Stimulation of the catalytic activity of the tyrosine kinase Btk by the adaptor protein Grb2
Figures
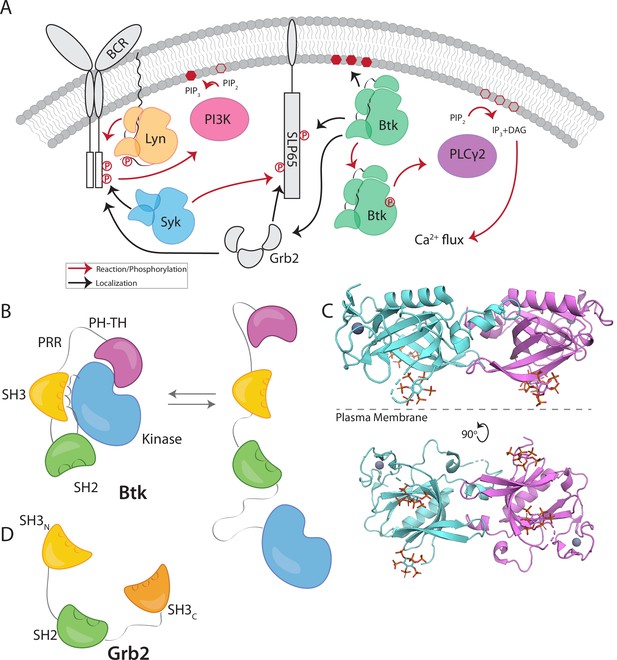
Btk and Grb2 are integral to B-cell signaling.
(A) The B-cell signaling cascade relies on kinases and adaptor molecules. (B) Btk is made up of five distinct domains. The Pleckstrin homology and Tec homology domains fold together into one module (PH-TH), which is connected through a linker containing two proline-rich regions (PRR) to the Src module (SH3, SH2, and Kinase domain). These domains together mediate autoinhibition of Btk. (C) Crystal structure of the Saraste dimer of the Btk PH-TH module, with each module bound to two inositol hexakisphosphate (IP6) molecules, mimicking PIP3 binding (PDB: 4Y94). (D) Grb2 domain architecture consists of two SH3 domains that flank an SH2 domain.
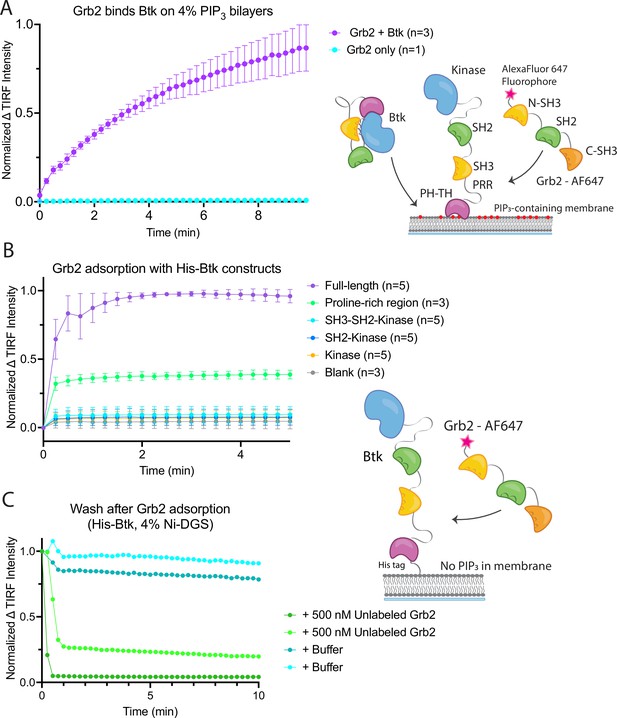
Grb2 interacts with membrane-bound Btk.
