Functional hierarchy among different Rab27 effectors involved in secretory granule exocytosis
Figures
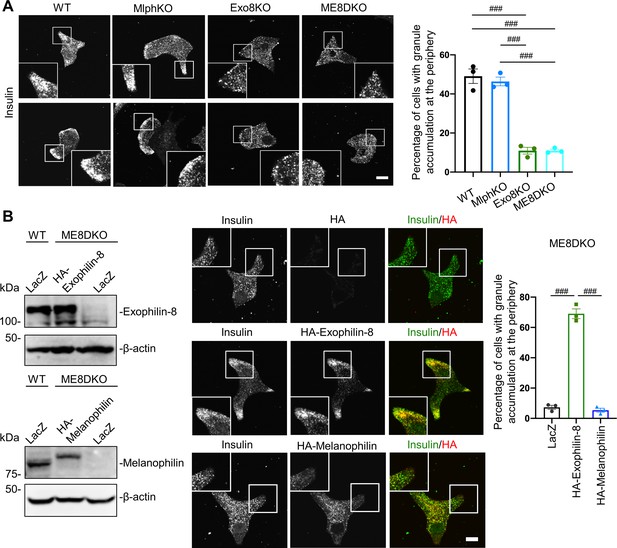
Exophilin-8, but not melanophilin, accumulates insulin granules in the action cortex.
(A) WT, MlphKO, Exo8KO, and ME8DKO beta cells were immunostained with anti-insulin antibody. A peripheral accumulation of insulin immunosignals was quantified under confocal microscopy: clustering of insulin immunosignals at least in one corner (upper) or along the plasma membrane (lower) were counted as positive. More than 100 cells were inspected in each of three independent experiments. Note that Exo8KO and ME8DKO cells do not show the peripheral accumulation of insulin granules in contrast to WT and MlphKO cells. (B) ME8DKO cells were infected by adenovirus expressing either HA-exophilin-8 or HA-melanophilin at the endogenous protein levels found in WT cells. LacZ was expressed in WT and ME8DKO cells as controls. The cell extracts were immunoblotted with the indicated antibodies (left). The ME8DKO cells expressing LacZ (upper), HA-exophilin-8 (middle), and HA-melanophilin (lower) were immunostained with anti-insulin and anti-HA antibodies (center), and a peripheral accumulation of insulin was quantified as in (A) (right). Insets represent higher magnification photomicrographs of a cell within the region outlined by frames. Note that expression of HA-exophilin-8, but not HA-melanophilin, rescues the peripheral granule accumulation in ME8DKO cells. Bars, 10 μm. ###p<0.001 by one-way ANOVA.
-
Figure 1—source data 1
Uncropped blot images of Figure 1B.
- https://cdn.elifesciences.org/articles/82821/elife-82821-fig1-data1-v2.zip
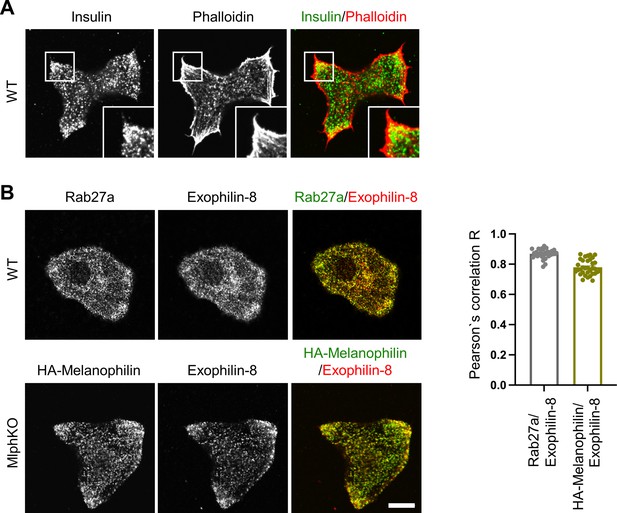
Melanophilin and exophilin-8 are colocalized on insulin granules accumulated in the actin-rich cell periphery.
(A) Primary beta cells from WT mice were double-stained with rhodamine-conjugated phalloidin and anti-insulin antibody, and were observed by confocal microscopy. (B) WT (upper) and MlphKO beta cells expressing HA-melanophilin at the endogenous level in WT cells (lower) were coimmunostained with goat anti-exophilin-8 and either anti-Rab27a or anti-HA antibodies. We investigated the intracellular distribution of HA-melanophilin expressed in MlphKO beta cells because there is no available anti-melanophilin antibody durable enough to stain endogenous melanophilin and because exogenous melanophilin expressed in the presence of endogenous melanophilin displays aberrant localization along F-actin (Wang et al., 2020). Colocalization between exophilin-8 and Rab27a in WT cells and that between exophilin-8 and HA-melanophilin in MlphKO cells were quantified by Pearson’s correlation coefficient (n = 30–37 from three mice each). Note that exophilin-8 and HA-melanophilin are accumulated and colocalized in the cell periphery. Bars, 10 μm.
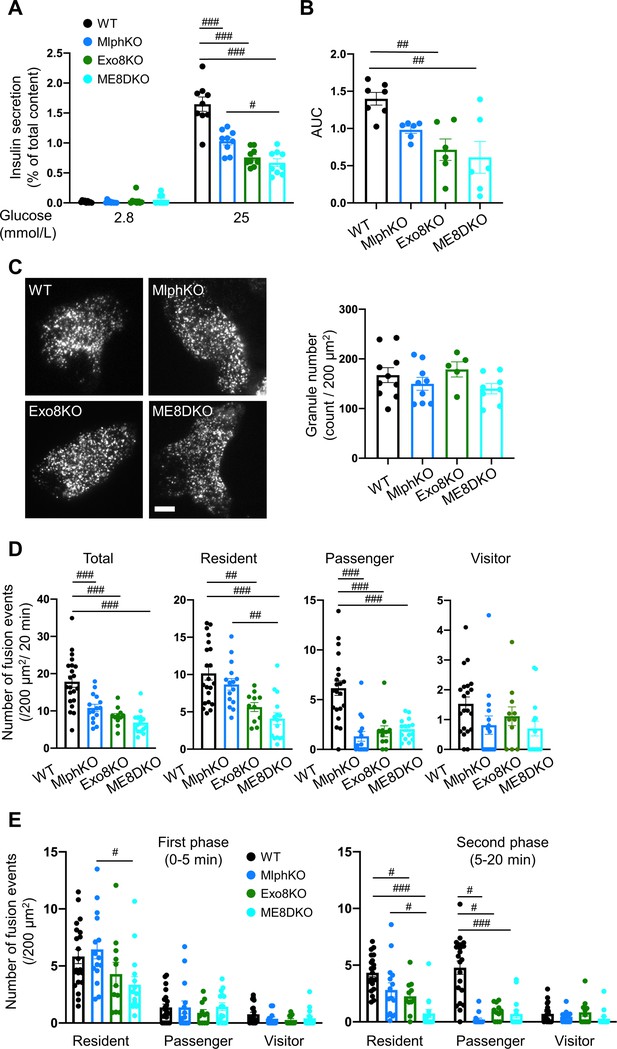
Insulin secretory defects of beta cells doubly deficient in melanophilin and exophilin-8 are indistinguishable from those singly deficient in exophilin-8.
