Microtubule-mediated GLUT4 trafficking is disrupted in insulin-resistant skeletal muscle
Figures
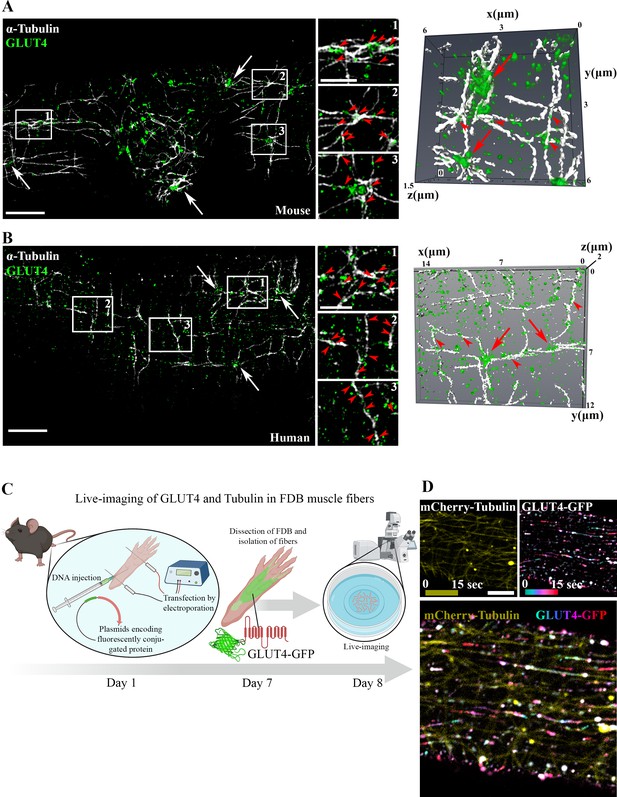
Glucose transporter 4 (GLUT4) was enriched at microtubule nucleation sites and traveled on microtubule filaments in mouse and human muscle.
Structured illumination microscopy (SIM) in mouse flexor digitorum brevis (FDB) muscle (A) and human vastus lateralis muscle (B) of endogenous α-tubulin and GLUT4 (left panel) and 3D reconstruction of GLUT4 (green) and α-tubulin (white) (right panel). Arrows indicate GLUT4 at microtubule nucleation sites, arrowheads mark GLUT4 vesicles along the microtubule filaments. (C) Overview of workflow for live-imaging of fluorescently conjugated proteins in adult mouse FDB muscle fibers. (D) Live-imaging of FDB-expressing GLUT4-GFP and mCherry-Tubulin. Yellow projection of mCherry-Tubulin outlines the microtubule filaments (top panel left). Movement of GLUT4-GFP was visualized by color-coded projection (first image cyan, last image red, top panel right). The merged projection (bottom), demonstrated movement of GLUT4-GFP along the mCherry-Tubulin containing microtubule filaments indicated by color-coded projections on top of the microtubule filaments. The movement of GLUT4-GFP is shown in Figure 1—video 1. (A, B) Images are representative of >5 fibers from ≥3 different mice in A + D and 3 different fibers from 3 different subjects in B. Scale bar = 5 µm (A, B, D) and 2 µm (inserts in B, D).
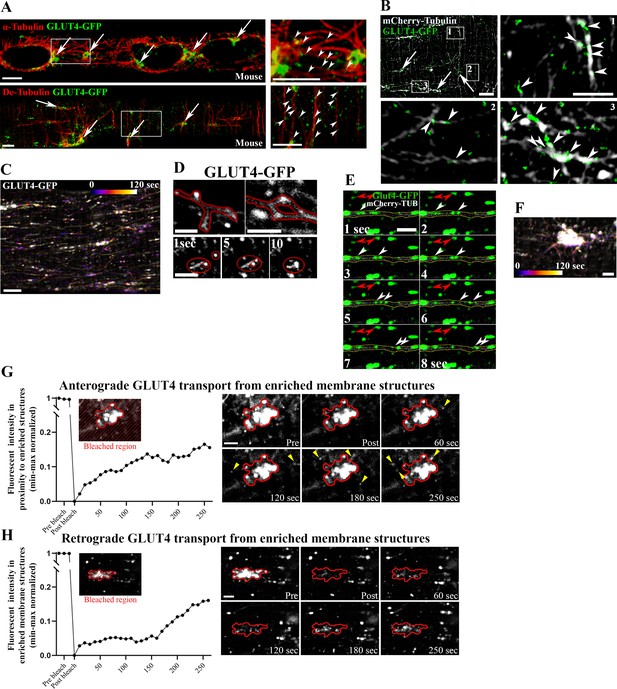
Glucose transporter 4 (GLUT4) undergoes budding and fusion on the microtubules and moves bidirectionally to and from the microtubule nucleation sites.