(A) Fluorescently labeled Grb2 was added to supported-lipid bilayers containing 4% PIP3, with (n=3) or without (n=1) Btk. The change in fluorescence intensity, monitored by TIRF, is plotted over time. (B) Various constructs of Btk [Btk (n=5), SH3-SH2-Kinase (n=5), SH2-Kinase (n=5), Proline-rich linker (n=3), Kinase (n=5), Blank (no protein, n=3)] with His tags were tethered to supported-lipid bilayers containing 4% DGS-NTA(Ni) lipids. The bilayers were washed after equilibration to remove any weakly bound Btk. Fluorescently-labeled Grb2 was added to these bilayers and the change in fluorescence intensity with time is shown. (C) The binding of Grb2 to membrane bound Btk is reversible. Grb2 was added to a bilayer decorated with His-tagged Btk. In one experiment (blue lines, n=2), the bilayer was washed with buffer, and in another experiment (green lines, n=2), the bilayer was washed with a solution containing 10-fold higher concentration of unlabeled Grb2. All error bars in this figure represent standard deviation across replicates. See Figure 2—source data 1 for TIRF intensity data for A-C, and Dryad repository for raw image files, Figure 2—source data 2 (https://dx.doi.org/10.5061/dryad.8sf7m0ctd).
-
Figure 2—source data 1
Raw image files used to generate Figure 2A–C.
- https://cdn.elifesciences.org/articles/82676/elife-82676-fig2-data1-v1.zip
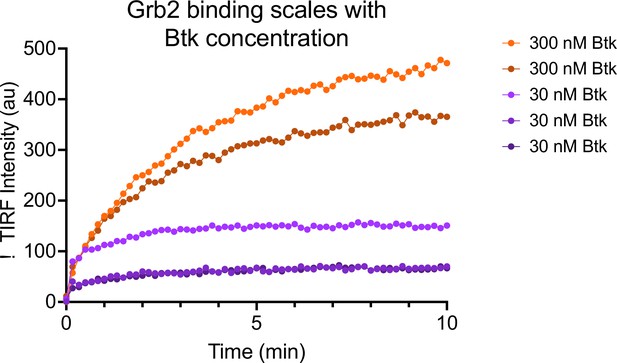
Grb2 adsorption scales with Btk concentration.
4% PIP3-containing bilayers were equilibrated with 50 nM Grb2-AlexFluor647. Subsequently, 30 nM (n = 3 replicates) or 300 nM (n = 2 replicates) Btk was added along with 50 nM Grb2-AlexaFluor647. See Figure 2—source data 1 for TIRF intensity data.
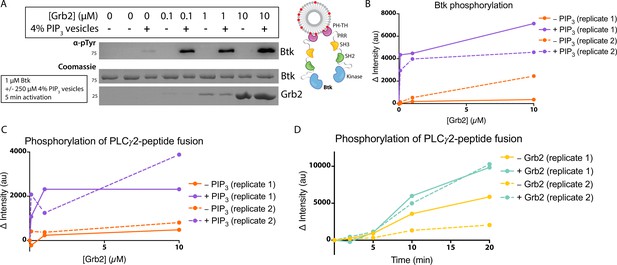
Grb2 enhances Btk kinase activity in a PIP3-dependent manner.
The activity of Btk is monitored by measuring the phosphorylation of Btk, Grb2, and a PLCγ2-peptide fusion protein by western blot. (A) Grb2 was titrated into samples containing Btk with or without 4% PIP3 lipid vesicles. These samples were activated for 5 min and then quenched with 100 mM EDTA. Total phosphorylation was measured by western blot with pan-phosphotyrosine antibody. See Figure 3—figure supplement 1 for an independent experimental replicate, and Figure 3—source data 1. (B) Btk phosphorylation measured from (A) along with one independent experimental replicate (Figure 3—figure supplement 1). (C) Phosphorylation of PLCγ2-peptide fusion by Btk. See blots in Figure 3—figure supplement 2 for raw data. Grb2 is titrated with or without PIP3, and phosphorylation of PLCγ2-peptide fusion is measured. Change in intensity is determined by comparison to the intensity observed for 0 nM Grb2 for either case (+/−Grb2). See Figure 3—source data 1 for raw images. (D) Measure of PLCγ2-peptide fusion phosphorylation over time with or without the addition of Grb2. All samples contain Btk, PLCγ2-peptide fusion and 4% PIP3 lipid vesicles. Samples were activated and quenched at the timepoints listed (0–20 min). See Figure 3—figure supplement 3 for raw data and Figure 3—source data 1.
-
Figure 3—source data 1
Raw images of gels and western membranes referenced in Figure 3.