(A) Islets isolated from WT, MlphKO, Exo8KO, and ME8DKO mice at 12–18 weeks of age were preincubated in 2.8 mmol/L low glucose (LG)-containing KRB buffer at 37℃ for 1 hr. They were then incubated in new LG buffer for 1 hr followed by 25 mmol/L high glucose (HG) buffer for 1 hr. The ratios of insulin secreted in the media to that left in the cell lysates (Figure 2—figure supplement 1B) are shown (left; n = 9 from three mice each). (B) Islets from age-matched mice (19- to 25-week-old) were perfused with 16.7 mmol/L glucose buffer for 30 min, and the ratios of insulin secreted in the media to that left in the cell lysates are plotted as in Figure 2—figure supplement 1C. The area under the curve (AUC) is shown (n = 9 from three mice each). (C–E) A monolayer of islet cells from the above four kinds of mice were infected with adenovirus encoding insulin-EGFP and were observed by total internal reflection fluorescence (TIRF) microscopy (C, left). The numbers of visible granules were manually counted for WT (n = 10 cells from three mice), MlphKO (n = 9 from three mice), Exo8KO (n = 5 cells from three mice), and ME8DKO (n = 8 cells from three mice) cells (C, right). Insulin granule fusion events in response to 25 mmol/L glucose for 20 min were counted as in Figure 2—figure supplement 1D for WT (n = 21 cells from five mice), MlphKO (n = 10 from three mice), Exo8KO (n = 12 cells from three mice), and ME8DKO (n = 12 cells from three mice) cells. The observed fusion events were categorized into three types: residents, visitors, and passengers (D). Summary of the three modes of fusion events (E) in the first phase (left, 0–5 min) and the second phase (right, 5–20 min). Note that the decrease in insulin exocytosis in ME8DKO cells is greater than that in MlphKO cells, specifically due to the decrease in the resident-type exocytosis. Bar, 10 μm. #p<0.05, ##p<0.01, ###p<0.001 by one-way ANOVA.
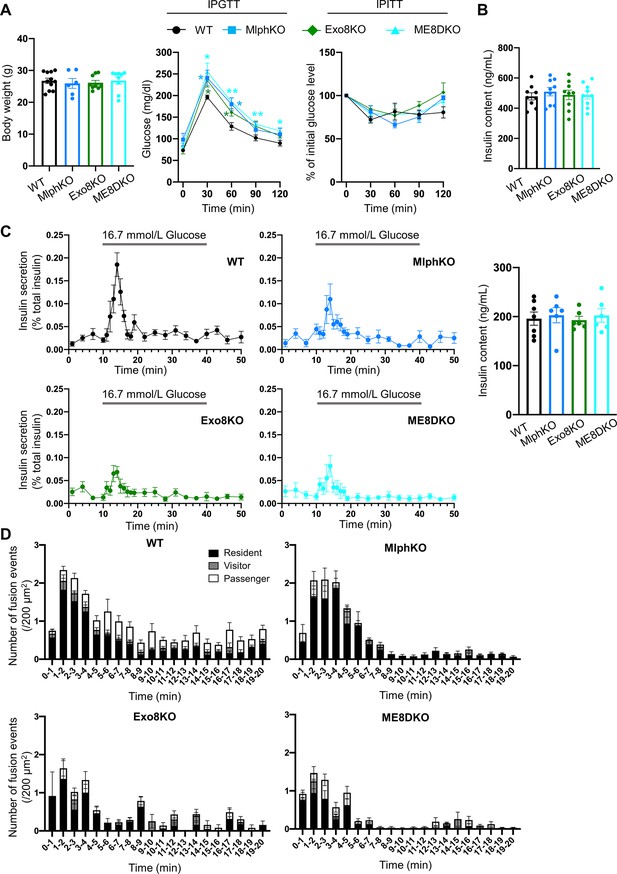
Phenotypic comparison among WT, MlphKO, Exo8KO, and ME8DKO mice.
(A) Body weight (left) of 20- to 22-week-old, WT (n = 11), MlphKO (n = 6), Exo8KO (n = 9), and ME8DKO (n = 9) mice, blood glucose concentrations during an intraperitoneal glucose tolerance test (IPGTT; middle) of 17–28-week-old mice (n = 5 each), and percentage of starting blood glucose concentration during an intraperitoneal insulin tolerance test (IPITT, right) of 18–24-week-old mice (n = 4–5 each). (B) Insulin levels left in the cell lysates in the experiments of Figure 2A. (C) Islets from age-matched mice (19- to 25-week-old) were perfused with 2.8 mmol/L glucose buffer 1 hr and then with 16.7 mmol/L glucose buffer for 30 min with pre- and post-incubation of 2.8 mmol/L glucose buffer for 10 min. Insulin levels secreted in buffer and left in the cell lysates (right) were measured (left; n = 6–7 from 4 to 5 mice each), and their ratios are shown (left; n = 9 from three mice each). (D) The histograms show the average numbers of fusion events per 200 mm2 at 1 min intervals in the experiments of Figure 2D. *p<0.05, **p<0.01 by Student’s t test.
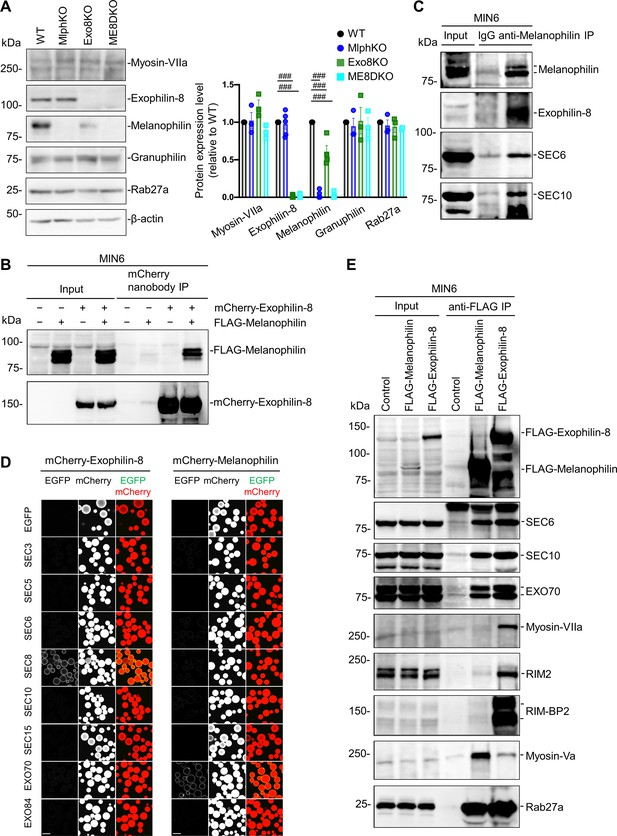
Exophilin-8 and melanophilin interact through the exocyst in beta cells.
