Live imaging reveals chromatin compaction transitions and dynamic transcriptional bursting during stem cell differentiation in vivo
Figures
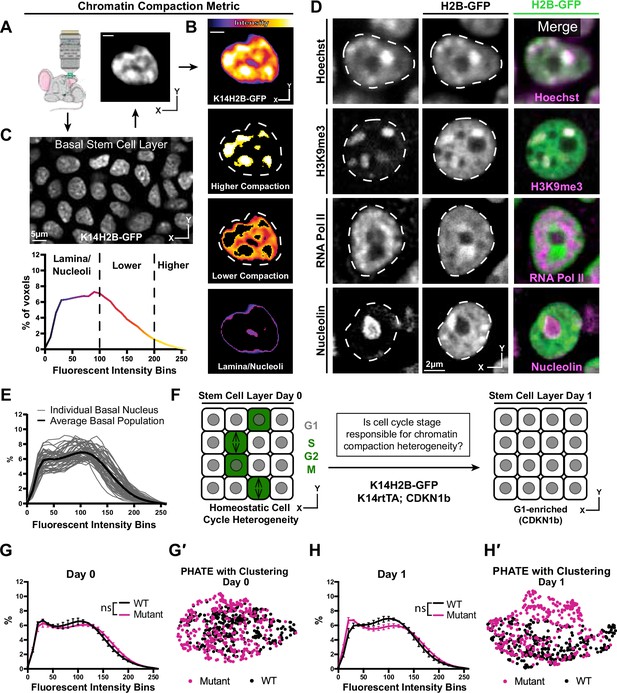
Chromatin compaction state is heterogeneous and independent of interphase cell cycle.
(A) Representative XY view of the basal stem cell layer showing the Kertain14-driven Histone2B-GFP allele in a live mouse. (B) A representative chromatin compaction profile of a single basal stem cell nucleus. Each voxel from the 3D volume of a nucleus was exported, normalized for mean fluorescent intensity, and plotted as a voxel percentage of volume against the 0–256 intensity bins (methods). Single optical slice. (C) A representative nucleus expressing H2B-GFP in a single optical slice, where the fluorescence intensity is displayed as a heatmap to illustrate the range of chromatin compaction within a nucleus. See Video 1 for a 3D rendering of this nucleus. (D) Fixed epidermal tissue expressing H2B-GFP (green) and co-stained with Hoechst or various subnuclear compartment markers (magenta), demonstrating that high H2B-GFP fluorescence intensity correlates with heterochromatin (H3K9me3), lower H2B-GFP fluorescence intensity correlates with euchromatin (RNA Pol II), and the lowest H2B-GFP fluorescence intensity (in part) correlates with nucleoli (Nucleolin). The colocalization/overlap of these two fluorophores are indicated by the presence of white signal. Nuclear outlines are traced in white, dotted lines. Single optical slice. (E) Chromatin compaction plots for 50 individual basal stem cells (grey lines) and the averaged population (bold, black line) revealing substantial heterogeneity of chromatin compaction states within the stem cell population. (F) Schematic of the genetic p27 (Cdkn1b) overexpression system to stall cells in late G1 after 1 day of doxycycline administration. An increased N of 1260 basal nuclei across all mice (3 mutant and 3 wild-type) over day 0 and day 1 because only a subset of basal stem cells are within a non-G1 cell cycle phase at any given time. (G) Comparison of chromatin compaction states of wild-type (K14H2B-GFP; K14rtTA) and mutant (K14H2B-GFP; K14rtTA; tetO-Cdkn1b) mice prior to doxycycline administration/induction on day 0. (G′) PHATE plot of the same wild-type and mutant cells from panel (G) on day 0 showing intermixed populations. (H) Comparison of chromatin compaction states of wild-type (K14H2B-GFP; K14rtTA) and mutant (K14H2B-GFP; K14rtTA; tetO-Cdkn1b) mice one day post doxycycline induction and stalling of the cell cycle in late G1 showing non-significant changes between wild-type and mutant populations. (H′) PHATE plot of the same wild-type and mutant cells from panel (H) on day 1 after Cdkn1b induction showing largely intermixed populations. Mean and standard deviation among mice shown in panels G and H.
-
Figure 1—source data 1
Heterogeneous chromatin compaction independent of interphase cell cycle status.
