Z-REX uncovers a bifurcation in function of Keap1 paralogs
Figures
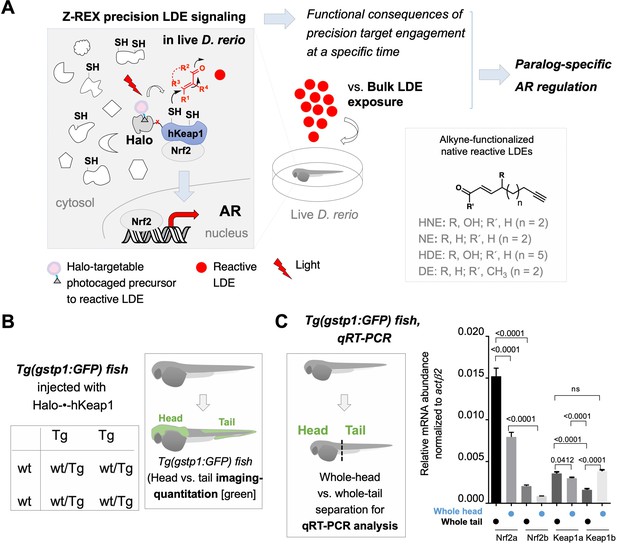
Z-REX directly evaluates the functional consequences of reactive electrophile–target engagement in live zebrafish embryos at a specific time.
(A) This work investigates the biological impacts on druggable antioxidant response (AR) pathway at the organismal level following Z-REX-enabled hKeap1-specific electrophilic modification in live zebrafish embryos and compares these results to those obtained from bulk reactive electrophile exposure. In the process, novel paralog-specific regulation of AR was discovered. Inset, lower right: Structures of alkyne-functionalized lipid-derived electrophiles (LDEs). Unless otherwise specified, all LDEs deployed were alkyne-functionalized. See also Figure 1—figure supplement 1A. (B) Left: Punnett square denoting how cross of wild-type zebrafish with a transgenic strain (homozygous, or heterozygous, not shown) ensures that all transgenic progeny are heterozygous for the AR-reporter GFP-gene. Right: Using whole-mount immunofluorescence (IF) imaging, Tg(gstp1:GFP) heterozygotes were quantified separately for AR levels in head and tail regions indicated in green. Note: GFP expression was detected using immunofluorescence (IF) in fixed fish, analyzed by red fluorescence. The IF protocol is used because auto-fluorescence in the green channel is high in fish and prevents accurate quantitation and this avoids concerns regarding effects of electrophile on GFP fluorescence. (For whole-head/whole-tail separation in qRT-PCR analysis, see Figure 1C, inset). (C) Inset, left: Illustration for head vs. tail qRT-PCR analysis, where the fish were mechanically separated as marked by the dashed line. Right: The relative levels of mRNA of each paralog were assessed using qRT-PCR following physical separation of head and tail (see inset on left). Number of embryos analyzed: Head, all paralogs (6); Tail zKeap1a and zKeap1b (10), zNrf2a (5), zNrf2b (6). Note: these segments contain tissue other than the areas that express the AR-reporter GFP-gene. All numerical data present mean ± sem. Numbers above the bars represent analysis by two-tailed t-tests.
-
Figure 1—source data 1
Quantification results.
- https://cdn.elifesciences.org/articles/83373/elife-83373-fig1-data1-v3.xlsx
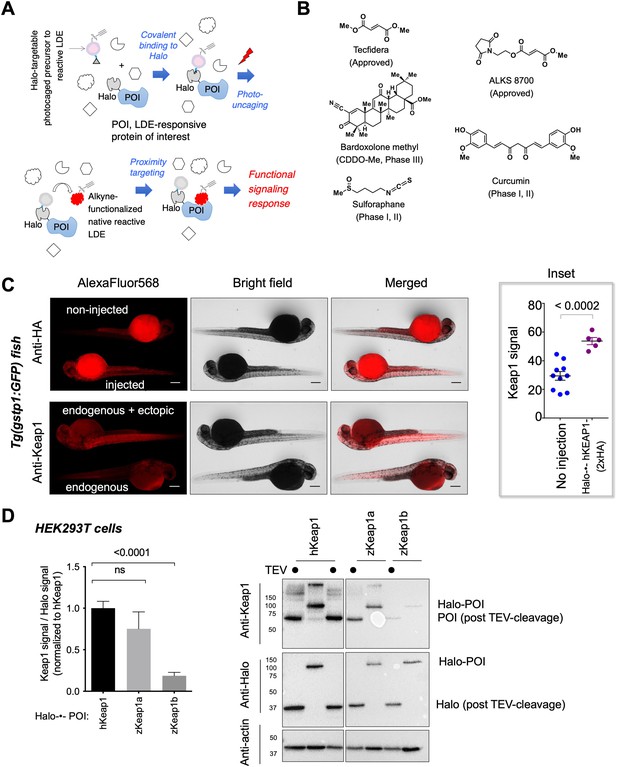
Halo-•-hKeap1-(2xHA) fusion protein deployed in Z-REX is ubiquitously expressed in fish to a similar level as endogenous zKeap1-paralogs.
(A) Z-REX workflow (also see Appendix-Scheme 1): zebrafish embryos transiently expressing Halo-POI are treated with the bioinert and live-fish permeable Halo-targetable photocaged LDE-precursor (Ht-PreLDEs) Parvez et al., 2016; Long et al., 2017c. A hexyl chloride linker within the small-molecule photocaged precursor selectively binds Halo in vivo. Low-energy UV light (365 nm, 5 mW/cm2, 1–4 min) liberates LDE in proximity of Halo-POI Long et al., 2016, resulting in POI modification provided POI is a kinetically-privileged first responder to the LDE Parvez et al., 2018; Liu et al., 2019. This concept enables a direct and precise evaluation of target engagement in vivo under electrophile-limited conditions. (B) Representative electrophilic covalent small-molecule therapeutics and candidates housing Michael acceptors (see text for details) Poganik and Aye, 2020; Cuadrado et al., 2019. (C) IF in zebrafish larvae injected with Halo-•-hKeap1-(2xHA) mRNA and probed with indicated antibodies conjugated to AlexaFluor568 shows ubiquitous expression of the indicated transgene and that Halo-•-hKeap1-(2xHA)-transgene expression levels are similar to those of endogenous Keap1. Top: Non-injected larvae; bottom: larvae derived from embryos injected with mRNA encoding transgene. The level of ectopic Halo-•-hKeap1-(2xHA) protein is comparable to endogenous Keap1. Inset: Quantitation of fluorescence intensity (i.e., Keap1 protein levels) in non-injected fish larvae and those ectopically expressing Halo-•-hKEAP1-(2xHA). Number of larvae evaluated: No injection: 10; Halo-•-Keap1-(2xHA): 5. (D) Quantification of affinity of Keap1-antibody to hKeap1 compared to the zebrafish paralogs. All Keap1 variants were expressed with a HaloTag in HEK293T cells. The Keap1 signal was normalized to the Halo signal to allow assessment of the relative sensitivity of our Keap1 antibody to each Keap1 variant. All numerical data present mean ± sem. Numbers above the bars represent analysis by two-tailed t-tests.
-
Figure 1—figure supplement 1—source data 1
Full view blot image.
- https://cdn.elifesciences.org/articles/83373/elife-83373-fig1-figsupp1-data1-v3.zip
-
Figure 1—figure supplement 1—source data 2
Raw blot images.
- https://cdn.elifesciences.org/articles/83373/elife-83373-fig1-figsupp1-data2-v3.zip
-
Figure 1—figure supplement 1—source data 3
Quantification results.
- https://cdn.elifesciences.org/articles/83373/elife-83373-fig1-figsupp1-data3-v3.xlsx
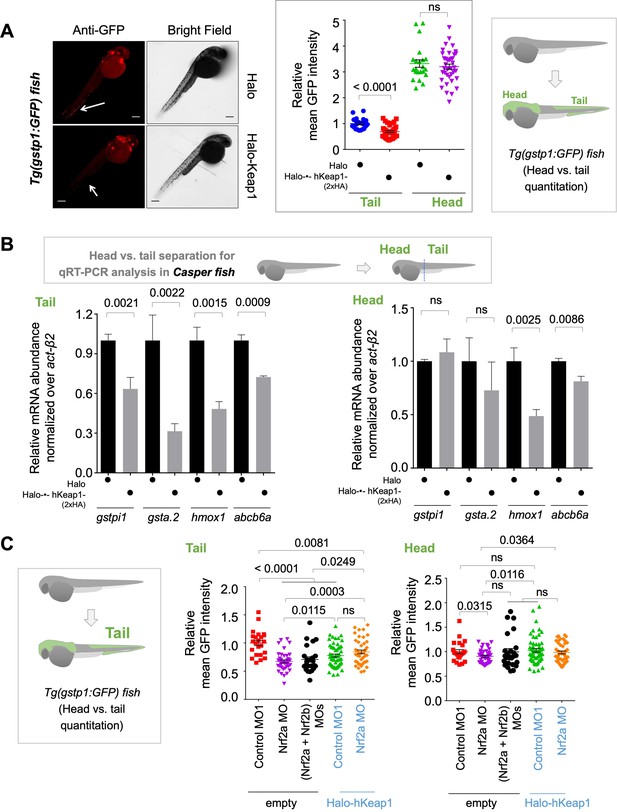
Halo-•-hKeap1-(2xHA) fusion protein expressed in fish is a functional antagonist of antioxidant response (AR) pathway.
(A) Ectopic Halo-•-hKeap1-(2xHA) is a functional negative-regulator of AR in zebrafish embryos. Left panel: Representative IF images in Tg(gstp1:GFP) embryos show a decrease in GFP signal intensity (immuno-stained with goat anti-GFP primary antibody and anti-goat secondary antibody conjugated with AlexaFluor568) in the tail of embryos injected with Halo-•-hKeap1-(2xHA)mRNA (bottom panel) relative to those injected with Halo mRNA alone (top panel). Arrows point to the region in the tail with upregulated AR. Middle panel: Image quantitation was performed on the head/tail-regions illustrated in the inset, on right. Halo: n=22; Halo-•-hKeap1-(2xHA)-(2xHA): n=38. Note: the immunofluorescence (IF) protocol is used because auto-fluorescence in the green channel is high in fish and prevents accurate quantitation. (B)Inset above: Illustrating separation of whole-head and whole-tail prior to RNA isolation separately from head and tails, for qPCR analysis. qRT-PCR analysis of casper zebrafish larvae expressing Halo-•-hKeap1-(2xHA) mRNA shows a lower-level abundance of endogenous transcripts of AR-regulated genes compared to those injected with Halo mRNA alone. 2 hr post Z-REX, embryos were euthanized, head and tail separated before RNA extraction. Comparable to results from IF-imaging-data described elsewhere, transcript levels of AR-regulated endogenous genes in head are less responsive compared to the tail to Halo-•-hKeap1-(2xHA) injection. n=3 independent biological replicates and 2 technical replicates per sample. (C) Halo-•-hKeap1-(2xHA) overexpression, or Nrf2a-alone downregulation, is sufficient for complete AR-suppression in the tail. Quantitation of AR in tails (middle panel) or the heads (right panel) of Tg(gstp1:GFP) embryos co-injected with the indicated reagents. In the tail, co-injection of Nrf2a ATG MO and Nrf2b ATG MO does not suppress AR further than injection of Nrf2a ATG MO alone, demonstrating that Nrf2a downregulation is sufficient for complete AR suppression. A similar observation is reached for Nrf2a ATG MO and Halo-•-hKeap1-(2xHA) mRNA injection. Empty: Control MO1 (23); Nrf2a ATG MO (40); (Nrf2a+Nrf2 b) ATG MOs (24); Halo-•-hKeap1-(2xHA): Control MO1 (54); and Nrf2a ATG MO (43). All numerical data present mean ± sem. Numbers above the bars represent analysis by two-tailed t-tests.
-
Figure 1—figure supplement 2—source data 1
Quantification results.
