Neural dynamics underlying self-control in the primate subthalamic nucleus
Figures
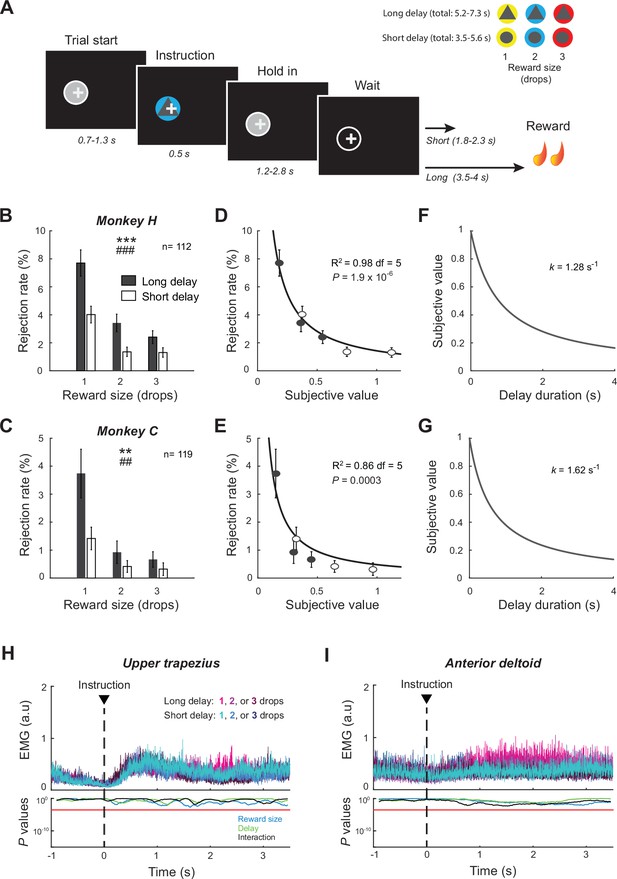
Delayed reward task and behavioral performance.
(A) Temporal sequence of task events. After the monkey initiated a trial by positioning a cursor (+) within a visual target (gray circle), an instruction cue was presented briefly signaling the reward size (one, two, or three drops of food) and the delay-to-reward (short or long). The animal was required to maintain the cursor position over the waiting period to successfully obtain reward. (B, C) Rejection rates (mean ± SEM) were calculated and averaged for the six possible reward contingencies across sessions. Measures were affected by both reward size and delay (two-way ANOVA). Size: ***p<0.001, **p<0.01; ddelay: ### p<0.001, ## p<0.01. (D–G) For each animal, a temporal discount factor (k) was found that yielded the best fit between averaged rejections rates and the hyperbolic model expressed by Equation 2. Goodness of fit was evaluated by the coefficient of determination (R2). (H, I) EMG signals collected in monkey H were aligned on the presentation of cues. The effects of reward size and delay were examined using a series of two-way ANOVAs. Red lines indicate the statistical threshold (p<0.05/173; Bonferroni correction).
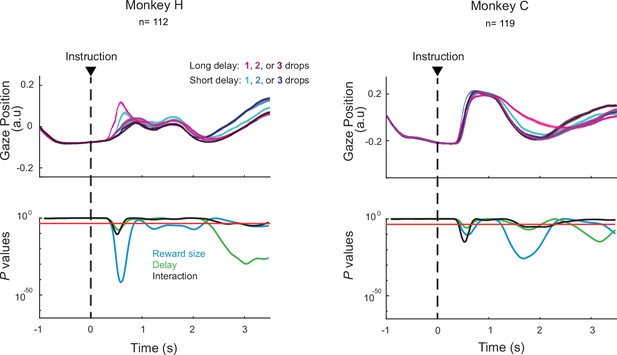
Eye positions varied according to the levels of reward and delay.
For each task condition, horizontal and vertical components were combined to obtain tangential coordinates (averaged across sessions; ± SEM). Potential interaction with task parameters was tested using a series of two-way ANOVAs (reward size × delay). Red lines indicate the statistical threshold (p<0.05/173, Bonferroni correction).
