LSD1 defines the fiber type-selective responsiveness to environmental stress in skeletal muscle
Figures
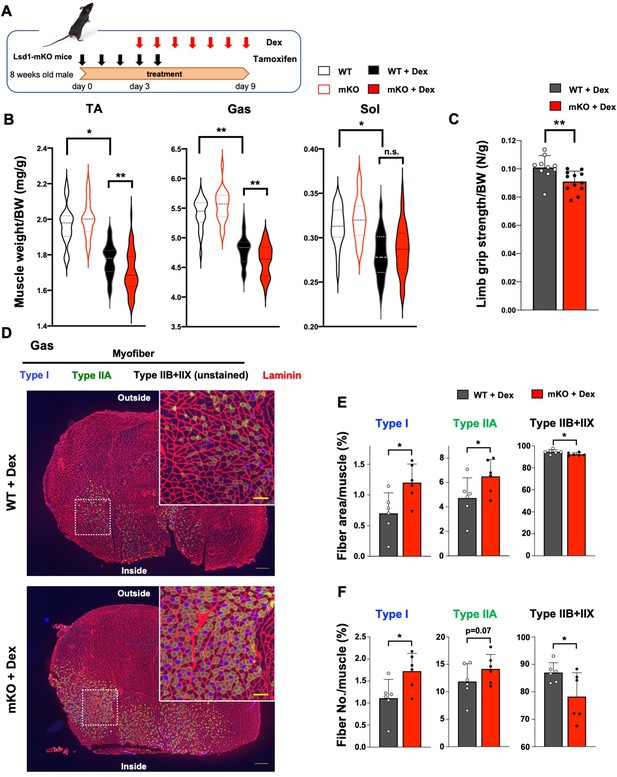
Muscle-specific lysine-specific demethylase-1 (LSD1)-knockout exacerbates dexamethasone (Dex)-induced fast muscle atrophy.
(A) Experimental design: LSD1 deletion was induced by tamoxifen injection for 5 days (days 0–4), and Dex was administered for 7 days (days 3–9) at 1 mg/kg. (B) Muscle weight normalized by the body weight. Tibialis anterior (TA), gastrocnemius (Gas), and soleus (Sol) muscles were obtained from Dex-treated wild type (WT) (n=40) and LSD1-mKO (n=44) mice. In the control set, WT (n=22) and mKO (n=22) mice that were not subjected to Dex treatment were sacrificed 1 month after the first tamoxifen administration. Box-plot elements (center line, median; box limits, upper and lower quartiles; whiskers, min to max). (C) All-limb grip strength of Dex-treated WT (n=10) and LSD1-mKO (n=12) mice. (D) Immunofluorescence staining of individual fiber types in Gas muscles of WT and LSD1-mKO mice after Dex treatment. Left: cross section of the whole tissue (scale bars, 300 µm). Right: magnified images of dotted squares in the images on the left (scale bars, 100 µm). Type I fiber (blue), type IIA fiber (green), and laminin (red) were stained. The fibers that were negative for types I and IIA were defined as type IIB+IIX. (E) Occupancy of each fiber type based on the cross-sectional area (WT, n=6; mKO, n=6). (F) Frequency of each muscle fiber type based on the fiber number (WT, n=6, mKO, n=6). Values are mean ± standard deviation (SD). *p<0.05, **p<0.01.
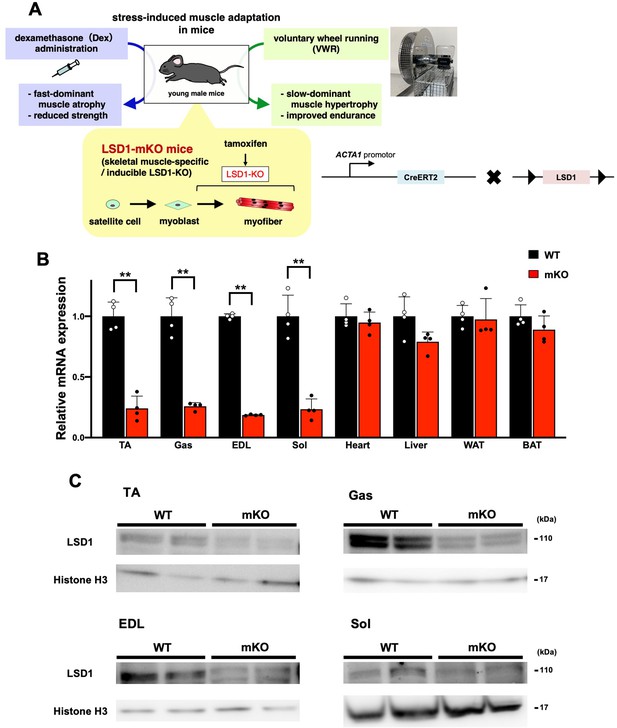
Generation of lysine-specific demethylase-1 (LSD1)-mKO mice.
(A) LSD1-mKO mice. Upon tamoxifen administration, LSD1 was depleted in differentiating and differentiated muscle cells, in which human ACTA1 promoter was activated. We performed the dexamethasone (Dex) administration and voluntary wheel running (VWR) tests, which induced muscle site-dependent adaptive responses. LSD1-mKO mice were generated by crossing Lsd1-floxed mice and knock-in mice expressing a CreERT2 recombinase under the control of human ACTA1 gene promoter. (B) LSD1 mRNA expression in wild type (WT) (n=4) and LSD1-mKO (n=4) mice. Quantitative reverse transcription-polymerase chain reaction (qRT-PCR) values were normalized to the 36B4 gene and presented as the fold differences, compared with those in WT samples. (C) LSD1 protein expression in muscles from LSD1-mKO mice. Values are mean ± SD. **p<0.01. ACTA1, actin alpha 1; TA, tibialis anterior muscle; Gas; gastrocnemius muscle; EDL, extensor digitorum longus muscle; Sol, soleus muscle; WAT, white adipose tissue; BAT, brown adipose tissue.