(A) Confocal imaging of α-tubulin (top panel), detyrosinated tubulin (De-Tubulin, bottom panel) and GLUT4-GFP in flexor digitorum brevis (FDB) fibers from muscles expressing GLUT4-GFP. (B) Structured illumination microscopy (SIM) imaging of FDB fiber-expressing GLUT4-GFP and mCherry-Tubulin. In A, B, arrows mark GLUT4-GFP localized at microtubule nucleation sites and arrowheads indicate GLUT4-GFP on the microtubule filaments. (C) Color-coded projection (first image blue, last image white as indicated) from 120-s live-imaging of FDB fiber-expressing GLUT4-GFP. (D) Live-imaging indicating spherical and tubular GLUT4-GFP structures undergoing fusion and fission. (E) Time-montage of GLUT4-GFP and mCherry-Tubulin from the recording shown in Figure 1D. White arrowheads mark GLUT4-GFP moving on the microtubule filament incorporated with mCherry-Tubulin. Red arrowheads mark static GLUT4-GFP localized away from the microtubule filament. (F) Color-coded projection (first image blue, last image white as indicated) from 120-s live-imaging of GLUT4-GFP-enriched structures at the microtubule nucleation sites in FDB fiber. Directionality of the GLUT4 trafficking is visible in Figure 1—video 2. (G) Quantified graph (left) and time series images (right) of GLUT4-GFP fluorescence in region surrounding microtubule nucleation sites (outside red outline), before and after photobleaching of the surrounding region to estimate plus-end-directed GLUT4 transport. Yellow arrowheads indicate presence of new GLUT4 structures. (H) Quantified graph (left) and time series images (right) of GLUT4-GFP fluorescence at microtubule nucleation site (encircled in red), before and after photobleaching of the central nucleation site region to estimate minus-end-directed GLUT4 transport. Time series images shown are representative of fibers from three different mice. Images are representative of at least 5 fibers from ≥3 different mice. Scale bar = 5 µm (A–C) and 2 µm (D–H and inserts in B).
Glucose transporter 4 (GLUT4) traveled on microtubules.
Video of live flexor digitorum brevis (FDB) fibers electroporated with GLUT4-GFP (green) and mCherry-Tubulin (magenta) 6 days earlier. The video is played at 10 frames per second with one frame representing 1 s. White arrows mark areas displaying frequent GLUT4 movement. Scale bar = 5 μm.
Glucose transporter 4 (GLUT4) traveled to and from GLUT4-enriched regions at microtubule nucleation sites.
Video of GLUT4-GFP at GLUT4-enriched/large structure in live flexor digitorum brevis (FDB) fibers showing spherical and tubular GLUT4-GFP structures that fuse and bud off from the GLUT4-enriched area. To facilitate visualization, the video was generated so that moving GLUT4 appear green-red flashing, whereas static GLUT4 appear yellow, as described in the methods section. The video is played at 10 frames per second with one frame representing 4 s. Scale bar = 5 μm.
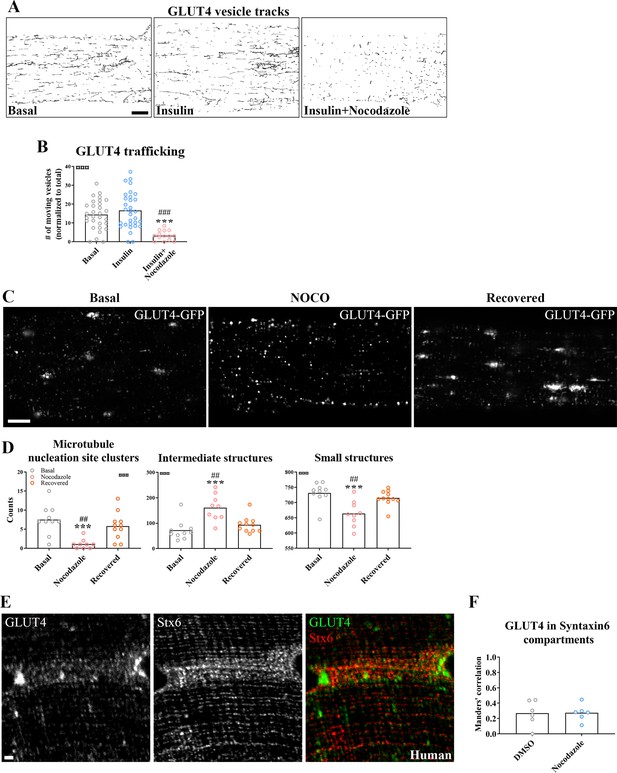
Glucose transporter 4 (GLUT4) trafficking and localization was dependent on an intact microtubule network.
(A) Representative time-lapse traces of GLUT4-GFP vesicle tracking in muscle fibers ± insulin (INS, 30 nM) for 15–30 min with or without microtubule network disruption by Nocodazole (Noco, 13 µM) for 4 hr prior to insulin. The dynamics of GLUT4-GFP in the different conditions are also exemplified in Figure 2—video 1. (B) Quantified microtubule-based GLUT4 trafficking. (C) Representative images of muscle fibers ± pre-treatment with Noco 13 µM, for 15 hr, followed by recovery in Noco-free medium for 9 hr. (D) Quantification of GLUT4 distribution between the microtubule nucleation sites (structures sized >4 µm2), intermediate-sized structures (0.4–4 µm2) and the smallest resolvable structures (<0.4 µm2) in fibers treated as in C. Compartment identification is described in Figure 2—figure supplement 1E. (E) GLUT4 and Syntaxin6 (Stx6) in muscle fiber from human vastus lateralis muscle. (F) GLUT4-Stx6 overlap in perinuclear region of mouse flexor digitorum brevis muscle fibers in Dimethylsulfoxide (DMSO) medium with and without Noco (13 µM) treatment. For A, B, n ≥ 14 muscle fibers from 5 different mice. For C, D, n = 9–11 muscle fibers from 3 different mice. For E, n = 3 subjects. Data are presented as mean with individual data points. ***p < 0.001 different from basal, ###p < 0.001 different from INS, ##p < 0.01 different from Noco recovery. ¤¤¤p < 0.001 analysis of variance (ANOVA) effect. Scale bar = 5 µm (A–C) and 2 µm (E).
