MYC overrides HIF-1α to regulate proliferating primary cell metabolism in hypoxia
Figures
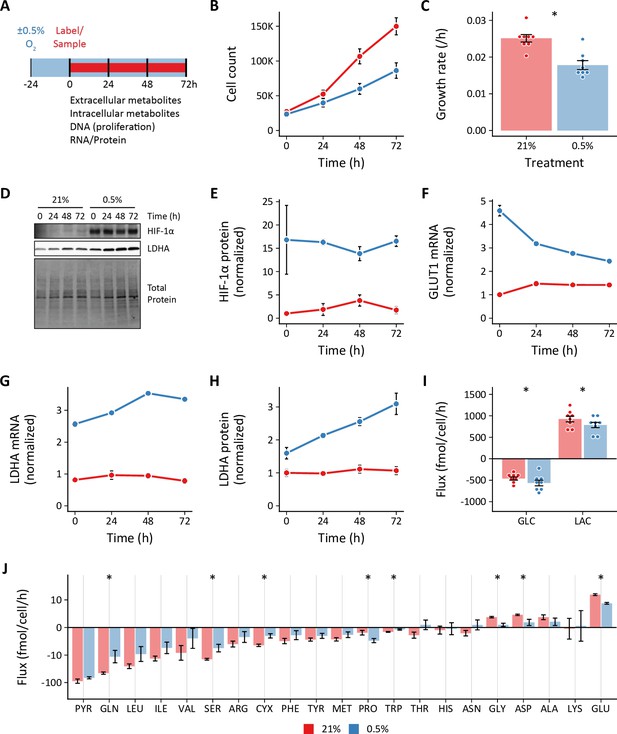
Effects of 0.5% oxygen on extracellular metabolite fluxes in lung fibroblasts.
(A) Lung fibroblasts (LFs) were cultured in 21% or 0.5% oxygen beginning 24 hr prior to time 0. Samples were collected every 24 hr for 72 hr. (B) Growth curves of LFs in each experimental condition (n=8). (C) Growth rates from (B) were determined by robust linear modeling of log-transformed growth curves. (D) Representative immunoblot of LF protein lysates cultured as in (A). (E) Relative change in HIF-1α protein levels from (D) normalized to 21% oxygen at time 0 (n=4). (F) Relative change in GLUT1 mRNA levels normalized to 21% oxygen treatment at time 0 (n=4). (G) Relative change in LDHA mRNA levels as in (F). (H) Relative change in LDHA protein levels as in (E). (I) Extracellular fluxes of glucose (GLC) and lactate (LAC) (n=8). By convention, negative fluxes indicate metabolite consumption. (J) Extracellular fluxes of pyruvate (PYR) and amino acids. Data are mean ± SEM (* p<0.05).
-
Figure 1—source data 1
Uncropped blot images for Figure 1.
- https://cdn.elifesciences.org/articles/82597/elife-82597-fig1-data1-v1.zip
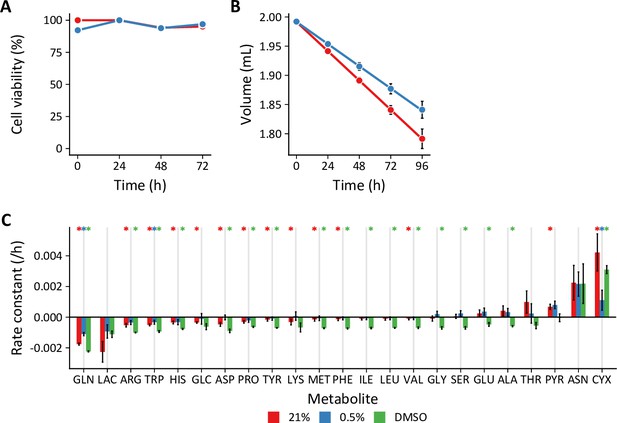
Supporting data for extracellular flux calculations.
(A) Cell viability as assessed by live/dead cell staining with acridine orange plus propidium iodide did not differ between 21% and 0.5% oxygen culture conditions (n=3 technical replicates). (B) Predicted well volumes were estimated from the change in culture plate mass over the experimental time course. Evaporation rates differed depending on the culture conditions and treatment. Although the mean evaporation rate is depicted, experiment-specific evaporation rates were used to calculate fluxes for each biological replicate (C) Metabolite accumulation (positive values) and degradation (negative values) rates. Data are mean ± SEM of three to eight biological replicates. Rates significantly different from 0 (*) based on a probability value <0.05 using Student’s one-sample t-test were incorporated into flux calculations.
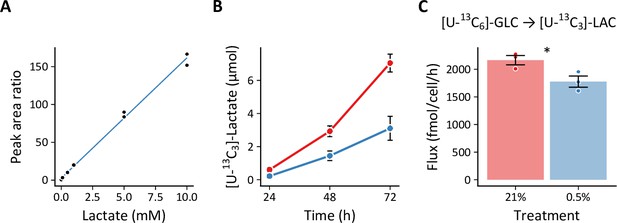
Quantifying lactate efflux generated by [U-13C6]-glucose.
Cells were cultured in MCDB131 supplemented with 8 mM [U-13C6]-glucose. Conditioned medium was collected, spiked with [D8]-ᴅʟ-valine internal standard, and analyzed by LC-MS. (A) A standard curve was generated from the peak area ratios of lactate and the internal standard prepared in unconditioned MCDB131 medium. (B) Lactate accumulates more slowly in the conditioned medium from hypoxic cells (n=3 biological replicates). (C) Lactate efflux was decreased in hypoxia, similar to the data obtained from enzymatic lactate assay. Data are mean ± SEM (* p<0.05).
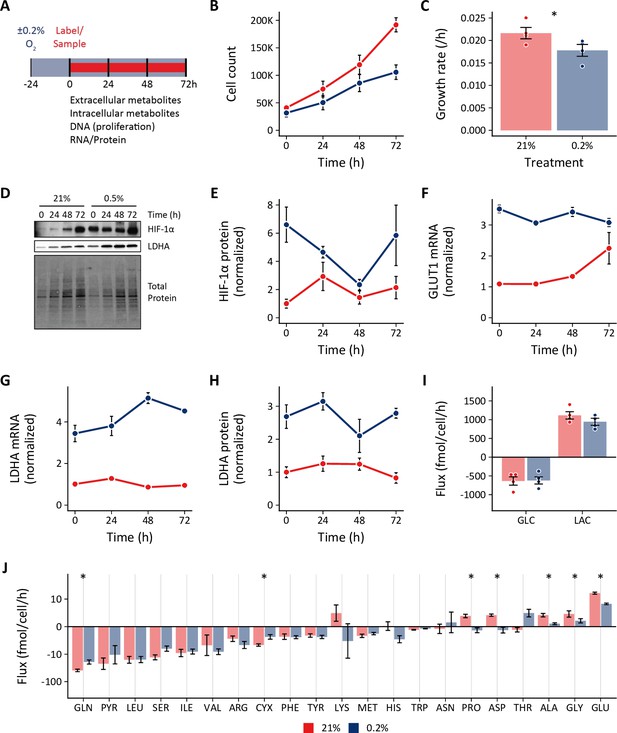
Effects of 0.2% oxygen on extracellular metabolite fluxes in lung fibroblasts.
(A) Lung fibroblasts (LFs) were cultured in 21% or 0.2% oxygen beginning 24 hr prior to time 0. Samples were collected every 24 hr for 72 hr. (B) Growth curves of LFs in each experimental condition (n=4). (C) Growth rates from (B) were determined by robust linear modeling of log-transformed growth curves. (D) Representative immunoblot of LF protein lysates cultured as in (A). (E) Relative change in HIF-1α protein levels from (D) normalized to 21% oxygen at time 0 (n=4). (F) Relative change in GLUT1 mRNA levels normalized to 21% oxygen treatment at time 0 (n=4). (G) Relative change in LDHA mRNA levels as in (F). (H) Relative change in LDHA protein levels as in (E). (I) Extracellular fluxes of glucose (GLC) and lactate (LAC) (n=4). By convention, negative fluxes indicate metabolite consumption. (J) Extracellular fluxes of pyruvate (PYR) and amino acids. Data are mean ± SEM (* p<0.05).
-
Figure 1—figure supplement 3—source data 1
Uncropped blot images for Figure 1—figure supplement 3.
- https://cdn.elifesciences.org/articles/82597/elife-82597-fig1-figsupp3-data1-v1.zip
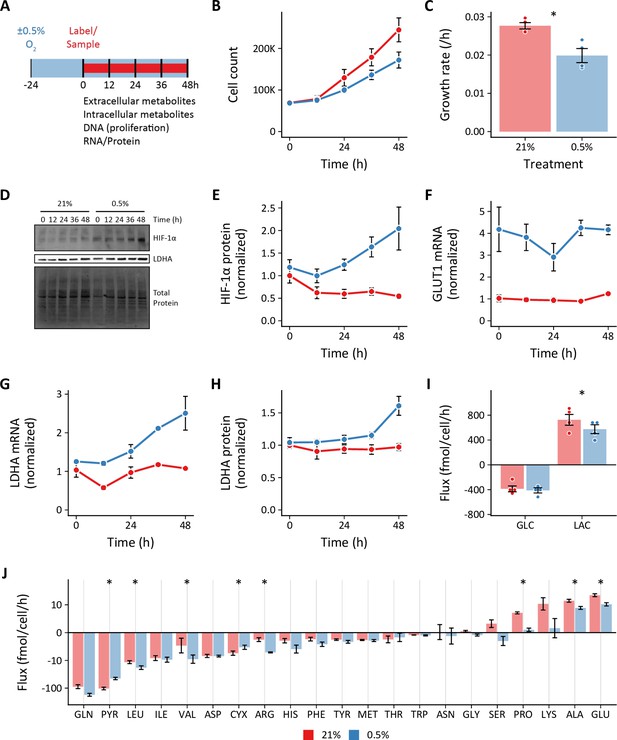
Effects of 0.5% oxygen on extracellular metabolite fluxes in pulmonary artery smooth muscle cells.
(A) Pulmonary artery smooth muscle cells (PASMCs) were cultured in 21% or 0.5% oxygen beginning 24 hr prior to time 0. Samples were collected every 24 hr for 72 hr. (B) Growth curves of LFs in each experimental condition (n=4). (C) Growth rates from (B) were determined by robust linear modeling of log-transformed growth curves. (D) Representative immunoblot of LF protein lysates cultured as in (A). (E) Relative change in HIF-1α protein levels from (D) normalized to 21% oxygen at time 0 (n=4). (F) Relative change in GLUT1 mRNA levels normalized to 21% oxygen treatment at time 0 (n=4). (G) Relative change in LDHA mRNA levels as in (F). (H) Relative change in LDHA protein levels as in (E). (I) Extracellular fluxes of glucose (GLC) and lactate (LAC) (n=4). By convention, negative fluxes indicate metabolite consumption. (J) Extracellular fluxes of pyruvate (PYR) and amino acids. Data are mean ± SEM (* p<0.05).
-
Figure 1—figure supplement 4—source data 1
Uncropped blot images for Figure 1—figure supplement 4.
- https://cdn.elifesciences.org/articles/82597/elife-82597-fig1-figsupp4-data1-v1.zip
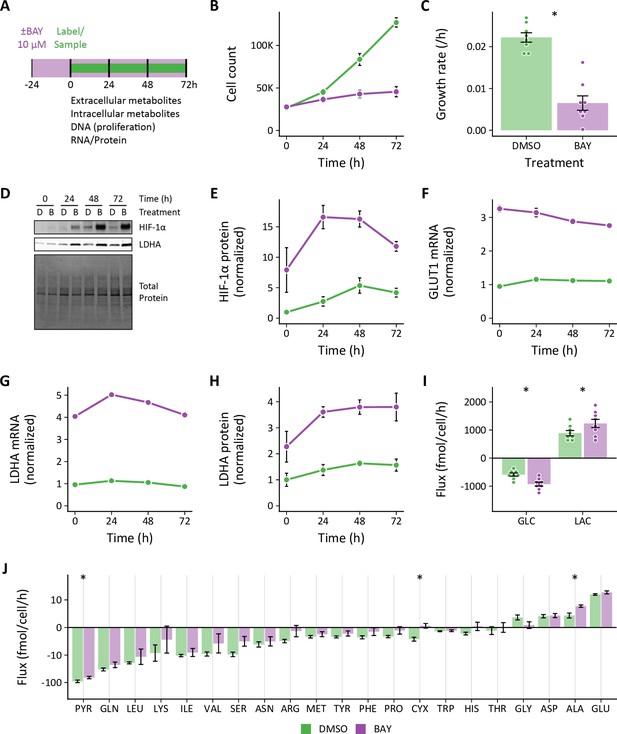
Effects of pharmacologic prolyl hydroxylase inhibition on extracellular metabolite fluxes in lung fibroblasts.