-
Figure 1—figure supplement 1—source data 1
Original blots for Figure 1—figure supplement 1C.
- https://cdn.elifesciences.org/articles/84618/elife-84618-fig1-figsupp1-data1-v1.zip
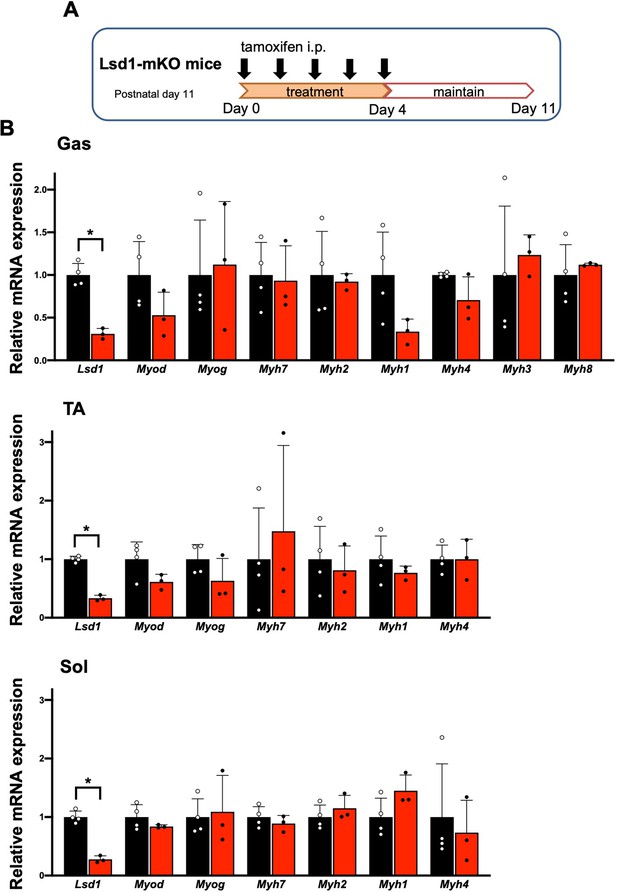
Effect of lysine-specific demethylase-1 (LSD1)-mKO at the postnatal developmental stage.
(A) Experimental design: postnatal day 11 male wild type (WT) or LSD1-mKO mice were injected with tamoxifen for 5 consecutive days. (B) The expression of differentiation markers of muscle fiber and fiber type-specific genes in the gastrocnemius (Gas), tibialis anterior (TA), and soleus (Sol) muscle from WT (n=4) and LSD1-mKO (n=3) mice. Quantitative reverse transcription-polymerase chain reaction (qRT-PCR) values are shown as the fold differences against those in the WT. Full descriptions of gene symbols are provided in the Supplementary file 3. Values are mean ± SD. *p<0.05.
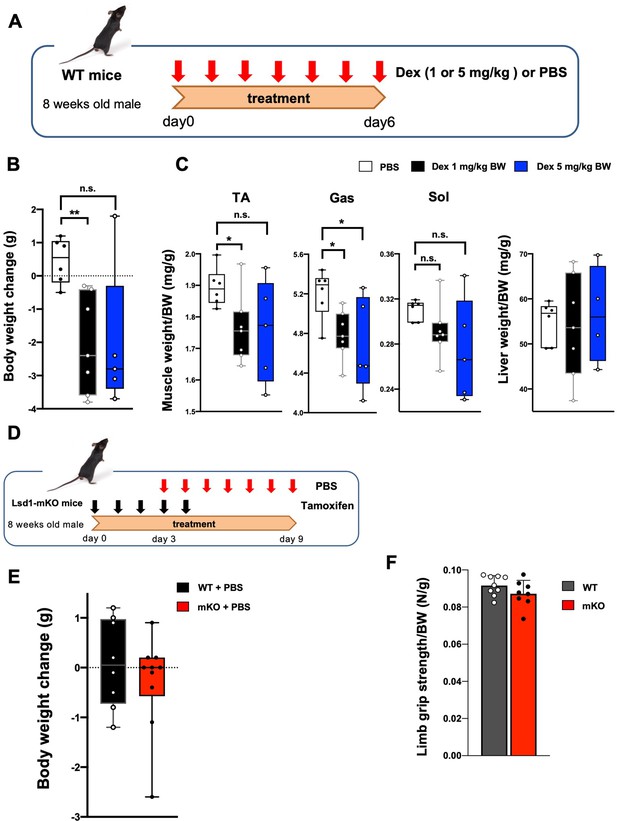
Effect of dexamethasone (Dex) dosage on atrophic phenotype.
(A) Experimental design: 8-week-old male wild type (WT) mice were injected with phosphate buffered saline (PBS) or Dex (1 or 5 mg/kg) for 7 days, consecutively. To arrange an experimental setting compatible with the knockout (KO) mouse tests, we administered tamoxifen intraperitoneally to WT mice for 5 consecutive days and the PBS/Dex injection started at the fourth day of tamoxifen administration. (B, C) Body weight change from day 0 to day 6 (B) and tissue weights (C) in mice treated with PBS (n=6), 1 mg/kg body weight (BW) (n=7) or 5 mg/kg BW (n=5) Dex in (A).(D) Experimental design: 8-week-old male WT or lysine-specific demethylase-1 (LSD1)-mKO mice were injected with PBS for 7 days. To arrange an experimental setting compatible with the Dex mouse tests, we administered tamoxifen intraperitoneally to WT mice for 5 consecutive days and the PBS injection started at the fourth day of tamoxifen administration. (E) BW change from day 0 to day 9 of the experiment in Figure 3D. (F) All-limb grip strength of WT (n=9) and LSD1-mKO (n=8) mice. Values are mean ± SD. *p<0.05.