- https://cdn.elifesciences.org/articles/83444/elife-83444-fig1-data1-v2.xlsx
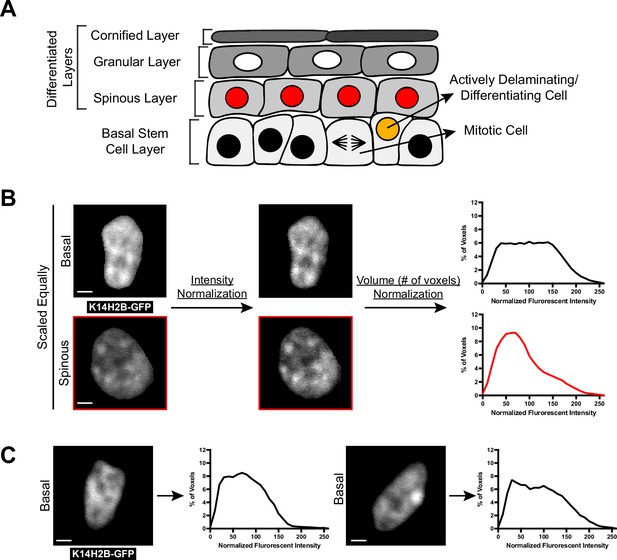
Chromatin compaction state analysis.
(A) XZ schematic of the mouse epidermis. The basal stem cell layer (bottom) contains actively cycling cells as well as cells that have committed to differentiation and are actively leaving the layer. Cells differentiate apically until they eventually die and form the most outer barrier of the epidermis. (B) Quantitative workflow of the chromatin compaction analysis from imaging data (methods). Individual nuclei (left) are surfaced in 3D, exported as raw voxel data, normalized for mean intensity and plotted as a voxel percentage of total nuclear volume. These normalized voxels can then be exported back as scaled, intensity-based images (right). (C) Two individual basal nuclei are shown with their corresponding chromatin compaction histograms. All scale bars = 2 μm.
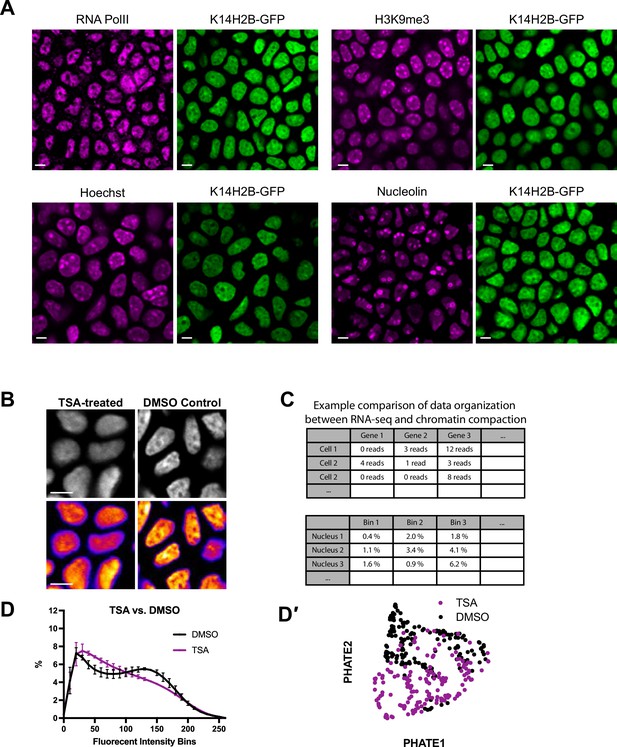
Organization of H2B-GFP allele.
(A) Representative crops of fixed tissue, single channel stainings of the basal stem cell layer. Genetically encoded K14H2B-GFP signal is shown in green, and immunofluorescent stainings in magenta. Single optical slice. (B) Representative regions of TSA-treated (left) and DMSO vehicle control (right) mice showing clear disruption of chromatin distribution. K14H2B-GFP intensity seen in greyscale (top) and in the FIRE LUT (bottom). (C) Comparison of mock data between scRNA-sequencing data typically applied to the PHATE/Louvain clustering algorithm, and the imaging-based voxel intensity data used. (D) Chromatin compaction analysis for TSA-treated and DMSO control nuclei showing altered chromatin compaction state in the TSA-treated mice. N=150 basal stem cell layer nuclei across three mice. (D′) PHATE representation of data from (E) showing largely separated clusters of TSA and DMSO-treated mice. All scale bars = 5 μm. Mean and standard deviation among mice shown in panel D.