-
Figure 2—source data 1
Data used for quantification of GLUT4-Stx6 overlap in perinuclear region of mouse flexor digitorum brevis muscle fibers in DMSO medium with and without Noco (13 µM) treatment.
- https://cdn.elifesciences.org/articles/83338/elife-83338-fig2-data1-v2.zip
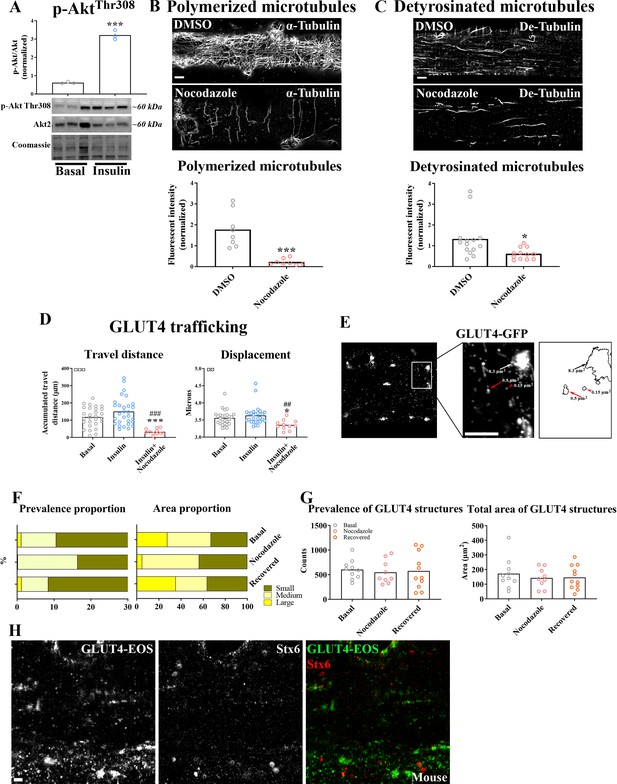
Glucose transporter 4 (GLUT4) trafficking and localization was dependent on an intact microtubule network.
(A) Phosphorylation of Akt Thr308 in GLUT4-GFP-expressing flexor digitorum brevis (FDB) fibers treated ± 30 nM insulin (INS) for 15 min. (B) Polymerized microtubules in GLUT4-GFP-expressing fibers treated ± Nocodazole (Noco, 13 µM) for 4 hr and stained with α-tubulin. (C) Fibers as in B stained for detyrosinated tubulin (De-Tubulin). (D) Microtubule-based GLUT4-GFP trafficking quantified as the traveled distance and the lateral displacement of particles within the dynamic fraction in muscle fibers treated ± Noco (13 µM) for 4 hr and ± INS (30 nM) for 15–30 min. (E) Identification of GLUT4-containing structures in GLUT4-GFP-expressing FDB fibers. Black/white arrows mark large (>4 µm2) structures indicative of GLUT4 clusters at the microtubule nucleation sites. Red arrow marks a structure sized between 0.4 and 4 µm2 and categorized as a intermediate-sized structure. Red arrowhead marks a structure of <0.4 µm2 which is categorized as a small structure. (F) Bar graphs showing the relative prevalence and area in the different membrane size categories in mouse fibers ± microtubule network disruption by addition of Noco (13 µM) 15 hr prior to imaging or 9 hr after Noco removal. (G) Total number and area of structures in mouse fibers as in F. (H) GLUT4-EOS and Syntaxin6 (Stx6) in mouse FDB fibers. For A, n = 3 mice, for B, C n ≥ 8 muscle fibers from 3 different mice. For D, n ≥ 14 muscle fibers from 5 different mice. For F, G, n = 9–11 muscle fibers from 3 different mice. For H, n = 6–7 fibers from 2 different mice. Data are presented as mean with individual data points. */***p < 0.05/0.001 different from Basal/DMSO. ##/###p < 0.01/0.001 different from INS. ¤¤/¤¤¤p < 0.01/0.001 analysis of variance (ANOVA) effect.
-
Figure 2—figure supplement 1—source data 1
Data used for quantification of Figure 2—figure supplement 1A–D, G and raw unedited blots for Figure 2—figure supplement 1A.
- https://cdn.elifesciences.org/articles/83338/elife-83338-fig2-figsupp1-data1-v2.zip
Glucose transporter 4 (GLUT4) movement was disrupted by Nocodazole treatment.
Video of live flexor digitorum brevis (FDB) fibers electroporated with GLUT4-GFP 6 days earlier. To facilitate visualization, the video was generated so that moving GLUT4 appears green-red flashing while static GLUT4 appears yellow, as described in Methods. The left panel shows fiber in the basal state, the middle panel shows fiber treated with insulin for 15 min, and the right panel shows fiber treated with insulin for 15 min after a prior treatment with 4 µg/ml Nocodazole for 4 hr. The video is played at 10 frames per second with one frame representing 4 s. Scale bar = 5 μm.