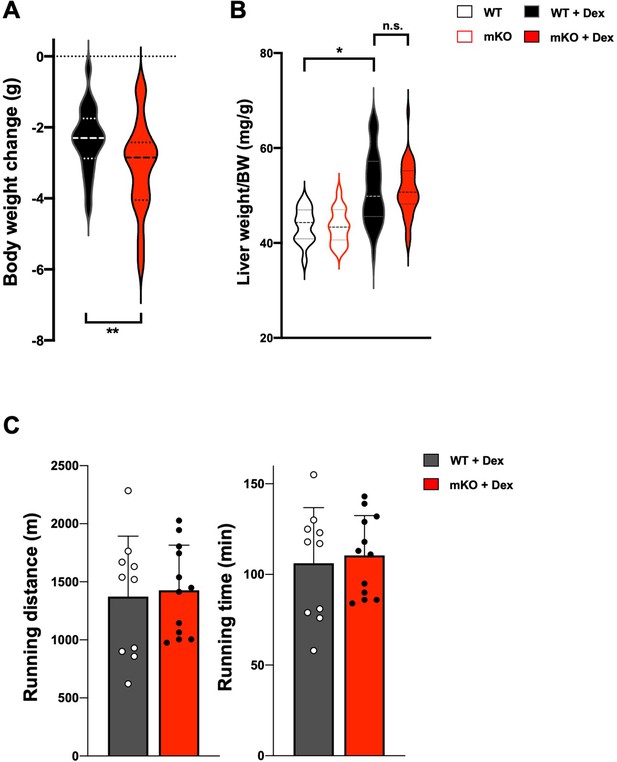
Effects of dexamethasone (Dex) treatment in lysine-specific demethylase-1 (LSD1)-mKO mice.
(A) Changes in body weight during the Dex administration period (days 3–9) in wild type (WT) (n=40) and LSD1-mKO (n=44) mice. (B) Liver weight in WT (n=14) and LSD1-mKO (n=9) mice without Dex administration, and WT (n=15) and LSD1-mKO (n=39) mice with Dex administration. (C) Treadmill performance of Dex-treated WT (n=10) and LSD1-mKO (n=12) mice. Running distance and time until exhaustion are shown. Values are mean ± SD. *p<0.05, **p<0.01.
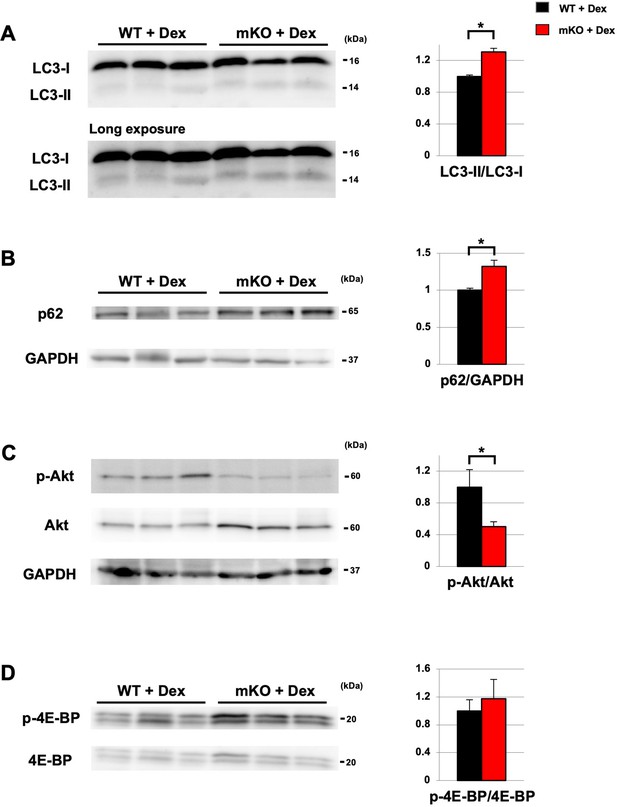
Effects of lysine-specific demethylase-1 (LSD1)-mKO on muscle atrophy- and hypertrophy-associated signaling pathways.
(A) Western blot analysis of an autophagy protein LC3 in EDL muscles from dexamethasone (Dex)-treated wild type (WT) and LSD1-mKO mice. Autophagic signaling was evaluated based on the LC3-II/LC3-I ratio. (B) Western blot analysis of an autophagy protein p62 and GAPDH protein in EDL muscles from Dex-treated WT and LSD1-mKO mice. Autophagic signaling was evaluated based on the p62/GAPDH ratio. (C) Western blot analysis of phosphorylated (p)-Akt, total Akt, and GAPDH protein in WT and LSD1-mKO EDL muscles after Dex treatment. Hypertrophic signaling was evaluated based on the p-Akt/total Akt ratio. (D) Western blot analysis of p-4E-BP and total 4E-BP protein in WT and LSD1-mKO EDL muscles after Dex treatment. Hypertrophic signaling was evaluated based on the p-4E-BP/total 4E-BP ratio. Band densities were quantified by densitometry. Values are mean ± SD, and shown as the fold difference against WT.
-
Figure 1—figure supplement 5—source data 1
Original blots for Figure 1—figure supplement 5.