(A) Islet extracts (40 μg) from WT, MlphKO, Exo8KO, and ME8DKO mice were immunoblotted with antibodies toward the indicated proteins (left). Protein expression levels were quantified by densitometric analyses from 3 to 5 independent preparations (right). (B) MIN6 cells were infected with adenoviruses encoding control LacZ, mCherry-exophilin-8, and/or FLAG-melanophilin. After 2 days, the cell extracts underwent immunoprecipitation with mCherry nanobody. The immunoprecipitates (IP), as well as the 1% extracts (Input), were immunoblotted with anti-FLAG and anti-red fluorescent protein (RFP) antibodies. (C) MIN6 cell extracts were immunoprecipitated with rabbit anti-melanophilin antibody or control IgG, and the immunoprecipitates were immunoblotted with antibodies toward the indicated proteins. (D) HEK293A cells cultured in 10 cm dishes were transfected with mCherry-fused, exophilin-8 (left) or melanophilin (right) with the indicated EGFP-fused exocyst components. After 48 hr, the cell lysates were subjected to immunoprecipitation with mCherry-nanobody-bound glutathione-Sepharose beads. EGFP and mCherry fluorescence on the precipitated beads was observed by confocal microscopy. (E) MIN6 cells expressing FLAG-tagged, melanophilin or exophlin-8 were immunoprecipitated with anti-FLAG antibody, and the immunoprecipitates were immunoblotted with antibodies toward the indicated proteins. Note that the exocyst complex components exist in both immunoprecipitates, whereas RIM-BP2, RIM2, myosin-VIIa, and myosin-Va largely exist only in one of them. Bars, 100 μm. ###p<0.001 by one-way ANOVA.
-
Figure 3—source data 1
Uncropped blot images of Figure 3A, B, C and E.
- https://cdn.elifesciences.org/articles/82821/elife-82821-fig3-data1-v2.zip
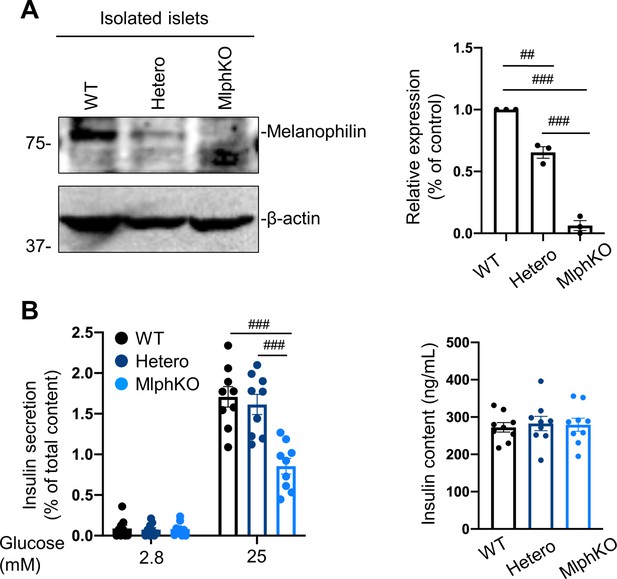
Heterozygous MlphKO cells do not decrease insulin secretion despite the reduced melanophilin expression.
(A) Islet extracts (30 μg) from WT, heterozygous and homozygous MlphKO mice were immunoblotted with antibodies toward the indicated proteins (left). Protein expression levels were quantified by densitometric analyses from three independent preparations (right). (B) Islets isolated from the mice at 8–12 weeks of age were subjected to insulin secretion assays as described in Figure 2A. Insulin levels secreted in the media and left in the cell lysates were measured (right), and their ratios are shown (left; n = 9 from three mice each). ##p<0.01, ###p<0.001 by one-way ANOVA.
-
Figure 3—figure supplement 1—source data 1
Uncropped blot images of Figure 3—figure supplement 1A.
- https://cdn.elifesciences.org/articles/82821/elife-82821-fig3-figsupp1-data1-v2.zip
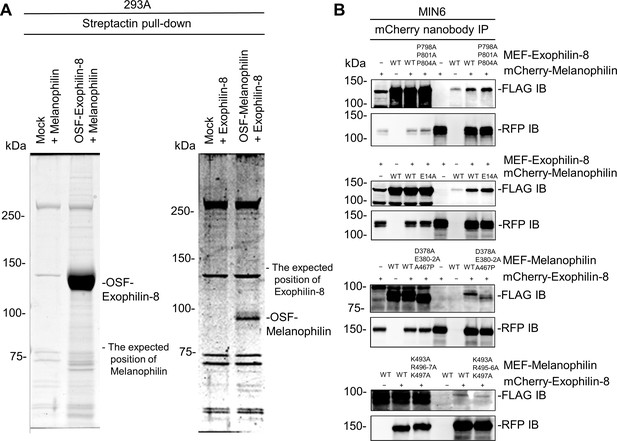
Exophilin-8 does not bind melanophilin directly or through the known interacting proteins.
(A) Melanophilin and OSF-tagged exophilin-8 (left), or exophilin-8 and OSF-melanophilin (right) were coexpressed by transfection in HEK293A cells. The cell extracts were pulled down using Strep-Tactin beads, and were subjected to gel electrophoresis followed by gel staining with Coomassie Brilliant Blue. Note that exogenously expressed exophilin-8 and melanophilin do not interact in HEK293A cells. (B) MEF-tagged, WT exophilin-8 and its P798A/P801A/P804A mutant that loses binding activity toward RIM-BP2 were expressed with or without mCherry-melanophilin by adenoviruses in MIN6 cells (top panel). Similarly, mCherry-melanophilin WT or its E14A mutant that loses binding activity toward Rab27a were coexpressed with or without MEF-exophilin-8 (second panel), whereas MEF-melanophilin D378A/E380A/E381A/E382A/A467P and K493A/R495A/R496A/K497A mutants that lose binding activity toward myosin-Va and actin, respectively, were coexpressed with or without mCherry-exophilin-8 (third and bottom panels). The cell extracts underwent immunoprecipitation with anti-mCherry nanobody, and the immunoprecipitates were immunoblotted with anti-FLAG and anti-RFP antibodies. Note that all the exophilin-8 and melanophilin mutants preserved the interaction with WT melanophilin and exophilin-8, respectively.
-
Figure 3—figure supplement 2—source data 1
Uncropped blot images of Figure 3—figure supplement 2B.