- https://cdn.elifesciences.org/articles/82676/elife-82676-fig3-data1-v1.zip
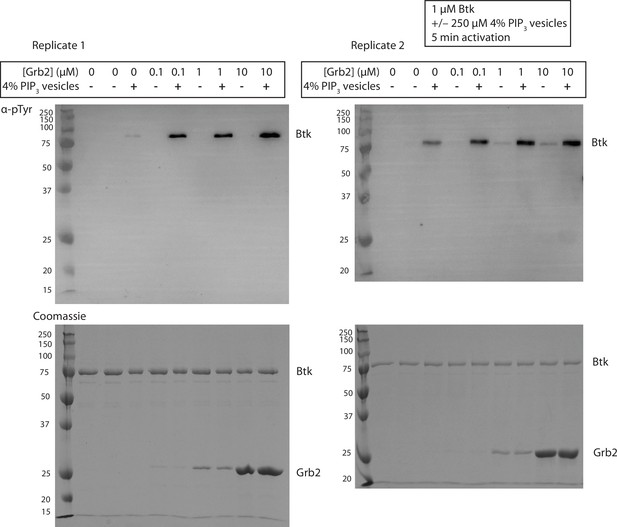
Grb2 enhances trans-autophosphorylation of Btk in the presence of PIP3 containing vesicles.
Entire blot from Figure 3A in replicate, looking at autophosphorylation of Btk. For each lane 1 µM Btk was activated for 5 min in the presence or absence of 250 µM lipids, in the form of single unilamellar vesicles containing 4% PIP3, and the indicated concentrations of Grb2.
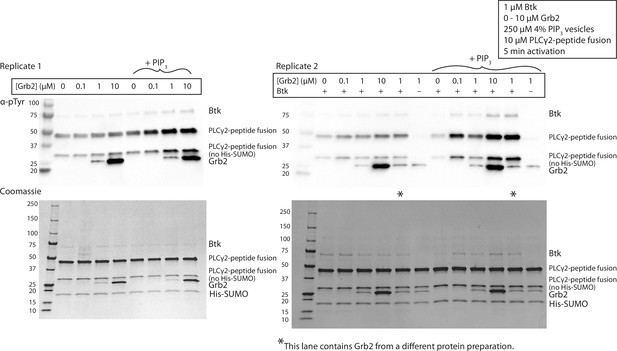
Grb2 enhances phosphorylation of PLCγ2 by Btk in the presence of PIP3 containing vesicles.
Replicate blots quantified in Figure 3C, looking at phosphorylation of PLCγ2-peptide fusion. 1 µM Btk was activated for 10 minutes with or without 250 µM lipids, single unilamellar vesicles containing 4% PIP3, 10 µM PLCγ2 and 0-10 µM Grb2. A phosphorylation signature for Grb2 is observed in the top panels, scaling with the Grb2 concentration in the reaction. MS/MS failed to detect any phosphorylation on Grb2, and the signal is still observed in the absence of Btk, indicating a non-specific antibody interaction.
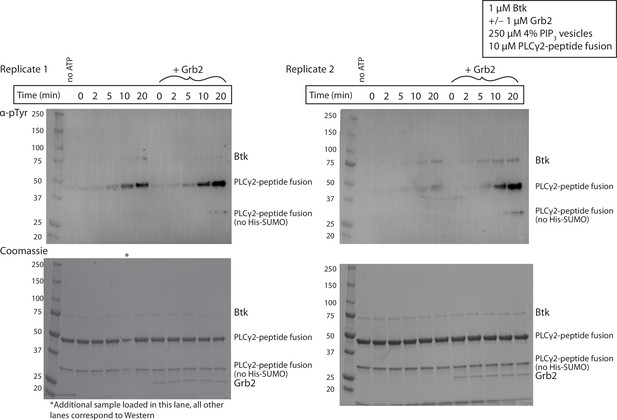
Grb2 enhances phosphorylation of PLCγ2 by Btk over time.
Entire blot from Figure 3C in replicate, looking at phosphorylation of PLCγ2-peptide fusion. 1 µM Btk was activated for the indicated amount of time in the presence of 250 µM lipids, single unilamellar vesicles containing 4% PIP3, 10 µM PLCγ2-peptide fusion with or without 1µM Grb2. Each experiment was carried out in at least duplicate.
