Structural basis for the Rad6 activation by the Bre1 N-terminal domain
Figures
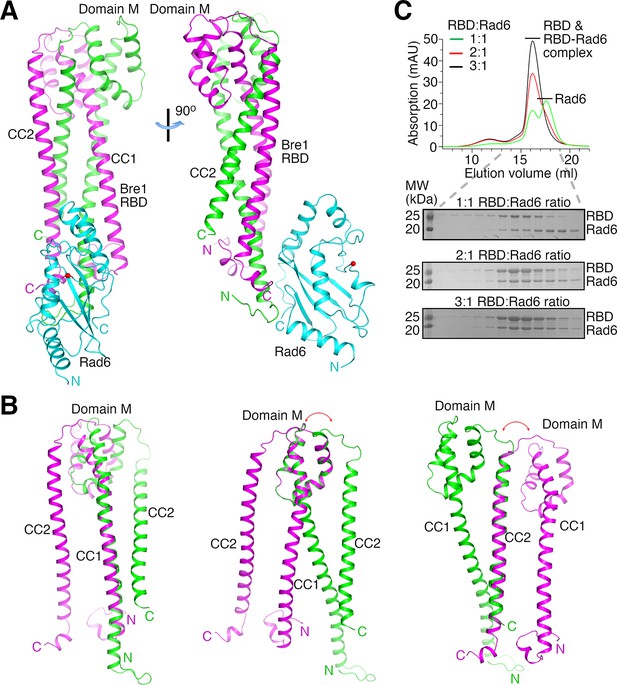
Bre1 Rad6-binding domain (RBD) forms a 2:1 complex with Rad6.
(A) Structure of the KlBre1 RBD–Rad6 complex. The two polypeptides in the KlBre1 RBD are colored in green and magenta, respectively. KlRad6 is colored in cyan. The red spheres indicate the KlRad6 active site. The N- and C-terminus of KlBre1 RBD and KlRad6 are indicated. This coloring scheme is used throughout the manuscript unless indicated otherwise. Structural figures were prepared with PyMOL (https://pymol.org/). (B) Structural comparison of the two polypeptides in the KlBre1 RBD. The CC1 (left), domain M (middle), or CC2 (right) regions in these polypeptides are aligned. The red arrows indicate the drastically different orientation of the domain M–CC2 linker in these polypeptides. (C) Gel filtration analysis of KlBre1 RBD and KlRad6 mixed at different molar ratios. 15 μM of KlRad6 was mixed with KlBre1 RBD (1-206) with the indicated molar ratio, injected to a Superdex 200 10/300 column (GE Healthcare) and eluted with a buffer containing 20 mM Tris (pH 7.5) and 200 mM sodium chloride. The lower panels show sodium dodecyl sulfate–polyacrylamide gel electrophoresis (SDS–PAGE) analysis of the gel filtration experiments. Source data for panel C are provided in Figure 1—source data 1.
-
Figure 1—source data 1
Original gel scan for panel C.
- https://cdn.elifesciences.org/articles/84157/elife-84157-fig1-data1-v2.zip
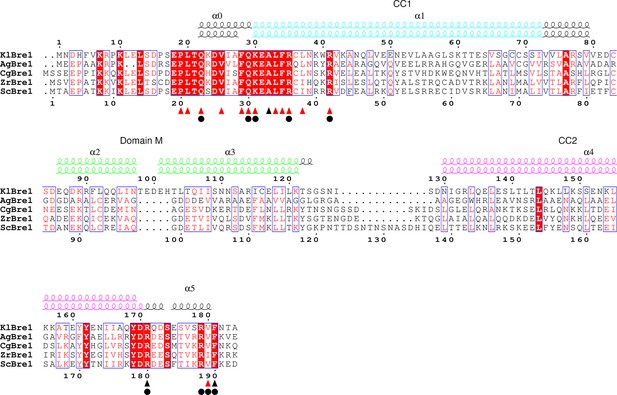
Sequence alignment of the N-terminal region in fungal Bre1 proteins.
Residues numbers for KlBre1 and ScBre1 are indicated above and below the alignment, respectively. Secondary structure elements for the two polypeptides in KlBre1 Rad6-binding domain (RBD) are indicated. The red triangles indicate residues mediating interactions with KlRad6 and between the two KlBre1 polypeptides in the RBD. The black triangles indicate residues mediating interactions with KlRad6 only. The black dots indicate residues substituted in our study. AgBre1, Ashbya gossypii Bre1; CgBre1, Candida glabrata Bre1; ZrBre1, Zygosaccharomyces rouxii Bre1.
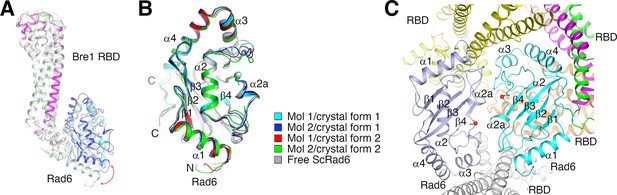
Structure of the KlBre1 Rad6-binding domain (RBD)–Rad6 complexes in the crystal.
(A) Structural alignment of the four complexes found in crystal forms 1 and 2. The KlBre1 RBD in these complexes are aligned. The pairwise root mean square deviations (RMSDs) for the equivalent Cα atoms in these KlBre1 RBD molecules are between 0.77 and 1.39 Å. Complex 1 in crystal form 1 is colored as in Figure 1A, KlRad6 molecule 1 in crystal form 2 is colored in blue, other molecules are colored in gray. The red arrow indicates the large structural difference between KlRad6 molecule 1 in crystal form 2 and other KlRad6 molecules in the crystal. (B) Structural alignment of the four KlRad6 molecules found in crystal forms 1 and 2. Structure of the free ScRad6 (PDB 1AYZ)(Worthylake et al., 1998) is aligned for comparison. The pairwise RMSDs for the equivalent Cα atoms in the four KlRad6 molecules and the free ScRad6 molecule are between 0.62 and 1.16 Å. The spheres indicate Rad6’s active site. (C) KlRad6 in complex 1 of crystal form 2 mediates extensive crystal packing interactions. The complex is colored as in Figure 1A. Protein molecules interacting with the KlRad6 molecule in this complex are highlighted with different colors. The red spheres indicate KlRad6’s active site. RBD, KlBre1 RBD. Structural elements surrounding the active site of this KlRad6 molecule interact with the same region in its symmetry equivalent, burying 1300 Å2 of surface area at the interface. In addition to the KlBre1 RBD dimer it forms a complex with, this KlRad6 molecule also interacts with three KlBre1 RBD dimers in the crystal, burying 880, 680, and 580 Å2 of surface area at the interfaces.