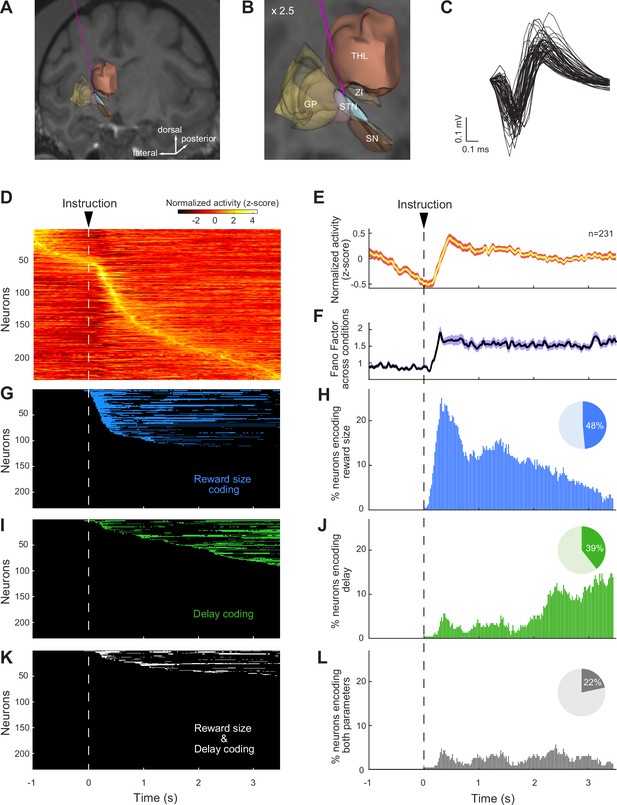
Subthalamic nucleus (STN) neurons were modulated by reward size and delay.
(A, B) Reconstruction of a trajectory used for STN recordings with a structural MRI and high-resolution 3-D templates of individual nuclei derived from Martin and Bowden, 1996. Globus pallidus (GP), substantia nigra (SN), zona incerta (ZI), and thalamus (THL). (C) Sample of action potential waveforms emitted by STN neurons. (D) Color map histograms of neuronal activities recorded from the STN. Each horizontal line indicates neural activity aligned to instruction cues averaged across trial types. Neuronal firing rates were Z-score normalized. (E) Population-averaged activities of STN neurons, and (F) Fano factors that showed the variability of the neural population ensemble across the six possible reward contingencies. The width of the curves indicates the population SEM. (G–L) Influence of reward size and delay on individual neural activities was detected by a series of two-way ANOVAs (p<0.05/173, Bonferroni correction). The time course of encoding of task-relevant information (left column) and the fractions of neurons modulated by reward size and/or delay (right column) were represented for each time bin. Pie charts show the total fraction of STN neurons influenced by reward size (blue), delay (green), or both task parameters simultaneously (gray).
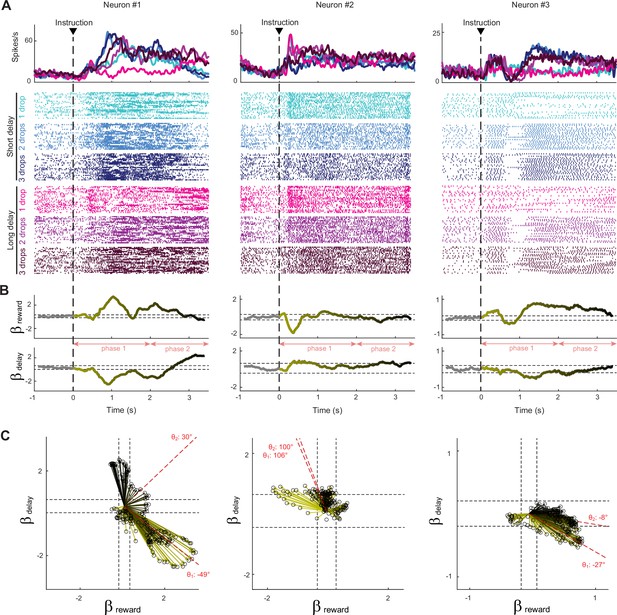
Response of subthalamic nucleus (STN) neurons to the six possible reward contingencies.
(A) The activity of three exemplar neurons that were classified as task-related cells. Spike density functions and raster plots were constructed separately around the presentation of instruction cues for the different cost–benefit conditions. (B) A sliding window regression analysis compared firing rates between trial types (as expressed by Equation 3). The regression coefficients (yellow-to-black lines) were used to characterize the dynamic encoding of reward size (βReward) and delay (βDelay). The horizontal dashed lines indicate the statistical threshold for significant β values (calculated from the pre-instruction period with a one-sample t-test, df = 46, p<0.05). (C) Time series of regression coefficients projected into an orthogonal space where reward size and delay composed the two dimensions. Vector time series were produced for significant β values. Black dashed lines indicate statistical thresholds. The angle (θ) of the vector sum (red dashed lines) was calculated to identify how neurons integrated cost–benefit conditions during the two consecutive phases of the waiting period (phase 1: 0–2 s, phase 2: 2–3.5 s).