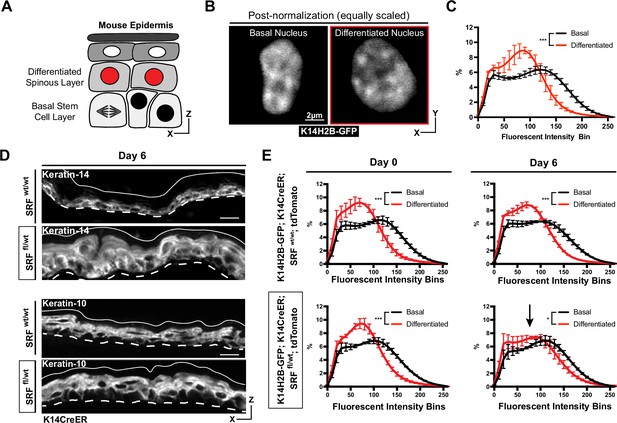
Chromatin compaction state changes through differentiation state.
(A) XZ schematic of the epidermis. The basal stem cell layer is shown with black nuclei and the differentiated (spinous) layer is shown apical with red nuclei. (B) Representative crops of individual nuclei from the basal and differentiated populations scaled identically. H2B-GFP signal in white. (C) Chromatin compaction profiles of averaged populations of cells from the basal stem cell and differentiated layers showing significant differences in chromatin plots. N=150 basal and 90 spinous cells across 3 mice. (D) Fixed, XZ tissue slices from Srf wt/wt and Srf fl/wt mice day 6 after tamoxifen recombination. The basal stem cell marker, KRT14, can be seen expanded into differentiated layers, and the differentiated marker, KRT10, can be seen localized correctly despite a thickened overall epidermis. (E) Chromatin compaction profiles for wild-type (Srf wt/wt; K14CreER; K14H2B-GFP; R26LSL-tdTomato) and mutant (Srf fl/wt; K14CreER; K14H2B-GFP; R26LSL-tdTomato) mice on day 0 and 6 after tamoxifen recombination. Black arrow in Srf fl/wt day 6 denotes that significant change in spinous cell chromatin compaction profile. N=150 basal and 90 spinous cells across 3 mice per day. Mean and standard deviation among mice shown in panels C and E.
-
Figure 2—source data 1
Chromatin compaction state changes with differentiation status.
- https://cdn.elifesciences.org/articles/83444/elife-83444-fig2-data1-v2.xlsx
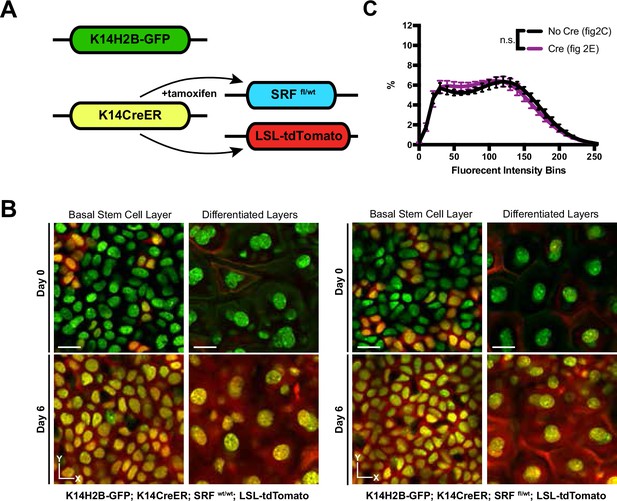
Genetic ablation of SRF alle in the basal stem cell layer.
(A) Schematic of the inducible genetic system used in Figure 2 to recombine and knock down Srf. (B) Representative crops from the basal stem cell and differentiated layers on days 0 and 6 in wild-type (top) and Srf heterozygous (bottom) mice. R26LSLtdTomato acts as a readout of recombination. (C) Chromatin compaction plots from Figure 2C and E (upper right panel) to determine the effect of Cre expression on chromatin compaction measurement. All scale bars = 10 μm. Mean and standard deviation among mice shown in panel C.