- https://cdn.elifesciences.org/articles/83373/elife-83373-fig1-figsupp2-data1-v3.xlsx
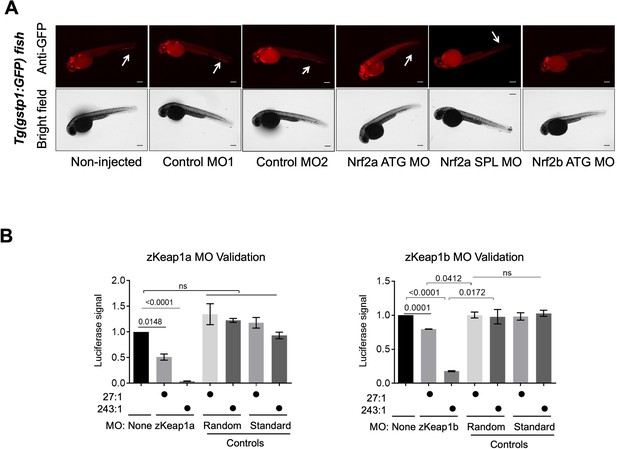
Validations of knockdown and MOs used by pathway response assessment and in vitro translation reporter analysis.
(A) Treatment with zNrf2 ATG/SPL-MOs results in AR-pathway suppression as expected. Representative IF images in Tg(gstp1:GFP) fish. Data show a decrease in GFP expression in embryos injected with Nrf2a and Nrf2b morpholinos (MO; 2 nL, 0.5 mM), either blocking protein translation (ATG MO) or splicing (SPL MO), relative to non-injected embryos or those injected with Control MOs (see Appendix). (B) zKeap1 ATG-MOs inhibit translation as expected. mRNA encoding the binding site of either zKeap1a or zKeap1b MOs upstream of an in-frame copy of the gene encoding Firefly luciferase (see Appendix) was generated. This mRNA was incubated with either control or corresponding MO for 5 min at room temperature, followed by addition to rabbit reticulocyte lysate. The sample was loaded into a white plate 96 well plate, and translated Firefly luciferase was quantified using standard methods. All numerical data present mean ± sem. Numbers above the bars represent analysis by two-tailed t-tests.
-
Figure 1—figure supplement 3—source data 1
Quantification results.
- https://cdn.elifesciences.org/articles/83373/elife-83373-fig1-figsupp3-data1-v3.xlsx
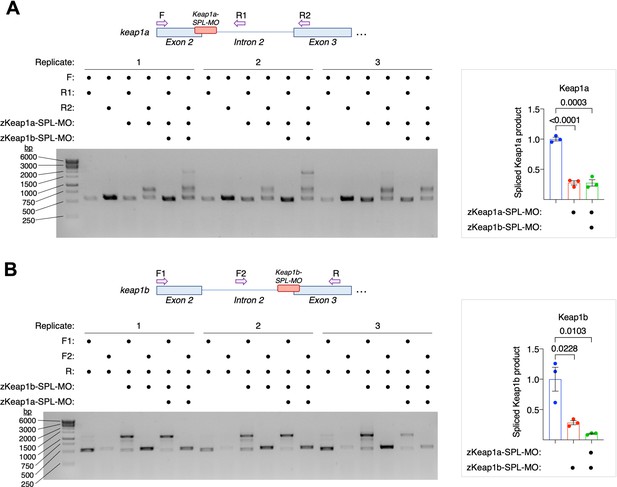
Validations of knockdown and MOs used by reverse transcription-PCR analysis.
(A–B) zKeap1a/b splice-blocking MOs inhibit zKeap1a/b-splicing expected. MOs (2 nl of 0.5 mM MO) targeting the indicated region of the zKeap1a/b-transcript were injected and RNA was isolated and reverse transcribed at 30hpf. Primers as indicated in the figures were used to amplify the targeted sites and the resulting PCR products were run on a gel. Inset at right: Bands corresponding to the correctly spliced product were quantitated using the Measure tool of image-J(NIH) (n=3 independent replicates). All numerical data present mean ± sem. Numbers above the bars represent analysis by two-tailed t-tests.
-
Figure 1—figure supplement 4—source data 1
Full view gel image.
- https://cdn.elifesciences.org/articles/83373/elife-83373-fig1-figsupp4-data1-v3.zip
-
Figure 1—figure supplement 4—source data 2
Raw gel image.
- https://cdn.elifesciences.org/articles/83373/elife-83373-fig1-figsupp4-data2-v3.zip
-
Figure 1—figure supplement 4—source data 3
Quantification results.
- https://cdn.elifesciences.org/articles/83373/elife-83373-fig1-figsupp4-data3-v3.xlsx
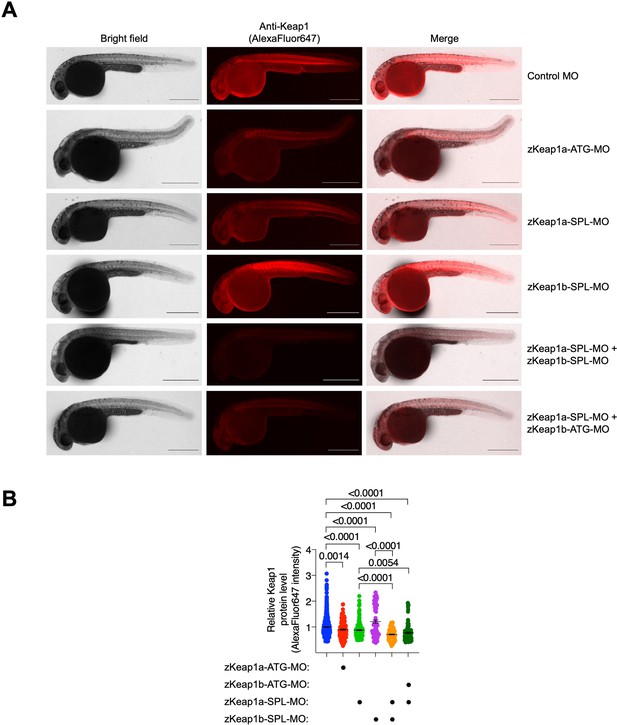
Validations of knockdown and MOs used by protein expression level assessment.
Treatment with zKeap1 ATG/SPL-MOs results in reduced zKeap1 expression. (A) Representative images of zebrafish injected with the indicated MO (2 nl of 0.5 mM MO), fixed at 30 hpf, and stained with anti-Keap1. Scale bars, 500 μm. (B) IF data were quantified using the measure tool of Image-J(NIH) (mean ± sem; sample sizes analyzed: Control MO, n=908; zKeap1a ATG-MO, n=153; zKeap1a-SPL-MO, n=192; zKeap1b-SPL-MO, n=75; zKeap1a+zKeap1 b SPL-MOs, n=131; zKeap1a-SPL-MO+zKeap1b-ATG-MO, n=115). All numerical data present mean ± sem. Numbers above the bars represent analysis by two-tailed t-tests.
-
Figure 1—figure supplement 5—source data 1
quantification results.
- https://cdn.elifesciences.org/articles/83373/elife-83373-fig1-figsupp5-data1-v3.xlsx
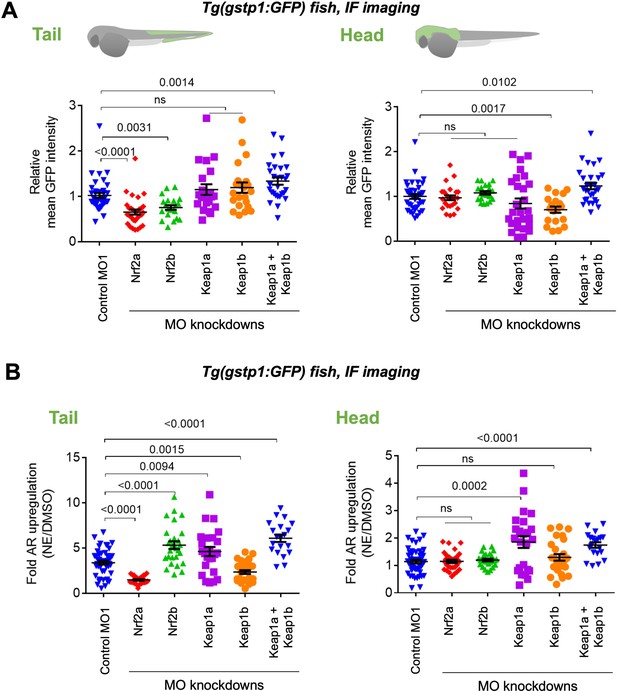
Assessments of AR-reporter in the fish tail vs head reveal differential roles of zKeap1a and zKeap1b.
Homozygous Tg(gstp1:GFP) fish were crossed with wt fish. Resulting heterozygous embryos were injected with the stated morpholino (MO) at the 1- to four-cell stage. (See experimental Workflow in Appendix 1-Scheme 1). Image quantitation was performed on the head/tail-regions as illustrated. Note: GFP expression was detected using immunofluorescence (IF) in fixed fish, analyzed by red fluorescence. The IF protocol is used because auto-fluorescence in the green channel is high in fish and prevents accurate quantitation and IF avoids concerns regarding effects of electrophile on GFP fluorescence. ATG MOs used for single-MO injection, SPL MOs used for simultaneously knocking down zKeap1a and zKeap1b; see Figure 1—figure supplements 3–5 and Figure 2—figure supplements 1–2 for MO validations and Appendix for MO sequences. Also see Figure 1—figure supplements 1–5, Figure 2—figure supplements 1–2, Figure 3—figure supplement 1. (A) Quantitation of GFP expression (which indicates relative basal AR-levels) in the tail (left panel) and head (right) of Tg(gstp1:GFP) zebrafish larvae following MO-knockdown of the indicated zKeap1 and zNrf2 paralogs. No. embryos analyzed: Control MO (38), zNrf2a MO (32), zNrf2b MO (21), zKeap1a MO (21), zKeap1b MO (22), zKeap1a and zKeap1b MOs (29). (B) Quantitation of the relative fold change of AR level (GFP signal) in the tail (left panel) and head (right) following bulk electrophile (NE; see Figure 1A inset) exposure. No. embryos analyzed: Control MO (48), zNrf2a MO (27), zNrf2b MO (27), zKeap1a MO (24), zKeap1b MO (29), zKeap1a and zKeap1b MOs (20). All numerical data present mean ± sem. Numbers above the bars represent analysis by two-tailed t-tests.
-
Figure 2—source data 1
Quantification results.
- https://cdn.elifesciences.org/articles/83373/elife-83373-fig2-data1-v3.xlsx
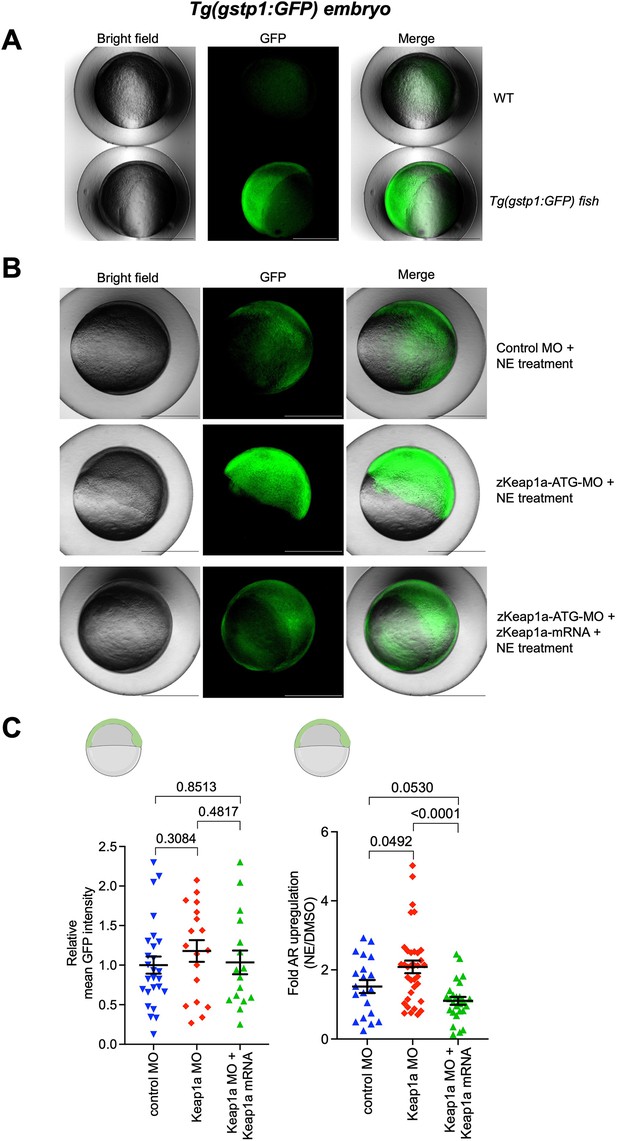
Validations of zKeap1a-ATG-MO.
zKeap1a-mRNA rescues the effects of MO knockdown. (Also see Figure 1—figure supplements 3B and 4,5). (A) Representative images of wildtype (WT) and Tg(gstp1:GFP) zebrafish at 8 hpf. (B) Representative images of zebrafish (co-)injected with the indicated MO and mRNA (2 nl of 0.2 mM zKeap1a-ATG-MO; 250 ng/μL Halo-TEV-zKeap1a mRNA), treated at 4 hpf with 10 μM NE (4 hr treatment period followed by image acquisition at 8 hpf). (C) GFP expression from the experiments in (B) was quantified using the measure tool of Image-J(NIH). Yolk sac was excluded from quantification (see sketches on top). Sample sizes analyzed: (left panel, DMSO-treated): Control MO, n=26; zKeap1a MO, n=18; zKeap1a MO + zKeap1 a mRNA, n=16; (right panel, NE-treated): Control MO, n=20; zKeap1 MO, n=35; zKeap1a MO + zKeap1 a mRNA, n=26. Fish age: 8 hpf. Scale bars, 500 μm. All numerical data present mean ± sem. Numbers above the bars represent analysis by two-tailed t-tests.