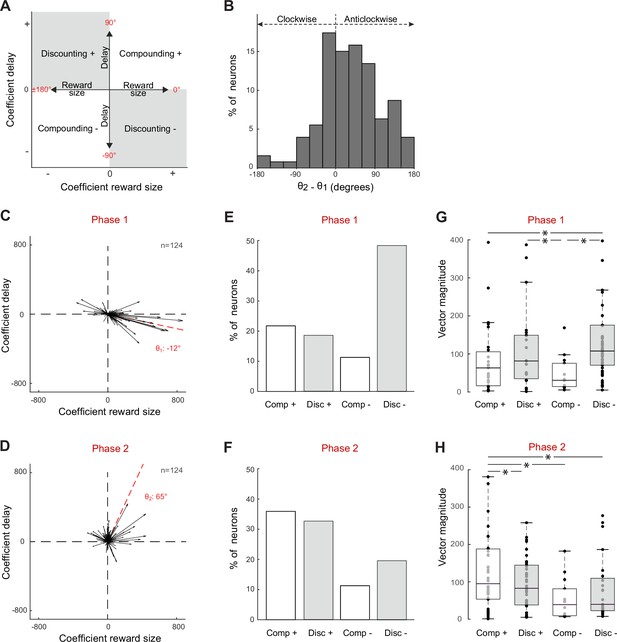
Subthalamic nucleus (STN) neurons exhibit mixed signals in phase 1 (0–2 s post-instruction) and phase 2 (2–3.5 s post-instruction).
(A) Schematic depiction of the regression subspace composed of reward size and delay. Various patterns of neural encoding could be categorized depending on the angle (θ) of vectors: Discounting– (between –90 and 0°); Discounting+ (between 90 and 180°); Compounding+ (between 0 and 90°); Compounding– (between –180 and –90°). (B) The angle differences calculated between phases 1 and 2 (θ2 – θ1) show how the neural encodings were modified during the course of the hold period. A positive angle difference corresponds to a turn anticlockwise, while a negative one corresponds to a turn clockwise. (C, D) Vectorial encoding of reward size and delay for all task-related neurons in phases 1 (C) and 2 (D). Vector sums were calibrated by subtracting the mean β values of the pre-instruction epoch and then dividing by 2 SD of this control period. The red dashed lines indicate the population vectors. (E, F) Fractions of task-related neurons categorized as Discounting cells (Disc-, Disc+) or Compounding cells (Comp+, Comp-). (G, H) Vector magnitudes (mean ± SEM) were compared between different categories of task-related neurons (one-way ANOVA *F > 2.1, p<0.05). The central line of the box plots represents the median, the edges of the box show the interquartile range, and the edges of the whiskers show the full extent of the overall distributions.
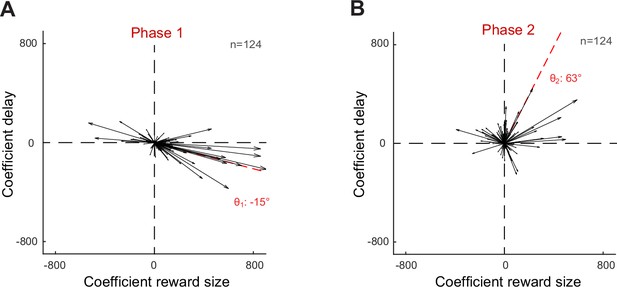
Population vectors of the encoding of reward size and delay in phase 1 (A) and phase 2 (B) using a regression model that excluded eye movement variables (SCi = β0 + βR Ri + βD Di).
These figures follow the conventions of Figure 4C and D. Individual vectors and mean population vectors produced here were very similar to results of Figure 4 (differences <3°).
