The integrated brain network that controls respiration
Figures
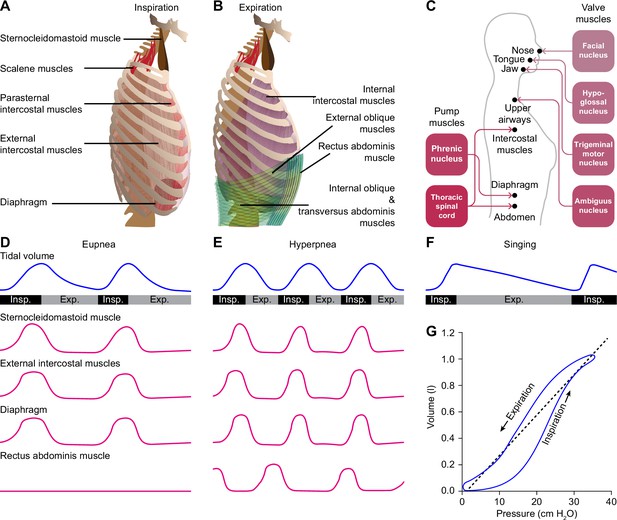
Respiratory muscles and their innervation.
(A) The main driving force for inspiration is delivered by the diaphragm in conjunction with the external intercostal muscles. Other muscles that can enlarge the chest, such as the parasternal intercostal, sternocleidomastoid and scalene muscles, may also contribute. (B) Active expiration involves contraction of the internal intercostal muscles together with abdominal muscles. (C) The pump muscles are innervated from the spinal cord, with the phrenic nucleus housing the motor neurons of the diaphragm, and the thoracic spinal cord those of the intercostal and abdominal muscles. (D) During regular breathing at rest (eupnea), inspiration is followed by a largely passive form of expiration termed post-inspiration or early expiration. During post-inspiration, the abdominal muscles are not (strongly) involved. (E) When the metabolic demand is higher, hyperpnea entails the activation of expiratory pump muscles during active expiration. (F) Prolonged post-inspiration, when required assisted by active expiration, ensures a longer period with constant outflow of air as exploited by professional singers. (G) Intrapleural pressure-volume curve during normal respiration in which the lung compliance is defined as the slope of the dotted line. Schematized data based on Bellani et al., 2018 and Pitts et al., 2015 (D–E), Salomoni et al., 2016 (F), and Albaiceta et al., 2005 (G). Exp.=expiration, Insp.=inspiration.
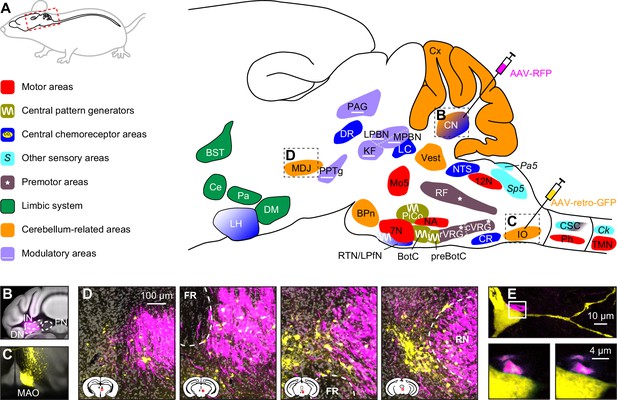
Brain areas involved in subconscious respiratory control.