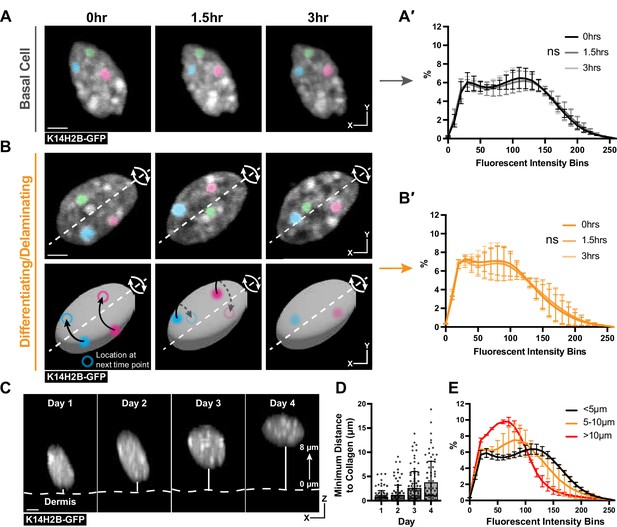
Chromatin compaction is stable over hours and progressively changes over days.
(A) Time lapse imaging data of a single nucleus crop at 0, 1.5, and 3 hr time points. H2B-GFP fluorescent signal is shown in white. Three, high-intensity chromocenters were chosen and pseudo-colored blue, green, and pink to demonstrate their static nature over the 3 hr. Max projection. (A′) Chromatin compaction profiles for cells in the basal stem cell layer at the 0, 1.5, and 3 hr time points showing no significant change over 3 hr. N=150 basal cells across 3 mice. Scale bar = 2 μm. (B) Same as in (A) but cells actively delaminating out of the basal stem cell layer, exhibiting rolling nuclei. The axis of rotation is show in the white dotted line, with the rotational direction shown in white arrows around that axis. Three, high-intensity chromocenters were chosen and pseudo-colored blue, green, and pink to demonstrate the dynamic spinning taking place, but the positional stability of global chromatin organization relative to itself. A cartoon (below) of the same nucleus to better visualize the rotation and orientation over the 3 hr with the blue and pink pseudocolored chromocenters tracked through time. Black arrows indicate where the chromocenter will move to in the next time point (hollow circle), dotted black arrow indicates rotation around the backside of the nucleus. Scale bar = 2 μm. Max projection. (B′) Chromatin compaction profiles for cells with spinning chromatin (actively delaminating cells) showing no significant change over 3 hr. N=146 spinning/delaminating cells over 3 mice. (C) XZ crops of the same nucleus tracked within the tissue over 4 days. Representative example of a differentiating cell over this time period. Bold, dotted white line denotes the epidermal/dermal interface. Solid, thin white line shows the minimum distance from collagen quantified in panel D. Scale bar = 2 μm. (D) H2B-GFP fluorescent signal shown in white. (D) Minimum distance from collagen for a randomly selected population of basal cells on day 1, some of which differentiated and moved apically by day 4, while others remained basally located. (E) Chromatin compaction profiles of nuclei within the tracked population from panel (D) binned as distance from collagen showing the direct transition in chromatin compaction profiles through differentiation. N=150 basal stem cells tracked over 4 days from 3 mice. Mean and standard deviation among mice shown for all chromatin compaction profiles.
-
Figure 3—source data 1
Chromatin compaction state changes slowly over days.
- https://cdn.elifesciences.org/articles/83444/elife-83444-fig3-data1-v2.xlsx
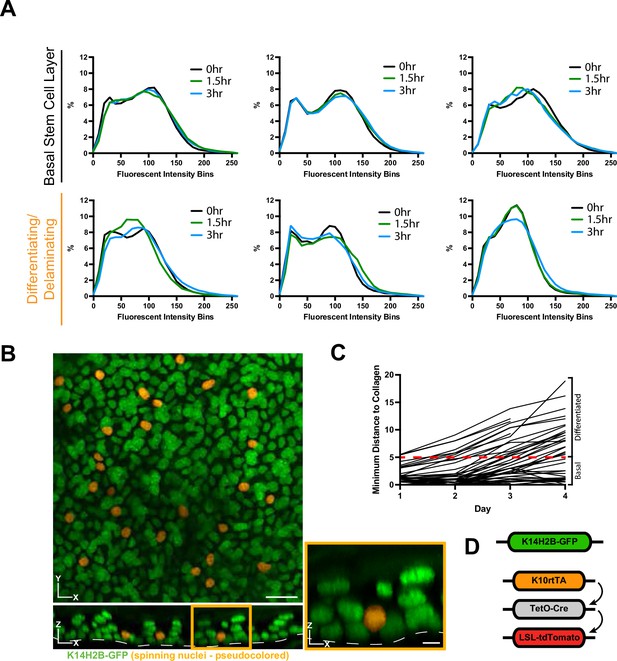
Single-cell chromatin compaction dynamics over hours.