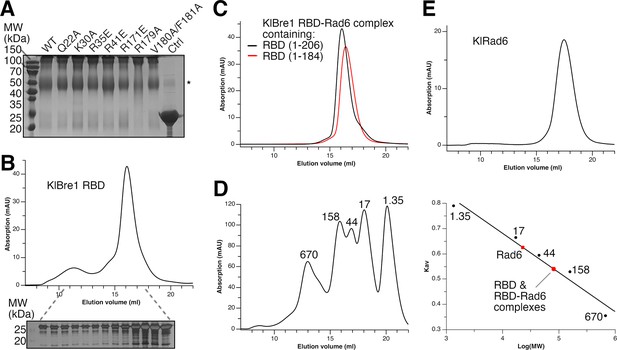
Cross-linking and gel filtration characterization of KlBre1 Rad6-binding domain (RBD), KlRad6, and their complexes.
(A) Cross-linking study of KlBre1 RBD. Sodium dodecyl sulfate–polyacrylamide gel electrophoresis (SDS–PAGE) analysis of the wild-type or substituted KlBre1 RBD (1-206) cross-linked by glutaraldehyde is presented. KlBre1 RBD (1-206) without the cross-linking treatment is included for reference (lane ‘Ctrl’). The * sign indicates the covalently cross-linked KlBre1 dimer. The higher molecular weight cross-linking products presumably represent multiple KlBre1 RBD dimers cross-linked to each other. (B) Gel filtration characterization of KlBre1 RBD. The lower panel shows an SDS–PAGE analysis of the gel filtration experiment. (C) Gel filtration characterization of KlBre1 RBD–Rad6 complexes used for crystallization. (D) Calibration of the gel filtration column. The column is calibrated with thyroglobulin, γ-globulin, ovalbumin, myoglobin, and vitamin B12 (Bio-Rad, their molecule weights are indicated in units of kDa in the figure). The left and right panels show the elution profile of a mixture of these molecules and the calibration curve, respectively. In the right panel, Kav and the logarithm of the molecular weight of these molecules are fitted to a linear equation. Kav is defined as (Ve − Vo)/(Vt − Vo) (Ve, elution volume; Vo, column void volume [7.2 ml]; Vt, total column volume [23.6 ml]). Molecular weight for KlBre1 RBD, KlRad6, and their complexes deduced from the calibration curve is indicated with the red dots. (E) Gel filtration characterization of KlRad6. The gel filtration experiments were performed with a Superdex 200 10/300 column (GE Healthcare) with a buffer containing 20 mM Tris (pH 7.5) and 200 mM sodium chloride. Source data for panels A and B are provided in Figure 1—figure supplement 3—source data 1.
-
Figure 1—figure supplement 3—source data 1
Original gel scans for panels A and B.
- https://cdn.elifesciences.org/articles/84157/elife-84157-fig1-figsupp3-data1-v2.zip
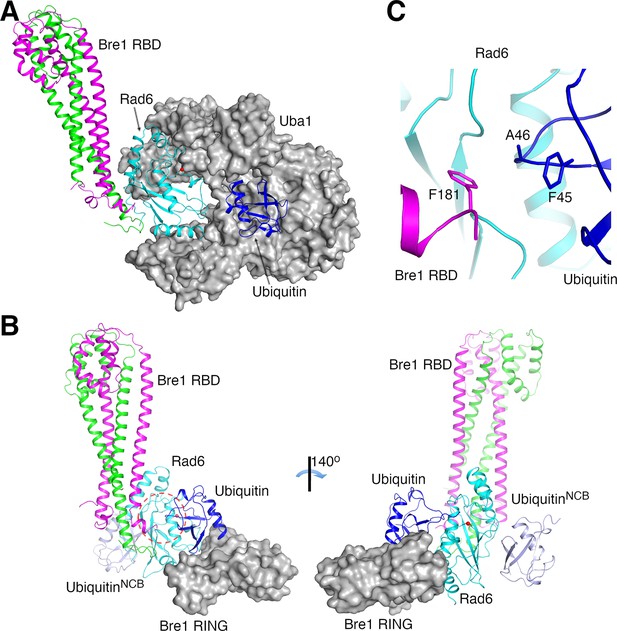
Structural modeling of Bre1 Rad6-binding domain (RBD) during the H2Bub1 catalysis.
(A) Model of the Bre1 RBD–Rad6–E1–ubiquitin complex in the ubiquitin charging reaction. The E1 enzyme (Uba1) and ubiquitin are modeled based on the structure of the Uba1–Ubc4–ubiquitin complex (PDB 4II2) (Olsen and Lima, 2013). (B) Model of the Bre1 RBD–Rad6–ubiquitin–Bre1 RING domain complex in the ubiquitin discharging reaction. Bre1’s RING domain and ubiquitin are modeled based on the structure of the RNF4 RING domain in complex with UbeV2, the ubiquitin–Ubc13 complex in the closed conformation (PDB 5AIT) (Branigan et al., 2015) and the structure of the Bre1 RING domain (PDB 4R7E) (Kumar and Wolberger, 2015). The ubiquitin molecule bound to the non-canonical back side of Rad6 (ubiquitinNCB) (Kumar et al., 2015) is shown. (C) Potential interaction between Bre1 RBD and ubiquitin stabilizing the closed conformation of the Rad6–ubiqutin conjugate. The region marked with the red circle in panel B is shown, important residues are highlighted. Uba1 and the Bre1 RING domain are presented in surface presentation in gray. The red spheres indicate Rad6’s active site.
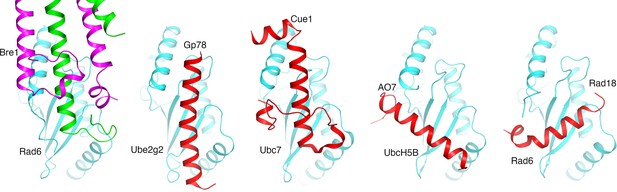
E3 enzymes bind to the back side of E2 enzymes with drastically different mechanisms.
Structures of the KlBre1 Rad6-binding domain (RBD)–Rad6 complex and the E2BBRs in Gp78 (PDB 3H8K) (Das et al., 2009), Cue1 (PDB 4JQU) (Metzger et al., 2013), AO7 (PDB 5D1K) (Li et al., 2015), and Rad18 (PDB 2YBF) Hibbert et al., 2011 in complex with the related E2 enzymes are shown. The E2 enzymes are aligned.