-
Figure 2—figure supplement 1—source data 1
Quantification results.
- https://cdn.elifesciences.org/articles/83373/elife-83373-fig2-figsupp1-data1-v3.xlsx
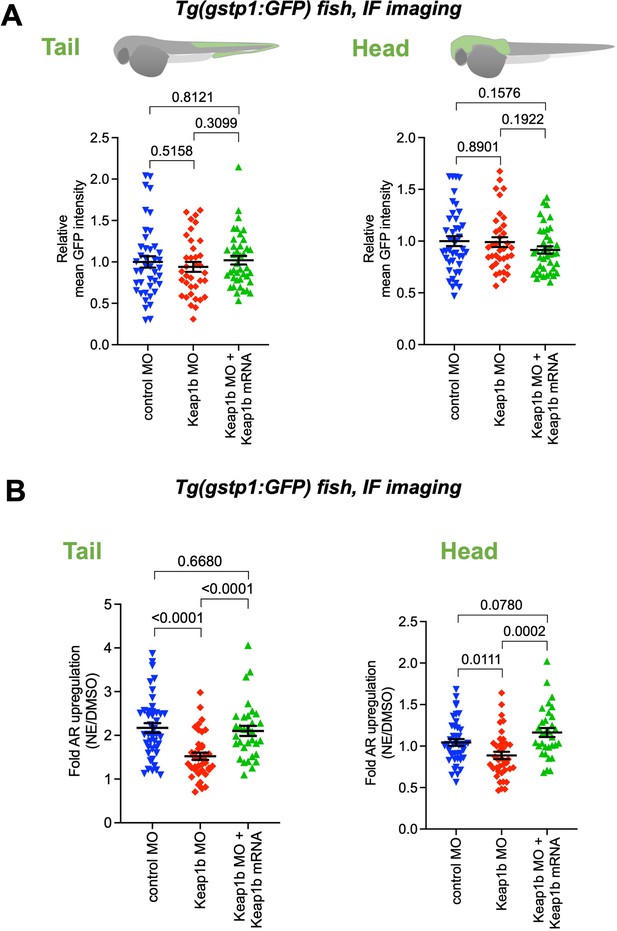
Validations of zKeap1b-ATG-MO.
zKeap1b-mRNA rescues the effects of MO knockdown. (Also see Figure 1—figure supplements 3B, 4 and 5,). (A) Quantitation of GFP expression in the tail (left panel) and head (right panel) of zebrafish (co-)injected with the indicated MO and mRNA (2 nl of 0.25 mM zKeap1b-ATG-MO; 250 ng/μL Halo-TEV-zKeap1b mRNA). Sample sizes analyzed: Control MO, n=42; zKeap1b MO, n=36; zKeap1a MO + zKeap1 a mRNA, n=40. (B) Quantitation of the relative fold change of AR level (GFP signal) in the tail (left panel) and head (right panel) following bulk NE exposure at 30 hpf (20 μM, 4 h treatment period followed, by image acquisition at 36 hpf). Embryos were (co-)injected with MO and mRNA at 1–4 cell stage (2 nL of 0.25 mM zKeap1b-ATG-MO and 250 ng/μL Halo-TEV-zKeap1b mRNA). Sample sizes analyzed: Control MO, n=42; zKeap1b MO, n=39; zKeap1a MO + zKeap1 a mRNA, n=32. Fish age: 34 hpf. All numerical data present mean ± sem. Numbers above the bars represent analysis by two-tailed t-tests.
-
Figure 2—figure supplement 2—source data 1
Quantification results.
- https://cdn.elifesciences.org/articles/83373/elife-83373-fig2-figsupp2-data1-v3.xlsx
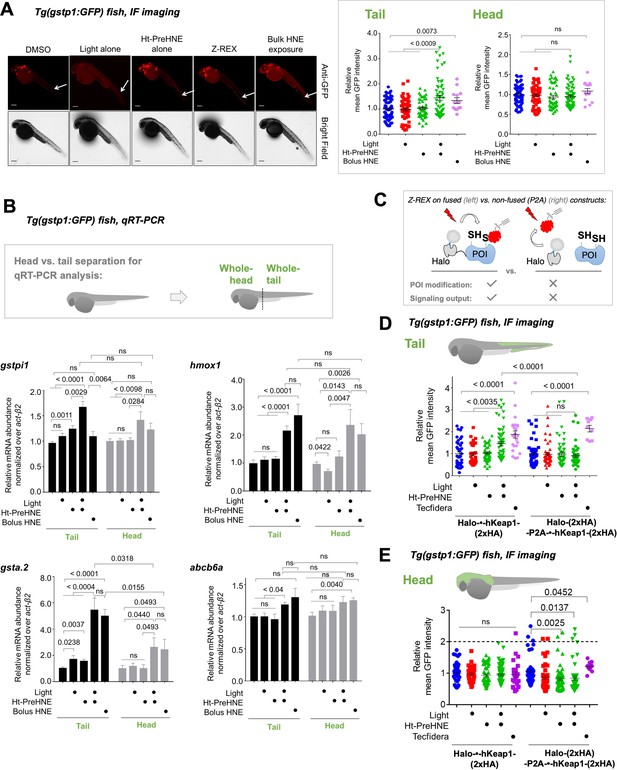
Fish expressing Halo-•-hKeap1-(2xHA) can mount AR following bolus-electrophile dosing or Z-REX-mediated hKeap1-specific electrophile labeling.
(A) Tg(gstp1:GFP) heterozygotes injected with Halo-•-hKeap1-(2xHA) mRNA at one- to four-cell stage. (See Appendix 1-Scheme 1 for workflow). Left: Representative IF-images of Tg(gstp1:GFP) fish 4 hr post Z-REX show an increase in GFP-signal intensity in the tail (arrows) subsequent to Z-REX-mediated Keap1-specific HNEylation. No AR activation was observed with all Z-REX controls (DMSO-treated, light alone, and Ht-PreHNE alone). [Note: GFP-expression was detected using red fluorescence because of high background signal in GFP (ex: 488 nm; em: 520–550 nm) channel]. Right: Image quantitation was performed on the head/tail-regions illustrated in Figure 1B. No. embryos analyzed: DMSO: No light (65), light (61); photocaged probe Ht-PreHNE: No light (47), with light (59); HNE (13). Also see Figure 1—figure supplements 1C and 2A, Figure 3—figure supplement 1. (B) Z-REX-targeted Keap1-specific HNEylation is sufficient to upregulate endogenous AR-genes represented by gstpi1, gsta.2, hmox1, and abcb6a (see Appendix). 2 hr post Z-REX or bolus HNE treatment, embryos were euthanized, RNA was isolated separately from head and tail and qRT-PCR analyses were performed as described in Methods. Inset above shows whole-head/-tail separation performed prior to RNA isolation. See, for workflow, Appendix 1-Scheme 1. n>4 independent biological replicates and 2 technical repeats for each sample. Also see Figure 1—figure supplement 2B. (C) Illustration of a ‘perfect’ negative control for Z-REX using the non-fused construct that allows Halo and POI (protein of interest) to be expressed separately in vivo. See text for discussions. Replicating T-REX/Z-REX using the non-fused construct (here, P2A construct) results in ablation of POI modification by LDE as well as ablation of downstream signaling that are otherwise observed using the fused Halo-POI construct. Also see Figure 3D–E and Figure 3—figure supplement 2. (D) Z-REX-mediated AR-upregulation in the tail is observed only in Halo-•-hKeap1-(2xHA)-fusion-protein-expressing fish embryos, but not in the non-fused construct [i.e. Halo-(2xHA)-P2A-•-hKeap1-(2xHA)-mRNA]-injected embryos (see Appendix for mRNA sequence). See also Figure 3C and Figure 3—figure supplement 2. Bolus treatment of embryos expressing either construct with Tecfidera (Figure 1—figure supplement 1B) results in AR-upregulation in the tail. Image quantitation was performed on the tail-regions as illustrated. No. embryos analyzed: Halo-•-hKeap1-(2xHA): DMSO (43), Light alone (29), Ht-PreHNE alone (47), Z-REX (58), and Tecfidera (24); Halo-(2xHA)-P2A-•-hKeap1-(2xHA): DMSO (55), Light alone (49), Ht-PreHNE alone (52), Z-REX (47), and Tecfidera (9). See also Figure 3—figure supplement 2B. (E) Z-REX-mediated-AR-upregulation is not observed in the head. The dashed line indicates the average level of AR-upregulation in the tail following bulk exposure to Tecfidera (Figure 1—figure supplement 1B). Image quantitation was performed on the head as illustrated. No. embryos analyzed: Halo-•-hKeap1-(2xHA): DMSO (43), Light alone (29), Ht-PreHNE alone (49), Z-REX (65), and Tecfidera (24); Halo-(2xHA)-P2A-•-hKeap1-(2xHA): DMSO (55), Light alone (48), Ht-PreHNE alone (54), Z-REX (49), and Tecfidera (10). All numerical data present mean ± sem. Numbers above the bars represent analysis by two-tailed t-tests.
-
Figure 3—source data 1
Quantification results.
- https://cdn.elifesciences.org/articles/83373/elife-83373-fig3-data1-v3.xlsx
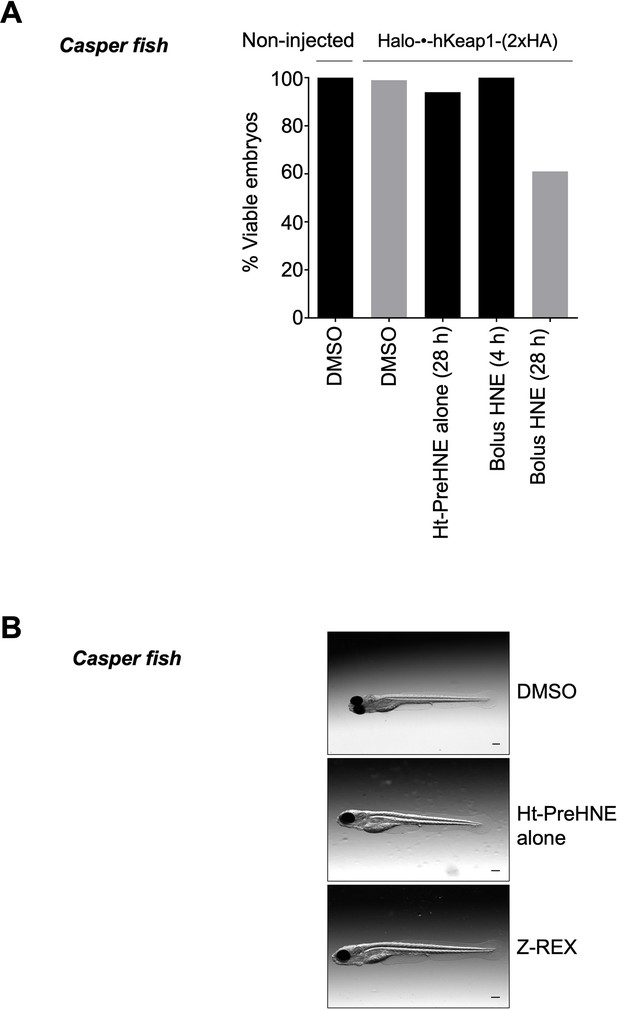
Z-REX execution maintains viability and proper development of embryos, whereas bolus LDE treated embryos show reduced viability following prolonged incubation.
