Photosynthesis: Surviving on low-energy light comes at a price
Life on Earth as we know it depends on photosynthesis. In this process, plants, algae and cyanobacteria use solar energy to convert water and carbon dioxide into the oxygen we breathe and the glucose that fuels many biological processes (Blankenship, 2002).
The photosynthetic machinery is made from an intricate and complicated collection of protein complexes containing mainly chlorophyll a molecules and carotenoid molecules. Several of these complexes work together in a synchronised fashion to accomplish an amazing feat: to split water molecules (one of the most stable molecules on earth) to extract electrons and produce oxygen, and to transfer electrons to quinone molecules so that photosynthesis can continue. The efficiency of this initial energy-conversion step determines the outcome of the whole process.
For many years it was thought that the red light absorbed by chlorophyll a (which has a wavelength of 680 nanometres) was the lowest-energy light that could be used to drive oxygenic photosynthesis while minimising the damage caused by high levels of light (Rutherford et al., 2012). However, previous research has shown that some cyanobacteria living in shaded environments can thrive in far-red light, which is close to the limit of what we can see: this light is lower in energy than red light because its wavelength is longer (720 nanometres) (Figure 1). These cyanobacteria contain chlorophyll d and chlorophyll f in addition to chlorophyll a, but they can still perform the same reactions as organisms that contain only chlorophyll a (Miyashita et al., 1996; Renger and Schlodder, 2008; Gan et al., 2014; Nürnberg et al., 2018; Davis et al., 2016). So far, however, it has not been clear if these species pay a price in terms of resilience to photodamage or energy conversion efficiency.
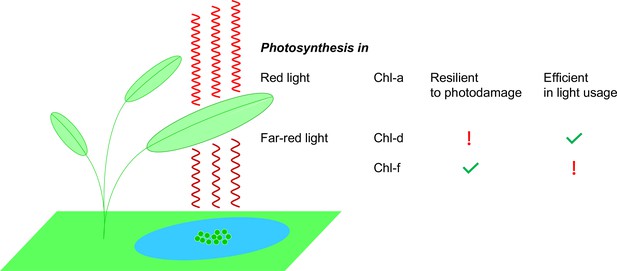
Photosynthesis in red light and far-red light.
Plants, algae and cyanobacteria use a molecule called chlorophyll a (Chl-a) to absorb red light to power the process of photosynthesis. Studies have shown that Chl-a is resilient to photodamage and is efficient in using light energy. Some cyanobacteria (green circles in the blue pond; not to scale) have adapted to their darker environments by using different chlorophyll molecules – chlorophyll d (Chl-d) and chlorophyll f (Chl-f) – to absorb far-red light (which is less energetic than red light). However, the use of these molecules comes at a price: Chl-d organisms are energy efficient but they are not resilient to photodamage; Chl-f organisms, on the other hand, are not energy efficient but they are resilient to photodamage.
Now, in eLife, Stefania Viola and A William Rutherford of Imperial College London and colleagues – who are based at Imperial, the CNR in Milan, the Free University of Berlin, Sorbonne University and CEA-Saclay – report new insights into far-red photosynthesis (Viola et al., 2022). The team studied two species of cyanobacteria that use far-red photosynthesis: Acaryochloris marina, which lives in shaded environments, and Chroococcidiopsis thermalis, which lives in variable light conditions and can switch between standard photosynthesis and far-red photosynthesis depending on the light energy. To assess whether these organisms have the same resilience to photodamage and energy conversion efficiency as organisms that contain only chlorophyll a, Viola et al. investigated many aspects of the first initial energy-conversion step in photosynthesis (as described above). This included measuring the amount of oxygen generated, as well as the quantity of reactive oxygen species produced, which can lead to photodamage.