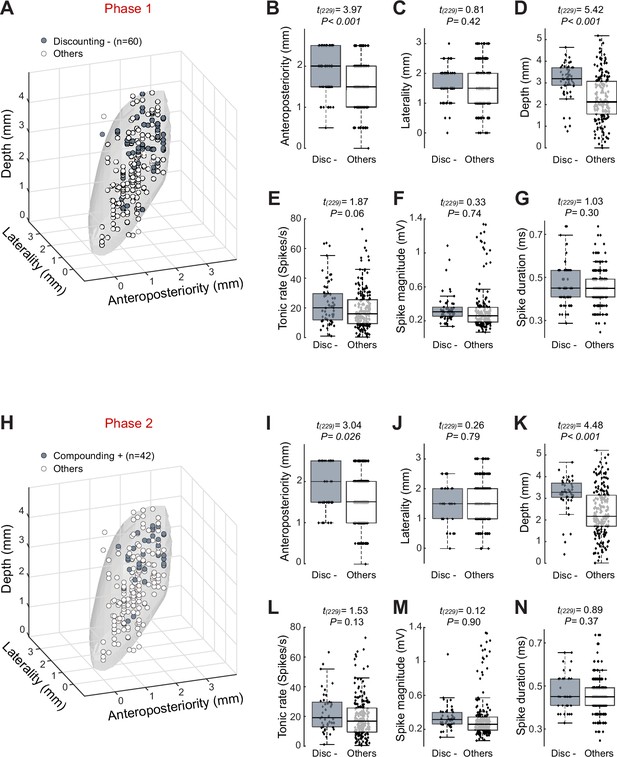
Topography of subthalamic nucleus (STN) neurons categorized as Discounting– in phase 1 and Compounding+ in phase 2.
(A) Three-dimensional plots of cell type distributions based on coordinates from the recording chamber. The template of STN (gray surface) is derived from the atlas (Martin and Bowden, 1996). (B–D) Comparisons of cell positions show that Discounting– neurons (Disc–) were located more dorsal and posterior than other categories of cell type (two-sample t-test). (E–G) No differences were found in the tonic rate (E), spike magnitude (F), and spike duration (G) between Discounting– neurons and other cells. The central line of the box plots represents the median, the edges of the box show the interquartile range, and the edges of the whiskers show the full extent of the overall distributions. (H–N) Neurons categorized as Compounding+ in phase 2 were located more dorsal and posterior than other cell types.
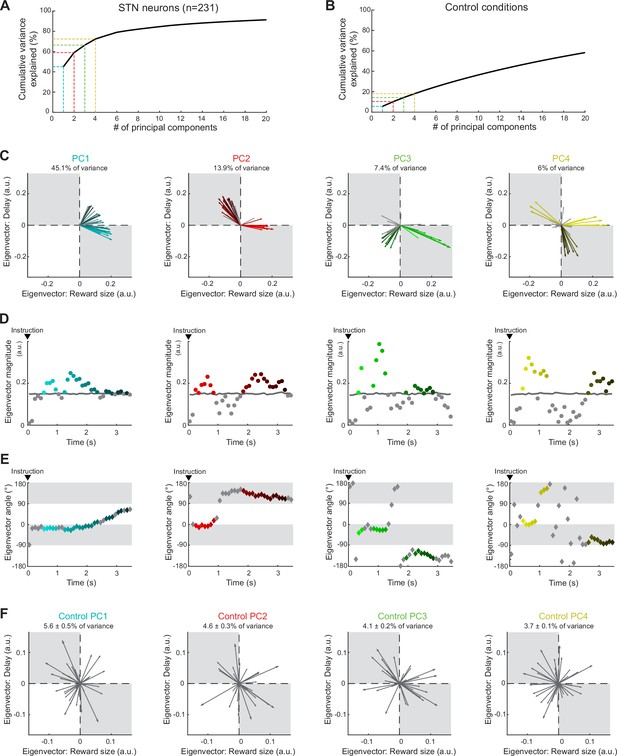
Neural population ensemble provides dynamic integration of reward size and delay.
(A) Cumulative variance explained by principal component analysis (PCA) in the population of all recorded neurons. Dashed lines indicate the percentages of variance explained by the first four principal components (PCs): PC1 (cyan), PC2 (red), PC3 (green), and PC4 (yellow). (B) Cumulative variance explained by a control shuffled procedure (data shuffled 1000 times). (C) Series of eigenvectors produced for the first four PCs. Eigenvectors capture the moment-by-moment signals in the subspace composed of reward size and delay. Colors indicated the eigenvectors with a significant magnitude relative to those calculated from the surrogate control PCAs. (D, E) For each PC, eigenvector magnitudes captured the extent of the signals dynamically transmitted (D), while angles (−180 to 180°) indicated how the ensemble integrates reward size and delay (E). (F) Examples of eigenvectors produced by the control shuffled procedure. Percentages of variance explained by the first four PCs were significantly higher than chance (permutation test, p>0.05).