See also Figure 5—figure supplement 1A. (A) Photocaged precursor compounds deployed in Z-REX are non-invasive, whereas fish viability is significantly affected by bolus electrophile treatment at one- to four-cell stage. Representative data shown for HNE (bolus) and Ht-PreHNE (Z-REX). Ht-PreHNE does not show any significant toxicity/teratogenicity compared to non-injected or DMSO-treated fish, despite prolonged treatment Sant et al., 2017. Number of embryos analyzed: Non-injected (30), Halo-•-hKeap1-(2xHA) injected: DMSO (101), Ht-PreHNE alone (72), Bolus HNE (31), Bolus HNE treated at one- to four-cell-stage (37). (B) Z-REX is non-teratogenic. Fish embryos were treated with 6 μM Ht-PreHNE at one- to four-cell stage. After 28.5 hr, fish were exposed to light for 4 min, and subsequently allowed to develop for another 72 hr before imaging. These fish were compared to photocaged compound-alone-treated (i.e. no light exposure) and DMSO-alone-treated fish.
-
Figure 3—figure supplement 1—source data 1
Quantification results.
- https://cdn.elifesciences.org/articles/83373/elife-83373-fig3-figsupp1-data1-v3.xlsx
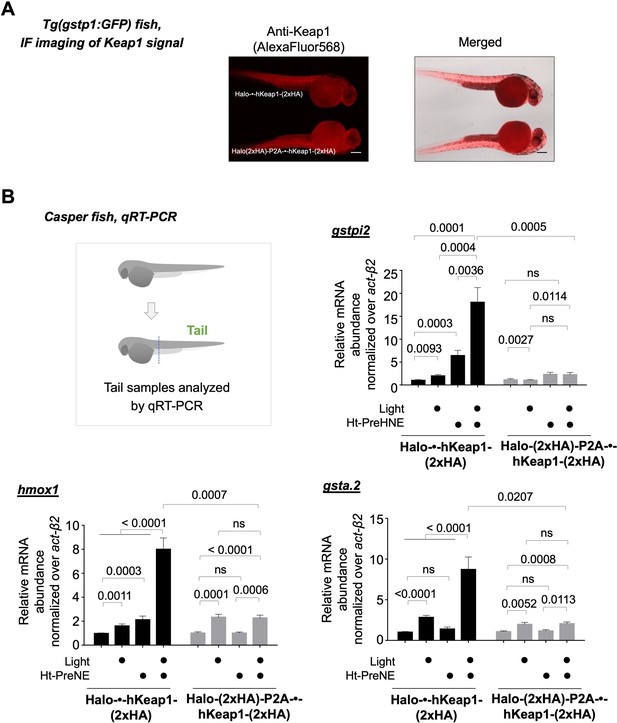
Comparison of results from Z-REX using fused vs non-fused Halo-POI constructs (see Figure 3C) allows precise measurement of ‘on-target/on-pathway’ responses in vivo.
(A) Fused vs. non-fused-construct validations: similar levels of Keap1 ubiquitous expression are obtained in Halo-•-hKeap1-(2xHA) and Halo-(2xHA)-P2A-•-hKeap1-(2xHA) mRNA injected fish. Appendix lists the gene sequences of Halo-•-hKeap1-(2xHA) proteins in fused and non-fused systems. (B) Replication of Z-REX using non-fused construct (see Figure 3C) rules out off-target responses. Inset: Whole-head/-tail separation was performed as indicated (see top left), prior to RNA isolation selectively from the tails. qRT-PCR analysis evaluating upregulation of a representative AR-driven endogenous gene in fish demonstrates Z-REX-mediated Keap1-modification-specific AR-upregulation. 2 hr post Z-REX, embryos expressing either Halo-•-hKeap1-(2xHA) or Halo-(2xHA)-P2A-•- hKeap1-(2xHA) were euthanized, and RNA was isolated separately from head and tail. qRT-PCR was performed on tail samples for gstpi2. n>4 independent biological replicates and two technical repeats. All numerical data present mean ± sem. Numbers above the bars represent analysis by two-tailed t-tests.
-
Figure 3—figure supplement 2—source data 1
quantification results.
- https://cdn.elifesciences.org/articles/83373/elife-83373-fig3-figsupp2-data1-v3.xlsx
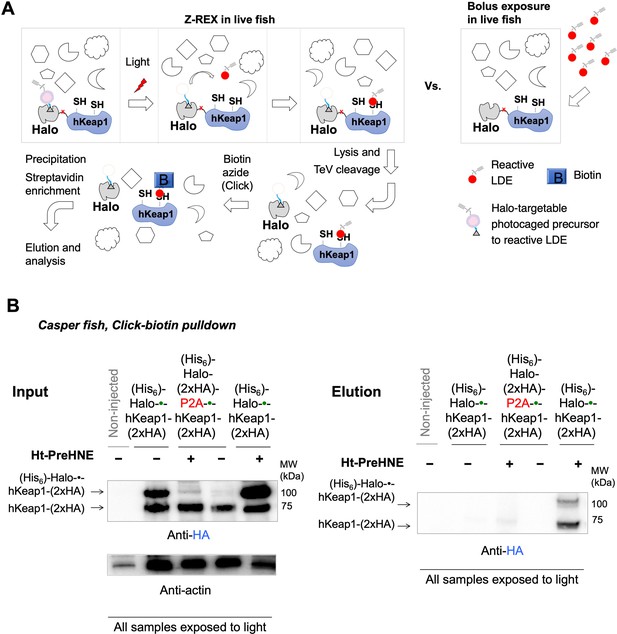
Z-REX on-target electrophile modification strategy validates proximity-directed electrophile responsivity.
(A) Comparison of results from Z-REX using fused vs non-fused Halo-POI constructs (see Figure 3C) allows precise measurement of ‘on-target’ electrophile modification in vivo, because fusion of Halo and Keap1 domains is required for Z-REX-mediated targeted electrophile delivery to Keap1 Parvez et al., 2016; Long et al., 2017c; Van Hall-Beauvais et al., 2018. Left panel: Workflow for electrophile-labeling analysis of protein of interest (POI), following Z-REX in vivo or bolus treatment of live embryos. Procedures involve biotin/streptavidin enrichment of LDE-modified proteins from fish lysate following Z-REX in vivo. Post Z-REX, zebrafish larvae are lysed, and Halo and hKeap1 domains separated using TeV protease. Click coupling with biotin azide in lysate biotinylates the alkyne handle on the LDE-modified POI, and the alkyne handle within the photocaged-precursor bound to Halo (if/when photouncaging is incomplete post light illumination). Enrichment using streptavidin agarose beads and subsequent western blot analysis evaluates extent of Keap1 modification. Right panel: Similar analysis is performed on fish directly treated with reactive LDEs. (B) Using procedure in (A) post Z-REX shows LDE-modification only when Halo-•-hKeap1-(2xHA) is expressed as a fusion protein. No labeling of hKeap1 is observed in fish injected with Halo-(2xHA)-P2A-•-hKeap1-(2xHA) mRNA. This result is representative of an experiment repeated three independent times, by three different operators, yielding identical results. (Note: each sample in pulldown analysis typically requires 75–150 fish, yielding 120–300 μg of fish lysates). Actin is used as loading control in input samples. The upper band around 110 kDa observable in certain samples in the ‘Input’ blot (left panel) corresponds to the full-length fusion protein. This band is variable due to adventitious protease cleavage post lysis. A faint signal corresponding to the same band is observed in the ‘Elution’ blot (right panel) (only in the Z-REX samples originating from fusion protein) due to incomplete photouncaging in vivo (i.e. alkyne from the remaining photocaged probe bound to Halo domain within the full-length Halo-•-hKeap1-(2xHA)), leading to simultaneous enrichment of this full-length protein (~110 kDa) upon biotin pulldown along with HNEylated TEV-cleaved Keap1 (~70 kDa). Note: association between Keap1 and its binding partners is not possible since washes and Click assays are carried out under denaturing conditions. Appendix lists the gene sequences of Halo-•-hKeap1-(2xHA) proteins in fused and non-fused systems.
-
Figure 3—figure supplement 3—source data 1
Full view blot image.
- https://cdn.elifesciences.org/articles/83373/elife-83373-fig3-figsupp3-data1-v3.zip
-
Figure 3—figure supplement 3—source data 2
Raw blot image.
- https://cdn.elifesciences.org/articles/83373/elife-83373-fig3-figsupp3-data2-v3.zip
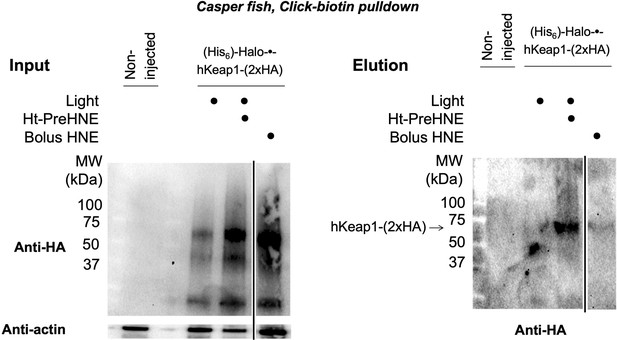
Markedly contrasting to Z-REX (i.e., combined treatment with both light and Ht-PreHNE), wherein liberated LDE efficiently labels hKeap1 in situ, the extent of hKeap1 labeling upon bolus HNE-treatment of fish is minimal.
Bolus HNE treatment of embryos expressing Halo-•-hKeap1-(2xHA) does not label hKeap1 efficiently, despite showing increase in AR and general upregulation of AR-controlled downstream genes (e.g. Figure 3A–B). MW: Halo-•-hKeap1-(2xHA) (~110 kDa), hKeap1-(2xHA) (~70 kDa). See Figure 3—figure supplement 3A for workflow of biotin pulldown (details are described in Methods).
-
Figure 3—figure supplement 4—source data 1
Full view blot image.
- https://cdn.elifesciences.org/articles/83373/elife-83373-fig3-figsupp4-data1-v3.zip
-
Figure 3—figure supplement 4—source data 2
Raw blot image.
- https://cdn.elifesciences.org/articles/83373/elife-83373-fig3-figsupp4-data2-v3.zip
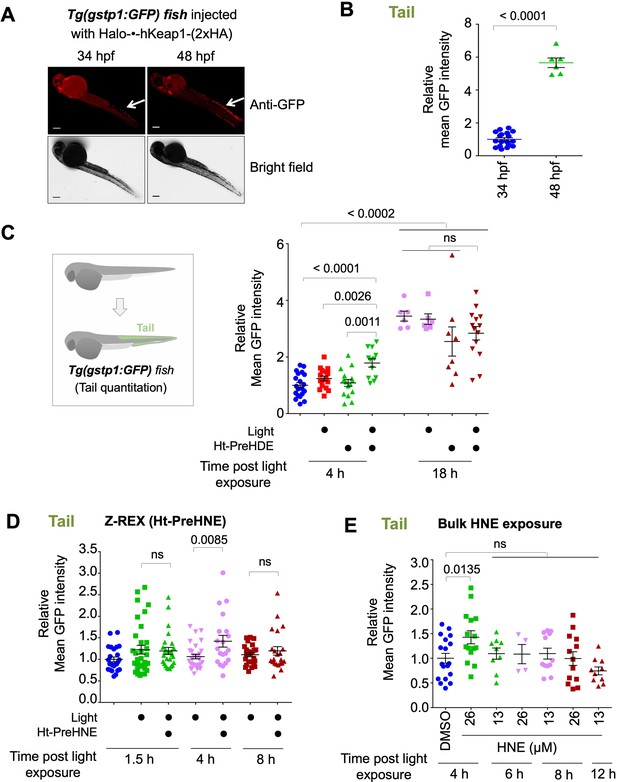
Z-REX-mediated AR upregulation following hKeap1-specific low-occupancy LDE-modification, is transient.