This revealed that both species of cyanobacteria produced comparable amounts of oxygen to chlorophyll a-only cyanobacteria. The photosystem II of A. marina, which contains 34 chlorophyll d molecules and just one chlorophyll a molecule, was highly efficient, but also produced high levels of reactive oxygen species when exposed to high-light levels, making it less resilient to photodamage. In contrast, when C. thermalis – which contains four chlorophyll f, one chlorophyll d and 30 chlorophyll a molecules – was grown in far-red light it produced fewer reactive oxygen species, but was also less energy efficient.
Viola et al. present a detailed picture of how these organisms have adapted to the low-light conditions specific to their environments and the associated costs of their traits. A better understanding of how photosynthetic organisms make use of low-energy light could help scientists to engineer far-red photosystems into algae or plants containing only chlorophyll a, thereby enhancing their use of sunlight and improving crop yields (Chen and Blankenship, 2011). Moreover, in the future, artificial systems could be designed to use low-energy light to generate solar fuels.
References
-
BookMolecular Mechanisms of PhotosynthesisOxford, UK: Blackwell Science Ltd.https://doi.org/10.1002/9780470758472
-
Expanding the solar spectrum used by photosynthesisTrends in Plant Science 16:427–431.https://doi.org/10.1016/j.tplants.2011.03.011
Article and author information
Author details
Publication history
Copyright
© 2022, Romero
This article is distributed under the terms of the Creative Commons Attribution License, which permits unrestricted use and redistribution provided that the original author and source are credited.
Metrics
-
- 2,685
- views
-
- 179
- downloads
-
- 1
- citations
Views, downloads and citations are aggregated across all versions of this paper published by eLife.
Download links
Downloads (link to download the article as PDF)
Open citations (links to open the citations from this article in various online reference manager services)
Cite this article (links to download the citations from this article in formats compatible with various reference manager tools)
Further reading
-
- Structural Biology and Molecular Biophysics
In addition to its role as cellular energy currency, adenosine triphosphate (ATP) serves as an extracellular messenger that mediates diverse cell-to-cell communication. Compelling evidence supports that ATP is released from cells through pannexins, a family of membrane proteins that form heptameric large-pore channels. However, the activation mechanisms that trigger ATP release by pannexins remain poorly understood. Here, we discover lysophospholipids as endogenous pannexin activators, using activity-guided fractionation of mouse tissue extracts combined with untargeted metabolomics and electrophysiology. We show that lysophospholipids directly and reversibly activate pannexins in the absence of other proteins. Secretomics experiments reveal that lysophospholipid-activated pannexin 1 leads to the release of not only ATP but also other signaling metabolites, such as 5’-methylthioadenosine, which is important for immunomodulation. We also demonstrate that lysophospholipids activate endogenous pannexin 1 in human monocytes, leading to the release of IL-1β through inflammasome activation. Our results provide a connection between lipid metabolism and purinergic signaling, both of which play major roles in immune responses.
-
- Structural Biology and Molecular Biophysics
Integral membrane proteins carry out essential functions in the cell, and their activities are often modulated by specific protein-lipid interactions in the membrane. Here, we elucidate the intricate role of cardiolipin (CDL), a regulatory lipid, as a stabilizer of membrane proteins and their complexes. Using the in silico-designed model protein TMHC4_R (ROCKET) as a scaffold, we employ a combination of molecular dynamics simulations and native mass spectrometry to explore the protein features that facilitate preferential lipid interactions and mediate stabilization. We find that the spatial arrangement of positively charged residues as well as local conformational flexibility are factors that distinguish stabilizing from non-stabilizing CDL interactions. However, we also find that even in this controlled, artificial system, a clear-cut distinction between binding and stabilization is difficult to attain, revealing that overlapping lipid contacts can partially compensate for the effects of binding site mutations. Extending our insights to naturally occurring proteins, we identify a stabilizing CDL site within the E. coli rhomboid intramembrane protease GlpG and uncover its regulatory influence on enzyme substrate preference. In this work, we establish a framework for engineering functional lipid interactions, paving the way for the design of proteins with membrane-specific properties or functions.