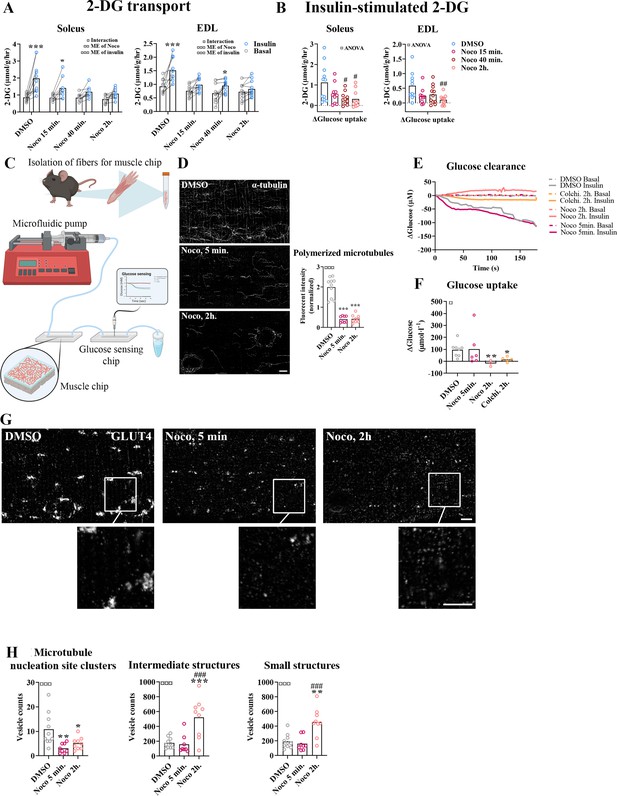
Time-dependent effect of microtubule disruption on insulin-induced muscle glucose uptake.
(A) 2-Deoxyglucose (2-DG) transport in basal and insulin-stimulated mouse soleus and extensor digitorum longus (EDL) muscles pretreated with Nocodazole (Noco, 13 µM) for the indicated time. (B) Insulin-stimulated 2-DG transport (insulin minus basal) from muscles shown in A. (C) Experimental setup for muscle-on-a-chip system with glucose sensor. (D) Microtubules imaged with α-tubulin in glucose transporter 4 (GLUT4)-GFP-expressing mouse flexor digitorum brevis (FDB) fibers treated ± Noco (13 µM) for 5 min or 2 hr. (E) 180-s measurements of glucose concentration in perifusate from basal and insulin-treated FDB muscle fibers in muscle chips pre-incubated with DMSO, Noco (13 µM, 5 min or 2 hr) or colchicine (25 µM, 2 hr). (F) Insulin-stimulated glucose uptake into FDB muscle fibers in muscle chips calculated from the last 20 s of the concentration curves in E. (G) Representative GLUT4 images from isolated mouse FDB muscle fibers treated ± Noco (13 µM) for 5 min or 2 hr. (H) Quantification of GLUT4 in large, intermediate- and small-sized membrane structures in FDB fibers treated ± Noco (13 µM) for 5 min or 2 hr. The membrane compartment division by size is shown in Figure 2E. For A, B, n = 6–7 muscles from 6 to 7 mice. For D, G, H, n = 8–10 muscle fibers from 3 different mice. For E, F, n ≥ 3 muscle chips from 3 to 4 mice. Data are presented as mean with individual data points. Paired observations from the same mouse are indicated by a connecting line. */**/***p < 0.05/0.01/0.001 different from basal/DMSO, #/##/###p < 0.05/0.01/0.001 different from DMSO. ¤/¤¤/¤¤¤p < 0.05/0.01/0.001 analysis of variance (ANOVA) effect. Scale bar = 5 µm.
-
Figure 3—source data 1
Data used for quantification of 2-DG transport and glucose clearance and uptake in Figure 3A, B, E, F, polymerized microtubules in Figure 3D and glucose transporter 4 (GLUT4) localization in Figure 3H.
- https://cdn.elifesciences.org/articles/83338/elife-83338-fig3-data1-v2.zip
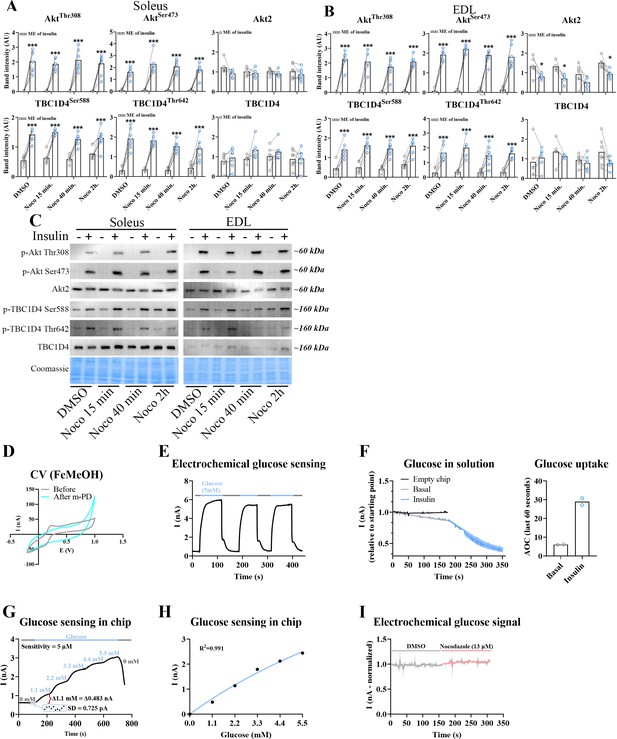
Time-dependent effect of microtubule disruption on insulin-induced muscle glucose uptake.