(A) IF in Tg(gstp1:GFP) fish shows basal AR is higher in the tail of larvae at 48 hpf compared to 34 hpf. (B) Quantitation of mean AR in the tails of embryos at 34 hpf and 48 hpf. 34 hpf: n=18, 48 hpf: n=6. (C) Selective modification of hKeap1 with HDE elicits AR upregulation at 4 hr post Z-REX (34 hpf) in the tails of zebrafish embryos. By contrast, at 18 h post Z-REX (48 hpf), no significant difference in AR upregulation is observed between controls and Z-REX. Number of fish evaluated: 18 hr post Z-REX, DMSO: No light (20), with light (16); Ht-PreHDE: no light (15), with light (12); 18 hr post Z-REX, DMSO: No light (6), with light (6); Ht-PreHDE: no light (8), with light (14). (D) Latency of AR upregulation post Z-REX. Tg(gstp1:GFP) fish were injected with mRNA encoding Halo-•-hKeap1-(2xHA). Fish were treated with Ht-PreHNE. The wash-out of Ht-PreHNE and light shining was staggered such as the endpoint of each condition was the same (34 hpf). 1.5 hr: DMSO (25), Light alone (34), Z-REX (26); 4 hr: Light alone (29), Z-REX (20); 8 hr: Light alone (24), Z-REX (22). (E) Same as in (D) except embryos were directly treated with two different concentrations of HNE for the indicated duration. Treatment was staggered such that the endpoint of each condition was the same. DMSO (38); HNE: 26 µM, 4 hr (15); 13 µM, 6 hr (9); 26 µM, 6 hr (4); 13 µM, 8 hr (12); 26 µM, 8 hr (13); 13 µM, 12 hr (10). All numerical data present mean ±sem. Numbers above the bars represent analysis by two-tailed t-tests.
-
Figure 3—figure supplement 5—source data 1
Quantification results.
- https://cdn.elifesciences.org/articles/83373/elife-83373-fig3-figsupp5-data1-v3.xlsx
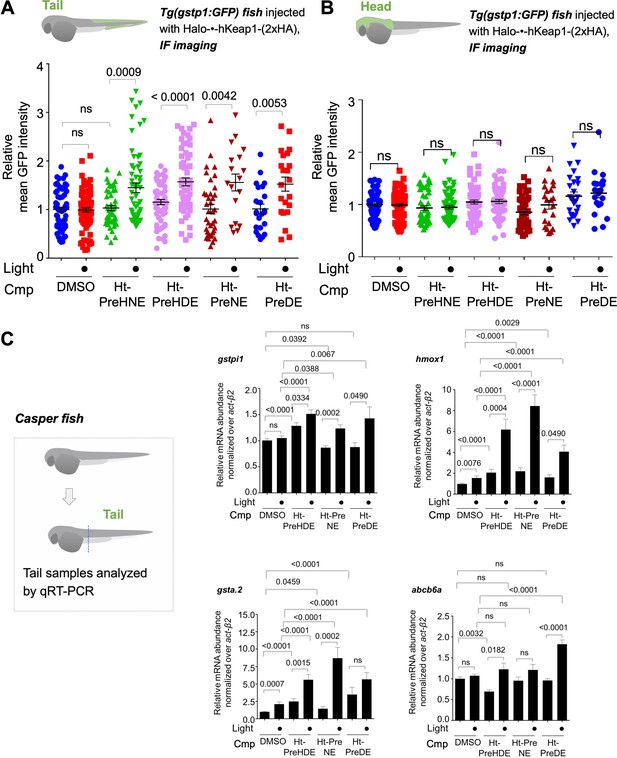
Z-REX delivery of 4-different electrophiles studied consistently labels hKeap1 and activates AR to similar extent (as previously observed in cell culture).
Also see Figures 5–6 and Figure 3—figure supplement 1, Figure 3—figure supplements 3–5, Figure 4—figure supplement 1, Figure 5—figure supplement 1. (A) Quantitation of mean AR-levels in the tail of embryos 4 h post Z-REX with indicated LDEs. Image quantitation was performed on the tail-regions as illustrated. No. embryos analyzed: DMSO: No light (65), with light (84); Ht-PreHNE: No light (47), with light (59); Ht-PreHDE: No light (59), with light (59); Ht-PreNE: No light (38), with light (18); Ht-PreDE: No light (23), with light (22). (B) Similar quantitation in the head shows no increase in AR post Z-REX. Image quantitation was performed on the head-regions as illustrated. No. embryos analyzed: DMSO: No light (65), with light (82); Ht-PreHNE: No light (49), with light (65); Ht-PreHDE: No light (63), with light (60); Ht-PreNE: No light (38), with light (21); Ht-PreDE: No light (23), with light (22). (C) hKeap1-modification alone is sufficient to drive endogenous AR-gene upregulation in the tail in casper zebrafish. Whole-head/-tail separation was performed as indicated in inset (left), prior to RNA isolation selectively from the tails. 2 h post Z-REX with indicated LDEs, embryos were euthanized, and RNA was isolated, and qRT-PCR analyses were performed on tail samples (see inset, left) targeting indicated downstream genes (see Appendix for primer sequences). n>4 independent biological replicates and 2 technical repeats for each sample. Inset: schematic for fish separation. Note: tail was taken as a representative segment in these experiments. All numerical data present mean ± sem. Numbers above the bars represent analysis by two-tailed t-tests.
-
Figure 4—source data 1
Quantification results.
- https://cdn.elifesciences.org/articles/83373/elife-83373-fig4-data1-v3.xlsx
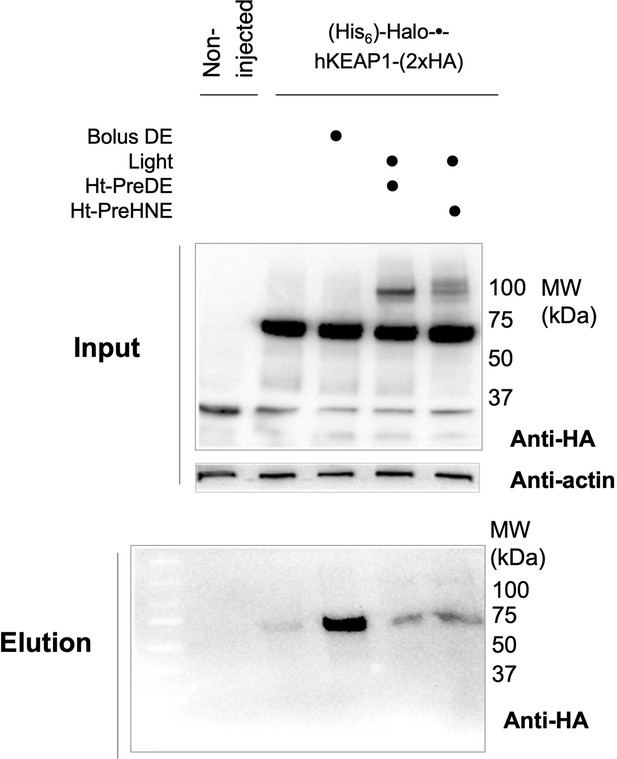
DE efficiently labels hKeap1 upon bolus treatment of whole embryos Cf. results from bolus treatment with 4-OH-bearing LDEs, such as HNE (Figure 3—figure supplement 4).
By contrast, Z-REX shows uniform labeling of hKeap1 irrespective of the LDE structure (see also Figure 3—figure supplements 3B and 4). For LDE-permeability assessment and whole-reactive-proteome labeling by various LDEs following bolus treatment, see Figure 6.
-
Figure 4—figure supplement 1—source data 1
Full view blot image.
- https://cdn.elifesciences.org/articles/83373/elife-83373-fig4-figsupp1-data1-v3.zip
-
Figure 4—figure supplement 1—source data 2
Raw blot image.
- https://cdn.elifesciences.org/articles/83373/elife-83373-fig4-figsupp1-data2-v3.zip
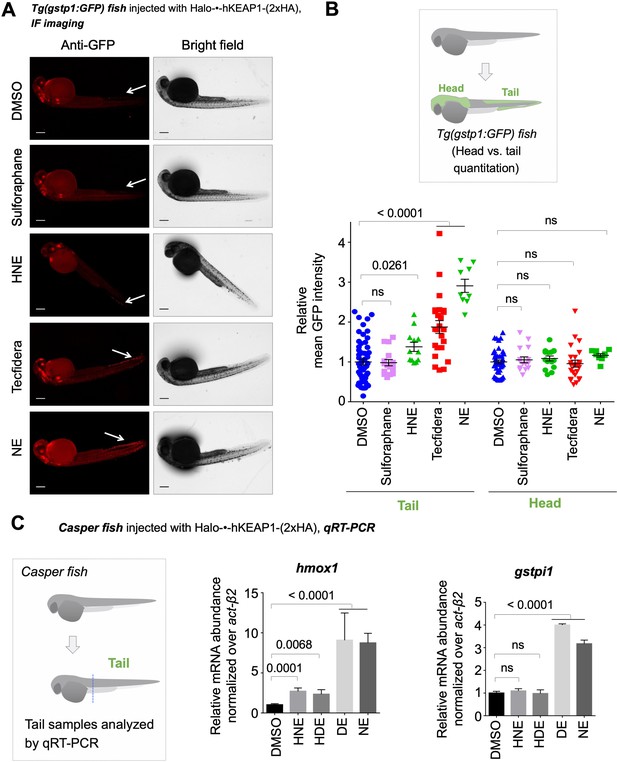
Bolus dosing with different LDEs or reactive covalent electrophilic drugs elicits complex AR responses.
Also see Figure 6, Figure 3—figure supplements 1 and 4 and 5E, Figure 4—figure supplement 1, and Figure 5—figure supplement 1. (A) Representative IF images of Tg(gstp1:GFP) fish expressing Halo-•-hKeap1-(2xHA), following bolus exposure to indicated electrophiles. (B) Quantitation of data in (A). Image quantitation was performed on the head/tail-regions as illustrated. No. embryos analyzed: Tail, DMSO (55), Sulforaphane (16), HNE (12), Tecfidera (24), NE (9); Head, DMSO (43), Sulforaphane (16), HNE (15), Tecfidera (24), NE (9). Sulforaphane and HNE elicit non-significant and 1.5-fold AR upregulation, respectively. Tecfidera gives medium (~2-fold) AR response and NE elicits the strongest AR upregulation (~3-fold) in tail. Consistent with data elsewhere (e.g., Figure 3A and C-E), head is not responsive. (C) qRT-PCR analysis of endogenous AR-responsive genes following bolus exposure of native reactive LDEs to whole fish similarly shows mixed responses. Whole-head/-tail separation was performed as indicated in inset (left), prior to RNA isolation selectively from the tails. 2 hr post Z-REX, embryos were euthanized, and RNA was isolated separately from tail (see inset, left). Data are presented as mean ± sem. n>3 independent biological replicates and two technical repeats for each sample. All numerical data present mean ± sem. Numbers above the bars represent analysis by two-tailed t-tests.
-
Figure 5—source data 1
Quantification results.