(A) Lung fibroblasts (LFs) were treated with the prolyl hydroxlyase inhibitor molidustat (BAY, 10 μM) or DMSO beginning 24 hr prior to time 0. Samples were collected every 24 hr for 72 hr. (B) Growth curves of LFs in each experimental condition (n=8). (C) Growth rates from (B). (D) Representative immunoblot of LF protein lysates cultured as in (A). (E) Relative change in HIF-1α protein levels from (D) normalized to DMSO at time 0 (n=4). (F) Relative change in GLUT1 mRNA levels normalized to DMSO at time 0 (n=4). (G) Relative change in LDHA mRNA levels as in (F). (H) Relative change in LDHA protein levels as in (E). (I) Extracellular fluxes of glucose (GLC) and lactate (LAC) (n=8). By convention, negative fluxes indicate metabolite consumption. (J) Extracellular fluxes of pyruvate (PYR) and amino acids. Data are mean ± SEM (* p<0.05).
-
Figure 2—source data 1
Uncropped blot images for Figure 2.
- https://cdn.elifesciences.org/articles/82597/elife-82597-fig2-data1-v1.zip
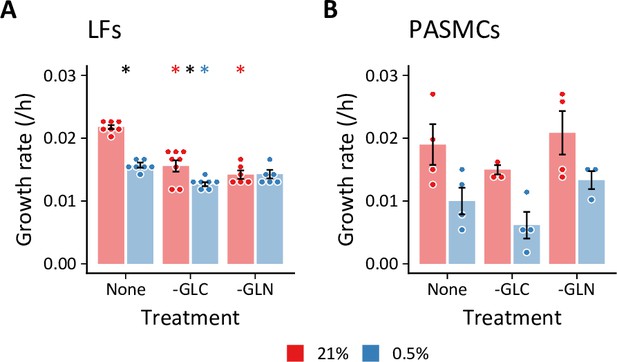
Cell growth rates following substrate deprivation.
(A, B) Lung fibroblasts (n=8 biological replicates) (A) and pulmonary artery smooth muscle cells (n=4 biological replicates) (B) were cultured in MCDB131 medium lacking either glucose (-GLC) or glutamine (-GLN) for 72 hr. Growth rates were calculated from total DNA quantification. Data are mean ± SEM (* p<0.05; black compares 21% and 0.5% oxygen within a given treatment, red and blue compare substrate deficiency to replete medium in 21% and 0.5% oxygen, respectively.).

Stable isotope labeling of lung fibroblasts following hypoxic and pharmacologic PHD inhibition.
(A) Mass isotopomer distribution (MID) of pyruvate (PYR) following 72 hr labeling with [U-13C6]-glucose (GLC). (B) MID of citrate after 72 hr labeling with [U-13C6]-GLC (C) MID of citrate after 72 hr labeling with [U-13C5]-glutamine (GLN). Data are mean ± SEM (n=4, p<0.05 indicated as * 0.5% v. 21% oxygen, † BAY v. DMSO, ‡ Δoxygen v. ΔBAY). (D) Fraction of M5 citrate indicating reductive carboxylation after labeling with [U-13C5]-GLN in LFs and PASMCs (n=3–4, * p<0.05).
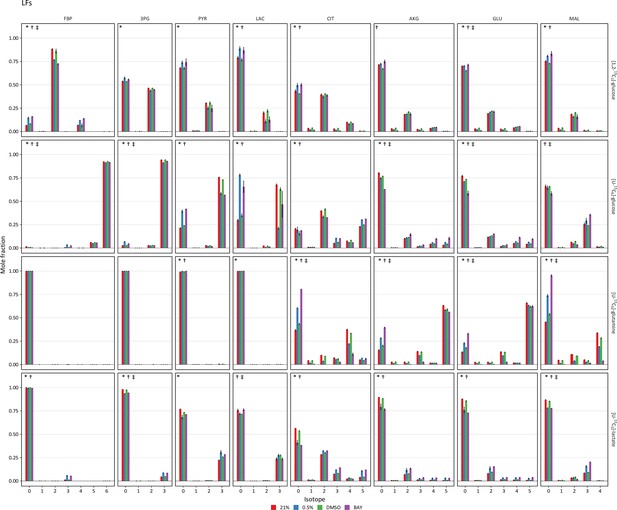
Mass isotopomer distributions after 72 hr of labeling in lung fibroblasts.
Lung fibroblasts (LFs) were labeled with the indicated tracers and intracellular metabolites were analyzed by LC-MS after 72 hr. Mass isotopomer distributions were adjusted for natural abundance. Data are the mean ± SEM of four biological replicates. Significant differences in labeling patterns between 21% and 0.5% oxygen (*), DMSO and BAY treatment (†), and 0.5% oxygen and BAY treatment (‡) for each combination of metabolite and tracer are highlighted.
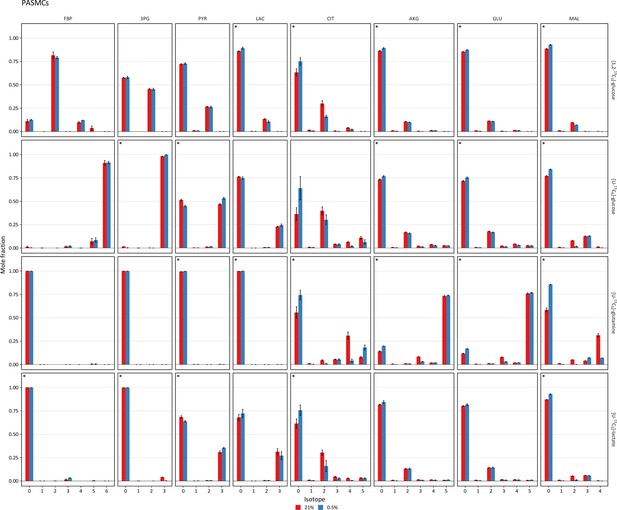
Mass isotopomer distributions after 36 hr of labeling in pulmonary artery smooth muscle cells.
Pulmonary artery smooth muscle cells (PASMCs) were labeled with the indicated tracers and intracellular metabolites were analyzed by LC-MS after 36 hr. Mass isotopomer distributions were adjusted for natural abundance. Data are the mean ± SEM of four biological replicates. Significant differences in labeling patterns between 21% and 0.5% oxygen (*) for each combination of metabolite and tracer are highlighted.

Metabolic flux analysis of lung fibroblasts following hypoxic and pharmacologic PHD inhibition.
(A) Ratio of modeled metabolic fluxes in 0.5% oxygen compared to 21% oxygen. Fluxes with non-overlapping confidence intervals are highlighted with arrows colored according to the magnitude of the fold change. Arrow thickness corresponds to the absolute flux measured in hypoxia. (B) Ratio of metabolic fluxes in BAY-treated cells compared to DMSO-treated control. Arrows are colored as in (A) and arrow weights correspond to the absolute flux as measured in BAY-treated cells.
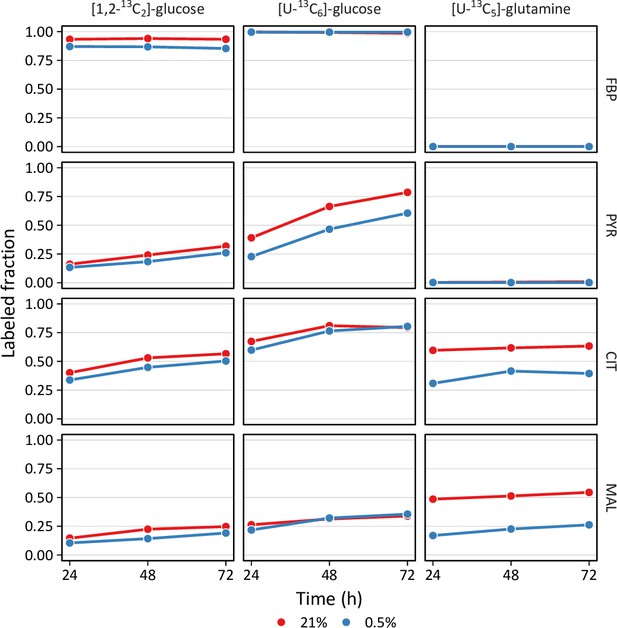
Isotope incorporation over the labeling time course.
LFs were cultured in 21% or 0.5% oxygen and labeled with the indicated tracers. Intracellular metabolites were analyzed by LC-MS (FBP, fructose-bisphosphate; PYR, pyruvate; CIT, citrate; MAL, malate). Mass isotopomer distributions were calculated and adjusted for natural abundance. Data show the total amount of metabolite labeling (i.e. 1 M0 fractional abundance). Data are the mean ± SEM of four biological replicates.
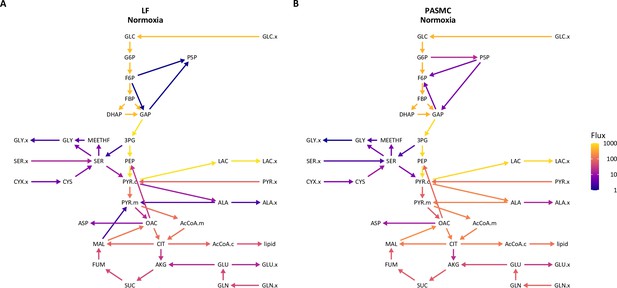
Isotopically non-stationary metabolic flux analysis of cell metabolism in 21% oxygen.
(A) Metabolic flux model of LF metabolism in 21% oxygen. Arrows are colored by log10(flux). (B) Metabolic flux model of PASMC metabolism in 21% oxygen as in (A).
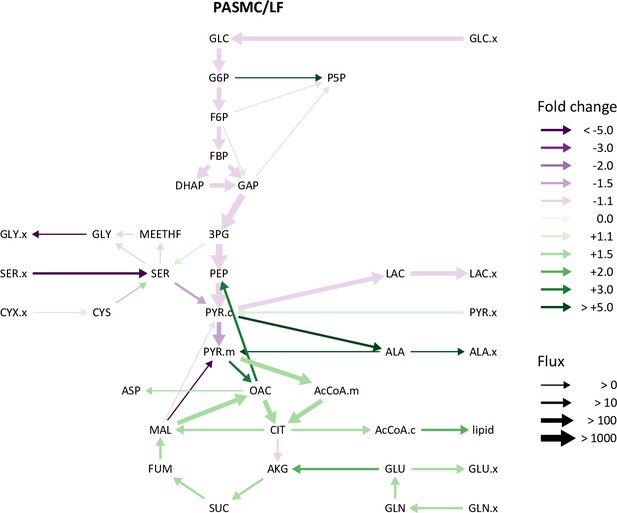
Comparison of lung fibroblast and pulmonary artery smooth muscle cell metabolic fluxes.
Ratio of metabolic fluxes in PASMCs compared to LFs. Fluxes with non-overlapping confidence intervals are highlighted with arrows colored according to the magnitude of the change. Arrow thickness corresponds to the absolute flux measured in LFs.