(A) Individual nucleus chromatin compaction profiles from 3 basal stem cell and 3 delaminating/spinning cells at 0-, 1.5-, and 3 hr time points showing little change over this time scale. (B) Representative XY (top) and XZ (bottom) max projection FOV of the epidermis (K14H2B-GFP in green) with spinning chromatin pseudocolored in orange. (C) Individual tracking of cells from Figure 3D showing trajectory of minimum distance from collagen over 4 days. (D) Schematic of the inducible genetic system used in Figure 4 to visualize cells within the basal stem cell layer that have started expressing Keratin-10. Scale bar (left)=15 μm. Scale bar (inset)=5 μm.
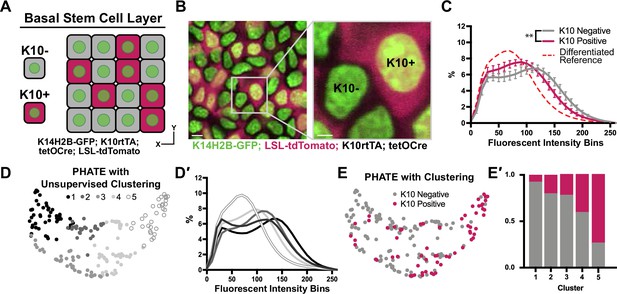
Chromatin compaction changes precede differentiation.
(A) XY schematic of genetic system (K14H2B-GFP; K10rtTA; tetO-Cre; R26LSL-tdTomato) allowing visualization of actively differentiating cells still within the basal stem cell layer (expressing differentiation-associated Keratin-10 gene). Keratin-10-positive cells indicated with red cytosol. (B) Representative XY crop of the basal stem cell layer showing Keratin-10-negative (no tdTomato signal) and Keratin-10-positive cells (tdTomato in cytosol) with a cropped inset on the right. Scale bar (left)=5 μm. Scale bar (inset)=2 μm. Max projection of basal stem cell layer. (C) Chromatin compaction profiles comparing K10 status (tdTomato on/off) in basal stem cells showing significant differences in chromatin compaction between groups. A differentiated reference line is shown by the dotted, red curve. Averaged K10 + cells shown in pink and averaged K10- cells shown in grey. N=187 K10- nuclei and N=132 K10+ nuclei from 3 mice. Statistical comparisons made between histogram groups, p<0.01. (D) PHATE plot of data from (C). Louvain clustering results are projected onto the PHATE plot. Each dot represents one nucleus profile, and the distance between dots represents the similarity in chromatin compaction profile. (D′) Chromatin compaction profiles of the averaged clusters identified through Louvain clustering in (D) elucidating directionality in the clustering from a more basal curve to a more differentiated curve. (E) The same PHATE/clustering dataset as in (D) with overlayed K10 status (on/off) again demonstrating directionality in the PHATE map. (E′) The ratio of K10 positive (red) and negative (grey) in each of the Louvain clusters. Mean and standard deviation among mice shown in panel C.
-
Figure 4—source data 1
Chromatin compaction state begins remodeling upstream of basal delamination.