- https://cdn.elifesciences.org/articles/84618/elife-84618-fig1-figsupp5-data1-v1.zip
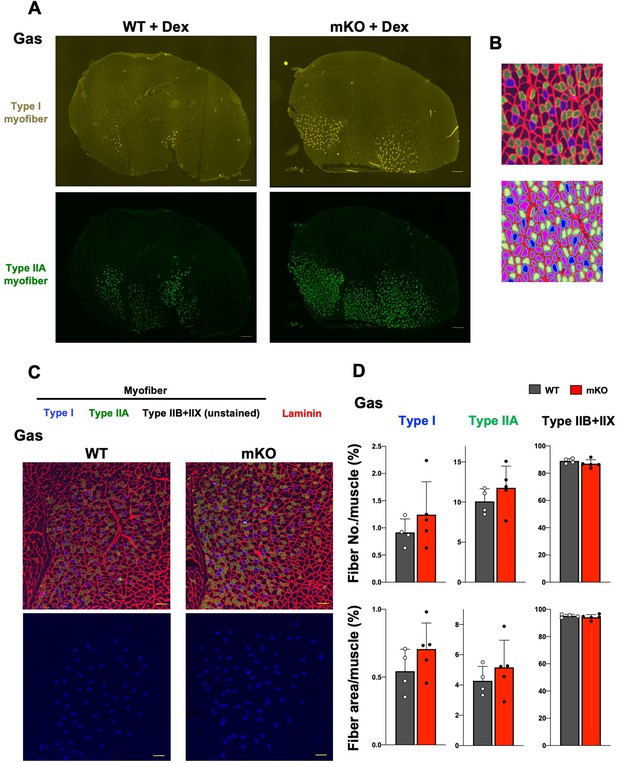
Increase in the number of slow fibers in dexamethasone (Dex)-treated lysine-specific demethylase-1 (LSD1)-mKO mice.
(A) Single color images of type I and IIA fibers shown in Figure 1D. Scale bars, 300 μm. (B) (Upper panel) The cross-sectional areas and numbers of each fiber type were determined by analyzing the individual fibers that were visualized by laminin staining. (Lower panel) Fiber areas depicted in blue (type I), yellow (IIA), and magenta (IIB+IIX) were detected by a BZ-X Analyzer software (KEYENCE). (C) Staining of individual fiber types in gastrocnemius (Gas) muscles from wild type (WT) and LSD1-mKO mice after tamoxifen administration (without Dex treatment). Scale bars, 100 μm. Representative images are shown. (D) Occupancy and frequency of each muscle fiber type based on the fiber cross-sectional area and number (WT, n=4, mKO, n=5).
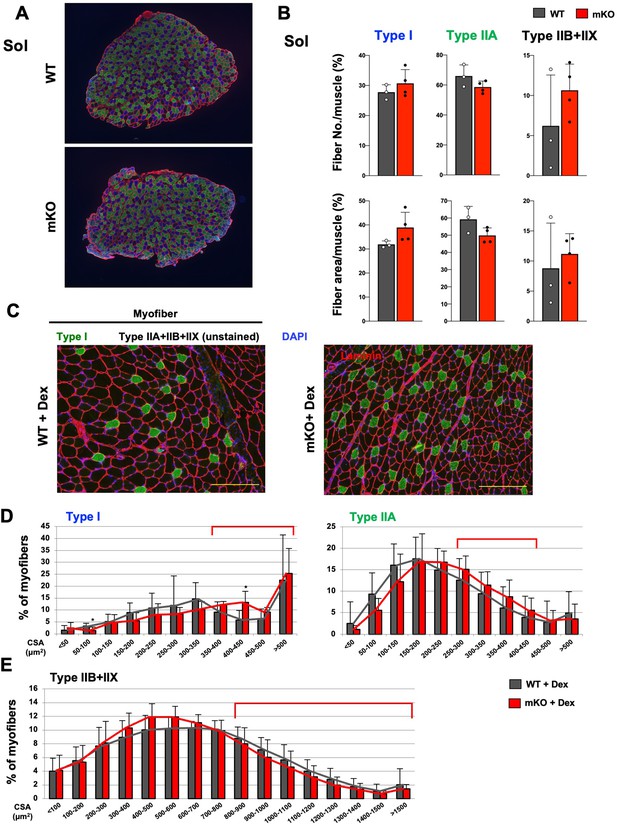
Fiber-type composition in dexamethasone (Dex)-treated lysine-specific demethylase-1 (LSD1)-mKO mice.
(A) Staining of individual fiber types in soleus (Sol) muscles from wild type (WT) and LSD1-mKO mice after tamoxifen administration (without Dex treatment). Scale bars, 100 μm. Representative images are shown. (B) Occupancy and frequency of each muscle fiber type based on the fiber cross-sectional area and number (WT, n=3, mKO, n=4). (C) Staining of the nuclei in gastrocnemius (Gas) muscles from Dex-treated WT and LSD1-mKO mice. Note that 5,6-diamidino-2-phenylindole (DAPI)-positive nuclei are located at the periphery of the fibers. Scale bars, 200 μm. (D, E) Size distributions of type I, type IIA (E), and type IIB+IIX fibers (F) (WT, n=6; mKO, n=6). Large type I and IIA fibers preferentially increased in LSD1-mKO muscle (D), while large type IIB+IIX fibers decreased (E) as highlighted by red bars.