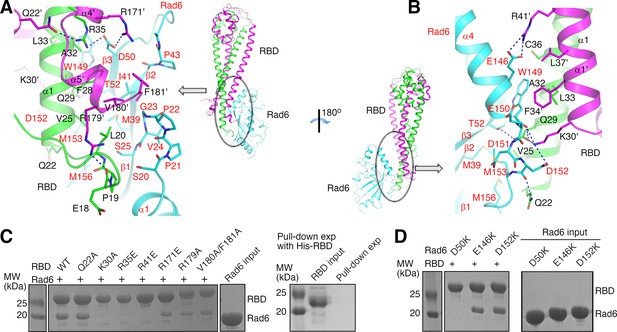
Structural basis of the KlBre1 Rad6-binding domain (RBD)–Rad6 interaction.
(A, B) Structure of the KlBre1 RBD–Rad6 interface. Residues important for the interaction are highlighted. The secondary structure elements for KlBre1 RBD are named as in Figure 1—figure supplement 1. Labels for KlRad6 are in red. Labels with the prime sign are for the KlBre1 RBD polypeptide 2. (C, D) KlBre1 RBD–Rad6 pull-down experiments. Sodium dodecyl sulfate–polyacrylamide gel electrophoresis (SDS–PAGE) analysis of strep-tagged KlBre1 RBD (1-206) precipitated with strep-tactin beads and co-precipitated KlRad6 is shown. A control experiment with his-tagged KlBre1 RBD (1-206) is presented in panel C. RBD, KlBre1 RBD. Source data for panels C and D are provided in Figure 2—source data 1.
-
Figure 2—source data 1
Original gel scans for panels C and D.
- https://cdn.elifesciences.org/articles/84157/elife-84157-fig2-data1-v2.zip
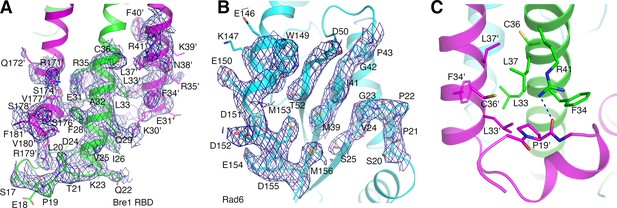
Residues at the KlBre1 Rad6-binding domain (RBD)–Rad6 interface.
Electron density map at the KlBre1 RBD–Rad6 interface in KlBre1 RBD (A) and KlRad6 (B). Refined electron density map for residues in complex 1/crystal form 1 at the interface and surrounding area is shown in blue. The map is contoured at 1 σ. (C) Interactions between polypeptides 1 and 2 in KlBre1 RBD mediated by residues that also participate in the KlBre1 RBD–Rad6 interaction.
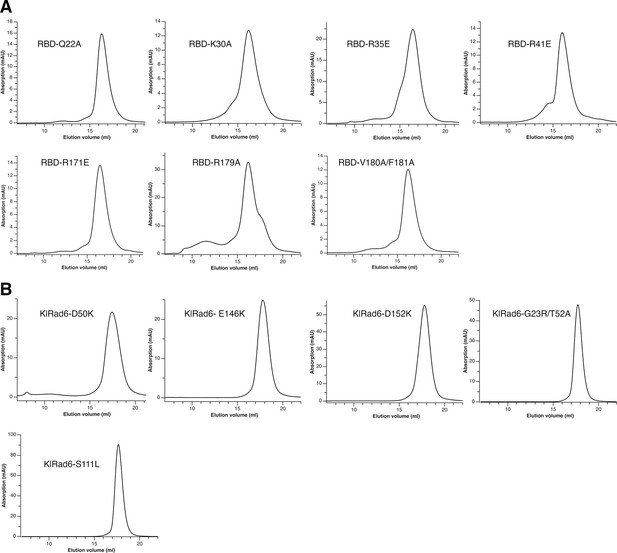
Gel filtration characterization of substituted KlBre1 Rad6-binding domain (RBD) (A) and KlRad6 (B).
The gel filtration experiments were performed with a Superdex 200 10/300 column (GE Healthcare) with a buffer containing 20 mM Tris (pH 7.5) and 200 mM sodium chloride.
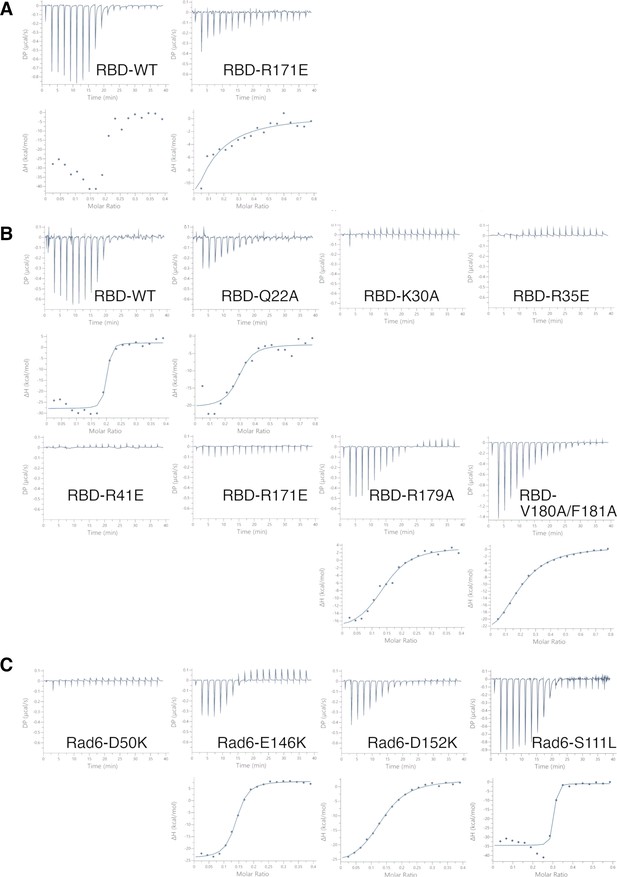
Isothermal titration calorimetry (ITC) experiments.
(A) ITC experiments with 200 mM sodium chloride and the wild-type KlRad6. (B) ITC experiments with 1 M sodium chloride and the wild-type KlRad6. (C) ITC experiments with 1 M sodium chloride and the wild-type KlBre1 Rad6-binding domain (RBD).
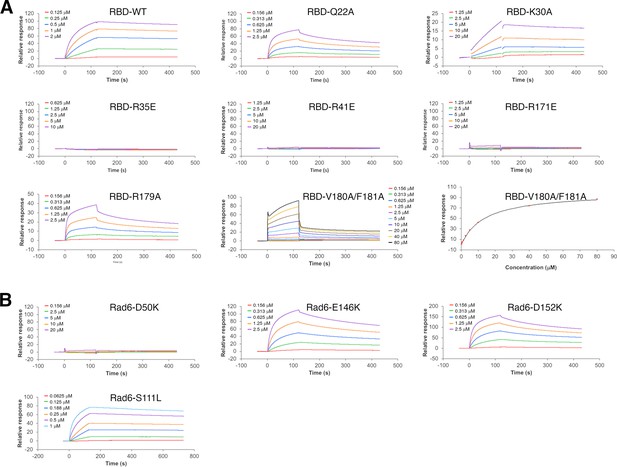
Surface plasmon resonance (SPR) experiments.