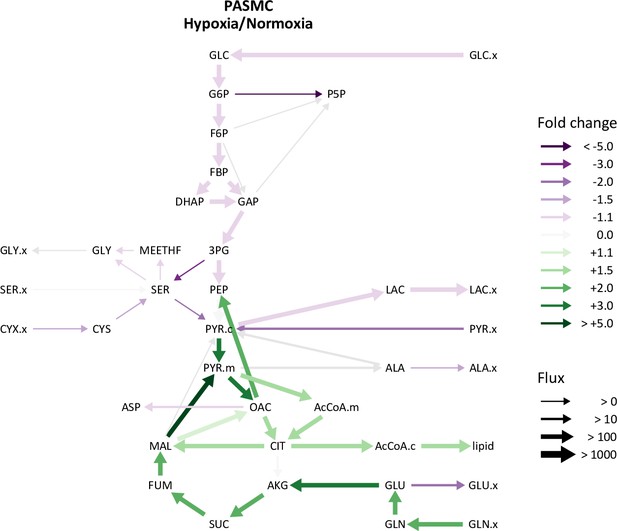
Comparison of pulmonary artery smooth muscle cell metabolic fluxes in 21% and 0.5% oxygen.
Ratio of modeled metabolic fluxes in 0.5% oxygen compared to 21% oxygen. Fluxes with non-overlapping confidence intervals are highlighted with arrows colored according to the magnitude of the fold change. Arrow thickness corresponds to the absolute flux measured in hypoxia.
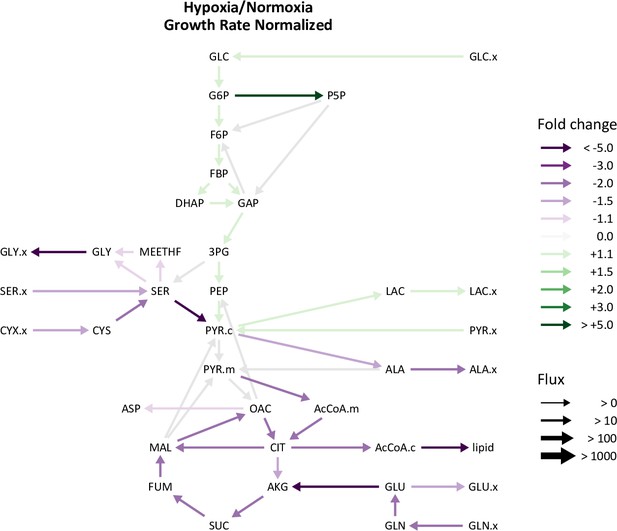
Growth rate adjusted changes in hypoxic lung fibroblast metabolism.
LF fluxes were normalized to cell growth rate. Graph depicts the ratio of normalized metabolic fluxes in LFs cultured in 0.5% oxygen compared to 21% oxygen control. Fluxes with non-overlapping confidence intervals are highlighted to indicate significant changes.
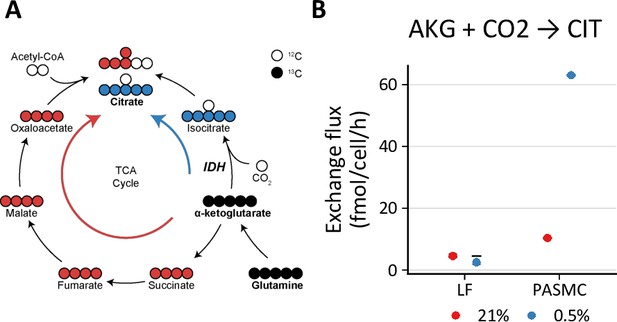
Hypoxia increases reductive carboxylation in pulmonary artery smooth muscle cells.
(A) Reductive carboxylation describes the converstion of α-ketoglutarate (AKG) to isocitrate by reverse flux through isocitrate dehydrogenase (IDH) (blue arrow). This yields M+5 citrate. (B) Exchange flux estimates for reductive carboxylation. Data show the model estimate with upper and lower bounds for LFs and PASMCs.
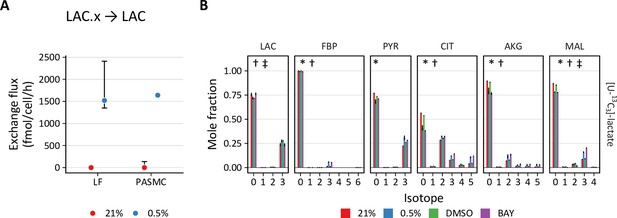
PHD inhibition increases lactate uptake and oxidation.
(A) Exchange flux estimates for lactate import presented as in (A). (C) Mass isotopomer distributions of lactate (LAC), fructose-bisphosphate (FBP), pyruvate (PYR), citrate (CIT), α-ketoglutarate (AKG), and malate (MAL) in LFs following 72 hr labeling with [U-13C3] lactate. Data are mean ± SEM (n=4, p<0.05 for the following comparisons: * 0.5% v. 21% oxygen, † BAY v. DMSO, ‡ Δoxygen v. ΔBAY).
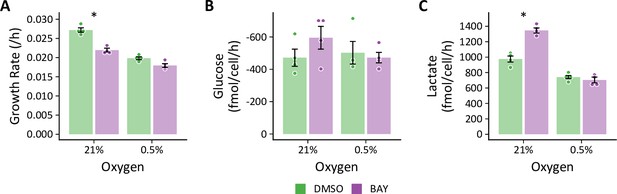
Hypoxia prevents BAY-stimulated increases in glycolysis.
(A) LFs were cultured in standard growth medium and treated with molidustat (BAY, 10 μM) or vehicle (DMSO, 0.1%) in 21% or 0.5% oxygen conditions for 72 hr. Growth rates were determined by linear fitting of log-transformed growth curves. (B) Glucose uptake in LFs treated with BAY cultured in hypoxia (note reversed y-axis). (C) Lactate efflux in LFs treated with BAY cultured in hypoxia. Data are mean ± SEM (n=4, * p<0.05 BAY v. DMSO within a given oxygen exposure).
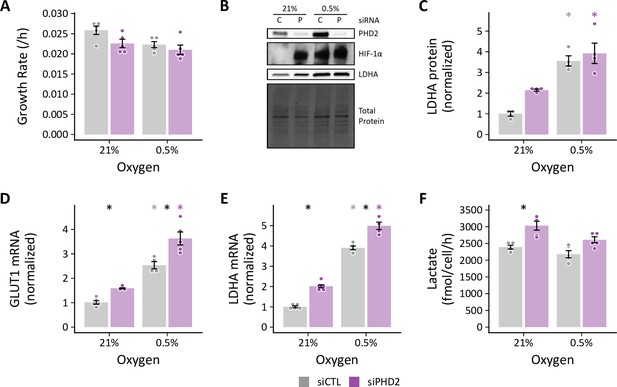
Hypoxia attenuates siPHD2-stimulated increases in glycolysis.
LFs were treated with siRNA to knock down PHD2 expression. (A) Cell growth was not impacted by siPHD2 treatment. (B) Representative immunoblot of LF cell lysates at 72 hr demonstrating substantial PHD2 knockdown associated with stabilization of HIF-1α. (C) Relative change in LDHA protein levels from (B) normalized to 21% siCTL (n=4). (D) GLUT1 and (E) LDHA mRNA determined by RT-qPCR (n=5). (F) Lactate efflux in siPHD2-treated cells (n=5). Data are mean ± SEM (p<0.05 with black * indicating a significant effect of treatment within a given oxygen tension and colored * indicating a significant effect of oxygen within a given treatment denoted by the color).
-
Figure 8—figure supplement 1—source data 1
Uncropped blot images for Figure 8—figure supplement 1.
- https://cdn.elifesciences.org/articles/82597/elife-82597-fig8-figsupp1-data1-v1.zip
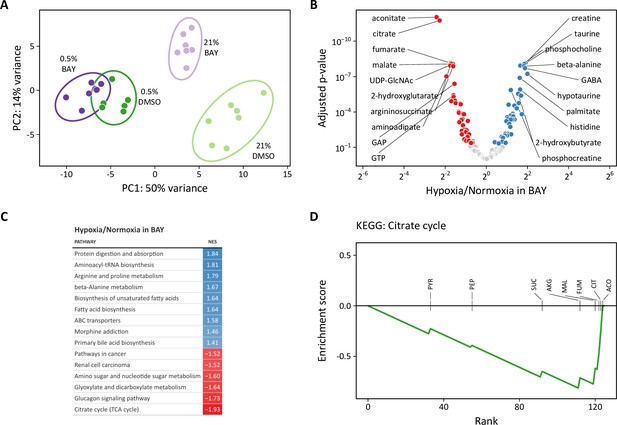
Metabolomic analysis of molidustat treatment in normoxia and hypoxia.
(A) Principal component analysis of intracellular metabolites following 72 hr of treatment suggests a dominant effect of hypoxia over pharmacologic PHD inhibition on the metabolome (n=7). (B) Volcano plot of intracellular metabolites of BAY-treated cells cultured in 21% or 0.5% oxygen. Filled circles indicate significantly increased (blue) and decreased (red) levels in hypoxia. (C) Metabolite set enrichment analysis of metabolites from (B). All KEGG pathways with p-values <0.05 are shown. (D) Leading edge analysis of the TCA cycle metabolite set. Negative values indicate the relative enrichment associated with BAY treatment alone compared to BAY plus hypoxia treatment. Abbreviations: PYR, pyruvate; SUC, succinate; PEP, phosphoenolpyruvate; CIT, citrate; AKG, α-ketoglutarate; MAL, malate; ACO, aconitate; FUM, fumarate.
-
Figure 9—source data 1
Source data for Figure 9C.
- https://cdn.elifesciences.org/articles/82597/elife-82597-fig9-data1-v1.csv
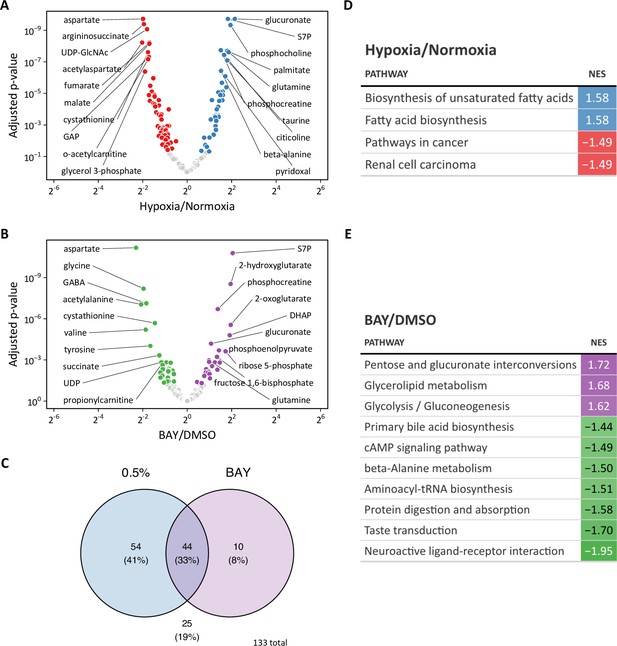
Metabolomic profiling of hypoxia and BAY treated lung fibroblasts.
(A) Volcano plot of differentially regulated metabolites following 0.5% oxygen culture. (B) Volcano plot of differentially regulated metabolites by BAY treatment in 21% oxygen. (C) Venn diagram illustrating the overlap among metabolites differentially regulated by hypoxia (blue) or BAY treatment (purple). (D) Metabolite enrichment in KEGG pathways following hypoxia. (E) Metabolite enrichment in KEGG pathways following BAY. Only significantly enriched metabolite sets with p<0.05 are shown. NES, normalized enrichment score.
-
Figure 9—figure supplement 1—source data 1
Source data for Figure 9—figure supplement 1D.
- https://cdn.elifesciences.org/articles/82597/elife-82597-fig9-figsupp1-data1-v1.csv
-
Figure 9—figure supplement 1—source data 2
Source data for Figure 9—figure supplement 1E.