- https://cdn.elifesciences.org/articles/83373/elife-83373-fig5-data1-v3.xlsx
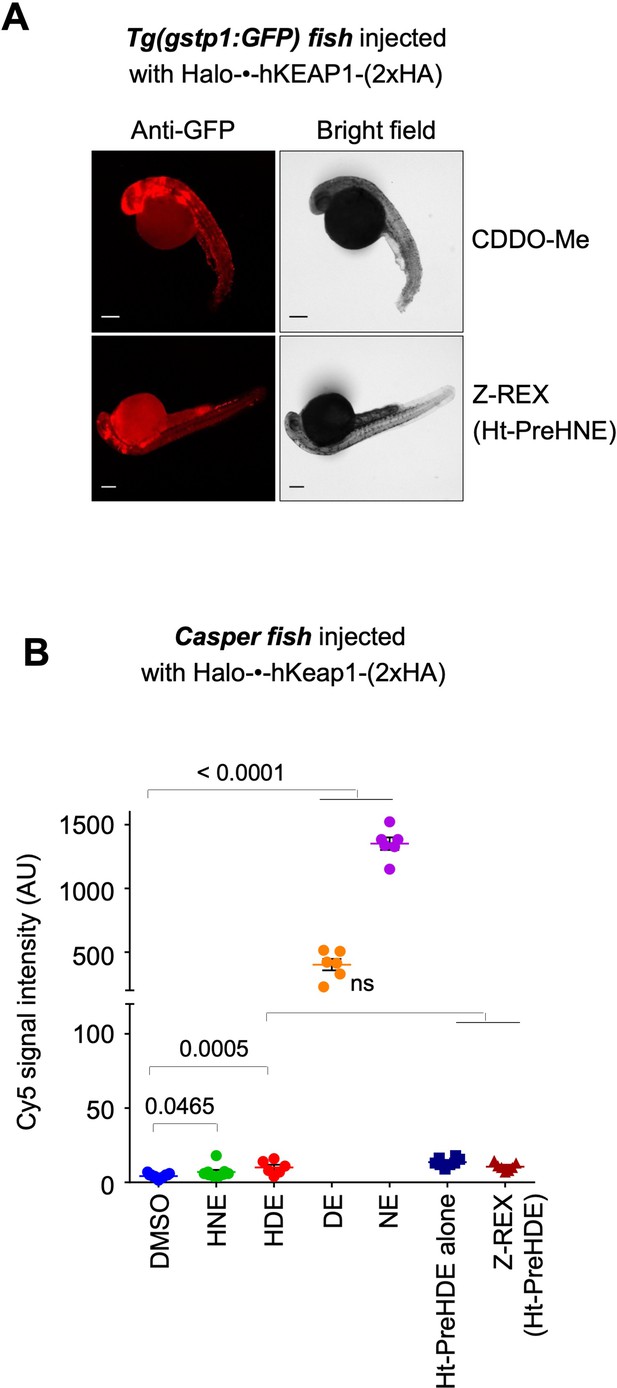
Uncontrolled bulk exposure of developing larvae with reactive electrophiles results in differential proteome labeling extent and adversely affects development/viability.
(A) Treatment with 25 µM CDDO-Me for 4 hr negatively impacts the morphology of the developing embryos (curved tail; malformed/missing caudal fin), whereas Z-REX photocaged probe treatment shows no negative impacts. See also Figure 3—figure supplement 1. Top: CDDO-Me treated, Bottom: Ht-PreNE treated. (B) Comparison of relative whole-reactive-proteome labeling extent by the respective alkyne-bearing small-molecules, following either bolus LDE treatment (2nd to 4th bars) or treatment with Z-REX-photocaged-LDE precursor alone (6th bar) or full Z-REX execution (7th bar) in live embryos. Zebrafish embryos at 32 hpf were directly treated with 25 μM of the indicated LDEs for 2 hr. After fixing and permeabilization, Cy5 azide was conjugated to alkyne labeled proteins using copper-assisted Click procedure as described in Methods. Larvae were imaged after washing away unbound dye. The Cy5 fluorescence intensity was analyzed using Image-J(NIH). Electrophiles devoid of 4-OH group such as DE and NE (4th and 5th bars) (see also Figure 1A inset) efficiently label the whole-reactive-proteome upon bolus treatment of whole embryos, whereas 4-OH-group containing electrophile such as HNE and HDE (2nd and 3rd bars) show a lower degree of whole-reactive-proteome labeling. See Figure 6 for representative images related to the data in 1st to 5th bars. All numerical data present mean ± sem. Numbers above the bars represent analysis by two-tailed t-tests.
-
Figure 5—figure supplement 1—source data 1
Quantification results.
- https://cdn.elifesciences.org/articles/83373/elife-83373-fig5-figsupp1-data1-v3.xlsx
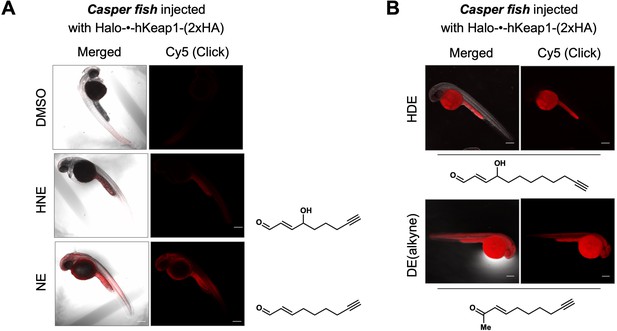
Bolus LDE treatment results in different extent of whole-reactive-proteome labeling that correlates with the magnitude of AR-upregulation (Figure 5A–C).
See also Figure 5—figure supplement 1. (A) Casper zebrafish were treated with indicated LDEs for 2 hr before euthanasia and fixing. See Methods for whole-reactive-proteome labeling procedure in fish using Click coupling. (B) Comparison of whole-reactive-proteome labeling extent of HDE and DE over time (2 hr) shows allylic alcohol motif within LDEs is likely responsible for reduced Keap1-labeling degree under bolus conditions.
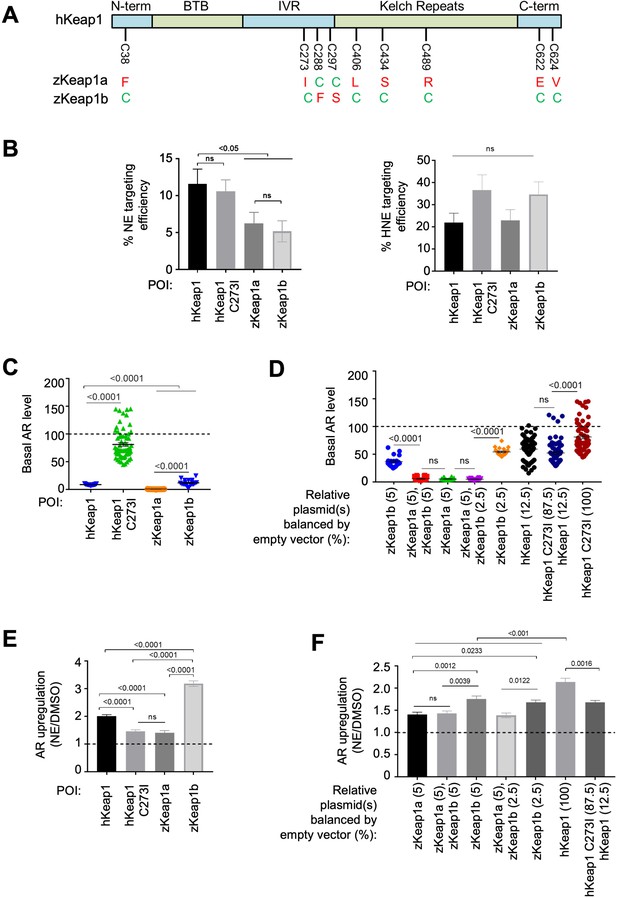
Cell-based studies of zKeap1a and zKeap1b recapitulate the dominant-negative behavior observed in developing embryos; cell-based T-REX analysis (Figure 1—figure supplement 1A) reveal similar electrophile sensitivity across all Keap1-variants.
See also Figure 8A–B, Figure 7—figure supplements 1–2 and Figure 8—figure supplement 1. (A) The nine cysteines within hKeap1 that are present in only one of the two Keap1 paralogs in zebrafish. (N-term, BTB, IVR, Kelch-Repeats, C-term are individual conserved domains of Keap1). All indicated cysteines are conserved between human and zebrafish Keap1. (B) HEK293T cells were transiently transfected to express indicated Halo-•-Keap1 constructs. (See Figure 1—figure supplement 1D, Figure 7—figure supplement 2A and D for validation of construct functionality). 36 hr post transfection, cells were treated with Ht-PreNE (10 µM, 2 hr), and after rinsing cycles, cells were then exposed to UV light (5 mW/cm2 365 nm lamp). Post lysis, samples were treated with TeV protease and subjected to Click coupling with Cy5-azide. The targeting efficiency of NE on each variant was calculated using a previously-reported procedure Parvez et al., 2016; Van Hall-Beauvais et al., 2018. See Figure 7—figure supplement 2B-C for representative in-gel fluorescence and western blot data. (C) HEK293T cells were transfected with plasmids encoding ARE:Firefly luciferase and CMV:Renilla Firefly reporters, human myc-Nrf2, and Keap1 (Halo-•-hKeap1, Halo-•-(3xFLAG)-zKeap1a, Halo-•-(3xFLAG)-zKeap1b, Halo-•-hKeap1 C273I, or empty vector). (See Figure 1—figure supplement 1D, Figure 7—figure supplement 2A and D for validation of construct functionality). Basal (non-electrophile-stimulated) AR levels were quantified using a standard procedure Parvez et al., 2016; Long et al., 2017b; Van Hall-Beauvais et al., 2018. The horizontal dotted line represents basal AR levels with no exogenous Keap1 introduction. All conditions show a significant drop compared to basal level (i.e. with no exogenous Keap1 overexpression). No. independent biological replicates: Halo-•-hKeap1 (n=65), Halo-•-hKeap1 C273I (n=63), Halo-•-(3xFLAG)-zKeap1a (n=19), Halo-•-(3xFLAG)-zKeap1b (n=19). These were all dosed at a plasmid loading equivalent to 100%. Also see Figure 7—figure supplement 2A and D. (D) HEK293T cells were transfected with a mixture of plasmids encoding Halo-•-(3xF)-zKeap1a and Halo-•-(3xF)-zKeap1b in various ratios this mix also contained empty vector as required, myc-Nrf2, and AR reporter plasmids, see (C) See Figure 1—figure supplement 1D, Figure 7—figure supplement 2A and D for validation of construct functionality. 36 hr post transfection, AR was measured using a standard procedure Parvez et al., 2016; Long et al., 2017b; Van Hall-Beauvais et al., 2018. The horizontal dotted line indicates the basal AR levels in the absence of exogenously-introduced Keap1. Percentages are relative to those analyzed in Figure 7—figure supplement 2D. No. independent biological replicates: n=22 for zKeap1a/b mixing, n=63 for WT/C273I hKeap1 mixing. (E) A similar experiment to (C) except AR in response to NE bolus dosing was measured in HEK293T cells transfected with: Halo-•-hKeap1(WT); Halo-•-(3xFLAG)-zKeap1b; Halo-•-hKeap1(C273I); and Halo-•-(3xFLAG)-zKeap1a. (See Figure 1—figure supplement 1D, Figure 7—figure supplement 2A and D for validation of construct functionality). The horizontal dotted line represents the normalized AR-level for respective Keap1-variants following DMSO-treatment in place of NE. No. independent biological replicates: hKeap1 WT (n=28), hKeap1 C273I (n=28), zKeap1a (n=20), zKeap1b (n=20). (F) A similar experiment to (D), except AR in response to NE bolus dosing was measured in HEK293T cells. (See Figure 1—figure supplement 1D, Figure 7—figure supplement 2A and D for validation of construct functionality). Note: the indicated mix of Halo-•-(3xFLAG)-zKeap1a and Halo-•-(3xFLAG)-zKeap1b upregulated AR to a similar extent as Halo-•-(3xFLAG)-zKeap1a alone. The horizontal dotted line represents the normalized AR-level for respective Keap1-variants following DMSO-treatment in place of NE. Percentages are relative to those described in Figure 7—figure supplement 2D. No. independent biological replicates: n=54 for zKeap1a/b mixing, n=20 for WT/C273I hKeap1 mixing. All numerical data present mean ± sem. Numbers above the bars represent analysis by two-tailed t-tests.
-
Figure 7—source data 1
Quantification results.
- https://cdn.elifesciences.org/articles/83373/elife-83373-fig7-data1-v3.xlsx
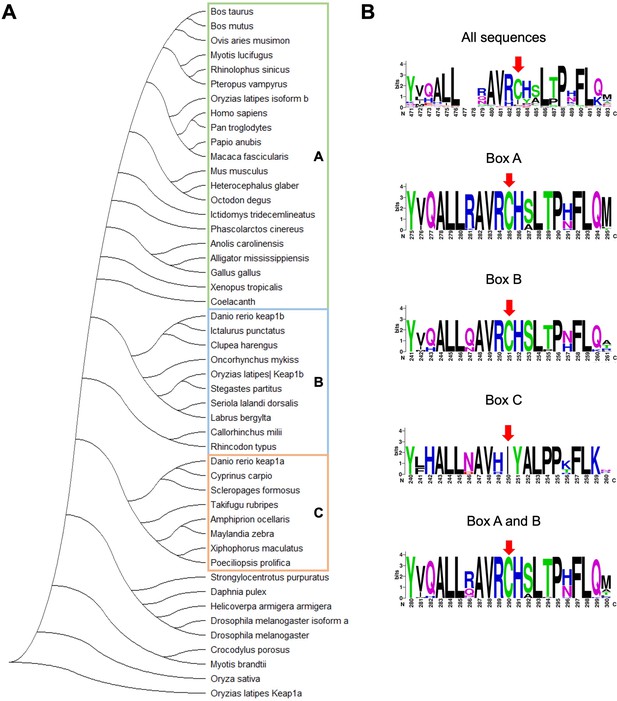
Keap1 is widely conserved and Keap1 variants from fish and human mutants sense NE to a similar extent.
