AGC CAG TGA TTC 3’ | 94 |
Slc1a1-/- | 5’ CTG TGC TCG ACG TTG TCA CTG 3’ | 5’ GAG AGC AGC AGC CAG TGA TTC 3’ | 680 |
Slc1a1+/+ | 5’ TAC CCC AGT GAC TCA TCA GC 3’ | 5’ CAT GGT GTT TAC CAG CGT GA 3’ | 269 |
Slc1a1f/f | 5’ TAC CCC AGT GAC TCA TCA GC 3’ | 5’ CAT GGT GTT TAC CAG CGT GA 3’ | 384 |
Drd1aCre/+ | 5’ GCT ATG GAG ATG CTC CTG ATG GAA 3’ | 5’ CGG CAA ACG GAC AGA AGC ATT 3’ | 340 |
Adora2aCre/+ | 5’ CGT GAG AAA GCC TTT GGG AAG CT 3’ | 5’ CGG CAA ACG GAC AGA AGC ATT 3’ | 350 |
Rosa26-/- | 5’ AAG GGA GCT GCA GTG GAG TA 3’ | 5’ CCG AAA ATC TGT GGG AAG TC 3’ | 297 |
Rosa26tm9/- | 5’ CTG TTC CTG TAC GGC ATG G 3’ | 5’ GGC ATT AAA GCA GCG TAT CC 3’ | 196 |
Drd1atdT/+ | 5’ CTT CTG AGG CGG AAA GAA CC 3’ | 5’ TTT CTG ATT GAG AGC ATT CG 3’ | 750 |
PCR protocol for all mice.
Initiation/melting | Denaturation | Annealing | Elongation | Amplification | Hold | |
---|---|---|---|---|---|---|
Temperature | 95 °C | 95 °C | 60 °C | 72 °C | 72 °C | 4 °C |
Duration | 3 min | 0.25 min | 0.25 min | 0.25 min | 1 min | ∞ |
Cycles | 1 | 35 | 1 |