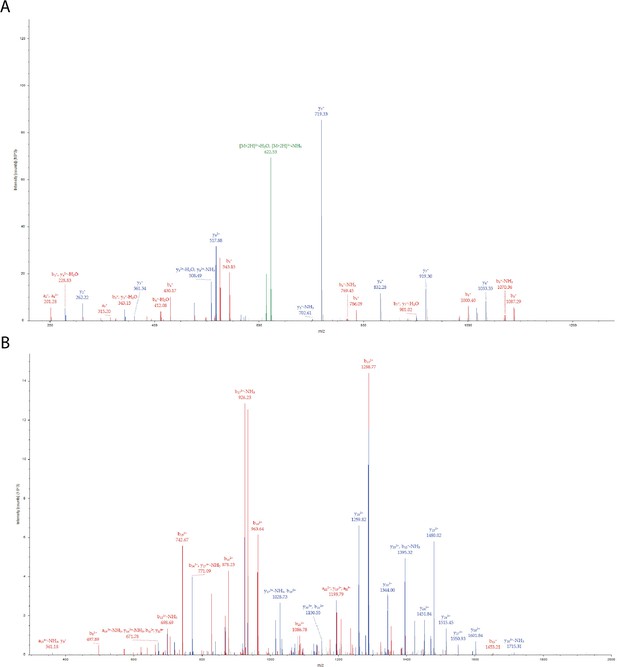
Phosphorylation of PLCγ2-peptide fusion Tyr corresponding to PLCγ2 Tyr 753 by Btk in the presence of Grb2.
(A) MS/MS spectrum for the precursor ion of m/z=631.2764, z=2 + , formed from a trypsin-digested sample of PLCγ2-peptide fusion incubated with Grb2 and Btk. The MS/MS spectrum identifies the precursor ion as the [M+2 H]2+ ion of the peptide, DINSL(pY)DVSR, corresponding to amino acid residues 376–385 of the PLCγ2-peptide fusion protein construct. The amino acid residue in position 381 of the PLCγ2-peptide fusion is identified as phosphotyrosine, this represents Tyr 753 in full-length PLCγ2. (B) MS/MS spectrum for the precursor ion of m/z=1111.0240,, z=4 + , formed from a trypsin-digested sample of His6-Sumo-eGFP-Plcg2 incubated with Grb2 and Btk. The MS/MS spectrum identifies the precursor ion as the [M+4 H]4+ ion of the peptide, RDHMVLLEFVTAAGITLGMDELYMGMERDINSL(pY)DVSR, corresponding to amino acid residues 348–385 of the PLCγ2-peptide fusion. The amino acid residue in position 381 is identified as phosphotyrosine, Tyr 753 in full-length PLCγ2. Each color represents a different type of ion in the tandem mass spectra, as follows: N-terminal fragment ions, that is, a and b-type ions (red), C-terminal fragment ions, that is, y-type ions (blue), precursor ion neutral losses (green). Fragment ions are labeled using standard nomenclature (Roepstorff and Fohlman, 1984).
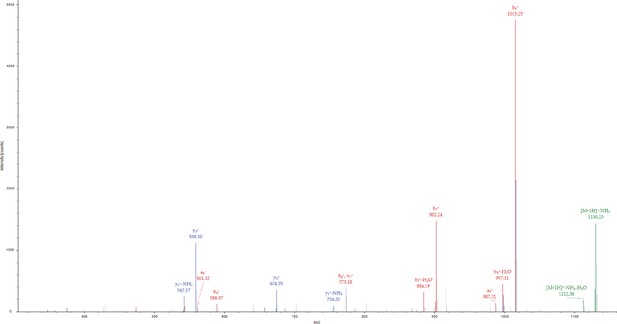
Phosphorylation of PLCγ2-peptide fusion Tyr corresponding to PLCγ2 Tyr 759 by Btk in the presence of Grb2.
MS/MS spectrum for the precursor ion of m/z=1147.4385, z=1 + , formed from a trypsin-digested sample of PLCγ2-peptide fusion incubated with Grb2 and Btk. The MS/MS spectrum identifies the precursor ion as the [M+H]+ ion of the peptide, M(pY)VDPSEIN, corresponding to amino acid residues 386–394 of the PLCγ2-peptide fusion. The amino acid residue in position 387 of the PLCγ2-peptide fusion is identified as phosphotyrosine, corresponding to Tyr 759 in full-length PLCγ2. Each color represents a different type of ion in the tandem mass spectra, as follows: N-terminal fragment ions (red), C-terminal fragment ions (blue), precursor ion neutral losses (green).