- https://cdn.elifesciences.org/articles/82597/elife-82597-fig9-figsupp1-data2-v1.csv
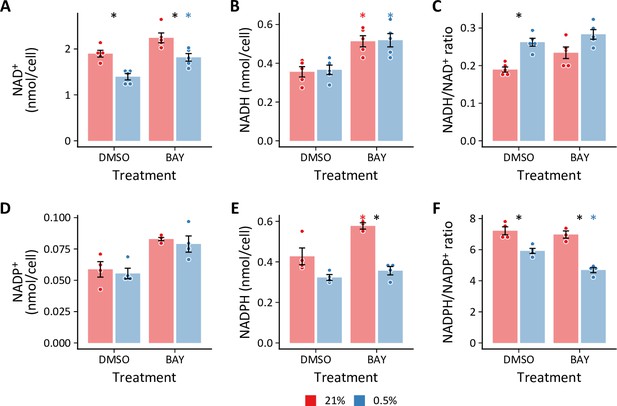
Changes in NAD(H) and NADP(H) following hypoxia and BAY treamtent.
(A) NAD+, (B) NADH, and (C) NADH/NAD+ from LFs cultured for 72 hr in 0.5% oxygen ±BAY (n=5).(D) NADP+, (E) NADPH, and (F) NADPH/NADP+ from LFs cultured for 72 hr in 0.5% oxygen ±BAY (n=4). Data are mean ± SEM (p<0.05 with black * indicating a significant effect of oxygen within a given treatment and colored * indicating a significant effect of treatment within a given oxygen tension as denoted by the color).

Transcriptomic analysis of molidustat treatment in normoxia and hypoxia.
(A) Principal component analysis of transcriptional changes in lung fibroblasts following 72 hr of treatment with 0.5% oxygen or molidustat (BAY), separately or together (n=4). (B) Volcano plot illustrating the effects of hypoxia in BAY-treated cells on gene expression. (C) Gene set enrichment analysis of transcripts from (B). (D) Volcano plot of those transcripts comprising the E2F Targets and G2/M Checkpoint Hallmark gene sets. (E) Volcano plot of those transcripts comprising the MYC Targets V1 and V2 Hallmark gene sets. (F) Volcano plot illustrating the results of a transcription factor enrichment analysis suggests mechanisms for differential regulation of gene expression following hypoxia or BAY treatment.
-
Figure 10—source data 1
Source data for Figure 10C.
- https://cdn.elifesciences.org/articles/82597/elife-82597-fig10-data1-v1.csv
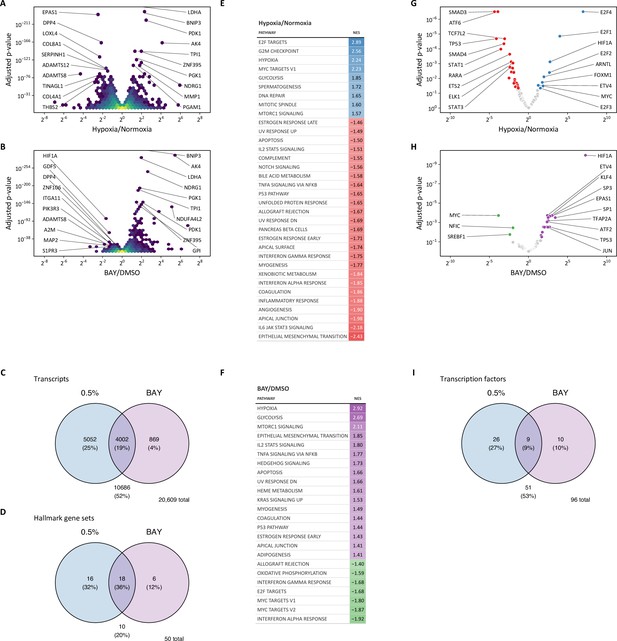
Transcriptomic profiling of hypoxia and BAY-treated lung fibroblasts.
(A) Volcano plot of differentially expressed genes following 0.5% oxygen culture. (B) Volcano plot of differentially expressed genes following BAY treatment. (C) Venn diagram illustrating the number of differentially expressed transcripts following hypoxia (blue) or BAY treatment (purple). (D) Venn diagram illustrating the number of differentially enriched Hallmark gene sets with hypoxia (blue) or BAY treatment (purple). (E) Gene set enrichment results of hypoxia-treated cells. (F) Gene set enrichment results of BAY-treated cells. All gene sets listed were significantly enriched at FDR <0.05. NES, normalized enrichment score. (G) Volcano plot illustrating the results of a transcription factor enrichment analysis in hypoxia-treated cells. (H) Volcano plot illustrating the results of a transcription factor enrichment analysis in BAY-treated cells. (I) Venn diagram illustrating the overlap among enriched transcription factors following hypoxia or BAY treatment.
-
Figure 10—figure supplement 1—source data 1
Source data for Figure 10—figure supplement 1E.
- https://cdn.elifesciences.org/articles/82597/elife-82597-fig10-figsupp1-data1-v1.csv
-
Figure 10—figure supplement 1—source data 2
Source data for Figure 10—figure supplement 1F.
- https://cdn.elifesciences.org/articles/82597/elife-82597-fig10-figsupp1-data2-v1.csv
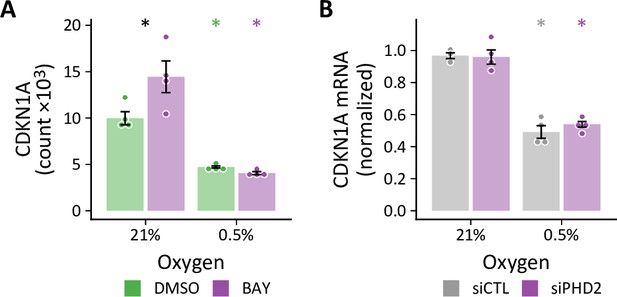
CDKN1A expression in BAY- and siPHD2-treated cells.
(A) Normalized mRNA counts from RNA-seq analysis of LFs treated with hypoxia ±BAY (n=4). (B) Relative CDKN1A expression by RT-qPCR in siPHD2-treated in normoxia and hypoxia (n=4). Data are mean ± SEM (p<0.05 with black * indicating a significant effect of treatment within a given oxygen tension and colored * indicating a significant effect of oxygen for a given treatment as denoted by the color).

MYC regulates HIF-dependent glycolytic flux.
(A) Representative immunoblot of MYC protein expression in lung fibroblasts (LFs) following 72 hr of treatment with 0.5% oxygen or molidustat BAY, (B). (B) Quantification of band densities from (A). (C) Working hypotheses: (1) Hypoxia-induced MYC antagonizes HIF-dependent metabolic and transcriptional events (upper panel). Silencing MYC with siRNA (siMYC) should therefore increase lactate efflux in hypoxia. (2) HIF activation in normoxia decreases MYC activity and increases glycolysis (lower panel). Therefore, overexpressing MYC (AdMYC) should restore MYC signaling and inhibit lactate efflux. (D) Representative immunoblot of LFs treated with siRNA targeting MYC (M) demonstrating adequate protein knockdown. (E) Growth rates of MYC-knockdown cells cultured in hypoxia. (F) Lactate efflux rates of MYC-knockdown cells cultured in hypoxia. (G) Representative immunoblot of LFs treated with MYC adenovirus. (H) Growth rates of MYC overexpressing cells cultured with BAY. (I) Lactate efflux rates of MYC overexpressing cells cultured with BAY. Data are mean ± SEM (n=3–7, p<0.05 as indicated by black * for differences within groups and colored * for differences between groups defined by the x-axis).
-
Figure 11—source data 1
Uncropped blot images for Figure 11A.
- https://cdn.elifesciences.org/articles/82597/elife-82597-fig11-data1-v1.zip
-
Figure 11—source data 2
Uncropped blot images for Figure 11D and G.
- https://cdn.elifesciences.org/articles/82597/elife-82597-fig11-data2-v1.zip
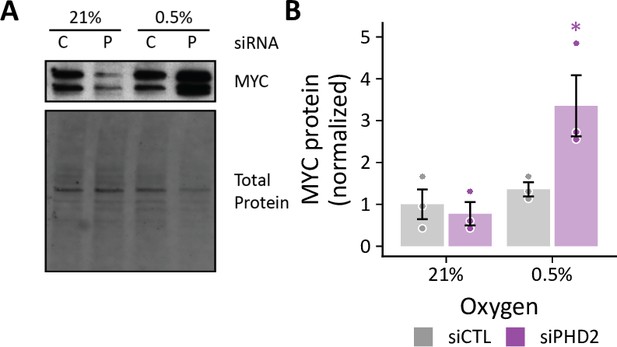
Effects of hypoxia on MYC protein in siPHD2-treated cells.
(A) Representative immunoblot of MYC protein levels in LFs treated with siPHD2 (P) or siCTL (C) and exposed to 0.5% oxygen for 72 hr. (B) Quantification of MYC band densities as in (A). Data are mean ± SEM n=3, p<0.05 as indicated by black * for differences within groups and colored * for differences between groups defined by the x-axis.
-
Figure 11—figure supplement 1—source data 1
Uncropped blot images for Figure 11—figure supplement 1.
- https://cdn.elifesciences.org/articles/82597/elife-82597-fig11-figsupp1-data1-v1.zip
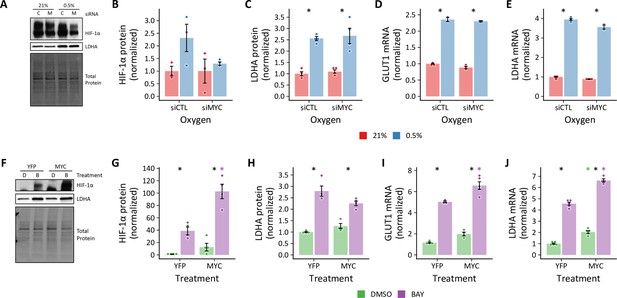
Effects of MYC on HIF stabilization and target gene exprssion.
(A) Representative immunoblot of HIF-1α and LDHA protein levels in LFs treated with siMYC (M) or siCTL (C) and exposed to 0.5% oxygen for 72 hr. (B) Quantification of HIF-1α band densities as in (A). (C) Quanitification of LDHA band densities as in (A). (D) GLUT1 mRNA levels were analyzed by RT-qPCR expressed as fold change relative to 21% siCTL cells. (E) LDHA mRNA levels by RT-qPCR. (F) Representative immunoblot of HIF-1α and LDHA protein levels in LFs treated with MYC (M) or YFP (Y) adenovirus in combination with BAY. (G) Quantification of HIF-1α band densities as in (F). (H) Quanitification of LDHA band densities as in (F). (I) GLUT1 mRNA levels were analyzed by RT-qPCR expressed as fold change relative to 21% siCTL cells. (J) LDHA mRNA levels by RT-qPCR. Data are mean ± SEM n=3–5, p<0.05 as indicated by black * for differences within groups and colored * for differences between groups defined by the x-axis.
-
Figure 11—figure supplement 2—source data 1
Uncropped blot images for Figure 11—figure supplement 2.
- https://cdn.elifesciences.org/articles/82597/elife-82597-fig11-figsupp2-data1-v1.zip
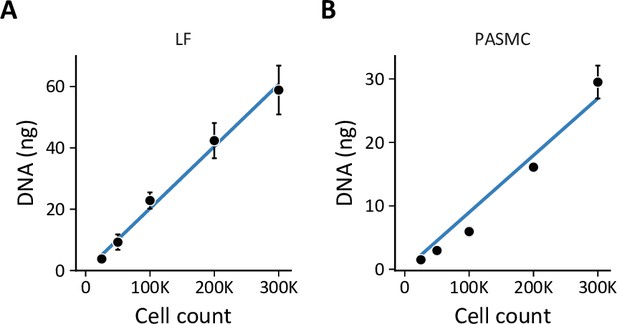
Total DNA as a surrogate for cell count.
(A) Standard curve of lung fibroblast (LF) cell count v. total DNA by PicoGreen measurement used to interpolate cell numbers from DNA measurements. Data are mean ± SEM of three biological replicates. (B) Standard curve of PASMC cell count v. total DNA as in (A).