(A) The subcortical areas involved in control of respiration were classified according to their main function and plotted at their approximate location on a sagittal projection of the mouse brain. 7N = facial nucleus, 12N=hypoglossal nucleus, BotC = Bötzinger complex, BPn = basal pons, BST = bed nucleus of the stria terminalis, Ce = central amygdala, Ck = Clarke’s column, CN = cerebellar nuclei, CR = caudal raphe nucleus, CSC = cervical spinal cord, cVRG = caudal ventral respiratory group, Cx = cerebellar cortex, DM = dorsomedial hypothalamus, DR = dorsal raphe nucleus, IO = inferior olive, KF = Kölliker-Fuse nucleus, LC = locus coeruleus, LH = lateral hypothalamus, LPBN = lateral parabrachial nucleus, LPfN = lateral parafacial nucleus, MDJ = nuclei of the mesodiencephalic junction, Mo5=trigeminal motor nucleus, MPBN = medial parabrachial nucleus, NA = nucleus ambiguus, NTS = nucleus of the solitary tract, Pa5=paratrigeminal nucleus, PAG = periaqueductal gray, Pa = paraventricular hypothalamus, Ph = phrenic nucleus, PiCo = postinspiratory complex, PPTg = pedunculopontine tegmental area, preBotC = pre-Bötzinger complex, RF = reticular formation, RTN = retrotrapezoid nucleus, rVRG = rostral ventral respiratory group, Sp5 = spinal trigeminal nucleus, TMN = thoracic motor neurons, Vest = vestibular nuclei. Neural tracing can reveal monosynaptic connections between brain regions, as illustrated with an example using an anterograde tracer in the cerebellar nuclei (B; see injection needle in panel A, AAV-RFP, pseudocolored in magenta), and a retrograde tracer in the inferior olive (C; AAV-retro-GFP, pseudocolored in yellow). DN = dentate nucleus, FN = fastigial nucleus, IN = interposed nucleus, MAO = medial accessory olive. (D) Both tracers can be observed in the MDJ, indicating the presence of monosynaptic projections from the cerebellar nuclei to the MDJ and from there to the inferior olive. Sagittal sections from rostral to caudal (see schemes in the lower left corners with red rectangles indicating locations of images). FR = fasciculus retroflexus, RN = red nucleus. (E) A neuron (yellow) in the MDJ that projects to the inferior olive. Close to the soma of this neuron, a bouton (magenta) of a neuron originating from the cerebellar nuclei can be seen (insets below, imaged at two levels 0.7 µm apart). Panels B-E originate from a representative mouse and are modified from Figure 7 from Wang et al., 2022.
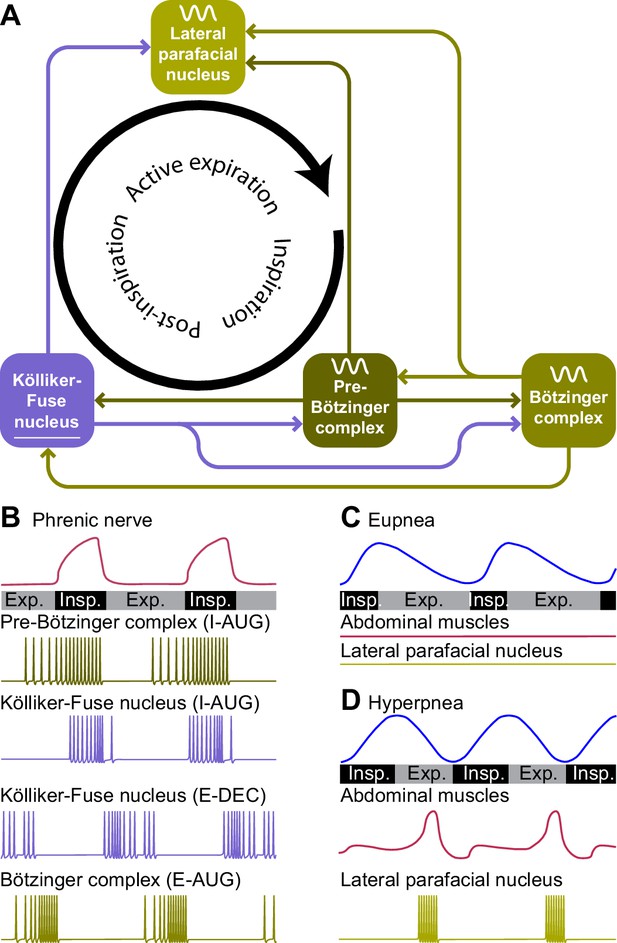
Central pattern generators encode the respiratory rhythm.