- https://cdn.elifesciences.org/articles/83444/elife-83444-fig4-data1-v2.xlsx
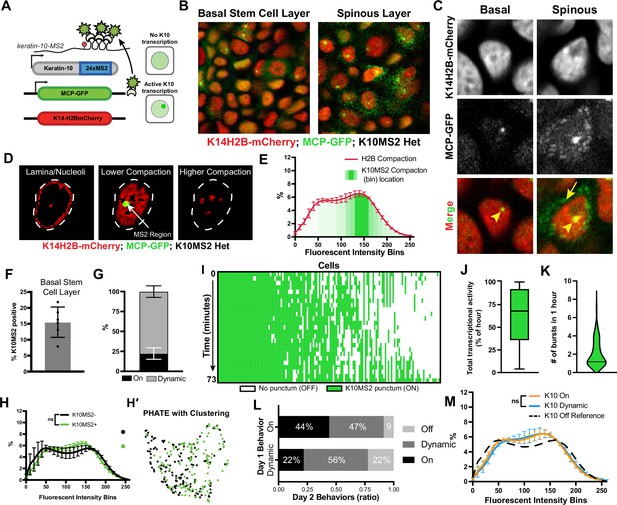
in vivo transcription of Keratin-10 precedes genome architecture changes through differentiation.
(A) Visual schematic of the MCP/MS2 system allowing visualization of a targeted gene under endogenous regulation. 24X MS2 repeats were knocked into the 3′UTR of the Keratin-10 locus and chromatin compaction visualized with K14H2B-mCherry. Presence of nuclear MCP punctum indicates active Keratin-10 transcription, where lack of signal indicates no active transcription at that locus. (B) Representative field of view of the K10MS2(het)/MCP-GFP system in the basal (left) and spinous (right) layers. Nuclei labeled in red and Keratin-10 RNA in green. Sum projection through each layer. (C) High-resolution insets of the K10MS2(het)/MCP-GFP system in basal and spinous cells. Yellow arrowhead = site of active Keratin-10 transcription inside the nucleus. Yellow arrow = mature Keratin-10 transcripts in the perinuclear space. Sum projection through nuclei. (D) Representative image of a nucleus separated into the same chromatin compaction regions as in Figure 1C. Active transcription of Keratin-10 can be seen within the lower compaction region from bins 100–200. Scale bar = 2 μm. Single optical slice. (E) Chromatin compaction profile of averaged H2B-mCherry basal stem cells (red line) and the fluorescent bin location of Keratin-10 transcription punctum (green, semi-translucent bars). N=150 Keratin-10 transcribing basal stem cells across 3 mice. Surfaced MCP signal was used to identify the mCherry fluorescent intensity bins in which Keratin-10 transcription occurred, and then plotted as increasing green transparency. (F) Populational percentage of active Keratin-10 transcription in the basal stem cell layer in K10MS2 Het mice. N=3 100 x 100 μm regions quantified over 3 mice. (G) Percentages of Keratin-10 dynamics within the Kertain-10-positive basal stem cell layer over 1 hour. ~25% of basal stem cells actively expressing Keratin-10 remained on throughout the 1 hr timelapse, while ~75% had dynamic transcriptional behaviors. (H) Chromatin compaction analysis of basal stem cells either actively transcribing Keratin-10 (green punctum in nucleus) or not transcribing Keratin-10. Despite active transcription of the differentiation gene, there is no significant chromatin compaction remodeling at this stage. N=150 Keratin-10 ‘off’ and 150 ‘on’ nuclei across 3 mice. (H′) PHATE plot of the data in (I) showing intermixed populations of Keratin-10 positive and negative basal stem cells. (I) Keratin-10 dynamics over 1.25 hr of cells that actively transcribed Keratin-10 for at least one time point. Cells ordered by total amount of time transcribing Keratin-10 over the timecourse. N=148 over 3 mice. (J) Average Keratin-10 total transcriptional activity over 1.25 hr per cell. (K) The number of Keratin-10 bursting events over 1.25 hours per cell. (L) Quantification of how Keratin-10 dynamics change over the course of 1 day. Keratin-10-positive nuclei from day 1 were binned into ‘on’ and ‘dynamic’ (y-axis) and the same nuclei located on day 2. Day 2 transcriptional dynamics were quantified (x-axis) showing surprising flexibility in transcriptional dynamics over a day. (M) Chromatin compaction analysis of the ‘on’ and ‘dynamic’ Keratin-10 transcription populations from (F) showing very little, non-significant differences between the two populations. The Keratin-10 ‘off’ curve from (H) is shown as a reference in the dotted, black line. N=187 ‘dynamic’ and 124 ‘on’ over 3 mice. Mean and standard deviation among mice shown for all chromatin compaction profiles.