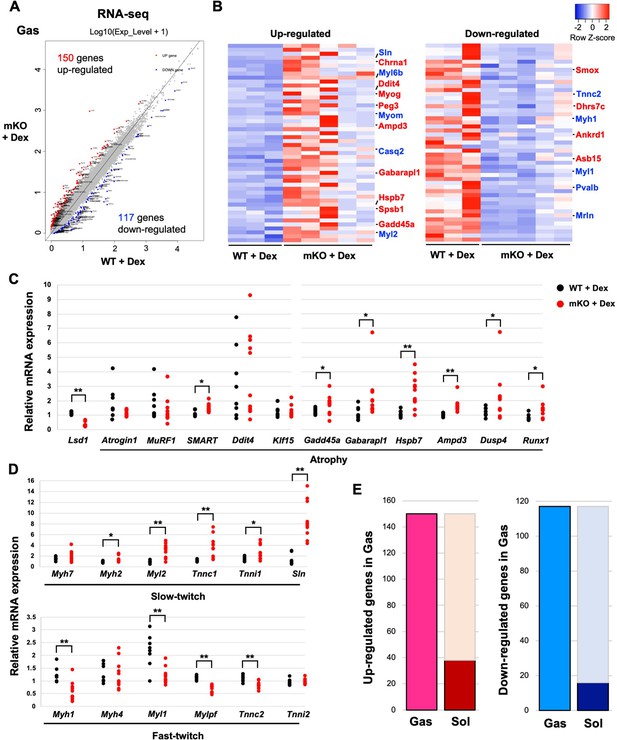
Transcriptome analysis reveals the upregulation of muscle atrophy and slow fiber genes in fast-dominant muscles in dexamethasone (Dex)-treated lysine-specific demethylase-1 (LSD1)-mKO mice.
(A) Comparison of the transcriptome data from LSD1-mKO and wild type (WT) gastrocnemius (Gas) muscles. The red and blue dots indicate significantly up- and downregulated genes, respectively, in LSD1-mKO (FDR >0.05). (B) Top 50 differentially expressed genes ranked by the FDR Z-score. In the upregulated gene list, muscle atrophy- (red) and slow fiber-associated (blue) genes are highlighted. In the downregulated list, muscle hypertrophy- (red) and fast fiber-associated genes (blue) are highlighted. The complete gene list is provided in Supplementary file 1. (C and D) Quantitative reverse transcription-polymerase chain reaction (qRT-PCR) analysis of atrophy- (C) and fiber type-associated genes (D) in the Dex-treated LSD1-mKO Gas muscles (WT, n=8; mKO, n=11). Expression levels were normalized to those of the 36B4 gene and shown as fold differences against the WT. Full descriptions of gene symbols are provided in Supplementary file 3. (E) Comparison of the transcriptome profiles of Gas and soleus (Sol) muscles from Dex-treated LSD1-mKO mice. The dark red and blue bars indicate the fraction of genes that are commonly upregulated and downregulated, respectively, in the mKO Gas and Sol muscles. The numbers of genes that were up- or downregulated in mKO Gas are also indicated. Values are mean ± SD. *p<0.05, **p<0.01.
-
Figure 2—source data 1
Lists of genes described in Figure 2A.
- https://cdn.elifesciences.org/articles/84618/elife-84618-fig2-data1-v1.xlsx
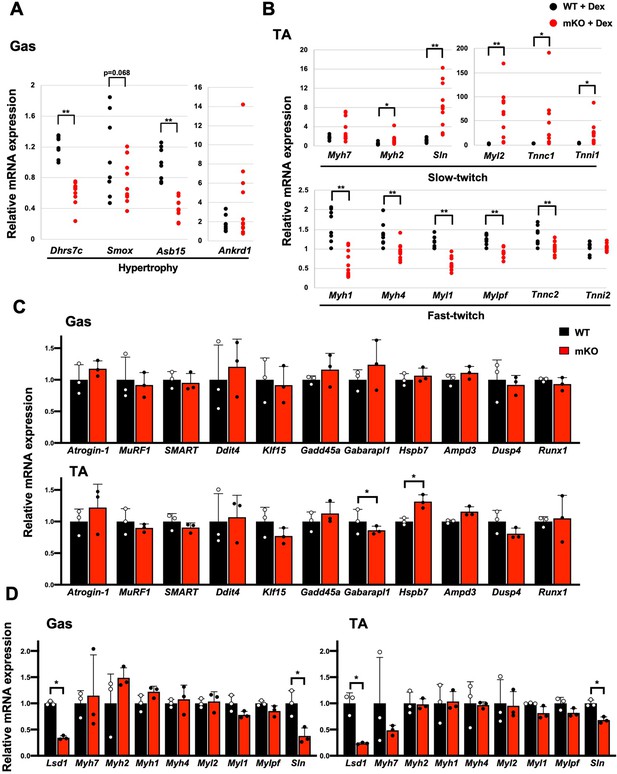
Effects of lysine-specific demethylase-1 (LSD1)-mKO on the expression of atrophy-, hypertrophy-, and fiber type-associated genes.
(A, B) Gene expression profiles in the muscles from dexamethasone (Dex)-treated wild type (WT) (n=8) and LSD1-mKO (n=11) mice. The expression of hypertrophy-associated genes in the gastrocnemius (Gas) muscle (A) and fiber type-specific genes in the tibialis anterior (TA) muscle (B) are shown. Quantitative reverse transcription-polymerase chain reaction (qRT-PCR) values are shown as the fold differences against those in the WT. (C, D) Gene expression profiles in the muscle from WT (n=3) and LSD1-mKO (n=3) mice without Dex treatment. Gas and TA muscles were dissected from the mice 4 weeks after the start of tamoxifen administration. The expression of atrophy-associated genes (C) and fiber type-specific genes (D) are shown. qRT-PCR values are shown as the fold differences against the WT. Full descriptions of gene symbols are provided in the Supplementary file 3. Values are mean ± SD. *p<0.05, **p<0.01.