(A) Connections between the pre-Bötzinger complex that organizes inspiration, the Kölliker-Fuse nucleus that relates to the inspiration/expiration switch, the lateral parafacial nucleus that triggers active expiration, and the Bötzinger complex related to expiration. (B) Neuronal activity in the central pattern generators varies during the respiratory cycle. The schematized traces represent action potential firing of selected neuronal cell types in relation to activity of the phrenic nerve that drives the main inspiratory pump muscle, the diaphragm. From top to bottom: augmenting inspiratory neurons (I-AUG) from the pre-Bötzinger complex and the Kölliker-Fuse nucleus, a decreasing expiratory neuron (E-DEC) from the Kölliker-Fuse nucleus, and an augmenting expiratory neuron (E-AUG) from the Bötzinger complex. These representations are based on in vitro studies of Marchenko et al., 2016 (pre-Bötzinger complex), Ezure and Tanaka, 2006 (Kölliker-Fuse nucleus), and Flor et al., 2020 (Bötzinger complex). (C) During eupnea, thus in the absence of active expiration, neither the expiratory pump muscles of the abdomen, nor the neurons of the lateral parafacial nucleus are active. (D) During hyperpnea, thus when active expiration takes place, abdominal expiratory pump muscles are active when the lateral parafacial nucleus neurons produce action potentials. Schematized based on in vivo recordings of anesthetized rats by Pagliardini et al., 2011. Insp.=inspiration, Exp.=expiration.
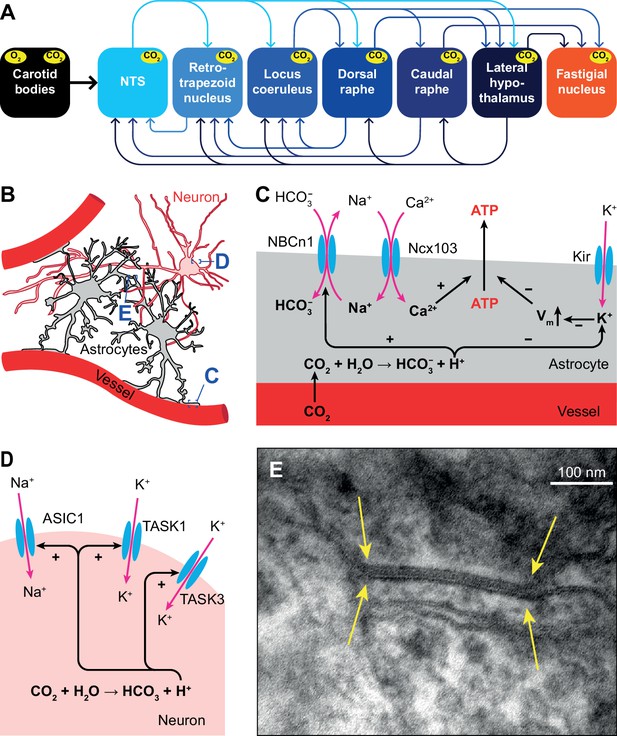
Respiratory chemoreception.
(A) Connections between the carotid bodies and the central chemoreceptor areas. (B) Astrocytes are in direct contact with blood vessels and neurons. (C) Chemoreceptor pathways in astrocytes triggering ATP release that can activate nearby neurons. (D) Chemoreceptor pathways in neurons, based on activation of Na+ channels and inward-rectifier K+ channels. (E) Gap junctions between glial cells, as shown here in the cerebellar cortex of a mouse (yellow arrows), can contribute to central chemoreception. In particular, the conductivity of gap junctions composed of Cx26 depends on pH. Previously unpublished electron microscopic image from our lab.