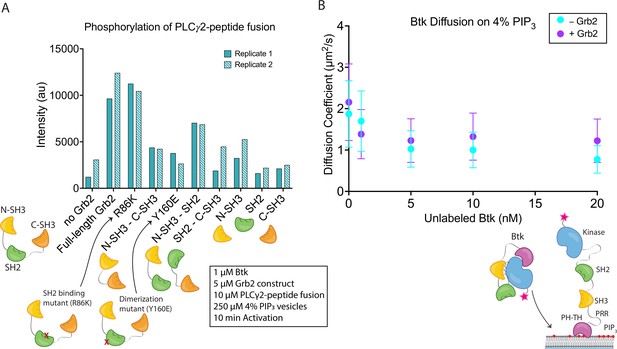
Effects of Grb2 on catalytic activity and membrane dynamics of Btk.
(A) Quantification of PLCγ2-peptide fusion phosphorylation measured from two independent experimental replicates. Phosphorylation of PLCγ2-peptide fusion by Btk in the presence of 4% PIP3 lipid vesicles was monitored in the presence of various Grb2 constructs. These constructs include: full length Grb2, R86K, N-terminal SH3 fused to C-terminal SH3, Y160E, N-terminal SH3-SH2, SH2-C-terminal SH3, N-terminal SH3, SH2, and C-terminal SH3. See Figure 4—figure supplement 1 and Figure 4—source data 1 for raw images. (B) Diffusion coefficients for membrane bound Btk were determined by single-molecule tracking, and fitting to step-size distributions for each concentration of unlabeled Btk, with or without Grb2. Error bars represent the standard deviation determined from the fit step-size distribution. See Dryad repository for source data for this panel, Figure 4B – source data (https://dx.doi.org/10.5061/dryad.jwstqjqfd).
-
Figure 4—source data 1
Raw images of gels and western membranes referenced in Figure 4A.
- https://cdn.elifesciences.org/articles/82676/elife-82676-fig4-data1-v1.zip
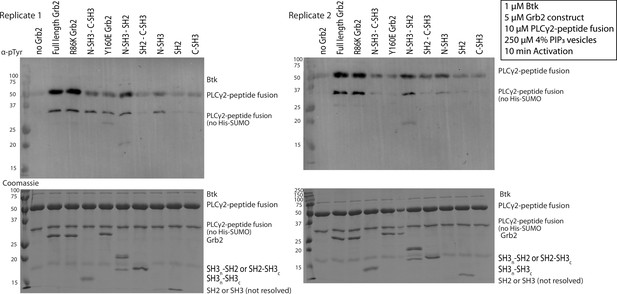
Phosphorylation of PLCγ2-peptide fusion by Btk with the addition of various Grb2 constructs.
Entire blot from Figure 4A and replicate, looking at phosphorylation of PLCγ2-peptide fusion in the presence of Btk and varying constructs of Grb2. 1 µM Btk was activated for 10 minutes in the presence of 250 µM lipids, in the form of single unilamellar vesicles containing 4% PIP3, 10 µM PLCγ2 peptide fusion with 10 µM of the indicated construct of Grb2. Each experiment was carried out in at least duplicate.
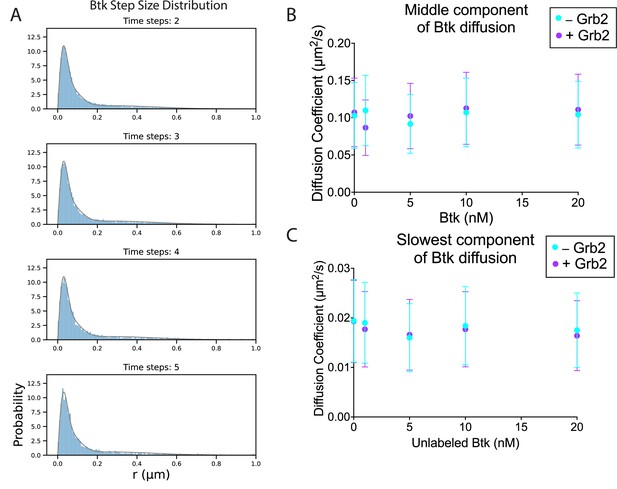
The diffusion of Btk on membranes in the absence and presence of Grb2.