Tables
LF fluxes in 21% and 0.5% oxygen.
Type | Pathway | ID | Reaction | 21% * | 0.5%† | Ratio | ||||
---|---|---|---|---|---|---|---|---|---|---|
Flux | LB | UB | Flux | LB | UB | |||||
NET | Transport | GLUT | GLC.x → GLC | 5.14E+02 | 5.11E+02 | 5.21E+02 | 4.41E+02 | 4.26E+02 | 4.58E+02 | 0.86 |
PYRR | PYR.x → PYR.c | 7.56E+01 | 7.31E+01 | 7.96E+01 | 6.21E+01 | 5.83E+01 | 6.60E+01 | 0.82 | ||
MCT | LAC ↔ LAC.x | 9.99E+02 | 9.98E+02 | 1.02E+03 | 8.91E+02 | 8.62E+02 | 9.25E+02 | 0.89 | ||
ALAR | ALA → ALA.x | 2.25E+00 | 1.95E+00 | 2.49E+00 | 5.84E-01 | 1.10E-03 | 1.16E+00 | 0.26 | ||
GLNR | GLN.x → GLN | 4.15E+01 | 4.06E+01 | 4.16E+01 | 1.43E+01 | 1.26E+01 | 1.94E+01 | 0.34 | ||
GLUR | GLU ↔ GLU.x | 1.62E+01 | 1.58E+01 | 1.68E+01 | 7.55E+00 | 6.88E+00 | 8.15E+00 | 0.47 | ||
ASPR | ASP → ASP.x | 2.57E+00 | 2.53E+00 | 2.68E+00 | 1.08E+00 | 4.17E-01 | 1.69E+00 | 0.42 | ||
SERR | SER.x → SER | 1.42E+01 | 1.35E+01 | 1.49E+01 | 5.49E+00 | 4.99E+00 | 6.06E+00 | 0.39 | ||
CYSR | CYX.x → CYS +CYS | 4.41E+00 | 4.23E+00 | 4.58E+00 | 1.65E+00 | 1.32E+00 | 2.08E+00 | 0.37 | ||
GLYR | GLY → GLY.x | 2.05E+00 | 1.90E+00 | 2.15E+00 | 2.60E-01 | 2.00E-02 | 4.92E-01 | 0.13 | ||
Glycolysis | HK | GLC → G6P | 5.14E+02 | 5.11E+02 | 5.21E+02 | 4.41E+02 | 4.26E+02 | 4.58E+02 | 0.86 | |
PGI | G6P ↔ F6P | 5.11E+02 | 4.99E+02 | 5.24E+02 | 4.23E+02 | 4.04E+02 | 4.40E+02 | 0.83 | ||
PFK | F6P → FBP | 5.09E+02 | 5.00E+02 | 5.12E+02 | 4.32E+02 | 4.17E+02 | 4.49E+02 | 0.85 | ||
ALDO | FBP ↔ DHAP +GAP | 5.09E+02 | 5.00E+02 | 5.12E+02 | 4.32E+02 | 4.17E+02 | 4.49E+02 | 0.85 | ||
TPI | DHAP ↔ GAP | 5.08E+02 | 5.06E+02 | 5.08E+02 | 4.31E+02 | 4.15E+02 | 4.48E+02 | 0.85 | ||
GAPDH | GAP ↔ 3 PG | 1.02E+03 | 9.96E+02 | 1.04E+03 | 8.69E+02 | 8.35E+02 | 9.03E+02 | 0.85 | ||
ENO | 3 PG → PEP | 1.01E+03 | 9.99E+02 | 1.03E+03 | 8.68E+02 | 8.36E+02 | 9.00E+02 | 0.86 | ||
PK | PEP → PYR.c | 1.04E+03 | 9.95E+02 | 1.04E+03 | 8.78E+02 | 8.36E+02 | 9.21E+02 | 0.84 | ||
LDH | PYR.c ↔ LAC | 9.99E+02 | 9.98E+02 | 1.02E+03 | 8.91E+02 | 8.62E+02 | 9.25E+02 | 0.89 | ||
GPT1 | PYR.c ↔ ALA | 1.19E+01 | 9.12E+00 | 1.19E+01 | 5.55E+00 | –9.08E+02 | 6.13E+00 | 0.47 | ||
GPT2 | PYR.m ↔ ALA | –2.58E+00 | –4.56E+00 | 2.87E+00 | –2.40E-03 | –3.22E+01 | 9.11E+02 | |||
Pentose phosphate pathway | G6PD | G6P → P5P+CO2 | 1.26E-07 | 0.00E+00 | 3.91E-01 | 1.62E+01 | 4.41E+00 | 2.89E+01 | 128571428.57 | |
TK1 | P5P+P5 P ↔ S7P+GAP | –9.11E-01 | –9.29E-01 | –8.30E-01 | 4.76E+00 | –1.22E-01 | 9.62E+00 | –5.23 | ||
TA | S7P+GAP ↔ F6P+E4 P | –9.11E-01 | –9.29E-01 | –8.30E-01 | 4.76E+00 | –1.22E-01 | 9.62E+00 | –5.23 | ||
TK2 | P5P+E4 P ↔ F6P+GAP | –9.11E-01 | –9.29E-01 | –8.30E-01 | 4.76E+00 | –1.22E-01 | 9.62E+00 | –5.23 | ||
Anaplerosis | PYRT | PYR.c → PYR.m | 1.16E+02 | 1.16E+02 | 1.19E+02 | 4.42E+01 | 3.82E+01 | 9.58E+02 | ||
PC | PYR.m+CO2 → OAC | 1.88E+01 | 1.74E+01 | 1.91E+01 | 1.37E+01 | 9.82E+00 | 2.69E+01 | |||
PEPCK | OAC → PEP +CO2 | 2.56E+01 | 1.58E+01 | 2.57E+01 | 9.66E+00 | 0.00E+00 | 2.60E+01 | |||
ME2 | MAL → PYR.m +CO2 | 2.05E+00 | 9.51E-02 | 2.68E+00 | 1.00E-07 | 0.00E+00 | 2.25E+01 | |||
ME1 | MAL → PYR.c +CO2 | 2.78E-02 | 0.00E+00 | 2.63E+01 | 8.71E-05 | 0.00E+00 | 2.52E+01 | |||
FAO | FAO → AcCoA.m | 1.00E-07 | 0.00E+00 | 2.13E+00 | 6.58E-06 | 0.00E+00 | 7.73E-01 | |||
GLDH | GLU ↔ AKG | 1.71E+01 | 1.56E+01 | 1.84E+01 | 9.11E-01 | –6.16E-01 | 7.27E+00 | 0.05 | ||
GLS | GLN ↔ GLU | 3.78E+01 | 3.60E+01 | 3.86E+01 | 1.17E+01 | 1.01E+01 | 1.70E+01 | 0.31 | ||
Tricarboxylic acid cycle | PDH | PYR.m → AcCoA.m +CO2 | 1.02E+02 | 8.76E+01 | 1.15E+02 | 3.05E+01 | 2.86E+01 | 5.24E+01 | 0.3 | |
CS | AcCoA.m+OAC → CIT | 1.02E+02 | 8.30E+01 | 1.11E+02 | 3.05E+01 | 2.88E+01 | 5.09E+01 | 0.3 | ||
IDH | CIT ↔ AKG +CO2 | 2.49E+01 | 2.42E+01 | 2.53E+01 | 1.01E+01 | 8.75E+00 | 1.41E+01 | 0.41 | ||
OGDH | AKG → SUC +CO2 | 4.19E+01 | 4.01E+01 | 4.25E+01 | 1.10E+01 | 7.87E+00 | 2.02E+01 | 0.26 | ||
SDH | SUC ↔ FUM | 4.19E+01 | 4.01E+01 | 4.25E+01 | 1.10E+01 | 7.87E+00 | 2.02E+01 | 0.26 | ||
FH | FUM ↔ MAL | 4.19E+01 | 4.01E+01 | 4.25E+01 | 1.10E+01 | 7.87E+00 | 2.02E+01 | 0.26 | ||
MDH | MAL ↔ OAC | 1.17E+02 | 1.08E+02 | 1.24E+02 | 3.14E+01 | 2.62E+01 | 5.70E+01 | 0.27 | ||
GOT | OAC ↔ ASP | 8.11E+00 | 8.06E+00 | 8.23E+00 | 4.98E+00 | 4.32E+00 | 5.64E+00 | 0.61 | ||
Amino acid metabolism | PST | 3 PG → SER | 1.95E+00 | 1.63E+00 | 2.00E+00 | 2.42E-01 | 1.34E-01 | 3.57E+01 | ||
SHT | SER ↔ GLY +MEETHF | 6.38E+00 | 6.22E+00 | 6.43E+00 | 3.91E+00 | 3.71E+00 | 4.10E+00 | 0.61 | ||
CYST | SER ↔ CYS | –7.12E+00 | –7.19E+00 | –6.81E+00 | –2.10E+00 | –2.97E+00 | –1.44E+00 | 0.3 | ||
SD | SER → PYR.c | 1.17E+01 | 1.04E+01 | 1.20E+01 | 2.82E-01 | 0.00E+00 | 1.47E+00 | 0.02 | ||
GLYS | CO2 +MEETHF → GLY | 3.39E+00 | 3.35E+00 | 3.49E+00 | 1.80E+00 | 1.66E+00 | 1.93E+00 | 0.53 | ||
Biomass | BIOMASS | 1216*AcCoA.c+295.6 *ALA +232.4*ASP +114.7*CO2 +71.43*CYS +57.14*DHAP +142.4*G6P+158.6 *GLN +190.1*GLU +324.2*GLY +125.6*MEETHF +114.7*P5P+217.2*SER → biomass | 2.38E-02 | 2.34E-02 | 2.39E-02 | 1.68E-02 | 1.61E-02 | 1.75E-02 | 0.71 | |
ACL | CIT → AcCoA.c +MAL | 7.74E+01 | 6.29E+01 | 1.04E+02 | 2.04E+01 | 1.95E+01 | 3.71E+01 | 0.26 | ||
LIPS | AcCoA.c → lipid | 4.84E+01 | 4.55E+01 | 4.84E+01 | 1.00E-07 | 0.00E+00 | 1.68E+01 | 0 | ||
Mixing | cPYR | 0*PYR.c → PYR.ms | 1.00E+00 | 8.47E-01 | 1.00E+00 | 1.42E-01 | 0.00E+00 | 1.00E+00 | ||
mPYR | 0*PYR.m → PYR.ms | 1.00E-07 | 0.00E+00 | 1.53E-01 | 8.58E-01 | 0.00E+00 | 1.00E+00 | |||
sPYR | PYR.ms → PYR.fix | 1.00E+00 | 1.00E+00 | 1.00E+00 | 1.00E+00 | 1.00E+00 | 1.00E+00 | |||
EXCH | Transport | MCT | LAC ↔ LAC.x | 1.00E-07 | 0.00E+00 | 1.05E-01 | 1.52E+03 | 1.35E+03 | 2.41E+03 | 15200000000 |
GLUR | GLU ↔ GLU.x | 5.10E+00 | 4.77E+00 | 5.23E+00 | 1.54E+00 | 1.11E+00 | 2.54E+00 | 0.3 | ||
Glycolysis | PGI | G6P ↔ F6P | 2.78E+05 | 1.77E+05 | Inf | 2.46E+05 | 0.00E+00 | Inf | ||
ALDO | FBP ↔ DHAP +GAP | 1.43E+02 | 1.43E+02 | 1.43E+02 | 3.20E+02 | 2.79E+02 | 3.60E+02 | 2.24 | ||
TPI | DHAP ↔ GAP | 4.33E+03 | 4.33E+03 | 1.09E+04 | 1.70E+03 | 1.06E+03 | 3.06E+03 | 0.39 | ||
GAPDH | GAP ↔ 3 PG | 4.42E+02 | 4.72E+00 | 4.50E+02 | 1.00E-07 | 0.00E+00 | 2.39E+02 | |||
LDH | PYR.c ↔ LAC | 1.63E+03 | 1.62E+03 | 1.80E+03 | 4.80E+00 | 0.00E+00 | 3.51E+02 | 0 | ||
GPT1 | PYR.c ↔ ALA | 1.00E-07 | 0.00E+00 | 2.61E-01 | 8.32E+02 | 0.00E+00 | 9.06E+02 | |||
GPT2 | PYR.m ↔ ALA | 4.21E-04 | 0.00E+00 | 2.92E+00 | 1.28E-04 | 0.00E+00 | ||||
Pentose phosphate pathway | TK1 | P5P+P5 P ↔ S7P+GAP | 9.97E+04 | 6.27E+03 | Inf | 1.47E+02 | 6.67E+01 | 2.60E+02 | 0 | |
TA | S7P+GAP ↔ F6P+E4 P | 5.93E+00 | 5.79E+00 | 6.97E+00 | 2.35E-04 | 0.00E+00 | 7.54E+00 | |||
TK2 | P5P+E4 P ↔ F6P+GAP | 1.00E+07 | -Inf | Inf | 9.05E+00 | 4.10E+00 | 1.43E+01 | |||
Anaplerosis | GLDH | GLU ↔ AKG | 1.52E+03 | 1.52E+03 | 7.13E+03 | 3.78E+02 | 1.93E+02 | 1.94E+03 | ||
GLS | GLN ↔ GLU | 3.99E-01 | 0.00E+00 | 8.04E-01 | 1.00E-07 | 0.00E+00 | 3.84E-01 | |||
Tricarboxylic acid cycle | IDH | CIT ↔ AKG +CO2 | 4.55E+00 | 4.03E+00 | 5.19E+00 | 2.52E+00 | 1.80E+00 | 4.50E+00 | ||
SDH | SUC ↔ FUM | 1.22E+03 | Inf | 7.60E+01 | 2.57E+01 | Inf | ||||
FH | FUM ↔ MAL | 3.66E+05 | 1.95E+05 | Inf | 5.05E+05 | 3.06E+02 | Inf | |||
MDH | MAL ↔ OAC | 1.11E+03 | 7.88E+02 | 2.38E+03 | 1.33E+02 | 7.22E+01 | 3.25E+02 | 0.12 | ||
GOT | OAC ↔ ASP | 1.00E+07 | -Inf | Inf | 4.42E+01 | 0.00E+00 | Inf | |||
Amino acid metabolism | SHT | SER ↔ GLY +MEETHF | 5.10E+00 | 8.92E-01 | 5.25E+00 | 6.07E-07 | 0.00E+00 | 3.32E+02 | ||
CYST | SER ↔ CYS | 1.52E-05 | 0.00E+00 | 2.55E-04 | 1.46E-02 | 0.00E+00 | Inf |
-
*
SSR 391.7 [311.2‐416.6] (95% CI, 362 DOF).