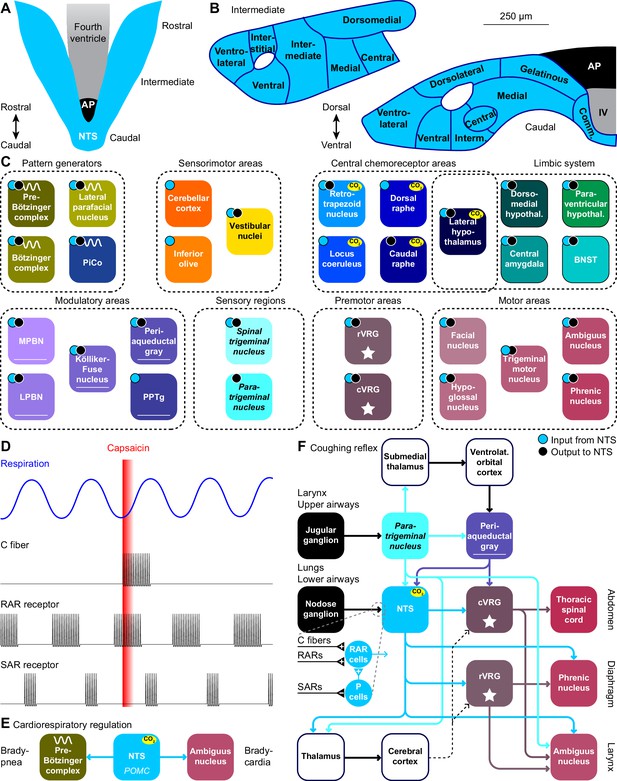
The nucleus of the solitary tract and vagal afferents.
Schematic drawings of the nucleus of the solitary tract (NTS) in relation to the area postrema (AP) and the fourth ventricle (IV). Dorsal (A) and coronal views at intermediate and caudal levels (B). (C) Brain regions that project to, or get input from the NTS. With most areas, bidirectional connections exist (cyan dots: areas innervated by NTS neurons; black dots: areas with neurons innervating the NTS). (D) Heterogeneity in vagal afferents. Capsaicin can, as a pulmonary irritant, evoke activity of C fibers, but not of rapidly or slowly adapting receptors (RAR and SAR, respectively). The latter two show phasic activity during regular breathing. Schematic drawing of action potential firing based on data presented in Ho et al., 2001. (E) Pro-opiomelanocortin-expressing (POMC) neurons of the NTS project to the pre-Bötzinger complex and to cardiac vagal motor neurons in the ambiguus nucleus. Via these pathways, they can reduce inspiration and cardiac function, respectively. (F) Coughing can be triggered by sensing an irritant via vagal projections from the larynx or upper airways via the jugular ganglion to the paratrigeminal nucleus, or from the lungs or lower airways via the nodose ganglion to the NTS. Distinct types of vagal fibers differentially affect RAR relay neurons, with SARs inhibiting pump neurons (P cells). The motor neurons of expiratory muscles are directly and indirectly activated from the paratrigeminal nucleus and the NTS. A specific cortical circuit for inhibiting reflexive coughing involving the submedial thalamus and the ventrolateral orbital cortex has been described, next to more general thalamo-cortical pathways that can modulate coughing. The latter pathways can use different connections to premotor nuclei (indicated with dotted lines).
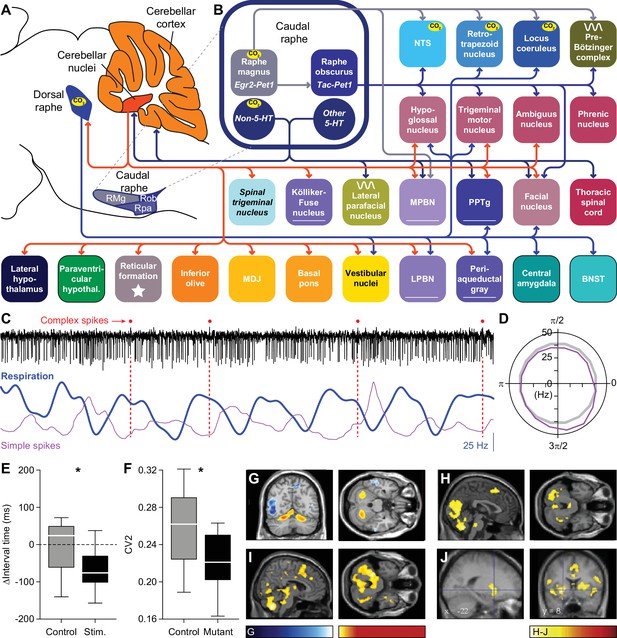
Raphe nuclei and cerebellum.