- https://cdn.elifesciences.org/articles/82821/elife-82821-fig3-figsupp2-data1-v2.zip
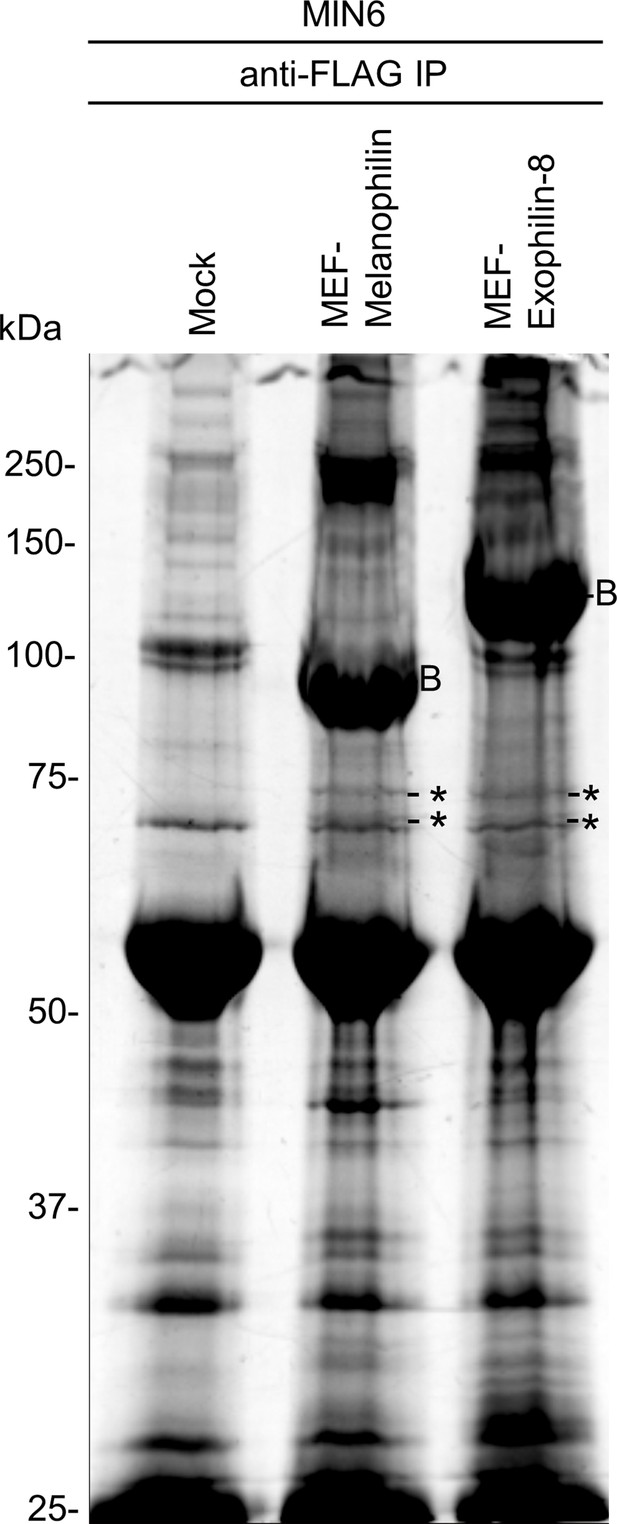
Analysis of the melanophilin and exophilin-8 protein complexes.
MIN6 cells were infected by adenoviruses encoding no protein (Mock), MEF-tagged melanophilin or exophilin-8. The binding proteins were pulled down using FLAG beads and were subjected to gel electrophoresis followed by Oriole fluorescent gel staining. The specific protein bands were cut and digested for LC-MS/MS. Asterisks and label B: bands containing the exocyst complex components and bait protein, respectively.
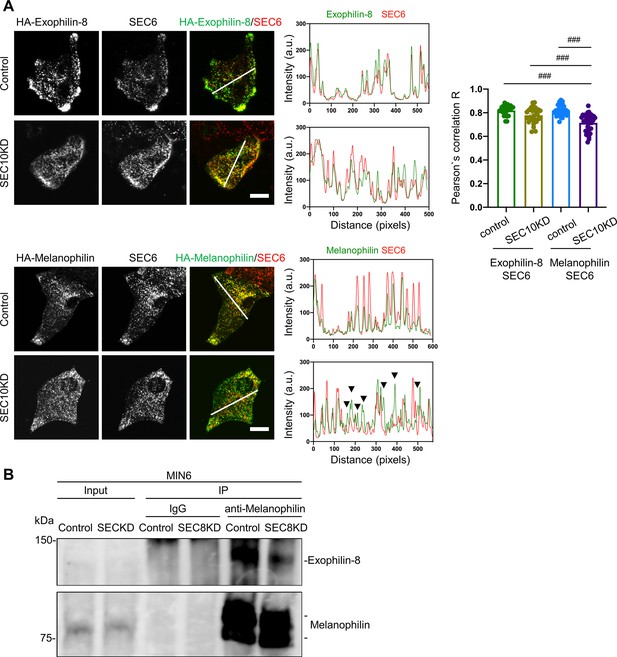
Knockdown of the exocyst component disrupts the interaction between melanophilin and exophilin-8.
(A) HA-exophilin-8 and HA-melanophilin were expressed in Exo8KO and MlphKO monolayer beta cells, respectively, at the endogenous levels in WT cells as described in Figure 1B. They were then transfected with control siRNA or siRNA against SEC10 #11 or #12, as shown in Figure 4—figure supplement 1A. After fixation, the cells were coimmunostained with anti-HA and anti-SEC6 antibodies and were observed by confocal microscopy (left). Fluorescent intensity profiles along the indicated line of SEC6 and either HA-exophilin-8 or HA-melanophilin are shown (center). Colocalization was quantified by Pearson’s correlation coefficient (right, n = 18–26 cells from two mice each). Note that SEC10 knockdown (KD) induces dissociation of melanophilin, but not exophilin-8, from SEC6 (black arrowheads). (B) MIN6 cells were transfected with control siRNA or siRNA against SEC8 #12, as shown in Figure 4—figure supplement 1A, and the cell extracts were subjected to immunoprecipitation with control IgG or anti-melanophilin antibody. The immunoprecipitates (IP), as well as the 1% extracts (Input), were immunoblotted with anti-exophilin-8 and anti-melanophilin antibodies. Bars, 10 μm. ###p<0.001 by one-way ANOVA.
-
Figure 4—source data 1
Uncropped blot images of Figure 4B.