Quantification of protein expression in soleus (A) and extensor digitorum longus (EDL) (B) muscles in the basal and insulin-stimulated state ± Nocodazole (Noco, 13 µM) as indicated. (C) Representative immunoblots of the proteins quantified in A, B. (D) Deposition of poly-(m-phenylenediamine) (m-PD) film as exclusion membrane to improve sensor selectivity demonstrated by abolished anodic signal in the voltammograms (scanning rate [SR] = 100 mV s−1) performed at the clean Pt surface in 1 mM FcMeOH after m-PD deposition. (E) Glucose oxidase presence validation by current (nA) change upon sensor transfer to and from a glucose solution (5 mM). (F) Current change and calculated area over the curve (AOC) in perifusate from mouse flexor digitorum brevis (FDB) fibers before and after insulin stimulation. (G) Typical calibration trace (Q = 1 μl s−1) when exposing glucose sensor to stepwise 1.1 mM glucose increments. Sensitivity of glucose measurement was calculated as 3*SD/Δ current * Δ glucose. Standard deviation (SD) was calculated from 10 baseline data points, Δ current was calculated when switching from 0 to 1.1 mM glucose as indicated by the red bracket. (H) Associated calibration curve, fit with a Michaelis–Menten model. (I) Current fluctuations in perifusate from FDB fibers ± Noco (13 µM). ME = main effect. For A–C, n = 6–7 muscles from 6 to 7 mice. Data are presented as mean with individual data points. Paired observations from the same mouse are indicated by a connecting line. F, I, graphs indicate mean ± SD. */***p < 0.05/0.001 different from DMSO. ¤/¤¤¤p < 0.05/0.001 analysis of variance (ANOVA) effect.
-
Figure 3—figure supplement 1—source data 1
Data used for quantification of protein expression and electrochemical glucose sensing in Figure 3—figure supplement 1.
Data used for quantification of Figure 3—figure supplement 1A, B, F, I and raw unedited blots for Figure 2—figure supplement 1A, B.
- https://cdn.elifesciences.org/articles/83338/elife-83338-fig3-figsupp1-data1-v2.zip
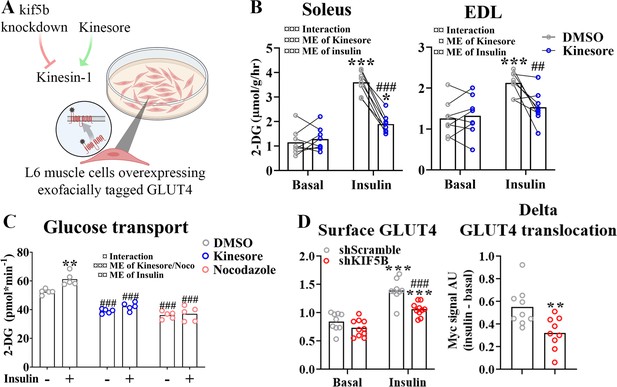
Kinesin-1 containing KIF5B-regulated glucose transporter 4 (GLUT4) localization and translocation.
(A) Schematic overview of L6 muscle cell system to assess GLUT4 surface content. (B) 2-Deoxyglucose (2-DG) transport in basal and insulin-stimulated mouse soleus and extensor digitorum longus (EDL) muscles pretreated with kinesore (50 µM) for 2 hr. (C) Deoxyglucose (2-DG) transport in basal and insulin-stimulated primary human myotubes pretreated ± kinesore (50 µM) or Noco (13 µM) for 2 hr. (D) Exofacial GLUT4 signal in serum starved (4 hr) basal and insulin-stimulated (100 nM, 15 min) L6 myoblasts (left) and insulin response (insulin minus basal, right) in GLUT4 surface content. L6 myoblasts were transfected with short hairpin scramble RNA (shScramble) or shRNA targeting Kif5b 72 hr prior to the experiment. Analysis of variance (ANOVA) main effect of insulin (¤¤¤) and shKif5b (¤¤¤) and interaction (¤). (B) n = 8 muscles in each group, lines indicate muscles from same mouse. (C) Each data point represents the average of 3 replicates and originate from at least 3 independent experiments. Data are presented as mean with individual data points. */**/***p < 0.01/0.001 effect of insulin. ##/###p < 0.01/0.001 different from DMSO/Scramble. ¤/¤¤/¤¤¤p < 0.05/0.01/0.001 ANOVA effect.
-
Figure 4—source data 1
Data used for quantification of glucose transporter 4 (GLUT4) localization and GLUT4 surface content in Figure 4.
Data used for quantification of 2-DG transport and GLUT4 translocation in Figure 4B–D.