(A) The caudal raphe consists of the raphe magnus (RMg), the raphe obscurus (Rob) and the raphe pallidus (Rpa). The cerebellum consists of the cerebellar cortex and the cerebellar nuclei. (B) Different populations of raphe neurons can have different projection patterns. For example, Egr2-positive serotonergic neurons of RMg have intrinsic chemoreceptor properties and project mainly to other central chemoreceptor areas. The downstream Tac-positive serotonergic neurons of Rob lack intrinsic chemoreceptor properties and project predominantly to respiratory motor neurons. Other neurons of the caudal raphe, whether serotonergic or non-serotonergic, extend the caudal raphe projections to further respiratory regions. These projections partially overlap with those of the dorsal raphe. Indicated are also the projections from the cerebellar nuclei. Note that also the locus coeruleus receives cerebellar input via direct Purkinje cells projections. (C) At rest, Purkinje cells of the lateral cerebellum can show modulation of their complex spike and simple spike frequency in relation to the respiratory cycle. This is illustrated with a representative electrophysiological recording of a Purkinje cell in an awake mouse. Complex spikes are noted with a red dot on top of the trace. In this example, they occur preferably just after the onset of expiration (downward phase of the blue trace). Simple spike firing is increased during the last phase of expiration, as illustrated by their instantaneous frequency (magenta curve). The duration of this fragment is 3.6 s. (D) For this Purkinje cell, the simple spike frequency is upregulated at the last phase of respiration, compared to the expected frequency (grey circle). 0=start inspiration. (E) Optogenetic stimulation of Purkinje cells in the lateral cerebellum can shorten the interval until the next inspiration. (F) In the absence of excitatory output of the cerebellar nuclei, in the Atoh1-En1/2 mouse model for cerebellar neuropathology, the respiratory cycle is more regular than in control mice, as quantified by the local coefficient of variation (CV2). fMRI scans indicate that specific brain regions, including the cerebellum, are activated during respiratory challenges: (G) Increasing respiratory resistance. (H) Hypoxia (13% oxygen for 1 min). (I) Actively slowed breathing. (J) During breath holding, the cerebellum is not activated. * p<0.05 Scaling of activity levels in G: –6.7 to –3.1 (blue colors) and +3.1 to+8.5 (red colors); in H-J: 0–15. Panels C and D are modified from Figure 1 from Romano et al., 2020, E from Figure 6 from Romano et al., 2020, F from Figure 4 from Taylor et al., 2022, and H and I from Figure 4 from Critchley et al., 2015.
© 2013, Elsevier. Panel G is reproduced from Figure 2 from Raux et al., 2013, with permission from Elsevier; this panel is not covered by the CC-BY 4.0 license and further reproduction of these panels would need permission from the copyright holder.
© 2008, Elsevier. Panel J is reproduced from Figure 4 from McKay et al., 2008, with permission from Elsevier; this panel is not covered by the CC-BY 4.0 license and further reproduction of these panels would need permission from the copyright holder.