(A) SPR experiments with the wild-type KlRad6. Steady-state affinity data analysis for the V180A/F181A-substituted KlBre1 Rad6-binding domain (RBD) is shown. (B) SPR experiments with the wild-type KlBre1 RBD.
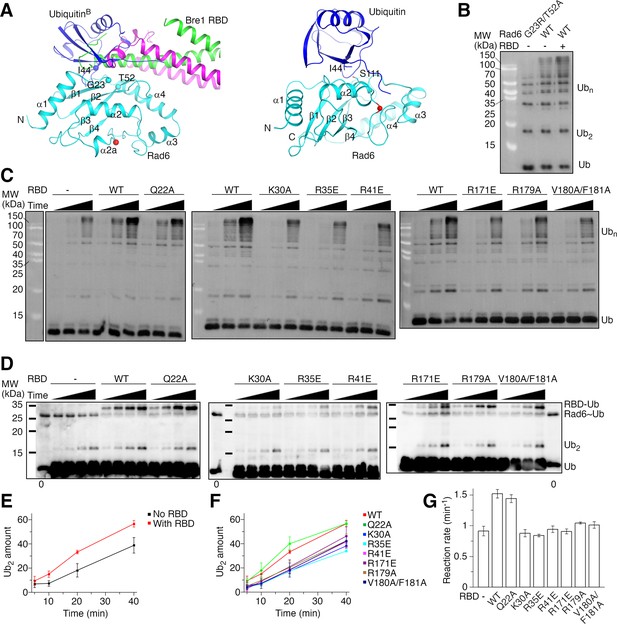
KlBre1 Rad6-binding domain (RBD) stimulates KlRad6’s activity.
(A) Structural modeling of the ubiquitin–KlRad6 interaction. The left panel shows a model of KlRad6 with ubiquitin bound at its back side (ubiquitinB), based on the structure of UbcH5c with ubiquitin bound at its back side (PDB 2FUH) (Brzovic et al., 2006). KlBre1 RBD observed in our structure is shown for reference. The right panel shows a model of the KlRad6–ubiquitin conjugate in the closed conformation, based on the closed conformation of the ubiqtuin–Ubc13 covalent complex (PDB 5ait) (Branigan et al., 2015). Secondary structural elements and the N- and C-termini of KlRad6 are indicated. Important residues are highlighted. The red spheres indicate KlRad6’s active site. (B) Western blot analysis of free ubiquitin chain production by KlRad6. The reactions were carried out for 30 min. KlBre1 RBD (1-206) and ubiquitin chains attached to it were removed before analysis. Ub, ubiquitin; Ub2 and Ubn, ubiquitin chains with 2 or n ubiquitin moieties, respectively. (C) Western blot analysis of free ubiquitin chain production in reactions with ubiquitin (I44A) and KlRad6 (S111L). Reactions carried out for 5, 10, and 20 min are presented. KlBre1 RBD (1-206) and ubiquitin chains attached to it were removed before analysis. Two independent repeats of the experiments were performed. (D) Western blot analysis of the Rad6–ubiquitin discharging reaction. ubiquitin (I44A/K0) charged to KlRad6 (S111L) was discharged to ubiquitin (I44A) in the absence or presence of KlBre1 RBD (1-206). The reactions were allowed to proceed for 5, 10, 20, and 40 min and analyzed by non-reducing sodium dodecyl sulfate–polyacrylamide gel electrophoresis (SDS–PAGE) followed by western blot for ubiquitin. In lanes marked with ‘0’, western blot analysis of the KlRad6–ubiqtuin conjugate prior to the discharging reaction is presented. Three independent repeats of the experiments were performed. (E, F) Quantification of the di-ubiquitin production in the KlRad6–ubiquitin discharging reactions. The intensity of the di-ubiquitin band divided by the intensity of the Rad6–ubiqtuin band in lane ‘0’ of the same blot times 100 was calculated to represent the di-ubiquitin amount. The error bars represent standard deviations of three independent experiments. Band intensities were read with ImageJ. (G) The discharge reaction rate. Data presented in panels E and F were fitted to a linear equation and the slope is used to represent the reaction rate. Errors were derived from data fitting. Source data for panels B–D are provided in Figure 3—source data 1, for panels E–G are provided in Figure 3—source data 2.
-
Figure 3—source data 1
Original gel scans for panels B-D.
- https://cdn.elifesciences.org/articles/84157/elife-84157-fig3-data1-v2.zip
-
Figure 3—source data 2
Data points for panels E-G.
- https://cdn.elifesciences.org/articles/84157/elife-84157-fig3-data2-v2.xlsx
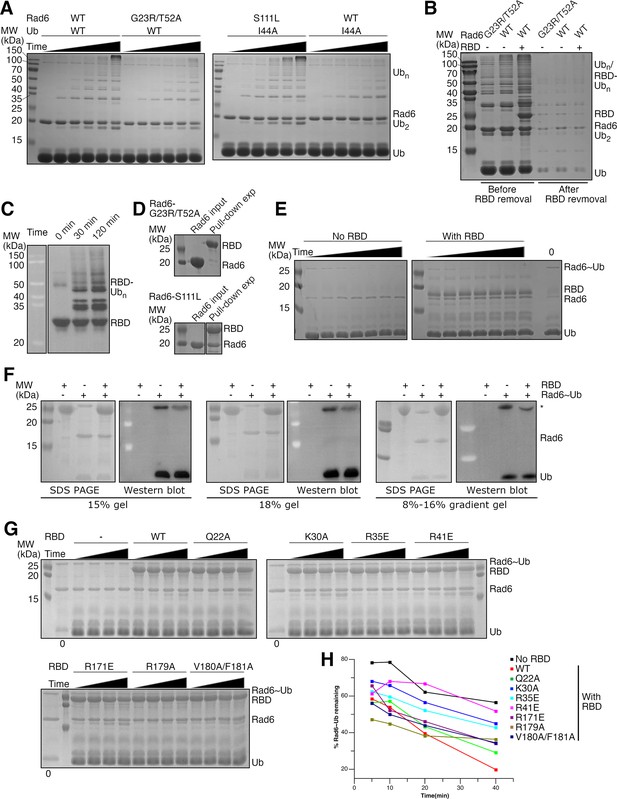
Rad6 activity assays.