-
†
SSR 334.3 [311.2‐416.6] (95% CI, 362 DOF).
LF fluxes following DMSO and BAY treatment.
Type | Pathway | ID | Reaction | DMSO* | BAY† | Ratio | ||||
---|---|---|---|---|---|---|---|---|---|---|
Flux | LB | UB | Flux | LB | UB | |||||
NET | Transport | GLUT | GLC.x → GLC | 6.12E+02 | 6.12E+02 | 6.12E+02 | 8.80E+02 | 8.80E+02 | 8.80E+02 | 1.44 |
PYRR | PYR.x → PYR.c | 9.98E+01 | 9.95E+01 | 1.01E+02 | 6.06E+01 | 6.06E+01 | 6.06E+01 | 0.61 | ||
MCT | LAC ↔ LAC.x | 8.19E+02 | 8.17E+02 | 8.20E+02 | 1.33E+03 | 1.33E+03 | 1.33E+03 | 1.62 | ||
ALAR | ALA → ALA.x | 2.67E+00 | 2.36E+00 | 3.29E+00 | 5.98E+00 | 5.88E+00 | 6.24E+00 | 2.24 | ||
GLNR | GLN.x → GLN | 3.78E+01 | 3.77E+01 | 3.79E+01 | 2.06E+01 | 2.06E+01 | 2.06E+01 | 0.54 | ||
GLUR | GLU ↔ GLU.x | 1.61E+01 | 1.56E+01 | 1.62E+01 | 1.68E+01 | 1.68E+01 | 1.68E+01 | 1.05 | ||
ASPR | ASP → ASP.x | 2.36E+00 | 2.32E+00 | 2.49E+00 | 1.80E+00 | 1.80E+00 | 1.81E+00 | 0.76 | ||
SERR | SER.x → SER | 1.03E+01 | 1.03E+01 | 1.06E+01 | 2.50E+00 | 2.50E+00 | 2.50E+00 | 0.24 | ||
CYSR | CYX.x → CYS+CYS | 2.79E+00 | 2.79E+00 | 2.95E+00 | 3.07E-01 | 3.06E-01 | 3.07E-01 | 0.11 | ||
GLYR | GLY → GLY.x | 2.52E+00 | 2.30E+00 | 2.73E+00 | 5.52E-01 | 4.30E-01 | 7.45E-01 | 0.22 | ||
Glycolysis | HK | GLC → G6P | 6.12E+02 | 6.12E+02 | 6.12E+02 | 8.80E+02 | 8.80E+02 | 8.80E+02 | 1.44 | |
PGI | G6P ↔ F6P | 6.09E+02 | 6.08E+02 | 6.09E+02 | 8.42E+02 | 8.42E+02 | 8.42E+02 | 1.38 | ||
PFK | F6P → FBP | 6.07E+02 | 6.07E+02 | 6.07E+02 | 8.65E+02 | 8.65E+02 | 8.65E+02 | 1.43 | ||
ALDO | FBP ↔ DHAP+GAP | 6.07E+02 | 6.07E+02 | 6.07E+02 | 8.65E+02 | 8.65E+02 | 8.65E+02 | 1.43 | ||
TPI | DHAP ↔ GAP | 6.06E+02 | 6.06E+02 | 6.06E+02 | 8.65E+02 | 8.65E+02 | 8.65E+02 | 1.43 | ||
GAPDH | GAP ↔ 3PG | 1.21E+03 | 1.21E+03 | 1.21E+03 | 1.74E+03 | 1.74E+03 | 1.74E+03 | 1.44 | ||
ENO | 3PG → PEP | 1.21E+03 | 1.21E+03 | 1.21E+03 | 1.57E+03 | 1.57E+03 | 1.57E+03 | 1.3 | ||
PK | PEP → PYR.c | 1.23E+03 | 1.19E+03 | 1.23E+03 | 1.65E+03 | 1.65E+03 | 1.65E+03 | 1.34 | ||
LDH | PYR.c ↔ LAC | 8.19E+02 | 8.17E+02 | 8.20E+02 | 1.33E+03 | 1.33E+03 | 1.33E+03 | 1.62 | ||
GPT1 | PYR.c ↔ ALA | 9.62E+00 | 9.44E+00 | 9.62E+00 | 9.36E+00 | 9.32E+00 | 9.42E+00 | 0.97 | ||
GPT2 | PYR.m ↔ ALA | 1.14E-01 | 2.28E-07 | –1.22E-05 | 6.41E-04 | |||||
Pentose phosphate pathway | G6PD | G6P → P5P+CO2 | 2.02E-02 | 0.00E+00 | 1.08E+00 | 3.64E+01 | 3.64E+01 | 3.64E+01 | 1801.98 | |
TK1 | P5P+P5P ↔ S7P+GAP | –9.06E-01 | –9.28E-01 | –9.06E-01 | 1.17E+01 | 1.17E+01 | 1.17E+01 | –12.89 | ||
TA | S7P+GAP ↔ F6P+E4P | –9.06E-01 | –9.28E-01 | –9.06E-01 | 1.17E+01 | 1.17E+01 | 1.17E+01 | –12.89 | ||
TK2 | P5P+E4P ↔ F6P+GAP | –9.06E-01 | –9.28E-01 | –9.06E-01 | 1.17E+01 | 1.17E+01 | 1.17E+01 | –12.89 | ||
Anaplerosis | PYRT | PYR.c → PYR.m | 4.99E+02 | 4.97E+02 | 4.99E+02 | 5.50E+02 | 5.50E+02 | 5.50E+02 | 1.1 | |
PC | PYR.m+CO2 → OAC | 2.11E+01 | 2.07E+01 | 2.17E+01 | 9.05E+01 | 9.05E+01 | 9.05E+01 | 4.28 | ||
PEPCK | OAC → PEP+CO2 | 1.36E+01 | 1.36E+01 | 1.37E+01 | 8.58E+01 | 8.58E+01 | 8.58E+01 | 6.31 | ||
ME2 | MAL → PYR.m+CO2 | 1.30E+01 | 1.28E+01 | 1.37E+01 | 1.00E-07 | 0.00E+00 | 9.49E-06 | 0 | ||
ME1 | MAL → PYR.c+CO2 | 3.20E-03 | 0.00E+00 | 1.73E+00 | 1.00E-07 | 0.00E+00 | 2.15E-05 | |||
FAO | FAO → AcCoA.m | 1.00E-07 | 0.00E+00 | 3.48E+00 | 1.09E-04 | 8.34E-06 | 4.14E-02 | |||
GLDH | GLU ↔ AKG | 1.33E+01 | 1.31E+01 | 1.35E+01 | –2.46E-01 | –2.47E-01 | –2.46E-01 | –0.02 | ||
GLS | GLN ↔ GLU | 3.40E+01 | 3.35E+01 | 3.42E+01 | 1.88E+01 | 1.88E+01 | 1.88E+01 | 0.55 | ||
Tricarboxylic acid cycle | PDH | PYR.m → AcCoA.m+CO2 | 4.90E+02 | 4.90E+02 | 4.92E+02 | 4.60E+02 | 4.60E+02 | 4.60E+02 | 0.94 | |
CS | AcCoA.m+OAC → CIT | 4.90E+02 | 4.84E+02 | 4.91E+02 | 4.60E+02 | 4.60E+02 | 4.60E+02 | 0.94 | ||
IDH | CIT ↔ AKG+CO2 | 2.70E+01 | 2.70E+01 | 2.76E+01 | 1.45E+01 | 1.45E+01 | 1.45E+01 | 0.54 | ||
OGDH | AKG → SUC+CO2 | 4.03E+01 | 3.99E+01 | 4.04E+01 | 1.43E+01 | 1.43E+01 | 1.43E+01 | 0.35 | ||
SDH | SUC ↔ FUM | 4.03E+01 | 3.99E+01 | 4.04E+01 | 1.43E+01 | 1.43E+01 | 1.43E+01 | 0.35 | ||
FH | FUM ↔ MAL | 4.03E+01 | 3.99E+01 | 4.04E+01 | 1.43E+01 | 1.43E+01 | 1.43E+01 | 0.35 | ||
MDH | MAL ↔ OAC | 4.91E+02 | 4.91E+02 | 4.92E+02 | 4.60E+02 | 4.60E+02 | 4.60E+02 | 0.94 | ||
GOT | OAC ↔ ASP | 7.91E+00 | 7.76E+00 | 7.98E+00 | 4.46E+00 | 4.46E+00 | 4.46E+00 | 0.56 | ||
Amino acid metabolism | PST | 3PG → SER | 4.03E-01 | 3.74E-01 | 5.04E-01 | 1.73E+02 | 1.73E+02 | 1.73E+02 | 429.83 | |
SHT | SER ↔ GLY+MEETHF | 6.63E+00 | 6.59E+00 | 6.65E+00 | 2.85E+00 | 2.79E+00 | 2.93E+00 | 0.43 | ||
CYST | SER ↔ CYS | –3.88E+00 | –3.91E+00 | –3.87E+00 | 2.03E-01 | 2.02E-01 | 2.03E-01 | –0.05 | ||
SD | SER → PYR.c | 2.80E+00 | 2.80E+00 | 2.80E+00 | 1.70E+02 | 1.70E+02 | 1.70E+02 | 60.81 | ||
GLYS | CO2+MEETHF → GLY | 3.63E+00 | 3.50E+00 | 3.65E+00 | 1.41E+00 | 1.30E+00 | 1.46E+00 | 0.39 | ||
Biomass | BIOMASS | 1216*AcCoA.c+295.6 *ALA +232.4*ASP+114.7*CO2+71.43*CYS+57.14*DHAP+142.4*G6P+ 158.6*GLN+190.1*GLU +324.2*GLY+125.6*MEETHF+114.7*P5P+217.2 *SER → biomass | 2.39E-02 | 2.39E-02 | 2.50E-02 | 1.14E-02 | 1.14E-02 | 1.14E-02 | 0.48 | |
ACL | CIT → AcCoA.c+MAL | 4.63E+02 | 4.63E+02 | 4.66E+02 | 4.45E+02 | 4.45E+02 | 4.45E+02 | 0.96 | ||
LIPS | AcCoA.c → lipid | 4.34E+02 | 4.29E+02 | 4.34E+02 | 4.32E+02 | 4.32E+02 | 4.32E+02 | |||
Mixing | cPYR | 0*PYR.c → PYR.ms | 1.00E+00 | 9.99E-01 | 1.00E+00 | 1.00E-07 | 0.00E+00 | 1.00E+00 | ||
mPYR | 0*PYR.m → PYR.ms | 1.00E-07 | 0.00E+00 | 9.83E-04 | 1.00E+00 | 0.00E+00 | 1.00E+00 | |||
sPYR | PYR.ms → PYR.fix | 1.00E+00 | 1.00E+00 | 1.00E+00 | 1.00E+00 | 1.00E+00 | 1.00E+00 | |||
EXCH | Transport | MCT | LAC ↔ LAC.x | 6.24E-04 | 0.00E+00 | 3.56E+00 | 7.11E+02 | 7.11E+02 | 7.11E+02 | 1139423.08 |
GLUR | GLU ↔ GLU.x | 5.06E+00 | 4.82E+00 | 5.75E+00 | 3.48E+00 | 3.48E+00 | 3.48E+00 | 0.69 | ||
Glycolysis | PGI | G6P ↔ F6P | 1.40E+06 | 1.39E+06 | Inf | 4.31E+06 | 4.31E+06 | 4.31E+06 | ||