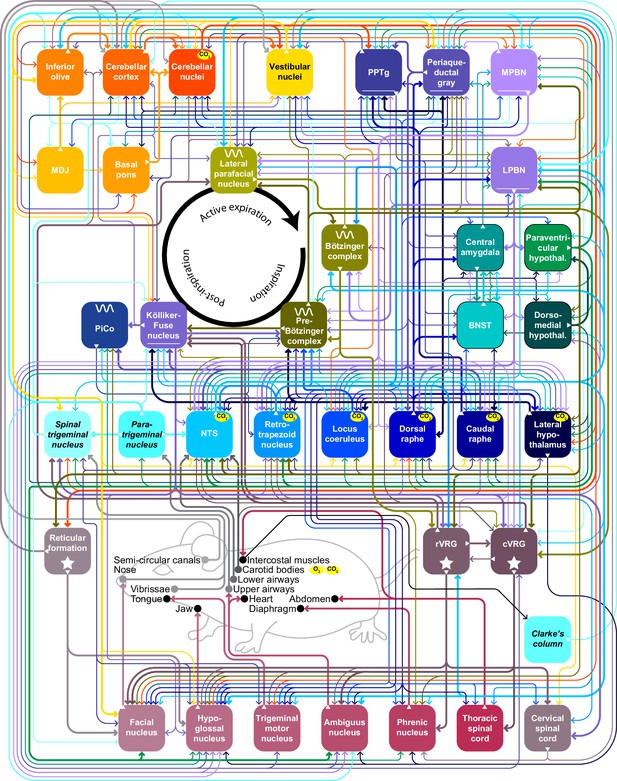
Main pathways underlying subconscious control of respiration Schematic drawing representing the brain areas most involved in subconscious control of respiration and the main pathways connecting them.
Fat lines indicate relatively strong connections and thin lines moderately strong ones. Sparse connections are not included in this scheme. When an area is marked with ‘CO2’ it contains central chemoreceptor properties, while the carotid bodies sense blood levels of CO2 and of O2. The central pattern generators are indicated with a sine wave. BNST = bed nucleus of the stria terminalis; cVRG = caudal ventral respiratory group; LPBN = lateral parabrachial nucleus; MPBN = medial parabrachial nucleus; NTS = nucleus tractus solitarii; PiCo = postinspiratory complex; PPTg = peripeduncular tegmental nucleus; rVRG = rostral ventral respiratory group. See also Supplementary file 1.
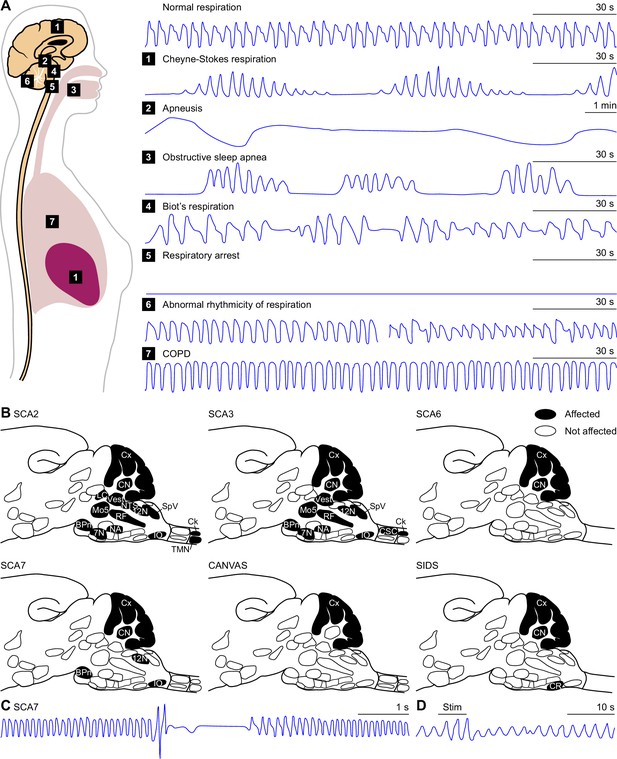
Pathology.