(A, B) Ubiquitin chain production by KlRad6. Sodium dodecyl sulfate–polyacrylamide gel electrophoresis (SDS–PAGE) analysis of the reactions are presented. Experiments presented in panel A were carried out without KlBre1 Rad6-binding domain (RBD) for 0, 5, 10, 20, 30, and 60 min. Reactions presented in panel B were carried out for 30 min. Ub, ubiquitin; Ub2 and Ubn, ubiquitin chains with 2 or n ubiquitin moieties, respectively. (C) KlBre1 RBD can be ubiquitinated. Western blot analysis against the strep-tagged KlBre1 RBD (1-206) after the ubiquitin chain formation reaction is presented. The reactions were allowed to proceed for the indicated time. (D) Pull-down experiments probing the KlBre1 RBD–Rad6 interaction. SDS–PAGE analysis of the experiments with the G23R/T52A- and S111L-substituted KlRad6 are presented. (E) Non-reducing SDS–PAGE analysis of the KlRad6–ubiquitin discharging reaction. The lane marked with ‘0’ shows analysis of the KlRad6–ubquitin conjugate prior to the reaction. Wild-type ubiquitin, KlRad6 and KlBre1 RBD (1-184) were used for the reactions, which were allowed to proceed for 10, 20, 40, 70, 100, 130, and 150 min before analysis. (F) KlBre1 RBD (1-206) interferes with western blot detection of the KlRad6–ubquitin conjugate. The same KlRad6–ubquitin conjugate sample was analyzed with non-reducing SDS–PAGE and western blot for ubiquitin. 30 μM of KlBre1 RBD (1-206) or the same volume of protein buffer was added before analysis. An analysis for KlBre1 RBD (1-206) is included for comparison. KlBre1 RBD (1-206) reduces the signal level of the KlRad6–ubiquitin conjugate in western blot. The star sign indicates the KlRad6–ubiquitin conjugate and KlBre1 RBD. (G) SDS–PAGE analysis for the KlRad6–ubiquitin conjugate. The conjugate in discharging reactions with KlRad6 (S111L), ubiquitin (I44A/K0), and ubiquitin (I44A) was analyzed with non-reducing SDS–PAGE with 18% gels. Reactions were allowed to proceed for 5, 10, 20, and 40 min. Lanes marked with ‘0’ show the conjugate prior to the discharging reaction. When indicated, wild-type or substituted KlBre1 RBD (1-206) was supplemented. (H) Quantification of the KlRad6–ubiquitin conjugate signal. The intensity of the conjugate signal in panel G was read by ImageJ and plotted. Source data for panels A–G are provided in Figure 3—figure supplement 1—source data 1, for panel H is provided in Figure 3—figure supplement 1—source data 2.
-
Figure 3—figure supplement 1—source data 1
Original gel scans and blots for panels A-G.
- https://cdn.elifesciences.org/articles/84157/elife-84157-fig3-figsupp1-data1-v2.zip
-
Figure 3—figure supplement 1—source data 2
Data points for panel H.
- https://cdn.elifesciences.org/articles/84157/elife-84157-fig3-figsupp1-data2-v2.xlsx
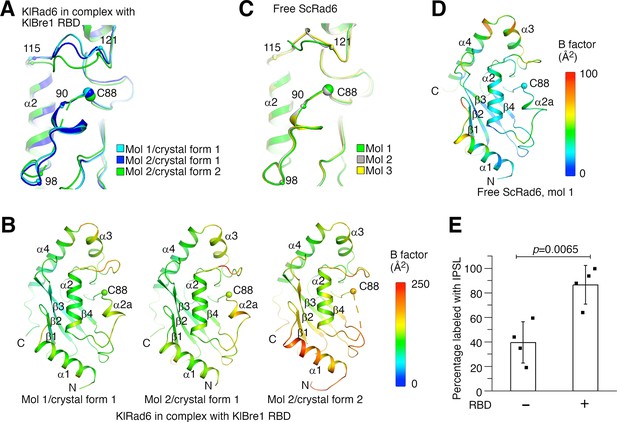
Bre1 Rad6-binding domain (RBD) increases Rad6’s active site accessibility.
(A) Structural comparison of KlRad6 molecules in our crystals. Regions surrounding the active site (C88) in KlRad6 molecules not mediating extensive crystal packing interactions are shown. The Cα positions of residues 90, 98, 115, and 121 are indicated. (B) Temperature factor distribution of KlRad6 molecules in our crystals. The structures of KlRad6 molecules not mediating extensive crystal packing interactions are colored according to the temperature factor. The average temperature factor for regions 90–98 and 115–121 in KlRad6 molecules 1 and 2 in crystal form 1 and molecule 2 in crystal form 2 are 143.7, 156.4, and 173.5 Å2, respectively, the average temperature factor for the rest of these molecules are 104.6, 117.1, and 149.2 Å2. (C) Structural comparison of ScRad6 molecules in the crystal structure of free ScRad6 (PDB 1AYZ) (Worthylake et al., 1998). (D) Temperature factor distribution of ScRad6 in the crystal structure of free ScRad6. Molecule 1 in the crystal is presented. The average temperature factor for its regions 90–98 and 115–121 is 45.7 Å2, for the rest of the molecule is 42.6 Å2. (E) KlBre1 RBD increase KlRad6’s active site accessibility. 3-(2-Iodoacetamido)-2,2,5,5-tetramethyl-1-pyrolidinyloxy (IPSL) labeling of KlRad6’s active site cysteine is presented. The error bars represent standard deviations of four independent experiments (represented by the black dots). The p value is derived from the two-sided Student’s t-test. Source data for panel E are provided in Figure 4—source data 1.
-
Figure 4—source data 1
Data points for panel E.
- https://cdn.elifesciences.org/articles/84157/elife-84157-fig4-data1-v2.xlsx
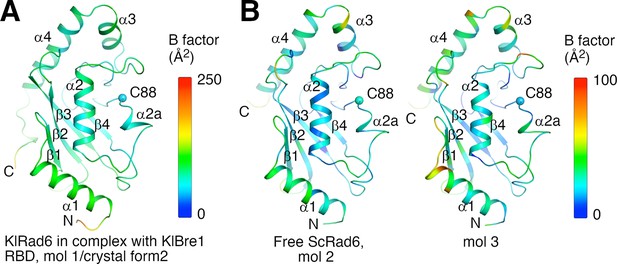
Temperature factor distribution of Rad6 molecules in crystal structures.
(A) Temperature factor distribution of KlRad6 molecule 1 in crystal form 2 in our study. (B) Temperature factor distribution of molecules 2 and 3 in the crystal structure of ScRad6.The average temperature factor for their regions 90–98 and 115–12 are 39.8 and 44.5 Å2, respectively; for the rest of the molecules are 34.7 and 38.1 Å2, respectively. The active site (C88) of the Rad6 molecules are indicated.