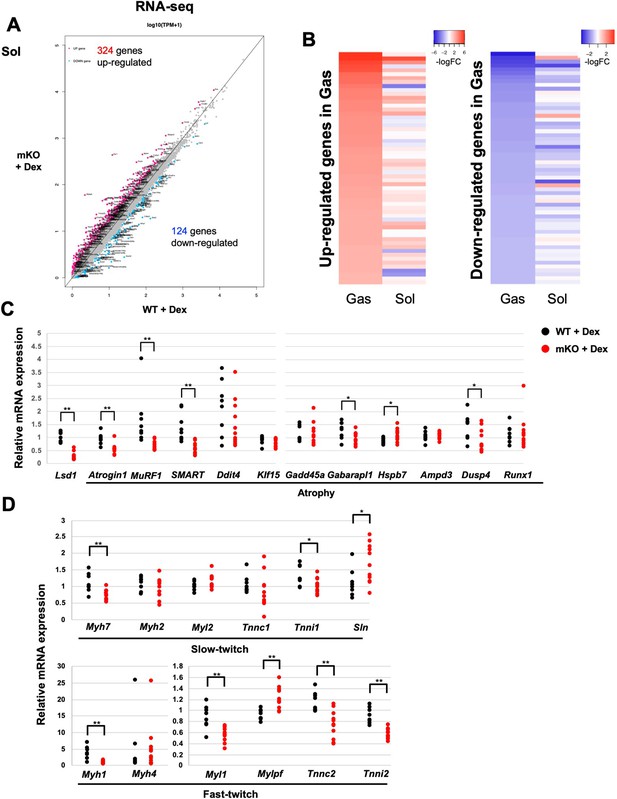
Transcriptome analysis of the soleus (Sol) muscle in lysine-specific demethylase-1 (LSD1)-mKO mice after dexamethasone (Dex) treatment.
(A) Comparison of the transcriptome data of the muscles from the Dex-treated WT (n=3) and LSD1-mKO (n=3) mice. The magenta and cyan dots represent the genes that were significantly upregulated and downregulated, respectively, in LSD1-mKO muscles, respectively (FDR >0.05). (B) Comparison of transcriptome profiles of gastrocnemius (Gas) and Sol muscles from Dex-treated LSD1-mKO mice. Genes that were significantly upregulated or downregulated in the LSD1-mKO Gas muscle are aligned in the heatmap according to the -logFC values against those in the WT. Alongside the Gas heatmap, -logFC values of corresponding genes in the Sol (mKO vs. WT) are indicated. (C, D) The expression of the atrophy-associated genes (C) and fiber type-specific genes (D) in the Sol muscle from Dex-treated WT (n=8) and LSD1-mKO (n=11) mice. Quantitative reverse transcription-polymerase chain reaction (qRT-PCR) values are shown as the fold differences against those in the WT. Full descriptions of gene symbols are provided in the Supplementary file 3. Values are mean ± SD. *p<0.05, **p<0.01.
-
Figure 2—figure supplement 2—source data 1
Lists of genes described in Figure 2—figure supplement 2A.
- https://cdn.elifesciences.org/articles/84618/elife-84618-fig2-figsupp2-data1-v1.xlsx
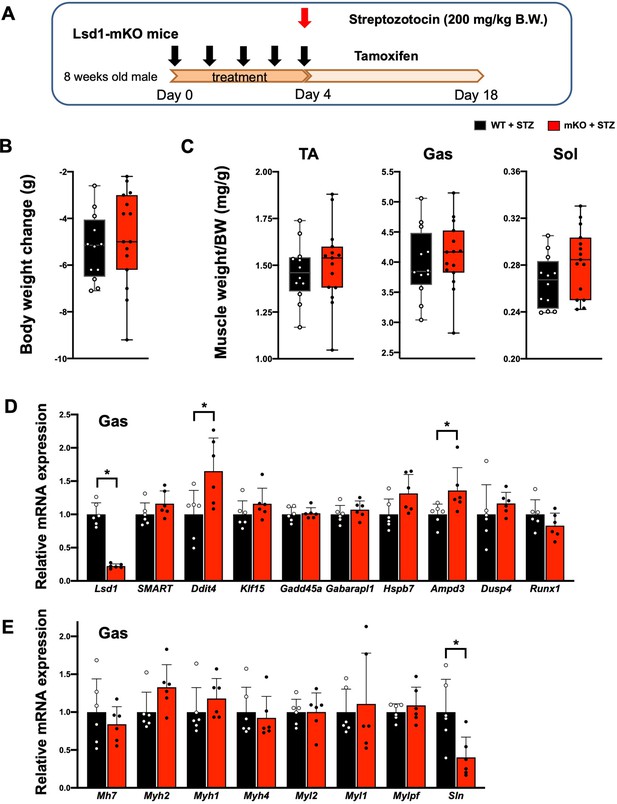
The role of lysine-specific demethylase-1 (LSD1) in streptozotocin (STZ)-induced muscle atrophy.
(A) Experimental design: 8-week-old male wild type (WT) or LSD1-mKO mice were administered tamoxifen intraperitoneally to WT mice for 5 consecutive days and the STZ was intraperitoneally injected at the fifth day of tamoxifen administration. (B, C) Body weight changes from day 0 to 18 (B), and tissue weights (C) in WT (n=12) and LSD1-mKO (n=15) mice treated with STZ. (D, E) The expression of the atrophy-associated genes (D) and fiber type-specific genes (E) in the gastrocnemius (Gas) muscle from STZ-treated WT (n=6) and LSD1-mKO (n=6) mice. Quantitative reverse transcription-polymerase chain reaction (qRT-PCR) values are shown as the fold differences against those in the WT. Full descriptions of gene symbols are provided in the Supplementary file 3. Values are mean ± SD. *p<0.05.