ALDO | FBP ↔ DHAP+GAP | 2.38E+02 | 2.38E+02 | 2.38E+02 | 1.02E+03 | 1.02E+03 | 1.02E+03 | 4.28 | ||
TPI | DHAP ↔ GAP | 9.99E+06 | Inf | 7.57E+03 | 7.57E+03 | 7.57E+03 | ||||
GAPDH | GAP ↔ 3PG | 5.81E+02 | 5.81E+02 | 7.25E+02 | 1.09E+02 | 1.07E+02 | 1.09E+02 | 0.19 | ||
LDH | PYR.c ↔ LAC | 2.65E+03 | 2.58E+03 | 2.65E+03 | 4.92E+01 | 4.91E+01 | 4.94E+01 | 0.02 | ||
GPT1 | PYR.c ↔ ALA | 1.00E-07 | 0.00E+00 | 5.60E-02 | 2.45E+03 | 2.45E+03 | 2.45E+03 | 24500000000 | ||
GPT2 | PYR.m ↔ ALA | 1.00E-07 | 0.00E+00 | 5.65E-02 | 1.00E-07 | 0.00E+00 | 1.20E-05 | |||
Pentose phosphate pathway | TK1 | P5P+P5P ↔ S7P+GAP | 1.28E+06 | 9.01E+03 | Inf | 1.00E+07 | -Inf | Inf | ||
TA | S7P+GAP ↔ F6P+E4P | 8.89E+00 | 8.88E+00 | 9.53E+00 | 5.10E+01 | 5.10E+01 | 5.10E+01 | 5.74 | ||
TK2 | P5P+E4P ↔ F6P+GAP | 6.93E+00 | 5.12E+00 | 6.98E+00 | 1.00E-07 | 0.00E+00 | 1.56E-04 | 0 | ||
Anaplerosis | GLDH | GLU ↔ AKG | 5.63E+03 | 4.43E+03 | 5.66E+03 | 1.42E+03 | 1.42E+03 | 1.42E+03 | 0.25 | |
GLS | GLN ↔ GLU | 1.27E+00 | 1.20E+00 | 1.50E+00 | 5.52E-01 | 5.51E-01 | 5.55E-01 | 0.43 | ||
Tricarboxylic acid cycle | IDH | CIT ↔ AKG+CO2 | 3.36E+00 | 3.24E+00 | 3.92E+00 | 4.66E+00 | 4.66E+00 | 4.66E+00 | 1.39 | |
SDH | SUC ↔ FUM | 4.30E+02 | 4.30E+02 | 1.46E+06 | 1.04E+04 | 1.04E+04 | 1.04E+04 | |||
FH | FUM ↔ MAL | 7.29E+06 | -Inf | Inf | 4.56E+06 | 4.56E+06 | 4.56E+06 | |||
MDH | MAL ↔ OAC | 5.49E+02 | 5.47E+02 | 5.49E+02 | 1.00E-07 | 0.00E+00 | 6.30E-03 | 0 | ||
GOT | OAC ↔ ASP | 1.04E+02 | 1.04E+02 | 1.04E+02 | 4.76E+05 | 4.76E+05 | 4.76E+05 | 4576.92 | ||
Amino acid metabolism | SHT | SER ↔ GLY+MEETHF | 1.39E+00 | 1.37E+00 | 1.41E+00 | 1.86E+03 | 1.86E+03 | 1.86E+03 | 1338.13 | |
CYST | SER ↔ CYS | 1.25E-07 | 0.00E+00 | 4.22E-02 | 1.33E-01 | 1.33E-01 | 1.33E-01 | 1064000 |
-
*
SSR 393.5 [311.2–416.6] (95% CI, 362 DOF).
-
†
SSR 392.4 [308.4–413.4] (95% CI, 359 DOF).
PASMC fluxes in 21% and 0.5% oxygen.
Type | Pathway | ID | Reaction | 21%* | 0.5%† | Ratio | ||||
---|---|---|---|---|---|---|---|---|---|---|
Flux | LB | UB | Flux | LB | UB | |||||
NET | Transport | GLUT | GLC.x → GLC | 4.28E+02 | 4.28E+02 | 4.28E+02 | 3.65E+02 | 3.65E+02 | 3.65E+02 | 0.85 |
PYRR | PYR.x → PYR.c | 1.04E+02 | 1.02E+02 | 1.09E+02 | 4.53E+01 | 4.31E+01 | 4.57E+01 | 0.44 | ||
MCT | LAC ↔ LAC.x | 8.01E+02 | 8.01E+02 | 8.04E+02 | 6.49E+02 | 6.49E+02 | 6.49E+02 | 0.81 | ||
ALAR | ALA → ALA.x | 1.43E+01 | 1.43E+01 | 1.46E+01 | 7.83E+00 | 7.83E+00 | 8.24E+00 | 0.55 | ||
GLNR | GLN.x → GLN | 7.73E+01 | 7.53E+01 | 7.73E+01 | 1.77E+02 | 1.77E+02 | 1.77E+02 | 2.29 | ||
GLUR | GLU ↔ GLU.x | 2.53E+01 | 2.52E+01 | 2.54E+01 | 1.19E+01 | 1.19E+01 | 1.22E+01 | 0.47 | ||
ASPR | ASP → ASP.x | 7.01E+00 | 6.99E+00 | 7.02E+00 | 6.92E+00 | 6.84E+00 | 7.00E+00 | |||
SERR | SER.x → SER | 2.54E+00 | 2.48E+00 | 2.55E+00 | 2.57E+00 | 2.55E+00 | 2.57E+00 | 1.01 | ||
CYSR | CYX.x → CYS +CYS | 6.39E+00 | 6.34E+00 | 6.45E+00 | 3.75E+00 | 3.75E+00 | 3.75E+00 | 0.59 | ||
GLYR | GLY → GLY.x | 3.66E-01 | 3.03E-01 | 4.19E-01 | 4.06E-01 | 3.86E-01 | 4.25E-01 | |||
Glycolysis | HK | GLC → G6P | 4.28E+02 | 4.28E+02 | 4.28E+02 | 3.65E+02 | 3.65E+02 | 3.65E+02 | 0.85 | |
PGI | G6P ↔ F6P | 4.06E+02 | 4.06E+02 | 4.07E+02 | 3.62E+02 | 3.62E+02 | 3.63E+02 | 0.89 | ||
PFK | F6P → FBP | 4.17E+02 | 4.17E+02 | 4.18E+02 | 3.61E+02 | 3.60E+02 | 3.61E+02 | 0.87 | ||
ALDO | FBP ↔ DHAP +GAP | 4.17E+02 | 4.17E+02 | 4.18E+02 | 3.61E+02 | 3.60E+02 | 3.61E+02 | 0.87 | ||
TPI | DHAP ↔ GAP | 4.16E+02 | 4.16E+02 | 4.16E+02 | 3.60E+02 | 3.60E+02 | 3.60E+02 | 0.87 | ||
GAPDH | GAP ↔ 3 PG | 8.39E+02 | 8.39E+02 | 8.41E+02 | 7.21E+02 | 7.21E+02 | 7.21E+02 | 0.86 | ||
ENO | 3 PG → PEP | 8.36E+02 | 8.35E+02 | 8.53E+02 | 7.20E+02 | 7.20E+02 | 7.20E+02 | 0.86 | ||
PK | PEP → PYR.c | 9.31E+02 | 9.30E+02 | 9.31E+02 | 9.24E+02 | 9.24E+02 | 9.24E+02 | 0.99 | ||
LDH | PYR.c ↔ LAC | 8.01E+02 | 8.01E+02 | 8.04E+02 | 6.49E+02 | 6.49E+02 | 6.49E+02 | 0.81 | ||
GPT1 | PYR.c ↔ ALA | 1.64E+02 | 1.62E+02 | 1.92E+02 | –1.36E+01 | –1.39E+01 | –1.35E+01 | –0.08 | ||
GPT2 | PYR.m ↔ ALA | –1.43E+02 | –1.43E+02 | –1.42E+02 | 2.62E+01 | 2.51E+01 | 2.65E+01 | –0.18 | ||
Pentose phosphate pathway | G6PD | G6P → P5P+CO2 | 1.89E+01 | 1.57E+01 | 1.93E+01 | 1.16E-07 | 0.00E+00 | 1.10E-03 | 0 | |
TK1 | P5P+P5 P ↔ S7P+GAP | 5.46E+00 | 4.44E+00 | 5.96E+00 | –6.15E-01 | –6.15E-01 | –5.77E-01 | –0.11 | ||
TA | S7P+GAP ↔ F6P+E4 P | 5.46E+00 | 4.44E+00 | 5.96E+00 | –6.15E-01 | –6.15E-01 | –5.77E-01 | –0.11 | ||
TK2 | P5P+E4 P ↔ F6P+GAP | 5.46E+00 | 4.44E+00 | 5.96E+00 | –6.15E-01 | –6.15E-01 | –5.77E-01 | –0.11 | ||
Anaplerosis | PYRT | PYR.c → PYR.m | 7.60E+01 | 7.59E+01 | 7.66E+01 | 3.36E+02 | 3.36E+02 | 3.36E+02 | 4.42 | |
PC | PYR.m+CO2 → OAC | 6.30E+01 | 6.29E+01 | 6.59E+01 | 2.37E+02 | 2.36E+02 | 2.37E+02 | 3.76 | ||
PEPCK | OAC → PEP +CO2 | 9.51E+01 | 9.51E+01 | 9.53E+01 | 2.03E+02 | 2.03E+02 | 2.04E+02 | 2.14 | ||
ME2 | MAL → PYR.m +CO2 | 1.20E-03 | 0.00E+00 | 5.20E-03 | 1.82E+02 | 1.81E+02 | 1.82E+02 | 151517.08 | ||
ME1 | MAL → PYR.c +CO2 | 3.29E-05 | 0.00E+00 | 1.15E+00 | 5.91E-05 | 0.00E+00 | 8.06E-02 | |||
FAO | FAO → AcCoA.m | 1.00E-07 | 0.00E+00 | 1.32E-02 | 1.15E-04 | 0.00E+00 | 1.56E-01 | |||
GLDH | GLU ↔ AKG | 4.43E+01 | 4.42E+01 | 4.45E+01 | 1.59E+02 | 1.59E+02 | 1.59E+02 | 3.6 | ||
GLS | GLN ↔ GLU | 7.38E+01 | 7.36E+01 | 7.38E+01 | 1.74E+02 | 1.74E+02 | 1.74E+02 | 2.36 | ||
Tricarboxylic acid cycle | PDH | PYR.m → AcCoA.m +CO2 | 1.56E+02 | 1.48E+02 | 1.66E+02 | 2.55E+02 | 2.55E+02 | 2.55E+02 | 1.63 | |
CS | AcCoA.m+OAC → CIT | 1.56E+02 | 1.56E+02 | 1.58E+02 | 2.55E+02 | 2.55E+02 | 2.55E+02 | 1.63 | ||
IDH | CIT ↔ AKG +CO2 | 2.11E+01 | 2.10E+01 | 2.11E+01 | 2.16E+01 | 2.16E+01 | 2.16E+01 | 1.03 | ||
OGDH | AKG → SUC +CO2 | 6.54E+01 | 6.51E+01 | 6.59E+01 | 1.81E+02 | 1.80E+02 | 1.81E+02 | 2.77 | ||
SDH | SUC ↔ FUM | 6.54E+01 | 6.51E+01 | 6.59E+01 | 1.81E+02 | 1.80E+02 | 1.81E+02 | 2.77 | ||
FH | FUM ↔ MAL | 6.54E+01 | 6.51E+01 | 6.59E+01 | 1.81E+02 | 1.80E+02 | 1.81E+02 | 2.77 | ||