- https://cdn.elifesciences.org/articles/82821/elife-82821-fig4-data1-v2.zip
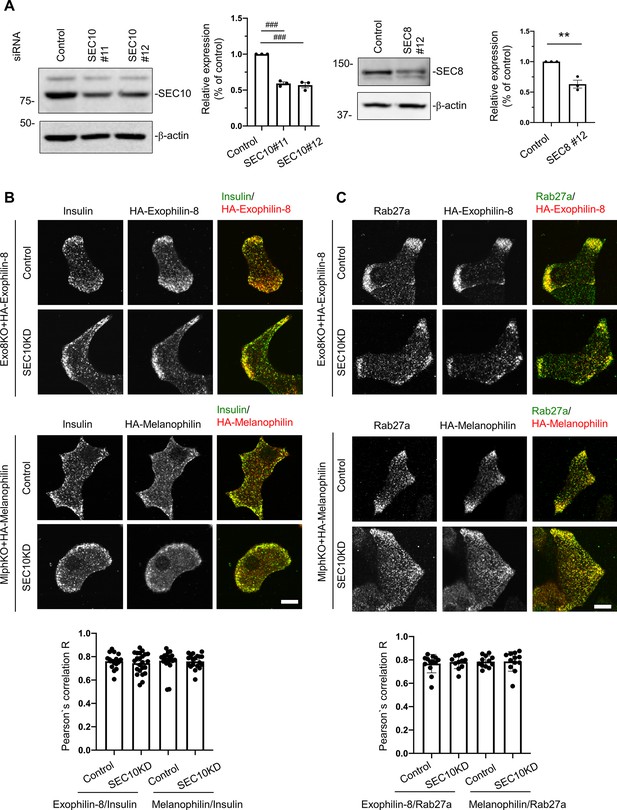
Knockdown of the exocyst component does not affect the granule localization of exophilin-8 or melanophilin.
(A) Pancreatic islet cells from WT mice were suspended in 1 × 105 cells/280 μl, and were transfected with 100 nmol/L control siRNA or siRNAs against SEC10 #11 or #12 (left) or SEC8#12 (right). After being plated on the 24-well plate for 48 hr, the cells were transfected with siRNAs for the second time. After another 48 hr, the cell extracts were immunoblotted with antibodies against SEC10, SEC8, and β-actin. Protein bands were quantified by densitometric analyses from three independent preparations. (B, C) Exo8KO or MlphKO beta cells expressing HA-exophilin-8 or HA-melanophilin were transfected with control siRNA or siRNA against SEC10. After fixation, the cells were coimmunostained with anti-HA antibody and either anti-insulin (B, n = 16–23 cells from two mice each) or anti-Rab27a antibody (C, n = 11–13 cells from two mice each), and were observed by confocal microscopy. Colocalization was quantified by Pearson’s correlation coefficient (bottom). Bars, 10 μm. ###p<0.001 by one-way ANOVA. **p<0.01 by Student’s t test.
-
Figure 4—figure supplement 1—source data 1
Uncropped blot images of Figure 4—figure supplement 1A.
- https://cdn.elifesciences.org/articles/82821/elife-82821-fig4-figsupp1-data1-v2.zip
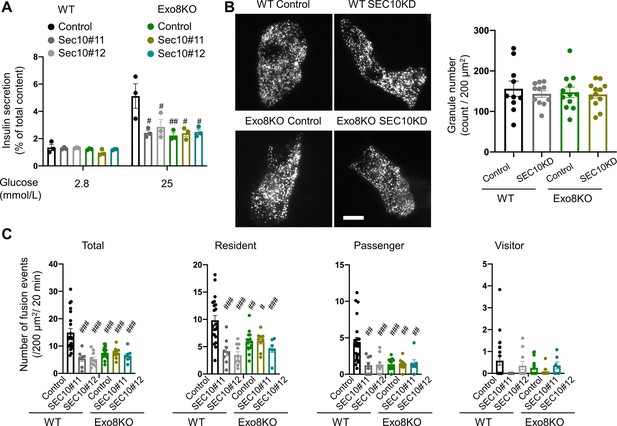
The exocyst affects insulin granule exocytosis only in the presence of exophilin-8.
(A–C) WT or Exo8KO mouse islet cells were twice transfected with control siRNA or siRNA against SEC10 #11 or #12, as shown in Figure 4—figure supplement 1A, and were plated in a 24-well plate (A) or glass base dish (B, C). (A) The transfected monolayer cells were incubated for 1 hr in KRB buffer containing 2.8 mmol/L glucose and were then stimulated for 1 hr in the same buffer or in buffer containing 25 mmol/L glucose. Insulin levels secreted in the media and left in the cell lysates were measured (Figure 5—figure supplement 1), and their ratios are shown (n = 3 from three mice each). (B) The control or SEC10 knockdown (KD) cells (control siRNA-treated WT cells, n = 10 from three mice; SEC10 siRNA#11 or #12-treated WT cells, n = 11 from three mice; control siRNA-treated Exo8KO cells, n = 12 from three mice; SEC10 siRNA#11 or #12-treated Exo8KO cells, n = 12 from three mice) were infected with adenovirus encoding insulin-EGFP and were observed by total internal reflection fluorescence (TIRF) microscopy (left). The numbers of visible granules were manually counted (right). (C) The transfected cells (control siRNA-treated WT cells, n = 22 from three mice; SEC10 siRNA#11-treated WT cells, n = 8 from three mice; SEC10 siRNA#12-treated WT cells, n = 10 from three mice; control siRNA-treated Exo8KO cells, n = 15 from three mice; SEC10 siRNA#11-treated Exo8KO cells, n = 9 from three mice; SEC10 siRNA#12-treated Exo8KO cells, n = 7 from three mice) were infected with adenovirus encoding insulin-EGFP, and fusion events in response to 25 mmol/L glucose for 20 min were counted and categorized under TIRF microscopy as described in Figure 2D. Bar, 10 μm. #p<0.05, ##p<0.01, ###p<0.001 by one-way ANOVA versus control siRNA-treated WT cells.
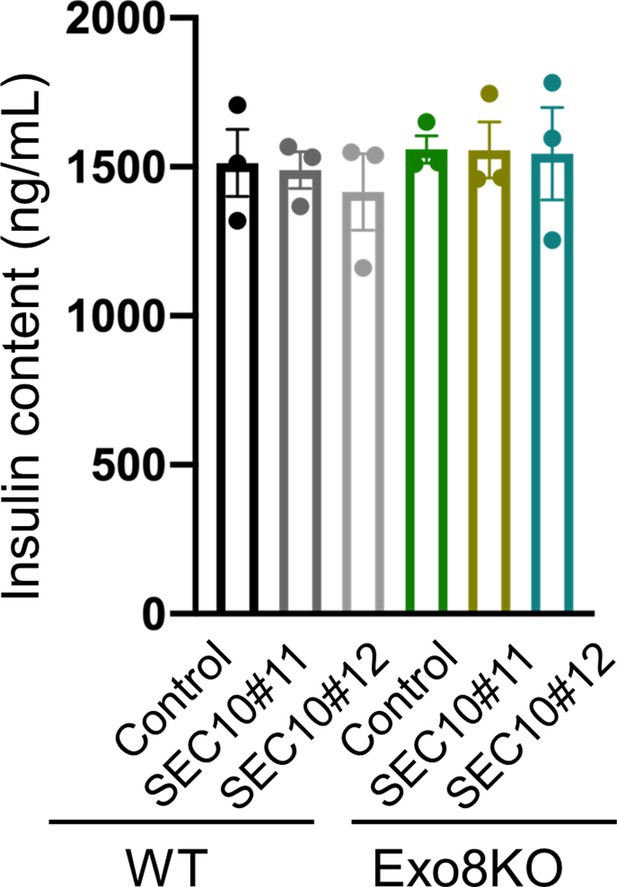
Insulin levels left in the cell lysates in batch insulin secretion assays.