- https://cdn.elifesciences.org/articles/83338/elife-83338-fig4-data1-v2.zip
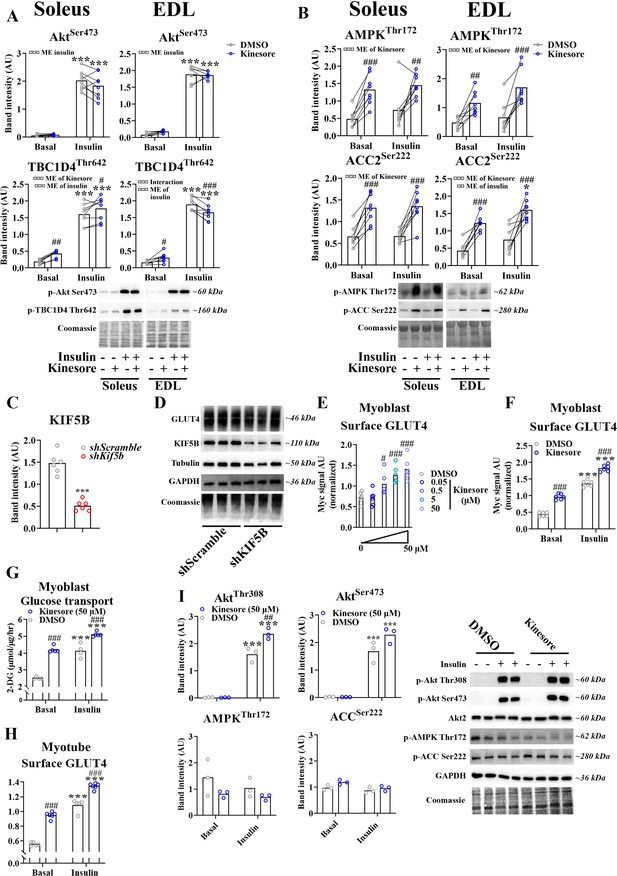
Kinesin-1 containing KIF5B-regulated glucose transporter 4 (GLUT4) localization and translocation.
(A, B) Quantification and representative immunoblots of protein expression in soleus and extensor digitorum longus (EDL) muscles in the basal and insulin-stimulated state ± 2 hr kinesore (50 µM) pretreatment. (C) Quantification of KIF5B protein expression in L6 myoblasts transfected with shScramble RNA or shRNA targeting Kif5b for 72 hr. (D) Representative immunoblots showing protein expression in L6 myoblasts as in C. (E) Exofacial GLUT4 signal at the surface membrane of L6 myoblast serum starved for 4 hr and treated ± kinesore at indicated concentration for the last 2 hr before cell fixation. Analysis of variance (ANOVA) effect (¤¤¤). (F) Exofacial GLUT4 signal at the surface membrane in L6 myoblast serum starved for 4 hr and treated ± kinesore (50 µM) for the last 2 hr before 15 min ± insulin (100 nM). ANOVA main effect of insulin (¤¤¤) and kinesore (¤¤¤) and interaction (¤¤). (G) 2-Deoxyglucose (2-DG) transport in L6 myoblast serum starved for 4 hr and treated ± kinesore (50 µM) for 2 hr before 20 min ± insulin (100 nM) and 5 min 2-DG accumulation. ANOVA main effect of insulin (¤¤¤) and kinesore (¤¤¤) and interaction (¤¤). (H) Exofacial GLUT4 signal at the surface membrane in 7 days differentiated L6 myotubes serum starved for 4 hr and treated ± kinesore (50 µM) for the last 2 hr before 15 min ± insulin (100 nM). ANOVA main effect of insulin (¤¤¤) and kinesore (¤¤¤) and interaction (¤¤). (I) Quantification and representative immunoblots showing protein expression and phosphorylation in basal and insulin-stimulated (100 nM, 15 min) L6 myoblasts serum starved for 4 hr and treated ± kinesore for 2 hr. ANOVA main effect of insulin (Akt Thr308 + Ser473 = ¤¤¤, ACC Ser222 = ¤), ANOVA main effect of kinesore (ACC Ser222 = ¤, Akt Ser473 + AMPK Thr172 p = 0.07), interaction (Akt Thr308 = ¤¤, Akt Ser473 p = 0.06). (A, B) n = 8 muscles in each group, lines indicate muscles from same mouse. (C, D) n = 6 replicates from 2 independent experiments. (E) n = 6 replicates from 2 independent experiments. (F) Each data point represents the average of 3 replicates and originate from 3 independent experiments. (G) n = 5–6 replicates from 2 independent experiments. (H) Each data point represents the average of 3 replicates and originate from 3 independent experiments. (I) n = 3 independent experiment. ***p < 0.001 effect of insulin/shKif5b. #/##/###p < 0.05/0.01/0.001 different from DMSO. ¤/¤¤/¤¤¤p < 0.05/0.01/0.001 ANOVA effect.
-
Figure 4—figure supplement 1—source data 1
Data used for quantification of glucose transporter 4 (GLUT4) surface content, protein expression, and glucose uptake in Figure 4—figure supplement 1.
Data used for quantification of Figure 4—figure supplement 1A–C, E–I and raw unedited blots for Figure 4—figure supplement 1A, B, D, I.