-
Figure 5—source data 1
Transcription of differentiation gene is highly dynamic and precedes significant chromatin compaction changes.
- https://cdn.elifesciences.org/articles/83444/elife-83444-fig5-data1-v2.xlsx
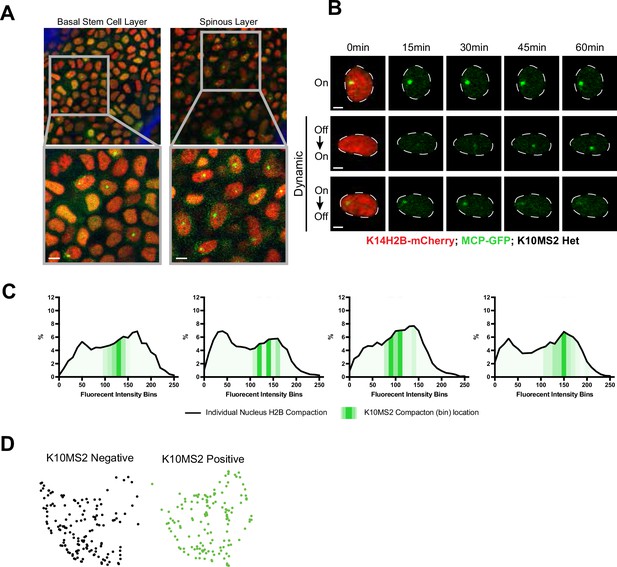
Imaging differentiation-associated cell identity.
(A) Representative crops of the differentiated spinous layer (left) and basal stem cell layer (right), as well as magnified inset showing active site of Keratin-10 transcription, and nascent transcripts in cytosol awaiting translation. Scale bar = 5 μm. (B) Representative crops of individual basal stem cell nuclei exhibiting different Keratin-10 transcriptional dynamics over the course of 1 hr. H2B-mCherry signal can be seen in red in the first time point for reference, and Keratin-10 transcription punctum in green in all time points. White, dotted line shows the nuclear border. Scale bar = 2 μm. (C) Individual nuclear chromatin compaction profiles for four basal stem cell layer nuclei. K14H2B-mCherry compaction profile shown by black line, and the MCP/MS2 Keratin-10 transcription regions in green translucency. (D) PHATE representation from Figure 5H′ separated by cell status (Keratin-10 ‘on’ or ‘off’). While largely intermixed, there appears a slight preference (though non-significant) for one side of the PHATE cluster vs. the other (‘off’ to the left, ‘on’ to the right).
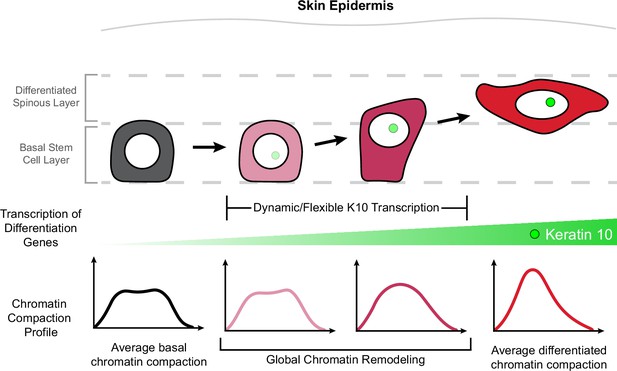
Chromatin architecture remodeling through epidermal differentiation.
Epidermal stem cells undergo incremental changes toward differentiation over 3–4 days (top). During this process, differentiation-committed cells within the basal stem cell layer begin expressing Keratin-10 dynamically over hours and flexibly over days (green circle/site of transcription in nucleus). Basal stem cell and fully differentiated spinous cells have specific chromatin architecture states associated with their different cellular identities (bottom row), and global chromatin remodeling begins before delamination and exit from the basal stem cell layer.
Videos
Chromatin compaction.
A single basal stem cell layer nucleus crop visualizing H2B-GFP intensity. The video first scans through the greyscale, intensity image (white), then through the FIRE LUT intensities shown in Figure 1B, then the three different binned intensities seen in Figure 1B and C. Nuclear outline is denoted by the white dotted line.
Spinning chromatin.
An XY field-of-view of the upper, basal stem cell layer in which chromatin (H2B-GFP) can be observed to spin. Timelapse is 3 hours long and looped 3 times.