Insulin levels left in the cell lysates in the experiments of Figure 5A.
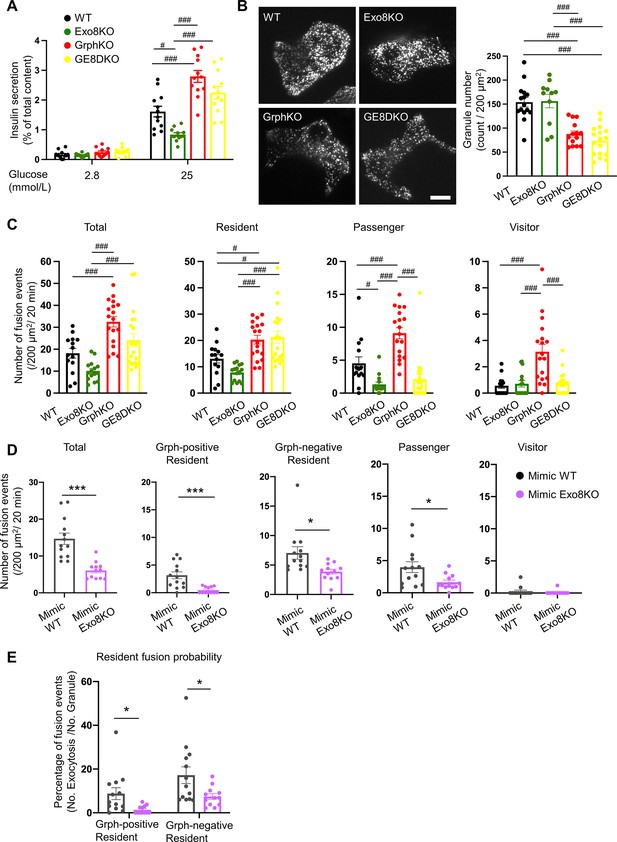
Exophilin-8 deficiency strongly inhibits the resident-type exocytosis from granuphilin-positive, stably docked granules.
(A) Islets isolated from WT, Exo8KO, GrphKO, and GE8DKO mice at 12–17 weeks of ages were preincubated in low glucose (LG) KRB buffer for 1 hr. They were then incubated in another LG buffer for 30 min followed by high glucose (HG) buffer for 30 min. The ratios of insulin secreted in the media of that left in the cell lysates (Figure 6—figure supplement 1A) are shown as in Figure 2A (n = 12 from four mice each). (B) A monolayer of islet cells from the above four kinds of mice were infected with adenovirus encoding insulin-EGFP and were observed by total internal reflection fluorescence (TIRF) microscopy (left). The numbers of visible granules were manually counted for WT (n = 15 cells from four mice), Exo8KO (n = 11 cells from three mice), GrphKO (n = 14 cells from four mice), and GE8DKO (n = 17 cells from four mice) cells (right). (C) Fusion events in response to 25 mmol/L glucose for 20 min were counted and categorized as described in Figure 2D (WT: n = 14 cells from three mice; Exo8KO: n = 15 cells from three mice; GrphKO: n = 18 cells from three mice; GE8DKO: n = 20 cells from three mice). Note that the increases in the passenger and visitor types in GrphKO cells were eliminated by the simultaneous absence of exophilin-8 in GE8DKO cells, whereas the increase in the resident type was completely unaffected at all. (D, E) A monolayer of GrphKO (n = 13) and GE8DKO (n = 12) islet cells from three mice each were infected by adenoviruses encoding insulin-EGFP and KuO-granuphilin to mimic WT and Exo8KO cells, respectively, as described in Figure 6—figure supplement 1B. Insulin granule fusion events in response to 25 mmol/L glucose for 20 min were counted and categorized under TIRF microscopy as in Figure 6C, except that the granuphilin-positive and -negative granules were distinguished (D). There were no granuphilin-positive granules showing either passenger or visitor-type exocytosis. The fusion probability of granuphilin-positive and -negative granules is shown as the percentage of those granules displaying the resident-type exocytosis (E). Bar, 10 μm. #p<0.05, ###p<0.001 by one-way ANOVA. *p<0.05, *** p<0.001 by Student’s t test.
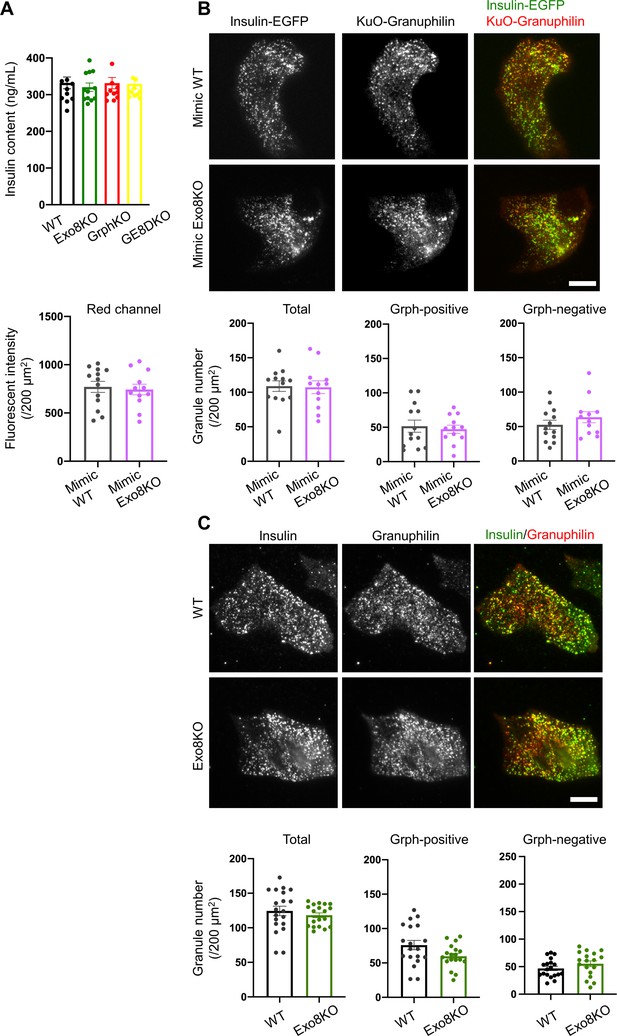
Visible insulin granules associated with granuphilin under total internal reflection fluorescence (TIRF) microscopy.