(A) Example of the step size distribution, taken from 1 nM Btk-Cy5 alone (no unlabeled Btk added). Frame skips (denoted as ‘time steps’ here) were taken to ensure that no artifacts arise from selection of the frame rate or size of the camera pixels relative to step size of the molecules. The three-component diffusion is fit to the step size distributions across all selected time steps globally. According to Fick’s law of diffusion, the scale factor of a chi distribution fit to a step-size distribution will correspond to the diffusion coefficient of that population. In this case, the population was fit to a sum of three chi distributions, corresponding to three different diffusion coefficients represented within the population. This fitting procedure is detailed in Materials and Methods. The fastest diffusion coefficient as determined by this fitting method is included in the main text (Figure 4B). (B) The middle component of the fit, remaining relatively constant across Btk concentrations. (C) The slowest component of the fit, also remaining relatively constant with increasing surface density of Btk. See Dryad repository for source data for this figure. Error bars in (B) and (C) represent the standard deviation determined from the fit step-size distribution.
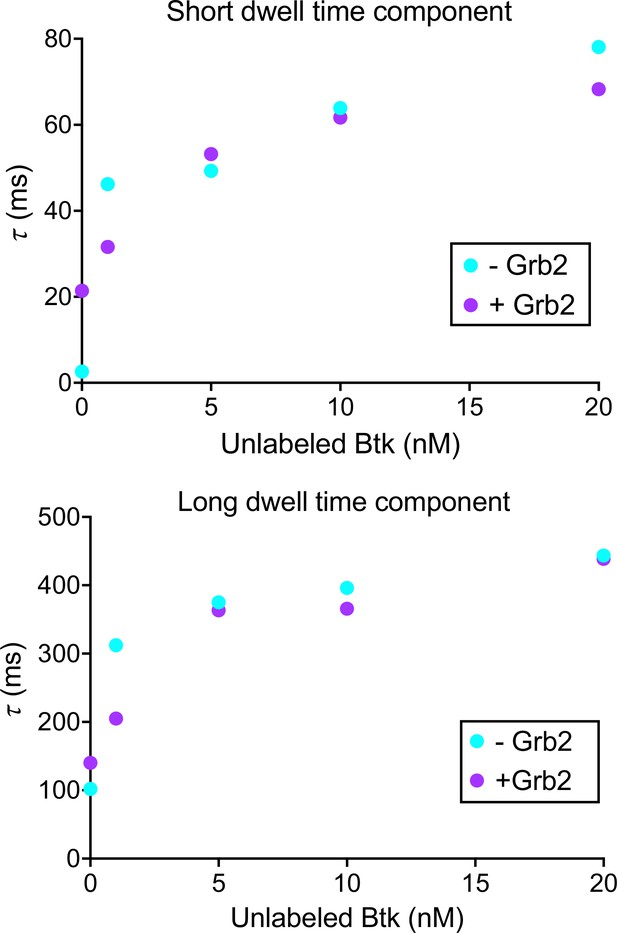
Btk dwell time confirms that Grb2 does not impact Btk membrane dynamics.
Dwell time was calculated by plotting a histogram of the length of tracks from the same data as the Figure 4—figure supplement 2. This histogram was then normalized to instead represent survival probabilities, which was then fit to the sum of two exponential decays. See Dryad repository for source data for this figure.
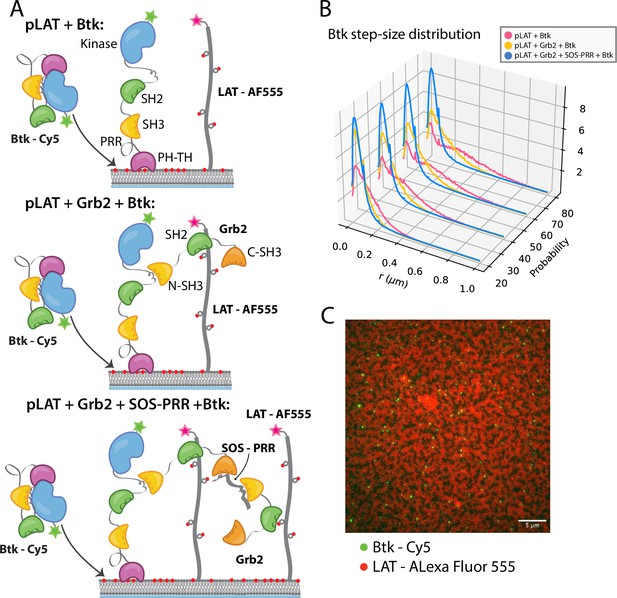
Btk can be recruited to scaffold proteins through interaction with Grb2.