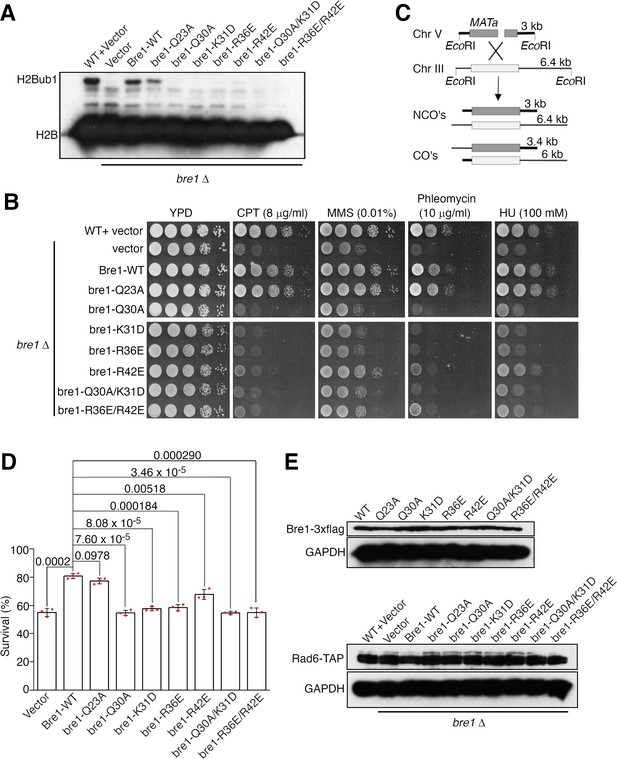
The Bre1 Rad6-binding domain (RBD)–Rad6 interaction is crucial for Bre1’s function inside the cell.
(A) The Bre1 RBD–Rad6 interaction is essential for the H2Bub1 formation inside the cell. Western blot analysis for H2Bub1 in BRE1 knock out cells complemented with pRS316-derived plasmids carrying the wild-type or substituted ScBre1 is shown. Data for the wild-type or BRE1 knock out cells complemented with the empty vector are included for comparison. (B) The Bre1 RBD–Rad6 interaction is essential for survival in the presence of replication stress/DNA damaging reagents. Sensitivity of BRE1 knock out cells complemented with pRS16-derived plasmids for the wild-type or substituted ScBre1 is shown. The cells were challenged with camptothecin (CPT), methylmethane sulfonate (MMS), phleomycin, or hydroxy urea (HU). Data for the wild-type or BRE1 knock out cells complemented with the empty vector are included for comparison. (C) Scheme showing the ectopic recombination system. CO, crossover; NCO, non-crossover. (D) The Bre1 RBD–Rad6 interaction is essential for the HR repair. Survival rate of BRE1 knock out cells complemented with the wild-type or substituted ScBre1 due to successful HR repair is shown. Data for cells complemented with the empty vector are included for comparison. Error bars represent standard deviations of three independent experiments (represented by the red dots). p values derived from the two-sided Student’s t-test are presented. (E) Substitutions in ScBre1 do not alter the Bre1 or Rad6 protein levels. Western blot analysis for Bre1 and Rad6 is presented. Western blot analysis for glyceraldehyde-3-phosphate dehydrogenase (GAPDH) is included as reference. Source data for panels A and E are provided in Figure 5—source data 1, for panel D is provided in Figure 5—source data 2.
-
Figure 5—source data 1
Original blots for panels A and E.
- https://cdn.elifesciences.org/articles/84157/elife-84157-fig5-data1-v2.zip
-
Figure 5—source data 2
Data points for panel D.
- https://cdn.elifesciences.org/articles/84157/elife-84157-fig5-data2-v2.xlsx
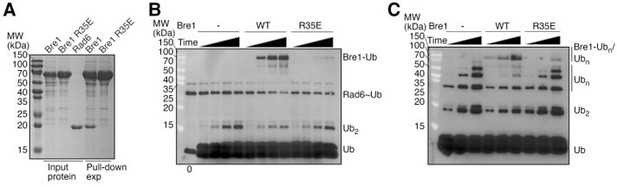
Biochemical characterization of the full length KlBre1.
The full length KlBre1 gene was inserted into vector pET26B, with a strep-tag engineered to its N-terminus. The protein was expressed in E. coli BL21 Rosetta (DE3) cells and purified by strep-tactin (Smart Lifesciences), Heparin (Hitrap Heparin HP, GE healthcare) and gel filtration (Superose 6 10/300, GE heathcare) columns and stored in a buffer containing 20 mM Tris (pH 7.5), 200 mM sodium chloride and 2 mM DTT. (A) KlBre1-Rad6 pull down experiments. The reaction mixture contains 10 μM of KlBre1 and 3.2 μM of KlRad6 and the reactions were performed similar to the KlBre1 RBD-Rad6 pull-down experiments described in the manuscript. SDS PAGE analysis of KlBre1 precipitated with strep-tactin beads and co-precipitated KlRad6 is shown. Analysis of the input proteins are shown on the left. (B) KlRad6~ubiquitin discharging experiments. Reactions analyzed by non-reducing SDS PAGE followed by western blot for ubiquitin are presented. The reactions were performed similar to the discharging reactions with KlRad6 (S111L), ubiquitin (K0/I44A) and ubiquitin (I44A) presented in the manuscript. They were allowed to proceed for 0, 10, 20 and 40 minutes. When indicated, KlBre1 is added to a final concentration of 15 μM. (C) Ubiquitin chain production experiments. The reactions were performed similar to the ubiquitin chain production experiments with KlRad6 (S111L) and ubiquitin (I44A) presented in the manuscript. When indicated, KlBre1 was added to a final concentration of 18 μM. Reactions were allowed to proceed for 5, 10 and 20 minutes before analysis by western blot for ubiquitin.
Tables
Data collection and structure refinement statistics.
Crystal form 1 | Crystal form 2 | |
---|---|---|
Data collection | ||
Space group | P6522 | P6122 |
Cell dimensions | ||
a, b, c (Å) | 94.44, 94.44, 534.64 | 113.22, 113.22, 386.17 |
α, β, γ (°) | 90, 90, 120 | 90, 90, 120 |
Resolution (Å) | 50–3.20 (3.26–3.2) | 50–3.05 (3.10–3.05) |
Rmerge | 0.200 (1.797) | 0.092 (0.863) |
I /σI | 17.0 (2.0) | 21.6 (1.1) |
CC1/2 | 0.985 (0.623) | 1.00 (0.876) |
Completeness (%) | 100.0 (99.0) | 99.7 (96.9) |
Redundancy | 18.0 (18.5) | 20.6 (16.7) |
Refinement | ||
Resolution (Å) | 40.89–3.20 (3.35–3.20) | 32.18–3.05 (3.11–3.05) |
No. reflections | 22,676 (2465) | 28,706 (2495) |
Rwork / Rfree (%) | 21.32/26.33 (32.57/39.34) | 23.72/28.60 (40.29/46.90) |
No. atoms | ||
Protein | 7563 | 7486 |
Ligand/ion | 0 | 17 |
B-factors | ||
Protein | 107.63 | 122.41 |
Ligand/ion | – | 118.27 |
R.m.s. deviations | ||
Bond lengths (Å) | 0.003 | 0.003 |
Bond angles (°) | 0.599 | 0.645 |
-
Values in parentheses are for the highest resolution shell.