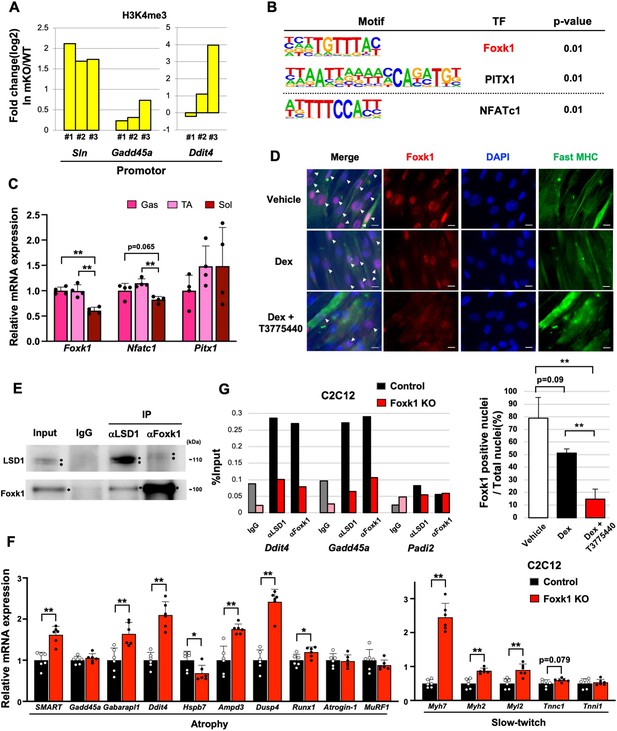
Lysine-specific demethylase-1 (LSD1) regulates the expression of atrophy-associated genes through the modulation of Foxk1.
(A) Chromatin immunoprecipitation (ChIP)-qPCR analyses of H3K4me3 at Sln, Gadd45a, and Ddit4 gene promoters in LSD1-mKO gastrocnemius (Gas) muscles after dexamethasone (Dex) treatment. The data are from three independent experiments. The enrichment levels of H3K4me3 were normalized to the input DNA and presented as fold differences in the LSD1-mKO against that of wild type (WT). (B) Motif analyses of the promoter regions of genes that were upregulated in Dex-treated LSD1-mKO Gas muscles. Analysis of the atrophy-associated genes identified Foxk1 and PITX1 motifs, while significant enrichment of NFATc1 motif was found when all the upregulated genes were scanned. (C) Expression of genes encoding the transcription factors (TFs) identified by the motif analyses. Quantitative reverse transcription-polymerase chain reaction (qRT-PCR) values (n=4) are shown as the fold differences, compared with those of the Gas muscles. (D) Effects of treatment with Dex and LSD1 inhibitor (T-3775440) on the expression of Foxk1 in C2C12 myotubes. Foxk1 and 5,6-diamidino-2-phenylindole (DAPI) co-staining revealed Foxk1-positive nuclei, which are indicated by arrowheads (scale bars, 20 μm). The graph shows the percentage of Foxk1-positive nuclei (n=3). (E) Co-immunoprecipitation of LSD1 and Foxk1. C2C12 myotubes were treated with insulin for 6 hr before harvest to enhance the nuclear retention of Foxk1. Input lane contains 10% amount of the whole-cell extract. (F) Effects of Foxk1-KO on the expression of atrophy- and slow fiber-associated genes in C2C12 myotubes (n=6). qRT-PCR values are shown as the fold differences, compared with those in the control-transfected cells (n=6). (G) ChIP-qPCR analyses of LSD1 and Foxk1 at Ddit4, Gadd45a, Padi2 (negative control) gene promoters in control and Foxk1-KO C2C12 myotubes. The enrichment levels of normal rabbit IgG, LSD1, and Foxk1 were normalized to the input DNA. Values are mean ± SD. *p<0.05, **p<0.01.
-
Figure 3—source data 1
Original blots for Figure 3E.
- https://cdn.elifesciences.org/articles/84618/elife-84618-fig3-data1-v1.zip
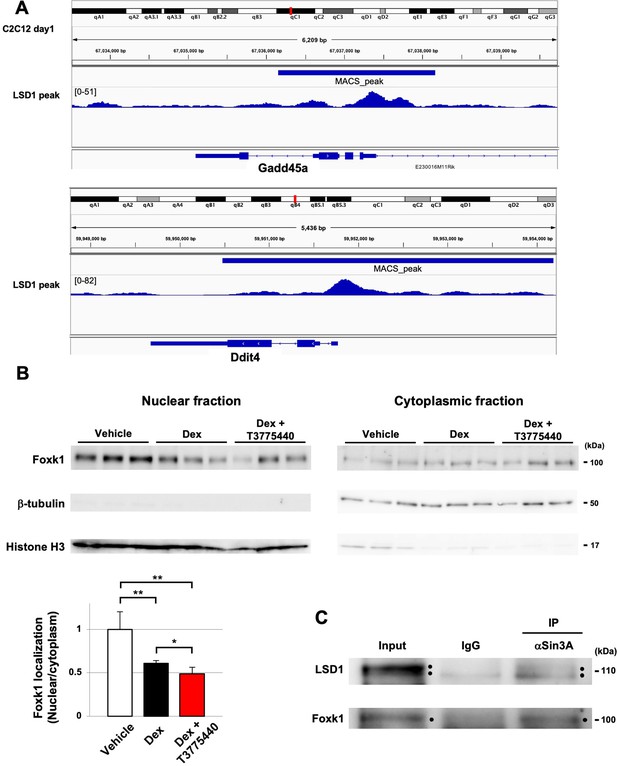
Lysine-specific demethylase-1 (LSD1) interacts with Foxk1 and increases the nuclear retention.
(A) Enrichment of LSD1 at the atrophy gene loci in C2C12 myoblasts. Chromatin immunoprecipitation (ChIP)-seq data reported by Tosic M et al. (Nat. Commun. 9, No. 366, 2018) was analyzed by Integrative Genomics Viewer (IGV). (B) Western blot analysis of Foxk1, β-tubulin, and histone H3 protein in the nuclear and cytoplasmic fraction of C2C12 myotubes treated with dexamethasone (Dex) or Dex+T3775440. The graph shows the Foxk1 localization which is evaluated by nuclear Foxk1/Histone H3 ratio/cytoplasmic Foxk1/β-tubulin ratio. (C) Co-immunoprecipitation of Sin3A and LSD1 or Foxk1. C2C12 myotubes were treated with insulin for 6 hr before harvest to enhance the nuclear retention of Foxk1. Input lane contains 10% amount of the whole-cell extract. Values are mean ± SD. *p<0.05, **p<0.01.