(A) Cartoon schematic of predicted mechanism for Btk recruitment into LAT signaling clusters. (B) Step-size distribution for Btk-Cy5 under each condition: phospho-LAT, phospho-LAT + Grb2, phase transitioned LAT (phospho-LAT + Grb2 + SOS-PRR). The step-size distribution was calculated at multiple delay times. These delay times represent frame skips that were taken to ensure that no artifacts arise from selection of the frame rate or size of the camera pixels relative to step-size of the molecules. For each delay time, the dataset used to create the step-size distribution consists of frames from every 20 ms (every frame), 40 ms (every other frame), 60 ms, or 80 ms (every fourth frame). Six different positions across the bilayer were recorded for 500–600 frames each, and one independent experiment was used to confirm trends observed here. (C) Overlay of image of Btk-Cy5 (green) and LAT-Alexa Fluor 555 (red) after LAT phase transition. See Dryad repository for source data for this figure, Figure 5—source data 1 (https://dx.doi.org/10.5061/dryad.prr4xgxrf).
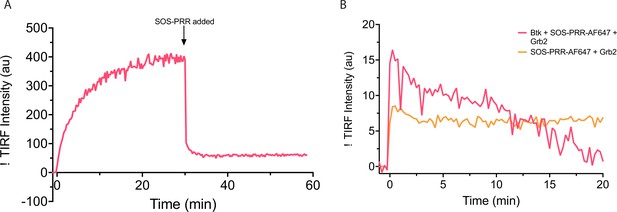
Behavior of Btk on PIP3-containing membranes with the addition of SOS proline rich region.
(A) Grb2-AlexaFluor647 and Btk were added to a PIP3-containing membrane at time 0, and AlexaFluor647 fluorescence intensity was measured. After 30 minutes SOS-PRR is added at 1.45 µM. SOS-PRR appears to compete with Btk for Grb2 binding. (B) Btk, Grb2, and SOS-PRR-AlexaFluor647 or Grb2 and SOS-PRR-AlexaFluor647 was added to PIP3 containing membrane and AlexaFluor647 fluorescence intensity was measured. SOS-PRR does not interact with Btk on supported-lipid bilayers. These data show that SOS-PRR is not recruited to Btk on supported-lipid bilayers.
Btk does not diffuse freely within phases that are dense in LAT.
One example video of data represented in Figure 5B of the pLAT + Grb2 + SOS-PRR + Btk condition after 1 hour incubation of all components. This video is an overlay of channels, one showing Btk (in green) and the other showing LAT (in red).
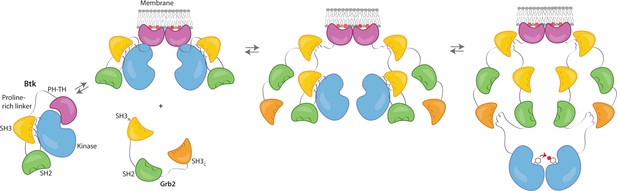
Grb2 enhances Btk activation at the membrane.
Our data are consistent with a model in which PIP3 binding at the membrane is not sufficient for full activation, and Btk is still able to maintain some autoinhibition after membrane recruitment. Upon recruitment of Grb2 to the proline-rich linker of Btk, the second Grb2 SH3 domain is able to bind the SH2-kinase linker of Btk and displace Btk’s SH3 domain, resulting in full release of autoinhibition. Here we are showing Grb2 as a monomer, however, as discussed, it is possible that Grb2 is binding Btk as a dimer as well.