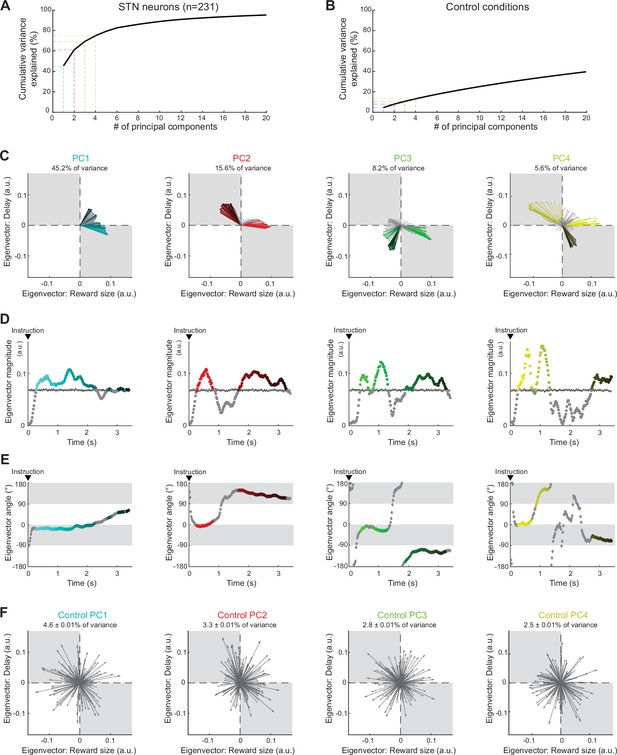
Population-based analysis performed using a sliding window method in the estimation of regression coefficients (200 ms test window stepped in 20 ms).
These figures follow the conventions of Figure 6. (A, B) Cumulative variance explained by principal component analysis (PCA) in the population of all recorded neurons (A), or by the control shuffled procedure (B). (C) Series of eigenvectors produced for the first four principal components (PCs): PC1 (cyan), PC2 (red), PC3 (green), and PC4 (yellow). Eigenvectors capture the moment-by-moment signals in the subspace composed of reward size and delay. Colors indicated the eigenvectors with a significant magnitude relative to those calculated from the population of surrogate control PCs (data shuffled 1000 times). (D, E) For each PC, eigenvector magnitudes captured the strength of the signals transmitted (D), while angles (−180 to 180°) indicated how the ensemble integrates reward size and delay (E). (F) Examples of eigenvectors produced by the control shuffled procedure. Note that the encoding patterns and the percent of variance explained here were very similar to results shown in Figure 6.
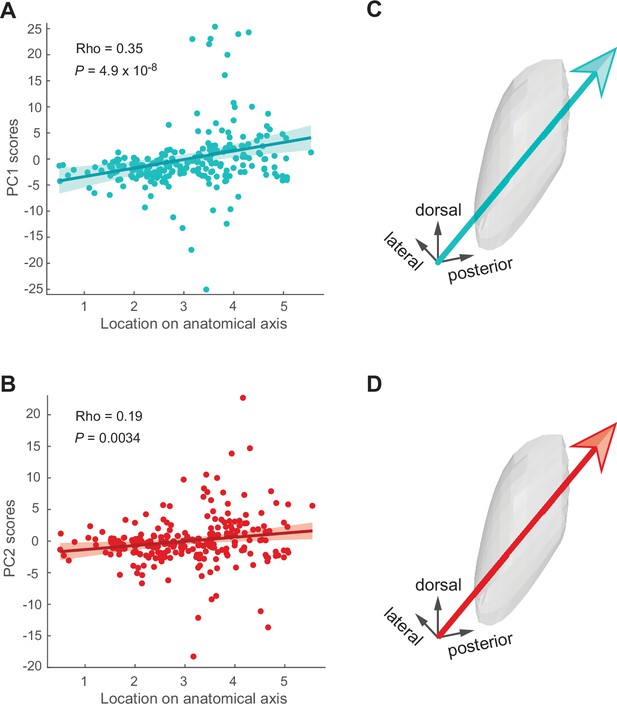
Signals vary along the antero-posterior axis of the subthalamic nucleus (STN).
(A, B) Correlations between component scores (PC1 [cyan] and PC2 [red]) of the neurons and their anatomical position. (C, D) Anatomical axis corresponding the most to PC1 (C) and PC2 (D). Arrows show direction of increasing component scores.