(A) Evolutionary analysis of Keap1 through various species shows C273I conservation. The phylogenetic tree was generated using MEGA-X (Kumar et al., 2018) and the Maximum Likelihood method based on JTT matrix-based model. The tree was generated using Neighbor-Join and BioNJ algorithms to a matrix of pairwise distances estimated using a JTT model. Topology with superior log likelihood value was selected. See (B) for the designation of boxes A-C in color. (B) Sequence logos of the flanking residues of C273 (263–283, human numbering). Various groups indicated by colored boxes in (A) are compared, and the conservation of C273 can be seen in higher level organisms. The relative size of residues is indicative of their frequency. WebLogo (invented by Steven E. Brenner et al, Computational Genomics Research Group, University of California, Berkeley) was used to generate the sequence logos.
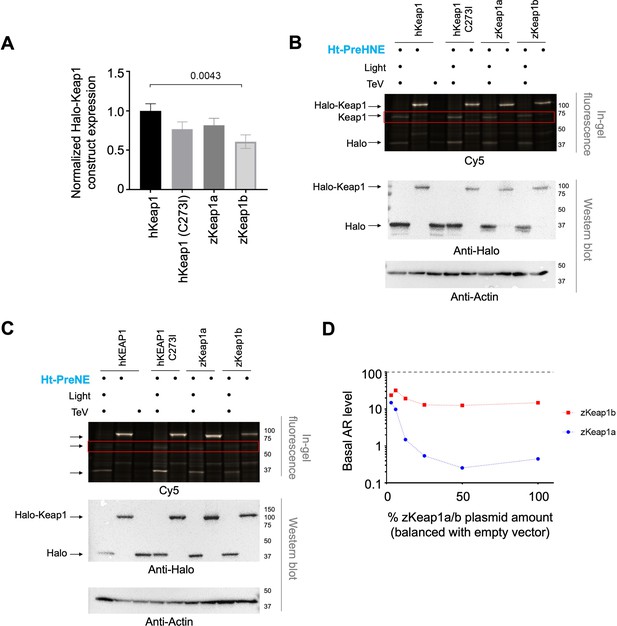
Keap1-paralogs/variants express at similar levels in cells; zKeap1a is an overall more-effective suppressor of basal AR than the other variants; but all manifest similar sensitivity to HNE and NE analyzed by cell-based T-REX assays.
(A) The relative expression levels in HEK293T cells ectopically expressing the indicated Keap1-variants, analyzed by western blot using anti-Halo antibody. Full constructs employed are Halo-•-hKeap1, Halo-•-hKeap1 (C273I), Halo-•-Flag3-zKeap1a, and Halo-•-Flag3-zKeap1b. (B–C) (The box in red emphasizes the region of interest pertaining to electrophile-modified Keap1 post TeV-protease cleavage; also see Figure 7B). Following workflow in Figure 1—figure supplement 1A in live HEK293T cells with the use of either: (B) Ht-PreHNE- or (C) Ht-PreNE-photocaged precursors, the extent of POI-modification by the indicated LDE (HNE in A and NE in B) was assessed by in-gel fluorescence analysis. POI-expression and lysate loading were analyzed by western blots using anti-Halo and anti-actin antibodies, respectively. Full constructs used are Halo-•-hKeap1, Halo-•-hKeap1 (C273I), Halo-•-Flag3-zKeap1a, and Halo-•-Flag3-zKeap1b. [Cmp, compound (photocaged LDE-precursor); TeV, TeV-protease]. (D) When expressed in HEK293T cells, both zebrafish paralogs suppressed basal AR levels, although zKeap1a regulates basal AR more effectively in a plasmid-dose-dependent manner. Neither paralog suppressed AR to undetectable levels. Horizontal dotted line designates basal AR-levels following transfection of empty vector in place of Keap1-encoding-plasmid. All numerical data present mean ± sem. Numbers above the bars represent analysis by two-tailed t-tests.
-
Figure 7—figure supplement 2—source data 1
Full view gel and blot image.
- https://cdn.elifesciences.org/articles/83373/elife-83373-fig7-figsupp2-data1-v3.zip
-
Figure 7—figure supplement 2—source data 2
Raw gel and blot image.
- https://cdn.elifesciences.org/articles/83373/elife-83373-fig7-figsupp2-data2-v3.zip
-
Figure 7—figure supplement 2—source data 3
Quantification results.
- https://cdn.elifesciences.org/articles/83373/elife-83373-fig7-figsupp2-data3-v3.xlsx
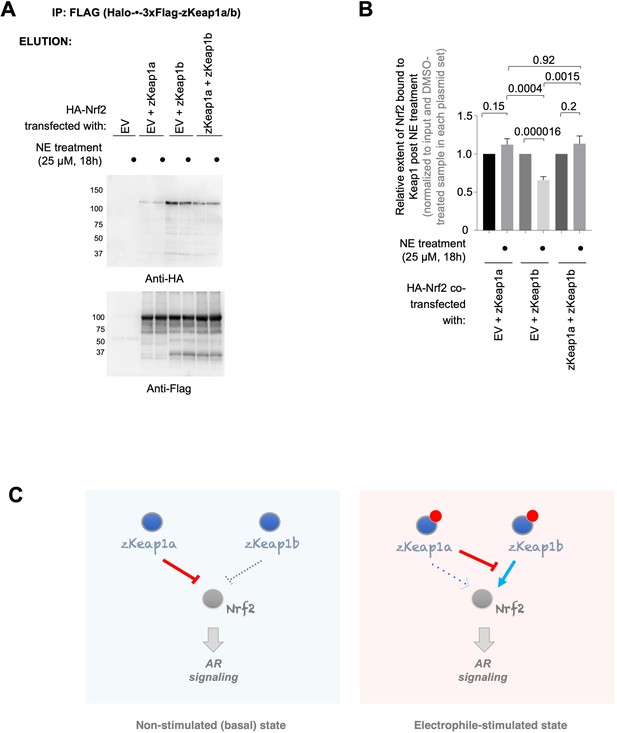
zKeap1a/b paralog-specific AR regulation is reflected by the differences in altered Nrf2 binding following electrophile stimulation.
(A) HEK293T cells were transfected with a plasmid encoding HA-Nrf2 [used because anti-HA-antibody is orthogonal to our anti-FLAG-antibody; and because this anti-HA-antibody (for detecting HA-Nrf2) is of higher sensitivity, compared to anti-myc-antibody to myc-Nrf2], and (an)other plasmid(s) encoding: either a mix of Halo-•–3xFlag-zKeap1a and Halo-•–3xFlag-zKeap1b, Halo-•–3xFlag-zKeap1a and empty vector (EV), Halo-•–3xFlag-zKeap1b and EV, or EV alone. The plasmid amount of HA-Nrf2 was 50% in all co-transfection conditions, and the rest of the co-transfected plasmids made up the other 50% (with equal 1:1 or 1:1:1 contribution from each plasmid, as applicable). Following whole-cell NE treatment (25 µM, 18 hr), normalized cell lysates were treated with anti-Flag M2 resin to evaluate the relative extent of association between zKeap1a/b and Nrf2 following NE stimulation. Representative blots for Elution. The band around ~37 kDa in anti-Flag blot, although of unknown identity, is present almost equally in both ‘zKeap1b’ and ‘zKeap1b+zkeap1 a’ samples, and thus its presence cannot be sufficient to explain the differences observed between these two data sets. See Figure 8—figure supplement 1 for the corresponding Input. (B) Quantification of (A) normalized over input (see Figure 8—figure supplement 1) and corresponding DMSO-treated samples in each set. Right panel: Quantification for Nrf2 association to zKeap1 upon NE bolus treatment for different ratios of zKeap1a:zKeap1b. (n=6 biological replicates). (C) Proposed model illustrating paralog-specific nuanced regulation of cellular antioxidant response (AR) under steady-state vs. electrophile-stimulated conditions. Left panel: under non-electrophile-stimulated conditions, zKeap1a is a more effective antagonist of Nrf2 (and hence, AR-signaling) than zKeap1b. Right: following electrophile stimulation, zKeap1b-modification results in a large upregulation in AR through significantly-reduced binding of Nrf2. By contrast, zKeap1a-modification gives rise to a weaker AR-upregulation, and zKeap1a—likely in the electrophile-modified or -non-modified state—functions as a negative regulator to suppress Nrf2/AR-pathway activation promoted by modified-zKeap1b. See also Figure 8A–B and Figure 8—figure supplement 1. All numerical data present mean ± sem. Numbers above the bars represent analysis by two-tailed t-tests.
-
Figure 8—source data 1
Full view blot image.
- https://cdn.elifesciences.org/articles/83373/elife-83373-fig8-data1-v3.zip
-
Figure 8—source data 2
Raw blot image.
- https://cdn.elifesciences.org/articles/83373/elife-83373-fig8-data2-v3.zip
-
Figure 8—source data 3
Quantification results.
- https://cdn.elifesciences.org/articles/83373/elife-83373-fig8-data3-v3.xlsx
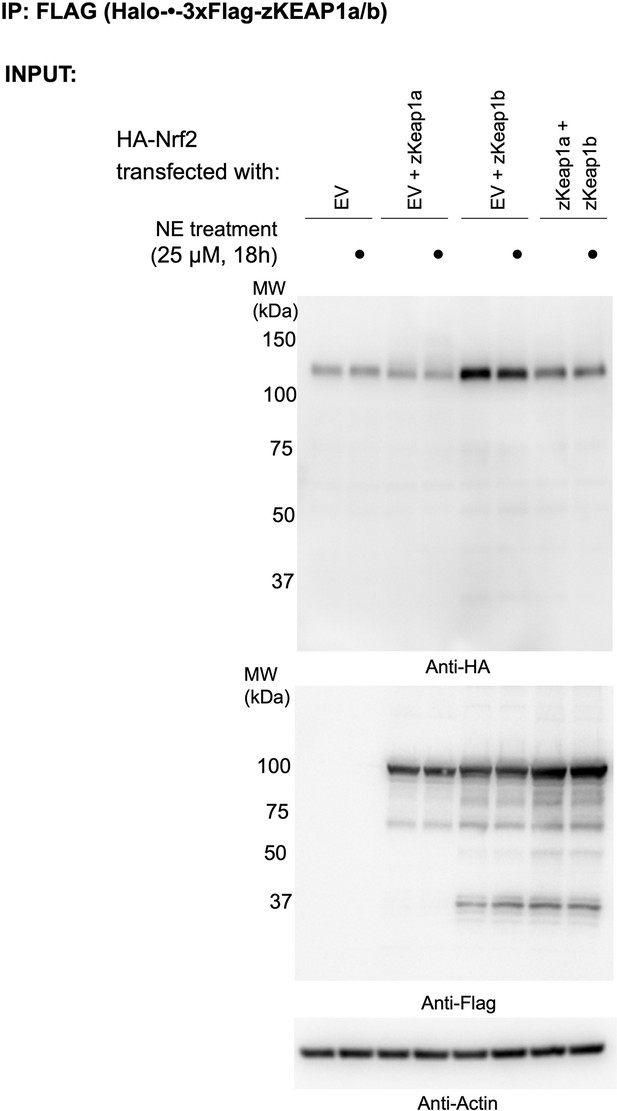
IP assays investigating differential association of zKeap1a and zKeap1b to Nrf2, following electrophile stimulation (see also Figure 8A–B).