Tables
Reagent type (species) or resource | Designation | Source or reference | Identifiers | Additional information |
---|---|---|---|---|
Recombinant DNA reagent | His6-TEV (Tobacco etch virus) site-Grb2 pET28 (plasmid) | This paper | Full-length, UniProt P62993 | |
Recombinant DNA reagent | Btk pET28 (plasmid) | This paper | Full-length, UniProt Q06187 | |
Recombinant DNA reagent | Btk SH3-SH2-kinase pET28 (plasmid) | This paper | Residues 212–659 | |
Recombinant DNA reagent | Btk SH2-Kinase pET28 (plasmid) | This paper | Residues 281–659 | |
Recombinant DNA reagent | Btk kinase pET28 (plasmid) | This paper | Residues 402–659 | |
Recombinant DNA reagent | Btk proline-rich linker pET28 (plasmid) | This paper | Residues 171–214 | |
Recombinant DNA reagent | PLCγ2-peptide fusion pET28 (plasmid) | This paper | PLCγ2 Residues 746–766 UniProt P16885, EGFP | |
Recombinant DNA reagent | His6-TEV site-Grb2 SH3N-SH2 pET28 (plasmid) | This paper | Residues 1–153 | |
Recombinant DNA reagent | His6-TEV site-Grb2 SH2-SH3C pET28 (plasmid) | This paper | Residues 60–217 | |
Recombinant DNA reagent | His6-TEV site-Grb2 SH3N-SH3C pET28 (plasmid) | This paper | Residues 1–59, 154–217 | |
Recombinant DNA reagent | His6-TEV site-Grb2 SH3N pET28 (plasmid) | This paper | Residues 1–59 | |
Recombinant DNA reagent | His6-TEV site-Grb2 SH2 pET28 (plasmid) | This paper | Residues 60–153 | |
Recombinant DNA reagent | His6-TEV site-Grb2 SH3C pET28 (plasmid) | This paper | Residues 154–217 | |
Recombinant DNA reagent | His6-TEV site-Grb2 R86K pET28 (plasmid) | This paper | Full-length, R86K | |
Recombinant DNA reagent | His6-TEV site-Grb2 Y160E pET28 (plasmid) | This paper | Full-length, Y160E | |
Recombinant DNA reagent | His6-SUMO-Btk T403AzF pET28 (plasmid) | This paper | Full-length, T403Amber STOP | |
Recombinant DNA reagent | His6-TEV site-LAT pET28 (plasmid) | This paper | Residues 252–520, UniProt P08631 | |
Recombinant DNA reagent | His6-TEV site-Hck pET28 (plasmid) | This paper | Residues 1051–1333, UniProt Q07889 | |
Recombinant DNA reagent | His6-TEV site-SOS-PRR pET28 (plasmid) | This paper | UniProt P15273 | |
Recombinant DNA reagent | YopH pCDFDuet (plasmid) | This paper | ||
Strain, strain background (Escherichia coli) | BL21 (DE3) | Thermo Fisher | C600003 | Chemically competent cells |
antibody | Anti-Phospho-Tyrosine mAb, pTyr-100 (Mouse monoclonal) | Cell Signaling | Cat#: 9411 | WB (1:2000) |
antibody | Anti-Mouse HRP linked antibody (Horse) | Cell Signaling | Cat#: 7076 | WB (1:5000) |
Other | 4-Azido-L-phenylalanine HCl | Amatek Chemical Co. | Cat#: 33173-53-4 | |
Other | dibenzocyclooctyl (DBCO)-Cy5 | Click Chemistry Tools | Cat#: A130 | |
Other | AlexFluor 647 C2 Maleimide | Thermo Fisher, | Cat#: A20347 | |
Other | AlexFluor 555 C2 Maleimide | Thermo Fisher | Cat#: A20346 | |
Other | PI(3,4,5)P3 di16 (ammonium salt) | Echelon Biosciences, Inc | Cat#: P-3916A | |
Other | 1,2-dioleoyl-sn-glycero-3-[(N-(5-amino-1-carboxypentyl)iminodiacetic acid)succinyl] (nickel salt) (DGS-NTA(Ni)) | Avanti Polar Lipids | Cat#: 790404 | |
Other | 18:1 1,2-dioleoyl-sin-glycero-3-phosphocholine (DOPC) | Avanti Polar Lipids | Cat#: 850375 | |
Software, algorithm | Trackmate | FIJI (Image J) | Version: 2.9.0/1.53t | |
Software, algorithm | Imtrack package | Groves lab (Darren McAffee, PhD) | https://github.com/lnocka/Single_molecule_tracking.git | |
Software, algorithm | multi_chi_global.py | This paper | https://github.com/lnocka/Single_molecule_tracking.git | |
Software, algorithm | analysis_overlay_3D_line.py | This paper | https://github.com/lnocka/Single_molecule_tracking.git |