Summary of isothermal titration calorimetry (ITC) experiments.
KlBre1 RBD | KlRad6 | N | KD (μM) | ΔH (kcal/mol) | TΔS (kcal/mol) | ΔG (kcal/mol) |
---|---|---|---|---|---|---|
With 200 mM salt | ||||||
WT | WT | * | ||||
R171E | WT | 0.047 ± 0.366 | 13.9 ± 31.2 | −80 ± 704 | −73.4 | −6.63 |
With 1 M salt | ||||||
WT | WT | 0.191 ± 0.003 | 0.012 ± 0.009 | −30.0 ± 1.32 | −19.1 | −10.8 |
Q22A | WT | 0.280 ± 0.02 | 0.207 ± 0.182 | −18.3 ± 2.38 | −9.16 | −9.12 |
K30A | WT | ND | ND | ND | ND | ND |
R35E | WT | ND | ND | ND | ND | ND |
R41E | WT | ND | ND | ND | ND | ND |
R171E | WT | ND | ND | ND | ND | ND |
R179A | WT | 0.142 ± 0.007 | 0.819 ± 0.312 | −22.6 ± 2.23 | −14.3 | −8.31 |
V180A/F181A | WT | 0.193 ± 0.005 | 7.64 ± 0.903 | −31.6 ± 1.78 | −24.6 | −6.98 |
WT | D50K | ND | ND | ND | ND | ND |
WT | S111L | 0.289 ± 0.004 | 0.007 ± 0.008 | −33.6 ± 1.52 | −22.5 | −11.1 |
WT | E146K | 0.134 ± 0.002 | 0.138 ± 0.021 | −32.3 ± 0.63 | −22.9 | −9.37 |
WT | D152K | 0.134 ± 0.002 | 0.827 ± 0.105 | −30.4 ± 1.02 | −22.1 | −8.30 |
-
ND, not detectable.
-
*
Unreliable data fitting.
Summary of surface plasmon resonance (SPR) experiments.
KlBre1 RBD | KlRad6 | ka (1/Ms) | kd (1/s) | KD (μM) |
---|---|---|---|---|
WT | WT | (1.42 ± 0.002) × 104 | (2.05 ± 0.01) × 10−4 | 0.0144 |
Q22A | WT | (2.41 ± 0.009) × 104 | (1.52 ± 0.004) × 10−3 | 0.0633 |
K30A | WT | (7.88 ± 0.04) × 102 | (3.55 ± 0.04) × 10−4 | 0.451 |
R35E | WT | ND | ND | ND |
R41E | WT | ND | ND | ND |
R171E | WT | ND | ND | ND |
R179A | WT | (3.20 ± 0.02) × 104 | (1.66 ± 0.005) × 10−3 | 0.0517 |
V180A/F181A | WT | * | 13.7 | |
WT | D50K | ND | ND | ND |
WT | S111L | (2.79 ± 0.005) × 104 | (1.47 ± 0.006) × 10−4 | 0.00529 |
WT | E146K | (1.82 ± 0.005) × 104 | (1.32 ± 0.002) × 10−3 | 0.0726 |
WT | D152K | (2.35 ± 0.007) × 104 | (1.51 ± 0.003) × 10−3 | 0.0641 |
-
ND, not detectable.
-
*
Steady-state affinity analysis was performed.
Reagent type (species) or resource | Designation | Source or reference | Identifiers | Additional information |
---|---|---|---|---|
Strain, strain background (Saccharomyces cerevisiae) | JKM139 | Lee et al., 1998 | ||
Strain, strain background (Saccharomyces cerevisiae) | tGI354 | Ira et al., 2003 | ||
Strain, strain background (Saccharomyces cerevisiae) | yZSH241 | This study | bre1::TRP1 | Parental strain: tGI354 |
Strain, strain background (Saccharomyces cerevisiae) | yMF001 | This study | bre1::TRP1 + pRS316-bre1-Q23A | Parental strain: tGI354 |
Strain, strain background (Saccharomyces cerevisiae) | yMF002 | This study | bre1::TRP1 + pRS316-bre1-Q30AK31D | Parental strain: tGI354 |
Strain, strain background (Saccharomyces cerevisiae) | yMF003 | This study | bre1::TRP1 + pRS316-bre1-R36ER42E | Parental strain: tGI354 |
Strain, strain background (Saccharomyces cerevisiae) | yMF004 | This study | bre1::TRP1 + pRS316 | Parental strain: tGI354 |
Strain, strain background (Saccharomyces cerevisiae) | yMF005 | This study | bre1::TRP1 + pRS316-BRE1 | Parental strain: tGI354 |
Strain, strain background (Saccharomyces cerevisiae) | yMF006 | This study | bre1::TRP1 + pRS316-bre1-Q23A | Parental strain: JKM139 |
Strain, strain background (Saccharomyces cerevisiae) | yMF007 | This study | bre1::TRP1 + pRS316-bre1-Q30AK31D | Parental strain: JKM139 |
Strain, strain background (Saccharomyces cerevisiae) | yMF008 | This study | bre1::TRP1 + pRS316-bre1-R36ER42E | Parental strain: JKM139 |
Strain, strain background (Saccharomyces cerevisiae) | yMF009 | This study | bre1::TRP1 + pRS316 | Parental strain: JKM139 |
Strain, strain background (Saccharomyces cerevisiae) | yMF010 | This study | bre1::TRP1 + pRS316-BRE1 | Parental strain: JKM139 |
Strain, strain background (Saccharomyces cerevisiae) | yLD002 | This study | bre1::TRP1 | Parental strain: JKM139 |
Strain, strain background (Saccharomyces cerevisiae) | yMF011 | This study | KanMX-FLAG-H2B bre1::TRP1 + pRS316-bre1-Q23A | Parental strain: JKM139 |
Strain, strain background (Saccharomyces cerevisiae) | yMF012 | This study | KanMX-FLAG-H2B bre1::TRP1 + pRS316-bre1-Q30AK31D | Parental strain: JKM139 |
Strain, strain background (Saccharomyces cerevisiae) | yMF013 | This study | KanMX-FLAG-H2B bre1::TRP1 + pRS316-bre1-R36ER42E | Parental strain: JKM139 |
Strain, strain background (Saccharomyces cerevisiae) | yMF014 | This study | KanMX-FLAG-H2B bre1::TRP1 + pRS316 | Parental strain: JKM139 |
Strain, strain background (Saccharomyces cerevisiae) | yMF015 | This study | KanMX-FLAG-H2B bre1::TRP1 + pRS316-BRE1 | Parental strain: JKM139 |
Strain, strain background (Saccharomyces cerevisiae) | yGX060 | This study | KanMX-FLAG-H2B bre1::TRP1 | Parental strain: JKM139 |