HEK293T cells were transfected with a plasmid encoding HA-Nrf2 (used because anti-HA-antibody is orthogonal to our anti-FLAG-antibody; and because this anti-HA-antibody to HA-Nrf2 is of higher sensitivity, compared to anti-myc-antibody to myc-Nrf2 which we have tested before), and (an)other plasmid(s) encoding: either a mix of Halo-•–3xFlag-zKeap1a and Halo-•–3xFlag-zKeap1b, Halo-•–3xFlag-zKeap1a and empty vector (EV), Halo-•–3xFlag-zKeap1b and EV, or EV alone. The plasmid amount of HA-Nrf2 was 50% in all co-transfection conditions, and the rest of the co-transfected plasmids made up the other 50% (with equal 1:1 or 1:1:1 contribution from each plasmid, as applicable). Following whole-cell NE treatment (25 µM, 18 hr), normalized cell lysates were treated with anti-Flag M2 resin to evaluate the relative extent of association between zKeap1a/b and Nrf2 following NE stimulation. Representative blots for Input. Note: The band around ~37 kDa in anti-Flag blot, although of unknown identity, is present almost equally in both ‘zKeap1b’ and ‘zKeap1b+zkeap1 a’ samples, and thus its presence cannot be sufficient to explain the differences observed between these two data sets.
-
Figure 8—figure supplement 1—source data 1
Full view blot image.
- https://cdn.elifesciences.org/articles/83373/elife-83373-fig8-figsupp1-data1-v3.zip
-
Figure 8—figure supplement 1—source data 2
Raw blot image.
- https://cdn.elifesciences.org/articles/83373/elife-83373-fig8-figsupp1-data2-v3.zip
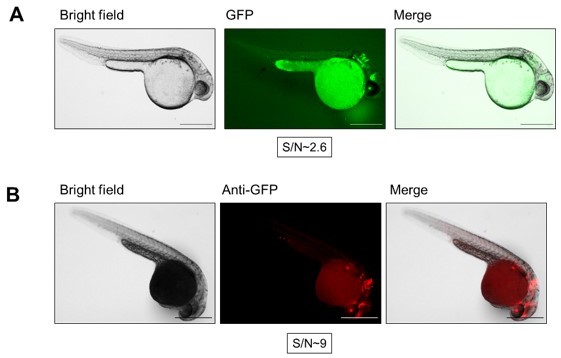
Comparison between live imaging (A) and whole-mount immunofluorescence (IF) (B).
Scale bars, 500 μm. (A) Live Tg(gstp1:GFP) embryos (1.5 dpf, injected with control MOs) were dechorionated and placed on an agarose pad and imaged using GFP fluorescence (Ex. 470 nm, Em. 525 nm) and bright field. Signal to noise (S/N) ratio was estimated by dividing fluorescence intensity of the fluorescent signal by the fluorescence intensity of the surrounding area for 4-6 fluorescent areas using Image-J (NIH). (B) Tg(gstp1:GFP) embryos (1.5 dpf, injected with control MOs) were dechorionated, fixed, and immunostained for GFP as described in the original manuscript. AlexaFluor568 (Ex. 560 nm, Em. 630 nm) channel shows GFP signal. GFP localization reported by GFP intrinsic fluorescence [in (A)] is similar to that reported by red fluorescence from IF in this figure. S/N ratio was calculated as in (A).

Endogenous human Keap1/Nrf2 expression does not interfere with AR measurement in cell-based assays.
(A) Left, Expression of ectopic Keap1 (labeled here as ‘HTK’, designating the construct used: Halo-TeV-Keap1) is large relative to endogenous hKEAP1 present in cells. HEK293T cells were transfected as indicated in the dual luciferase assay described in Figure 7C-F (original manuscript) and after 42 hours harvested for western blotting. Right, quantification of endogenous (blue bars) and overexpressed Keap1 (red bars) for each transfection condition from n=6 replicates. The affinity of the antibody to each Keap1 variant (Supp. Figure 1D, original manuscript) was used to normalize the Keap1 signal for zKeap1a and zKeap1b. The horizontal dotted line represents the average level of overexpressed hKEAP1. (B) Left, introduction of ectopic Nrf2 alone increases the AR signal by 1000-fold (note: maximal AR is normalized to 100; lowest AR is 0.1). Center and Right; using the dual luciferase assay described in Figure 7C-F (original manuscript), two different siRNAs targeting endogenous hKEAP1 were used to reduce endogenous hKEAP1 levels, while the cells were also transfected to express hNrf2 (Graph at center) only, or low levels of zKeap1a/b (Graph on right), using transfection conditions as in Figure 7D,F (original manuscript). siRNAs have little effect on AR output in either condition. si(control) i, ii, iii correspond to Santa Cruz siRNA controls “A, C, and E (vendor’s labels)”, respectively. si(hKEAP1) i and ii correspond to Dharmacon siGENOME human KEAP1 siRNAs D-012453-03 and D-012453-04. Dharmafect Duo was used for transfection. (C) Left, representative blot for siRNA knockdown efficiency assessment under assay conditions deployed in the original manuscript (data shown is from a single continuous blot); Right, quantification (n=4). Horizontal dotted line represents average level of Keap1 signal of all siControls.
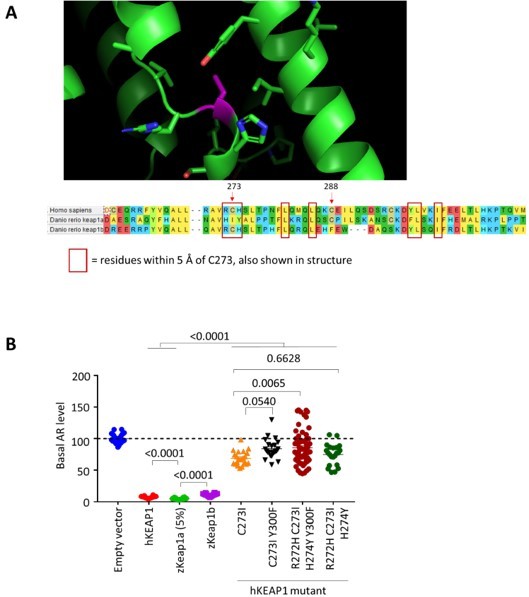
Initial explorations regarding potential rescue of basal AR-activity of hKEAP1 C273I through mutation.
(A) Top; No full-length hKEAP1 crystal structure is available, therefore crystal structure of human Kelch-like protein-11 (PDB: 4AP2) was used as a model onto which the hKEAP1 sequence was threaded using Swiss-Prot. Bottom; Amino acid sequence alignment (performed using MEGA-X) between hKEAP1, zKeap1a, and zKeap1b. Residues with side chains within 5 Å of C273 (magenta) are shown and boxed in red in the sequence alignment shown below. These residues served as putative residues for potentially rescuing the basal AR-activity of hKEAP1 C273I. (B) The cell-based AR assay from Figure 7 C-F (original manuscript) was used to determine the extent of basal AR suppression elicited by each double/triple mutant. C273I is the single point mutant that recapitulated zKeap1a behavior but lost the ability to suppress basal AR to the same extent as hKEAP1 WT can do. The double, quadruple, and triple mutants (last three sets of hKEAP1-mutant on graph) introduce additional zKeap1a residues into hKEAP1 C273I.

Comparison of GFP immunofluorescence (IF) staining between WT and transgenic fish.
WT (top) or Tg(gstp1:GFP) (bottom) embryos (1.5 dpf, injected with control MOs) were dechorionated, fixed, and immunostained for GFP as described in the original manuscript. Scale bars, 500 μm.
Tables
Reagent type (species) or resource | Designation | Source or reference | Identifiers | Additional information |
---|---|---|---|---|
Strain, strain background (Escherichia coli) | XL10-Gold Ultracompetent Cells | Agilent Technologies | Cat# 200315 | N/A |
Cell line (Homo-sapiens) | 293T (ATCC CRL-3216) | ATCC | Cat# CRL-3216, RRID:CVCL_0063 | https://www.atcc.org/products/crl-3216 |
Genetic reagent (Danio rerio) | Tg(–3.5gstp1:GFP)/it416b | Japanese National BioResource Project | N/A | https://shigen.nig.ac.jp/zebra/index_en.html |
Genetic reagent (Danio rerio) | Brian’s wildtype | Professor Joseph Fetcho’s lab (Cornell University) | N/A | A strain of wt-zebrafish that shows low pigmentation |
Genetic reagent (Danio rerio) | Wild-type line Casper | Professor Joseph Fetcho’s lab (Cornell University) | PMCID:PMC2292119 | N/A |
Recombinant DNA reagent | pGL4.37[luc2P/ARE/Hygro] | Promega | Cat# E3641 | N/A |
Recombinant DNA reagent | pGL4.75[hRluc/CMV] | Promega | Cat# E6931 | N/A |
Recombinant DNA reagent | pCDNA3 myc-Nrf2 | Addgene | Cat# 21555, RRID:Addgene_21555 | N/A |
Recombinant DNA reagent | pCS2 +8 vector | Addgene | Cat# 34931, RRID:Addgene_34931 | N/A |
Recombinant DNA reagent | pFN21a Halo-TEV-Keap1 | Halo ORFeome, Promega Kazusa collection | Cat# FHC00420 | N/A |
Antibody | Anti-GFP (FITC) (goat polyclonal) | Abcam | Cat# ab6662, RRID: AB_305635 | IF (1:1000) |
Antibody | Anti-Flag (mouse monoclonal) | Sigma | Cat# F3165, RRID:AB_259529 | WB (1:4000) |
Antibody | Anti-Keap1 (mouse monoclonal) | Abcam | Cat# ab119403, RRID: AB_10903761 | IF (1:500) |
Antibody | Anti-HaloTag (rabbit polyclonal) | Promega | Cat# G9281, RRID:AB_713650 | WB (1:3000) |
Antibody | Anti-HA HRP (mouse monoclonal) | Sigma | Cat# H3663, RRID: AB_262051 | IF and WB (1:500) |
Antibody | Anti-β-actin HRP (mouse monoclonal) | Sigma | Cat# A3854, RRID: AB_262011 | WB (1:30,000) |
Antibody | Anti-mouse-HRP (horse polyclonal) | Cell Signaling | Cat# 7076, RRID:AB_330924 | WB (1:2000) |
Antibody | Anti-rabbit-HRP (goat polyclonal) | Cell Signaling | Cat# 7074, RRID:AB_2099233 | WB (1:2000) |
Antibody | Anti-goat IgG H&L (AlexaFluor568) (rabbit polyclonal) | Abcam | Cat# ab175707, RRID: AB_2923275 | IF (1:2000) |
Antibody | Anti-rat IgG H&L (AlexaFluor568) (donkey polyclonal) | Abcam | Cat# ab175475, RRID: AB_2636887 | IF (1:500) |
Antibody | Anti-mouse IgG H&L (AlexaFluor568) (donkey polyclonal) | Abcam | Cat# ab175472, RRID: AB_2636996 | IF (1:1000) |
Antibody | Anti-mouse IgG H&L (AlexaFluor647) (donkey polyclonal) | Abcam | Cat# ab150107, RRID:AB_2890037 | IF (1:1000) |
Control MO1: | Keap1a+ Keap1b MOs: |
---|---|
0.718147 | 1.207962 |
0.878549 | 0.994521 |
0.948409 | 1.913047 |
1.186984 | 1.378654 |
0.884733 | 0.940147 |
2.546936 | 1.047857 |
0.951229 | 1.642165 |
0.754858 | 1.062155 |
0.851931 | 1.152548 |
0.92753 | 0.849358 |
0.824372 | 1.882668 |
0.846983 | 2.276351 |
0.881814 | 0.776578 |
0.835752 | 1.685852 |
1.077839 | 1.445002 |
1.096838 | 1.091 |
0.561753 | 0.992839 |
0.978788 | 1.430555 |
1.246553 | 1.413931 |
0.439883 | 0.520341 |
0.74971 | 2.35952 |
0.914093 | 1.018022 |
1.073679 | 1.400721 |
1.111666 | 1.259565 |
1.065044 | 1.550881 |
0.872091 | 1.638009 |
1.359449 | 1.074574 |
1.50905 | 1.613914 |
1.068633 | 1.139783 |
0.718333 | |
0.920063 | |
1.123783 | |
0.880229 | |
1.310766 | |
1.520599 | |
0.810581 | |
1.49313 | |
0.776006 |