(A) Based on the location of a lesion or structural abnormality, several types of disordered breathing can be expected. Cheyne-Stokes respiration can result from a bilateral hemispheric or diencephalic lesion. Apneustic breathing has originally been described as the consequence of a lesion at the level of the Kölliker-Fuse nucleus, but can probably also be caused by damage to surrounding tissue. A Biot’s respiration pattern can develop after a lesion in the medulla, while a respiratory arrest occurs after damage to the pre-Bötzinger complex or the upper spinal cord. Obstructive sleep apnea typically shows interrupted breathing pattern during sleep. Cerebellar dysfunction can lead to more (left) or less (right) rhythmic respiration. COPD patients show increased respiratory rates and reduced passive expiration. It should be noted however that cardiopulmonary or metabolic causes can also trigger all these respiratory patterns. Schematized traces of the tidal volume based on: Cheyne-Stokes respiration: Lorenzi-Filho et al., 1999; Apneusis: Mador and Tobin, 1990; Biot’s respiration: Farney et al., 2003: Abnormal rhythmicity: Ebert et al., 1995; COPD (and normal): Jolley et al., 2009. (B) Respiration-related brain areas demonstrating structural damage in several disorders, projected in the same notation as in Figure 2A. Note that SCA6 is mainly a cerebellar disorder. While CANVAS affects the cerebellum, the syndrome has also peripheral nerve involvement. In SIDS multiple non-cerebellar causes have been described in post mortem studies. Some post mortem brain studies, however, have shown irregularly formed Purkinje cells in the cerebellar cortex. See Supplementary file 1. (C) Apneustic breathing pattern in a mouse model of spinocerebellar ataxia type 7. Schematic representation based on Fusco et al., 2021. (D) Electrical stimulation (Stim) adjacent to the fastigial nucleus induces a fastigial pressor response in an anesthetized cat. During stimulation, the tidal volume is increased, and suppressed afterwards. Based on Miura and Takayama, 1988.
Additional files
-
Supplementary file 1
List of studies demonstrating the existence of monosynaptic projections between areas relevant for subconscious control of respiration.
Connections that were identified as sparse by the authors of these studies are not listed in this table. Note that the list of neurotransmitters involved is not complete. 1 Tree shrew (Tupaia belangeri chinensis); 2 Rufous horseshoe bat (Rhinolophus rouxi); 3 Rhesus monkey (Macaca mulatta); 4 Crab-eating macaque (Macaca fascicularis); 5 Squirrel monkey (Saimiri sciureus). 5-HT = serotonin, A = anterograde, aa = amino acids, AAV = adeno associated virus, ACh = acetyl choline, BDA = biotinylated dextran amines, Biotinam = biotinamide, CRH = corticotropin-releasing hormone, CTb = cholera toxin B subunit, cVRG = caudal part of the ventral respiratory group, DA = dopamine, Degen = degeneration, DTR = dextran-Texas red, DY = diamidino yellow, EB = Evans blue, Exc = excitatory (not specified which neurotransmitter), E-phys = electrophysiology, FB = fast blue, FG = fluorogold, FR = fluoro ruby, GABA = γ-aminobutyric acid, GFP = green fluorescent protein, Glu = glutamate, Gly = glycine, HSV = herpes simplex virus, HRP = horseradish peroxidase, Inh = inhibitory (not specified which neurotransmitter), m = muscle, MDJ = nuclei of the meso-diencephalic junction, n = nucleus or nuclei, NA = noradrenaline / norepinephrine, Nbiotin = neurobiotin, NMB = neuromedin B, NT = neurotransmitter, NTS = nucleus tractus solitarii, Optogen = optogenetic stimulation, OXT = oxytocin, PHA-L = Phaseolus vulgaris leucoagglutinin, PiCo = post-inspiration complex, PPTg = pedunculopontine tegmental nucleus, PI = propidium iodide, POMC = pro-opiomelanocortin, Pseudorab = Pseudorabies, R = retrograde, rVRG = rostral part of the ventral respiratory group, term = terminalis, TMR = tetramethylrhodamine, VP = vasopressin, WGA = wheat germ agglutinin. b – Respiratory control areas affected by pathology Summary of brain regions involved in respiratory control and affected by selected diseases or syndromes. An area is listed in this table if a study reported damage to that area in a majority of subjects included in that study. Case studies with a single patient are not included in this table.
- https://cdn.elifesciences.org/articles/83654/elife-83654-supp1-v1.docx