- https://cdn.elifesciences.org/articles/83338/elife-83338-fig4-figsupp1-data1-v2.zip
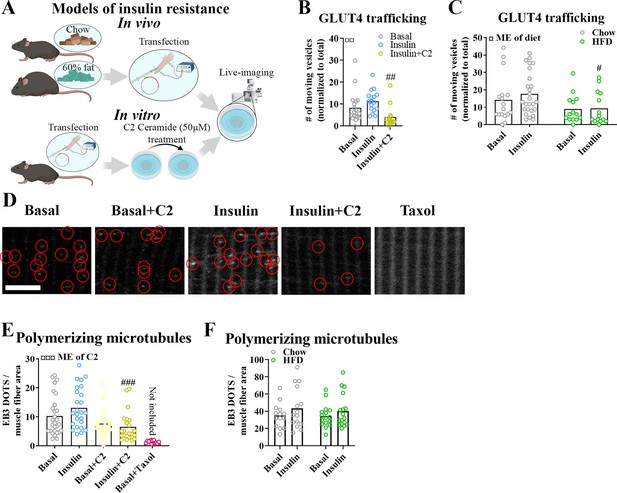
Insulin resistance impairs microtubule-based glucose transporter 4 (GLUT4) trafficking.
(A) Overview of in vitro and in vivo insulin resistance models used. (B) Quantified microtubule-based GLUT4 trafficking in basal, insulin (INS, 30 nM) and insulin + C2 ceramide (C2) (INS + C2, 30 nM + 50 µM) treated flexor digitorum brevis (FDB) muscle fibers. (C) Quantified microtubule-based GLUT4 trafficking in basal or INS (30 nM) treated FDB fibers from chow or high-fat diet (HFD) fed mice. (D) Representative images of polymerizing microtubules in EB3-GFP-expressing FDB muscle fibers treated ± C2 (50 µM), paclitaxel (Taxol, 10 µM) for 2 hr prior to 15–30 min of INS (30 nM) stimulation. Red circles highlight microtubule tip-bound EB3-GFP. (E) Quantification of polymerizing microtubules based on EB3-GFP in FDB fibers treated as in D. (F) Quantification of polymerizing microtubules based on EB3-GFP in FDB fibers isolated from chow or 60% HFD fed mice and treated ± INS (30 nM) for 15–30 min. For B–F, n ≥ 13 muscle fibers from 3 to 4 mice. Taxol-treated muscle fibers were only used as a control and not included in the statistical analysis. NA = not statistically analysed. Data are presented as mean with individual data points. #/##/###p < 0.05/0.01/0.001 different from INS (B) or different from corresponding group in chow fed mice (C) or control fibers (E). ¤/¤¤/¤¤¤p < 0.05/0.01/0.001 main effect (ME) of diet/C2.
-
Figure 5—source data 1
Data used for quantification of glucose transporter 4 (GLUT4) trafficking and microtubule polymerization in Figure 5B, C, E, F.
- https://cdn.elifesciences.org/articles/83338/elife-83338-fig5-data1-v2.zip
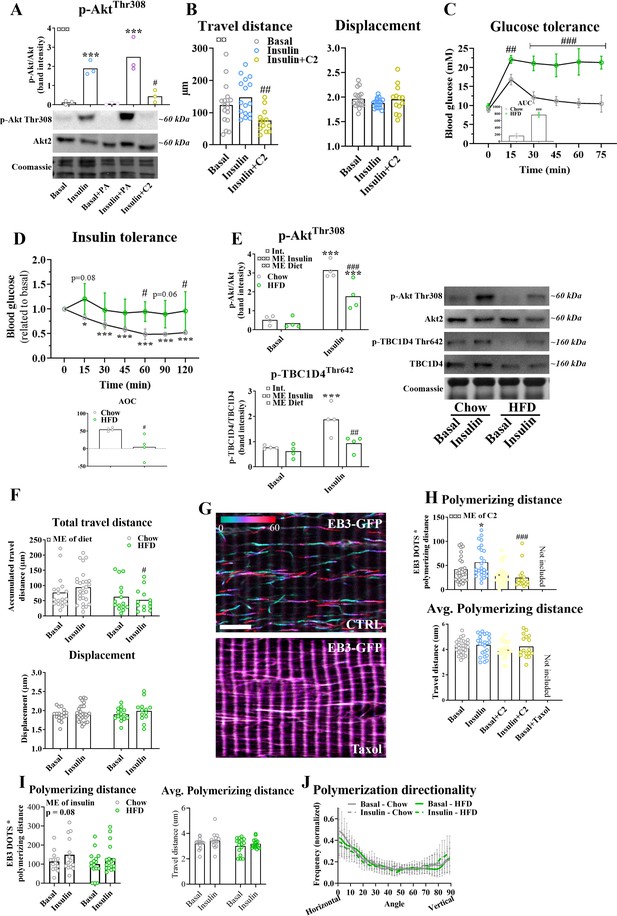
Insulin resistance impairs microtubule-based glucose transporter 4 (GLUT4) trafficking.