Strain, strain background (Saccharomyces cerevisiae) | yZS059 | This study | bre1::TRP1 + pRS316-bre1-Q30A | Parental strain: tGI354 |
Strain, strain background (Saccharomyces cerevisiae) | yZS060 | This study | bre1::TRP1 + pRS316-bre1-K31D | Parental strain: tGI354 |
Strain, strain background (Saccharomyces cerevisiae) | yZS061 | This study | bre1::TRP1 + pRS316-bre1-R36E | Parental strain: tGI354 |
Strain, strain background (Saccharomyces cerevisiae) | yZS062 | This study | bre1::TRP1 + pRS316-bre1-R42E | Parental strain: tGI354 |
Strain, strain background (Saccharomyces cerevisiae) | yZS063 | This study | bre1::TRP1 + pRS316-bre1-Q30A | Parental strain: JKM139 |
Strain, strain background (Saccharomyces cerevisiae) | yZS064 | This study | bre1::TRP1 + pRS316-bre1-K31D | Parental strain: JKM139 |
Strain, strain background (Saccharomyces cerevisiae) | yZS065 | This study | bre1::TRP1 + pRS316-bre1-R36E | Parental strain: JKM139 |
Strain, strain background (Saccharomyces cerevisiae) | yZS066 | This study | bre1::TRP1 + pRS316-bre1-R42E | Parental strain: JKM139 |
Strain, strain background (Saccharomyces cerevisiae) | yZS067 | This study | bre1::TRP1 + pRS316-BRE1-3FLAG | Parental strain: JKM139 |
Strain, strain background (Saccharomyces cerevisiae) | yZS068 | This study | bre1::TRP1 + pRS316-bre1-Q23A-3FLAG | Parental strain: JKM139 |
Strain, strain background (Saccharomyces cerevisiae) | yZS069 | This study | bre1::TRP1 + pRS316-bre1-Q30A-3FLAG | Parental strain: JKM139 |
Strain, strain background (Saccharomyces cerevisiae) | yZS070 | This study | bre1::TRP1 + pRS316-bre1-K31D-3FLAG | Parental strain: JKM139 |
Strain, strain background (Saccharomyces cerevisiae) | yZS071 | This study | bre1::TRP1 + pRS316-bre1-R36E-3FLAG | Parental strain: JKM139 |
Strain, strain background (Saccharomyces cerevisiae) | yZS072 | This study | bre1::TRP1 + pRS316-bre1-R42E-3FLAG | Parental strain: JKM139 |
Strain, strain background (Saccharomyces cerevisiae) | yZS073 | This study | bre1::TRP1 + pRS316-bre1-Q30AK31D-3FLAG | Parental strain: JKM139 |
Strain, strain background (Saccharomyces cerevisiae) | yZS074 | This study | bre1::TRP1 + pRS316-bre1-R36ER42E-3FLAG | Parental strain: JKM139 |
Strain, strain background (Saccharomyces cerevisiae) | yZS075 | Dharmacon (Yeast TAP Tagged ORFs) | RAD6-TAP-HIS | Parental strain: By4741 |
Strain, strain background (Saccharomyces cerevisiae) | yZS076 | This study | RAD6-TAP-HIS bre1::KanMX | Parental strain: By4741 |
Strain, strain background (Saccharomyces cerevisiae) | yZS077 | This study | RAD6-TAP-HIS+pRS316 | Parental strain: By4741 |
Strain, strain background (Saccharomyces cerevisiae) | yZS078 | This study | RAD6-TAP-HIS bre1::KanMX + pRS316 | Parental strain: By4741 |
Strain, strain background (Saccharomyces cerevisiae) | yZS079 | This study | RAD6-TAP-HIS bre1::KanMX + pRS316-BRE1 | Parental strain: By4741 |
Strain, strain background (Saccharomyces cerevisiae) | yZS080 | This study | RAD6-TAP-HIS bre1::KanMX + pRS316-bre1-Q23A | Parental strain: By4741 |
Strain, strain background (Saccharomyces cerevisiae) | yZS081 | This study | RAD6-TAP-HIS bre1::KanMX + pRS316-bre1-Q30A | Parental strain: By4741 |
Strain, strain background (Saccharomyces cerevisiae) | yZS082 | This study | RAD6-TAP-HIS bre1::KanMX + pRS316-bre1-K31D | Parental strain: By4741 |
Strain, strain background (Saccharomyces cerevisiae) | yZS083 | This study | RAD6-TAP-HIS bre1::KanMX + pRS316-bre1-R36E | Parental strain: By4741 |
Strain, strain background (Saccharomyces cerevisiae) | yZS084 | This study | RAD6-TAP-HIS bre1::KanMX + pRS316-bre1-R42E | Parental strain: By4741 |
Strain, strain background (Saccharomyces cerevisiae) | yZS085 | This study | RAD6-TAP-HIS bre1::KanMX + pRS316-bre1-Q30AK31D | Parental strain: By4741 |
Strain, strain background (Saccharomyces cerevisiae) | yZS086 | This study | RAD6-TAP-HIS bre1::KanMX + pRS316-bre1-R36ER42E | Parental strain: By4741 |
Antibody | anti-ubiquitin (Mouse monoclonal) | Santa Cruz Biotechnology | Cat# sc-8017, RRID: AB_628423 | 1:2000 |
Antibody | anti-strep (Mouse monoclonal) | ABclonal | Cat# AE066, RRID: AB_2863792 | 1:5000 |
Antibody | anti-FLAG (Mouse monoclonal) | Sigma-Aldrich | Cat# F3165, RRID: AB_259529 | 1:3000 |
Antibody | anti-FLAG (Mouse monoclonal) | Sigma-Aldrich | Cat# F1804, RRID: AB_262044 | 1:3000 |
Antibody | anti-TAP (Mouse monoclonal) | Zen-BioScience | Cat# 250067 | 1:6000 |
Antibody | anti-GAPDH (Mouse monoclonal) | ABclonal | Cat# AC033, RRID: AB_2769570 | 1:50,000 |
Peptide, recombinant protein | HRP-conjugated mouse IgGκ light chain binding protein | Santa Cruz Biotechnology | Cat# sc-516102, RRID: AB_2687626 | 1:6000 |