(A) Insulin levels left in the cell lysates in the experiments of Figure 6A. (B) A monolayer of GrphKO (n = 13) and GE8DKO (n = 12) islet cells from three mice each was infected by adenoviruses encoding insulin-EGFP and KuO-granuphilin to mimic WT and Exo8KO cells, respectively. These cells were observed by TIRF microscopy, and the fluorescent intensities of the red channel were measured using NIS Elements Viewer software to confirm similar expression levels of KuO-granuphilin. The numbers of total, KuO-granuphilin-positive and -negative, visible granules were counted under TIRF microscopy and were compared by Student’s t test, as in Figure 6B. (C) A monolayer of WT (n = 19) and Exo8KO (n = 18) islet cells from three mice each was immunostained with anti-insulin and anti-granuphilin antibodies and was observed by TIRF microscopy. Total and granuphilin-positive granules were counted and compared as in (B). Bars, 10 μm.
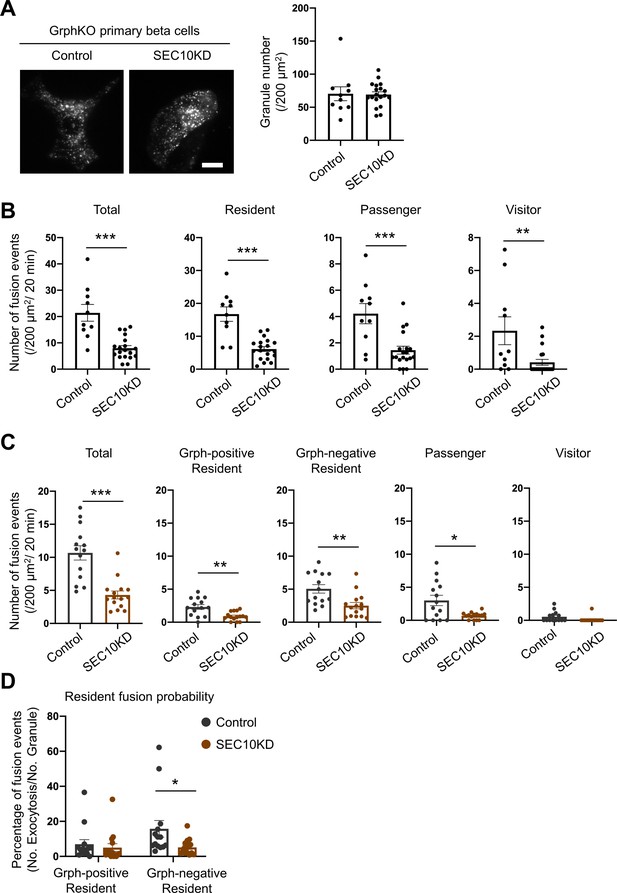
Exocyst deficiency strongly inhibits the resident-type exocytosis from granuphilin-negative, untethered granules.
(A) GrphKO beta cells were transfected with control siRNA (n = 10 from three mice) or siRNA against SEC10 #11 or #12 (n = 19 from three mice) were infected with adenovirus encoding insulin-EGFP as described in Figure 5B. They were observed by total internal reflection fluorescence (TIRF) microscopy (left), and numbers of visible granules were manually counted (right). (B) The control (n = 10 from three mice) or SEC10 knockdown (KD) cells (n = 19 from three mice) were infected with adenovirus encoding insulin-EGFP, and fusion events in response to 25 mmol/L glucose for 20 min were counted and categorized under TIRF microscopy as described in Figure 6C. Note that in contrast to exophilin-8 knockout (Figure 6C), SEC10 knockdown suppresses the resident-type exocytosis in GrphKO cells. (C, D) GrphKO islet cells from three mice were transfected with control (n = 14) or SEC10 siRNAs (n = 15) twice, and were infected with adenoviruses encoding insulin-EGFP and KuO-granuphilin on the next day. Because these cells that had undergone two times siRNA transfection and two kinds of adenovirus infection failed to respond to 25 mmol/L glucose stimulation well, they were depolarized by 60 mmol/L potassium. Fusion events during 6 min were counted and categorized (C) as in Figure 6D. The fusion probability of granuphilin-positive and -negative granules is shown (D) as in Figure 6E. Note that SEC10 knockdown selectively suppresses it from granuphilin-negative granules. Bar, 10 μm. *p<0.05, **p<0.01, ***p<0.001 by Student t test.
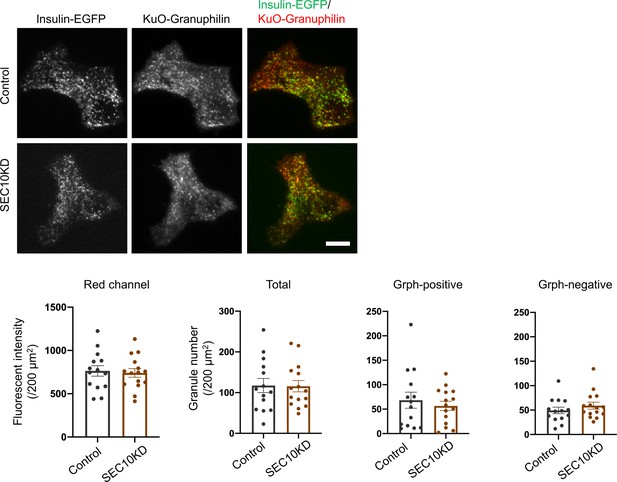
Visible insulin granules associated with granuphilin under total internal reflection fluorescence (TIRF) microscopy.
GrphKO islet cells from three mice were transfected with control (n = 14) or SEC10 siRNAs (n = 15) twice as described in Figure 7A. In the next day, these cells were infected with adenoviruses encoding insulin-EGFP and KuO-granuphilin and were observed by TIRF microscopy (upper). The fluorescent intensities of the red channel and the numbers of total, KuO-granuphilin-positive and -negative, visible granules were counted and compared as in Figure 6—figure supplement 1B. Bar, 10 μm.
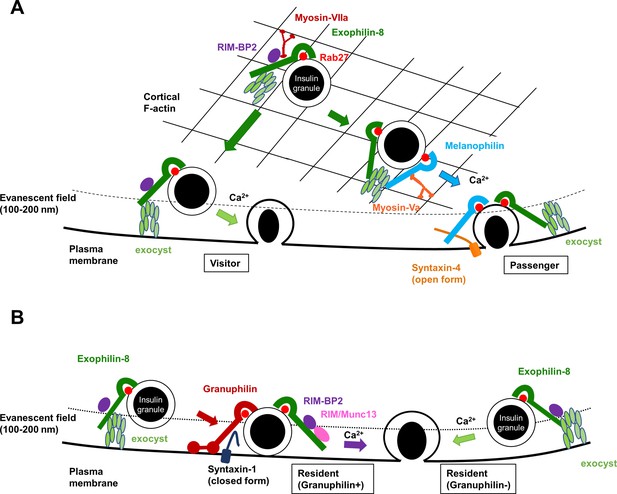
A schematic model for the functional relationship among different Rab27 effectors and the exocyst in insulin granule exocytosis.