(A) Phosphorylation of AktThr308 in GLUT4-GFP-expressing flexor digitorum brevis (FDB) muscle fibers cultured ± 0.5 mM palmitic acid (PA) for 24 hr or 50 µM C2 ceramide (C2) for 2 hr prior to 15 min ± 30 nM insulin (INS) stimulation. (B) Quantified microtubule-based GLUT4 trafficking assessed as the traveled distance and the displacement within the dynamic fraction of the GLUT4-GFP in basal, insulin (INS, 30 nM) or insulin and C2 ceramide (INS + C2, 30 nM + 50 µM)-treated FDB muscle fibers. (C, D) Glucose and insulin tolerance tests of mice fed a control chow or a high-fat diet (HFD) after intraperitoneal injection of glucose (2 g kg−1 body weight) or insulin (0.5 U kg−1 body weight). AUC/AOC = area under/over the curve. Analysis of variance (ANOVA) main effect of diet (¤¤¤/¤, GTT/ITT), and time (¤¤¤) and interaction (¤¤¤/¤¤, GTT/ITT). (E) Phosphorylation of AktThr308 and TBC1D4Thr642 in isolated FDB fibers from muscles from chow or HFD fed mice treated ± INS (30 nM) for 15 min. (F) Quantified microtubule-based GLUT4 trafficking assessed as the traveled distance and the displacement within the dynamic fraction of the GLUT4-GFP in basal or INS (30 nM)-stimulated FDB muscle fibers isolated from chow or HFD-fed mice. (G) Color-coded projection (first image cyan, last image red as indicated by color code bar) of 60-s live-imaging of FDB fibers expressing EB3-GFP and treated without (CTRL) or with 10 µM paclitaxel (Taxol) for 2 hr. Scale bar = 5 µm. EB3-GFP dynamics are also illustrated in Figure 5—video 1. (H) Quantification of the total and average polymerizing distance of EB3-GFP containing microtubules in fibers incubated ±50 µM C2 ceramide (C2) or 10 µM Taxol for 2 hr prior to 15–30 min of INS (30 nM) stimulation. (I) Quantification of the total and average polymerizing distance of EB3-GFP containing microtubules in fibers isolated from chow or HFD fed mice and stimulated ± INS (30 nM) for 15 min. (J) Quantification of microtubule polymerization directionality based on EB3-GFP dynamics recording as in I. For A, C–E, n = 3–4 mice, for B, F, and H–J n ≥ 13 muscle fibers from 3 to 4 mice. (G) Representative of >10 fibers from 2 different mice. Taxol-treated muscle fibers were only used as a control and not included in the statistical analysis. NA = not analysed. Data are presented as mean with individual data points or mean ± standard deviation (SD). */***p < 0.05/0.001 different from basal, #/##/###p < 0.05/0.01/0.001 different from insulin (A, B) or different from corresponding group in chow fed mice (C–F, H). ¤/¤¤/¤¤¤p < 0.05/0.01/0.001 ANOVA main effect (ME).
-
Figure 5—figure supplement 1—source data 1
Data used for quantification of protein expression, glucose transporter 4 (GLUT4) trafficking, glucose and insulin tolerance, microtubule polymerization and polymerization directionality in Figure 5—figure supplement 1.
Data used for quantification of Figure 5—figure supplement 1A–F and H–J and raw unedited blots for Figure 5—figure supplement 1A, D.
- https://cdn.elifesciences.org/articles/83338/elife-83338-fig5-figsupp1-data1-v2.zip
Polymerizing microtubules in muscle fibers.
Video of polymerizing microtubule (MT) tips detected by EB3-GFP in live flexor digitorum brevis (FDB) fibers electroporated with EB3-GFP 6 days earlier. To facilitate visualization, the video was generated so that moving MT tips (EB3 dots) appear green-red flashing whereas static EB3 appear yellow, as described in Methods. The video is played at 10 frames per second with one frame representing 4 s. Scale bar = 5 μm.
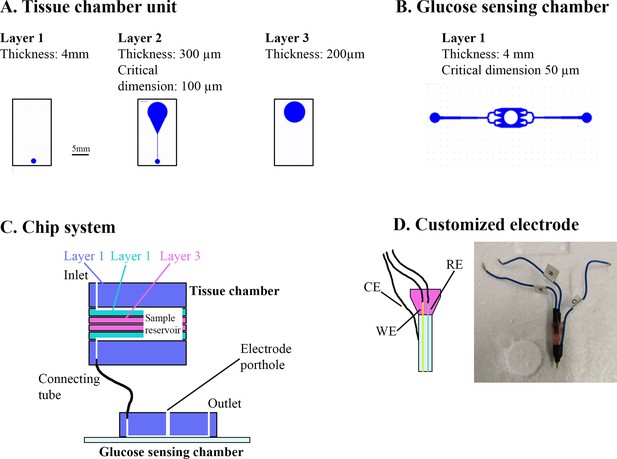
Overview of muscle chip for glucose sensing.
(A) Overview of the different poly(dimethylsiloxane) (PDMS) layers for the tissue chamber unit. Scale bar = 5 mm. (B) Microfluidic system for the glucose-sensing chamber. The electrode was placed in the center of the system in the punched hole. (C) Overview of the muscle chip system showing the various layers of the tissue chamber as well as the connection to the glucose-sensing chamber. (D) Schematic drawing and picture of the customized electrode based on glucose oxidase fabricated to sense glucose. RE = reference electrode, CE = counter electrode, WE = working electrode.