MDH | MAL ↔ OAC | 2.01E+02 | 2.01E+02 | 2.01E+02 | 2.32E+02 | 2.32E+02 | 2.33E+02 | 1.16 | ||
GOT | OAC ↔ ASP | 1.22E+01 | 1.17E+01 | 1.24E+01 | 1.07E+01 | 1.06E+01 | 1.07E+01 | 0.87 | ||
Amino acid metabolism | PST | 3 PG → SER | 2.69E+00 | 2.57E+00 | 2.80E+00 | 7.12E-01 | 7.01E-01 | 7.21E-01 | 0.26 | |
SHT | SER ↔ GLY +MEETHF | 5.19E+00 | 5.15E+00 | 5.20E+00 | 3.82E+00 | 3.81E+00 | 3.86E+00 | 0.74 | ||
CYST | SER ↔ CYS | –1.12E+01 | –1.17E+01 | –1.11E+01 | –6.35E+00 | –6.35E+00 | –6.35E+00 | 0.57 | ||
SD | SER → PYR.c | 6.39E+00 | 6.23E+00 | 6.44E+00 | 2.33E+00 | 2.33E+00 | 2.33E+00 | 0.36 | ||
GLYS | CO2 +MEETHF → GLY | 2.39E+00 | 2.36E+00 | 2.42E+00 | 1.80E+00 | 1.79E+00 | 1.81E+00 | 0.75 | ||
Biomass | BIOMASS | 978*AcCoA.c+237.8* ALA +187*ASP +92.3* CO2 +57.46*CYS +45.97*DHAP +114.5*G6P+127.6* GLN +153*GLU +260.8*GLY +101.1*MEETHF +92.3*P5P+174.8* SER → biomass | 2.77E-02 | 2.70E-02 | 2.79E-02 | 2.00E-02 | 2.00E-02 | 2.00E-02 | 0.72 | |
ACL | CIT → AcCoA.c +MAL | 1.35E+02 | 1.34E+02 | 1.38E+02 | 2.33E+02 | 2.33E+02 | 2.33E+02 | 1.72 | ||
LIPS | AcCoA.c → lipid | 1.08E+02 | 9.99E+01 | 1.08E+02 | 2.14E+02 | 2.14E+02 | 2.14E+02 | 1.98 | ||
Mixing | cPYR | 0*PYR.c → PYR.ms | 5.77E-01 | 5.64E-01 | 5.92E-01 | 1.00E+00 | 9.96E-01 | 1.00E+00 | 1.73 | |
mPYR | 0*PYR.m → PYR.ms | 4.23E-01 | 4.08E-01 | 4.36E-01 | 1.00E-07 | 0.00E+00 | 4.40E-03 | 0 | ||
sPYR | PYR.ms → PYR.fix | 1.00E+00 | 1.00E+00 | 1.00E+00 | 1.00E+00 | 1.00E+00 | 1.00E+00 | |||
EXCH | Transport | MCT | LAC ↔ LAC.x | 1.00E-07 | 0.00E+00 | 1.36E+02 | 1.64E+03 | 1.63E+03 | 1.65E+03 | 16400000000 |
GLUR | GLU ↔ GLU.x | 1.00E-07 | 0.00E+00 | 2.27E-02 | 5.69E-05 | 0.00E+00 | 1.71E-02 | |||
Glycolysis | PGI | G6P ↔ F6P | 4.88E+06 | 4.88E+06 | Inf | 9.92E+06 | 9.85E+04 | Inf | ||
ALDO | FBP ↔ DHAP +GAP | 2.89E+02 | 2.80E+02 | 2.89E+02 | 2.57E+02 | 2.56E+02 | 2.57E+02 | 0.89 | ||
TPI | DHAP ↔ GAP | 9.86E+06 | -Inf | Inf | 1.65E+03 | 1.63E+03 | 1.68E+03 | |||
GAPDH | GAP ↔ 3 PG | 1.12E+03 | 0.00E+00 | 5.88E+05 | 1.00E-07 | 0.00E+00 | 2.27E-01 | |||
LDH | PYR.c ↔ LAC | 1.47E+03 | 1.39E+03 | 1.47E+03 | 4.49E+02 | 4.49E+02 | 4.49E+02 | 0.31 | ||
GPT1 | PYR.c ↔ ALA | 2.74E+02 | 2.73E+02 | 2.77E+02 | 1.00E-07 | 0.00E+00 | 4.28E-02 | 0 | ||
GPT2 | PYR.m ↔ ALA | 1.38E+02 | 1.38E+02 | 1.49E+02 | 9.64E+01 | 0.00E+00 | 1.01E+02 | 0.7 | ||
Pentose phosphate pathway | TK1 | P5P+P5 P ↔ S7P+GAP | 7.99E+02 | 7.97E+02 | 8.08E+02 | 3.54E+01 | 3.54E+01 | 3.55E+01 | 0.04 | |
TA | S7P+GAP ↔ F6P+E4 P | 1.53E-01 | 0.00E+00 | 5.82E-01 | 2.55E+00 | 2.54E+00 | 2.57E+00 | 16.67 | ||
TK2 | P5P+E4 P ↔ F6P+GAP | 3.33E+00 | 2.62E+00 | 3.35E+00 | 1.29E+01 | 1.29E+01 | 1.29E+01 | 3.88 | ||
Anaplerosis | GLDH | GLU ↔ AKG | 5.36E+02 | 5.34E+02 | 8.37E+02 | 1.23E+03 | 1.23E+03 | 1.23E+03 | 2.29 | |
GLS | GLN ↔ GLU | 3.20E-01 | 0.00E+00 | 2.74E+00 | 1.12E+00 | 1.07E+00 | 1.74E+00 | |||
Tricarboxylic acid cycle | IDH | CIT ↔ AKG +CO2 | 1.04E+01 | 1.02E+01 | 1.04E+01 | 6.30E+01 | 6.30E+01 | 6.31E+01 | 6.09 | |
SDH | SUC ↔ FUM | 2.78E-01 | 0.00E+00 | Inf | 3.34E+06 | 3.34E+06 | 3.34E+06 | |||
FH | FUM ↔ MAL | 1.03E-04 | 0.00E+00 | 1.58E+01 | 2.18E+02 | 2.18E+02 | 2.18E+02 | 2114238.83 | ||
MDH | MAL ↔ OAC | 1.01E+03 | 8.27E+02 | 1.01E+03 | 3.67E+03 | 3.67E+03 | 3.69E+03 | 3.63 | ||
GOT | OAC ↔ ASP | 2.27E+02 | 2.27E+02 | 2.47E+02 | 1.54E+01 | 1.54E+01 | 1.55E+01 | 0.07 | ||
Amino acid metabolism | SHT | SER ↔ GLY +MEETHF | 3.55E+00 | 3.52E+00 | 3.59E+00 | 1.60E-01 | 1.36E-01 | 1.70E-01 | 0.05 | |
CYST | SER ↔ CYS | 1.04E+03 | 1.03E+03 | 1.04E+03 | 2.00E-03 | 0.00E+00 | 2.00E-03 | 0 |
-
*
SSR 575.6 [499.1–630.6] (95% CI, 563 DOF).
-
†
SSR 521.3 [482.2–611.6] (95% CI, 545 DOF).
Reagent type (species) or resource | Designation | Source or reference | Identifiers | Additional information |
---|---|---|---|---|
Antibody | anti-HIF-1α (Mouse monoclonal) | BD Biosciences | 610958 | 1:1000 |
Antibody | anti-c-MYC (Rabbit monoclonal) | Cell Signaling Technologies | D84C12 | 1:1000 |
Antibody | anti-LDHA (Rabbit polyclonal) | Cell Signaling Technologies | 2012 | 1:1000 |
Antibody | HRP-anti-Rabbit IgG (Goat polyclonal) | Cell Signaling Technologies | 7074 | 1:5000 |
Antibody | HRP-anti-Mouse IgG (Goat polyclonal) | Cell Signaling Technologies | 7076 | 1:5000 |
Transfected construct (human) | MYC | Vector Biolabs | 1285 | adenovirus to express MYC |
Transfected construct (human) | YFP | Oldham et al., 2015 | adenovirus control to express YFP | |
Chemical compound, drug | [1,2–13 C2]-glucose | Cambridge Isotope Labs | CLM-504-PK | |
Chemical compound, drug | [U-13C6]-glucose | Cambridge Isotope Labs | CLM-1396-PK | |
Chemical compound, drug | [U-13C5]-glutamine | Cambridge Isotope Labs | CLM-1822-H-PK | |
Chemical compound, drug | [U-13C3]-lactate | Sigma | 485926 | |
Chemical compound, drug | BAY | Cayman | 15297 | Molidustat (BAY-85–3934); 10 μM in DMSO |
Commercial assay or kit | Glucose colorimetric assay kit | Cayman | 10009582 | |
Commercial assay or kit | ʟ-Lactate assay kit | Cayman | 700510 | |
Commercial assay or kit | Pyruvate assay kit | Cayman | 700470 | |
Commercial assay or kit | NADP/NADPH-Glo Assay | Promega | G9081 | |
Cell line (human) | LFs | Lonza | CC-2512 | Normal human lung fibroblasts |
Cell line (human) | PASMCs | Lonza | CC-2581 | Pulmonary artery smooth muscle cells |
Sequence-based reagent | ACTB | Life Technologies | Hs03023943_g1 | qPCR probe |
Sequence-based reagent | GLUT1 | Life Technologies | Hs00892681_m1 | qPCR probe |
Sequence-based reagent | LDHA | Life Technologies | Hs00855332_g1 | qPCR probe |
Sequence-based reagent | CDKN1A | Life Technologies | Hs00355782_m1 | qPCR probe |
Transfected construct (human) | siMYC | Dharmacon | L-003282-02-0005 | ON-TARGETplus siRNA |
Transfected construct (human) | siPHD2 | Dharmacon | L-004276-00-0005 | ON-TARGETplus siRNA |
Transfected construct (human) | siCTL | Dharmacon | D-001810-10-05 | ON-TARGETplus non-targeting control pool |