At least, four different types of insulin granule exocytosis are discriminated by total internal reflection fluorescence (TIRF) microscopic analyses: the passenger type and the visitor type derived from granules anchored in the actin cortex (A), and the resident type from granules docked to the plasma membrane or from granules that remain untethered beneath the plasma membrane (B). Exophilin-8, melanophilin, granuphilin, and the exocyst differentially regulate each type of exocytosis. See details in ‘Discussion.’
Tables
Reagent type (species) or resource | Designation | Source or reference | Identifiers | Additional information |
---|---|---|---|---|
Gene (Mus musculus) | MyRIP (Exophilin-8) | GenBank | Gene ID: 245049 | |
Gene (M. musculus) | Mlph (Melanophilin) | GenBank | Gene ID: 171531 | |
Gene (M. musculus) | Sytl4 (Granuphilin) | GenBank | Gene ID: 27359 | |
Cell line (Homo sapiens) | HEK293A | Invitrogen | Cat#: R70507; RRID: CVCL_6910 | The cell line has been authenticated and tested negative for mycoplasma |
Cell line (M. musculus) | Insulinoma | Miyazaki et al., 1990 | RRID:CVCL_0431 | The cell line has been authenticated and tested negative for mycoplasma |
Transfected construct (M. musculus) | On-Target plus non-targeting pool siRNA | Horizon Discovery Ltd | Cat#: D-001810-10-05 | Silencer Select |
Transfected construct (M. musculus) | siRNA to Exoc5 (SEC10) | Horizon Discovery Ltd | Cat#: 105504 | On-Target plus Set of 4 siRNA (J-047583-11 and -12) |
Transfected construct (M. musculus) | siRNA to Exoc4 (SEC8) | Horizon Discovery Ltd | Cat#: 20336 | On-Target plus Set of 4 siRNA (J-051541-12-0050) |
Biological sample (M. musculus) | Primary pancreatic beta cells | This paper | Freshly isolated islets from male mouse pancreas | |
Antibody | Anti-Rab27a/b (rabbit polyclonal) | IBL | Cat#: 18975; RRID:AB_494635 | WB (1:2000) |
Antibody | Anti-Rab27a (mouse monoclonal) | BD Biosciences | Cat#: 558532; RRID: AB_647327 | IF (1:100) |
Antibody | Anti-Exophilin-8 (goat polyclonal) | Abcam | Cat#: ab10149; RRID:AB_296882 | IF (1:100) |
Antibody | Anti-Melanophilin (goat polyclonal) | Everest Biotech | Cat#: EB05444; RRID:AB_2146092 | WB (1:2000) |
Antibody | Anti-Melanophilin (rabbit polyclonal) | Proteintech | Cat#: 10338-1-AP; RRID: AB_2146104 | WB (1:2000) |
Antibody | Anti-Myosin-VIIa (rabbit polyclonal) | Abcam | Cat#: ab3481; RRID: AB_303841 | WB (1:2000) |
Antibody | Anti-Myosin-Va (rabbit polyclonal) | Cell Signaling Technology | Cat#: 3402; RRID: AB_2148475 | WB (1:2000) |
Antibody | Anti-RIM-BP2 (rabbit polyclonal) | Proteintech | Cat#: 15716-1-AP; RRID:AB_2878173 | WB (1:2000) |
Antibody | Anti-RIM1/2 (rabbit polyclonal) | Synaptic Systems | Cat#: 140213; RRID: AB_2832237 | WB (1:2000) |
Antibody | Anti-SEC6 (mouse monoclonal) | Assay Designs | Cat#: ADI-VAM-SV021; RRID: AB_10618264 | IF (1:100), WB (1:2000) |
Antibody | Anti-SEC8 (rabbit polyclonal) | Proteintech | Cat#: 11913-1-AP; RRID: AB_2101565 | WB (1:2000) |
Antibody | Anti-SEC10 (rabbit polyclonal) | Proteintech | Cat#: 17593-1-AP; RRID: AB_2101582 | WB (1:2000) |
Antibody | Anti-EXO70 (rabbit polyclonal) | Proteintech | Cat#: 12014-1-AP; RRID: AB_2101698 | WB (1:2000) |
Antibody | Anti-FLAG (rabbit polyclonal) | Sigma-Aldrich | Cat#: F7425; RRID: AB_439687 | WB (1:2000) |
Antibody | Anti-HA (rabbit polyclonal) | MBL | Cat#: 561; RRID: AB_591839 | WB (1:2000) |
Antibody | Anti-HA (rat monoclonal) | Roche | Cat#: 11867423001; RRID: AB_390918 | IF (1:100) |
Antibody | Anti-β-actin (mouse monoclonal) | Sigma-Aldrich | Cat#: A5316; RRID: AB_476743 | WB (1:10,000) |
Antibody | Anti-red fluorescent protein (RFP) (rabbit polyclonal) | MBL | Cat#: PM005; RRID:AB_591279 | WB (1:10,000) |
Commercial assay or kit | AlphaLISA insulin kit | PerkinElmer | Cat#: AL350HV/C/F | Insulin detection |
Commercial assay or kit | Insulin high range kit | Cisbio | Cat#: 62IN1PEG | Insulin detection |
Commercial assay or kit | Insulin ultra sensitive kit | Cisbio | Cat#: 62IN2PEH | Insulin detection |
Recombinant DNA reagent | pEGFP-C3 (plasmid) | Addgene | Cat#: 53755-53762; RRID: Addgene_53755–53762 | GFP version of SEC3, SEC5, SEC6, SEC8, SEC10, SEC15, EXO70, and EXO84 |
Software, algorithm | ImageQuant TL software | Cytiva | RRID:SCR_014246 | Quantify immunoblotting |
Software, algorithm | NIS Element Viewer | Nikon | RRID:SCR_014329 | Quantify colocalization and analyze fusion events |
Software, algorithm | Protein pilot software | SCIEX | RRID:SCR_018681 | Mass spectrometry |
Other | Rhodamine-conjugated phalloidin | Thermo Fisher Scientific | Cat#: R415 | IF (1:100) |
Other | Anti-FLAG M2 Affinity Gel | Sigma-Aldrich | Cat#: A2220 | IP |
Other | Protein G Sepharose 4 Fast Flow | GE Healthcare Biosciences | Cat#: GE17-0618-01 | IP |
Other | Lipofectamine 3000 | Invitrogen | Cat#: L3000001 | Transfection |
Other | Lipofectamine RNAiMax | Invitrogen | Cat#: 13778075 | Transfection |