-
Figure 3—figure supplement 1—source data 1
Original blots for Figure 3—figure supplement 1B and C.
- https://cdn.elifesciences.org/articles/84618/elife-84618-fig3-figsupp1-data1-v1.zip
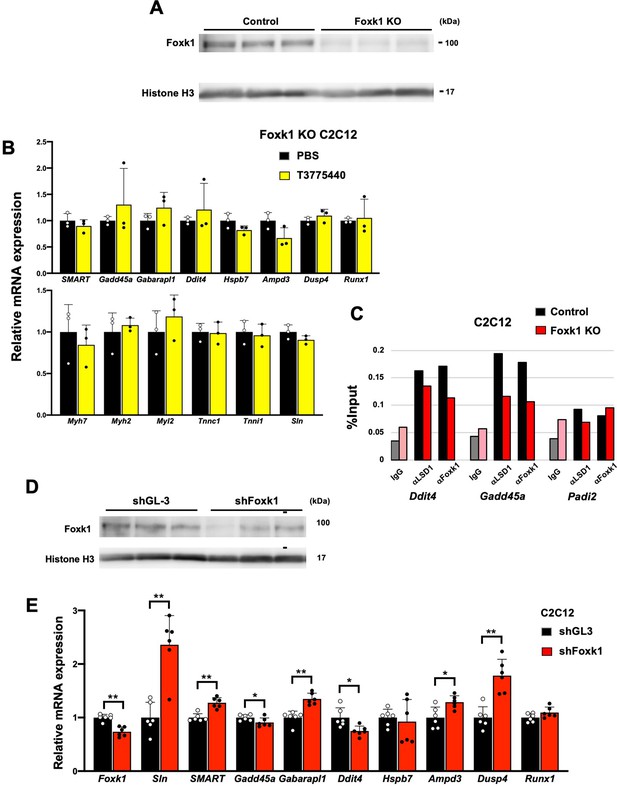
Foxk1 cooperates with lysine-specific demethylase-1 (LSD1) to control the expression of atrophy-associated genes.
(A) Foxk1 protein expression in Foxk1-KO C2C12 cells. C2C12 cells were transfected with control or Foxk1-KO Double Nickase Plasmids and subjected to puromycin selection. (B) The expression of the atrophy-associated genes and fiber type-specific genes in the Foxk1-KO C2C12 myotubes treated with phosphate buffered saline (PBS) (n=3) or 10 nM T3775440 (n=3) for 48 hr. Quantitative reverse transcription-polymerase chain reaction (qRT-PCR) values are shown as the fold differences against those in the PBS-treated Foxk1-KO C2C12 myotubes. Full descriptions of gene symbols are provided in the Supplementary file 3. (C) Chromatin immunoprecipitation (ChIP)-qPCR analyses of LSD1 and Foxk1 at Ddit4, Gadd45a, Padi2 (negative control) gene promoters in control and Foxk1-KO C2C12 myotubes. The data are independent experiments from Figure 3F. The enrichment levels of normal rabbit IgG, LSD1, and Foxk1 were normalized to the input DNA. (D) shRNA-mediated knockdown (KD) of the Foxk1 gene in C2C12 cells. C2C12 cells expressing an shRNA against the firefly luciferase gene (shGL3) were used as control. (E) Expression of atrophy-associated genes in Foxk1-KD C2C12 cells. qRT-PCR values are shown as the fold differences against shGL3 (n=6). Values are mean ± SD. *p<0.05, **p<0.01.
-
Figure 3—figure supplement 2—source data 1
Original blots for Figure 3—figure supplement 2A and D.
- https://cdn.elifesciences.org/articles/84618/elife-84618-fig3-figsupp2-data1-v1.zip
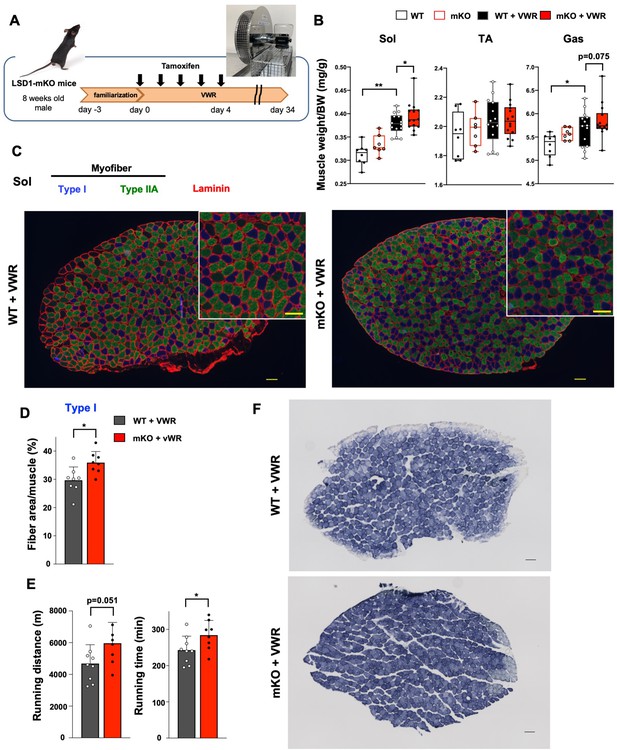
Lysine-specific demethylase-1 (LSD1)-mKO augments the effects of voluntary wheel running (VWR) by enhancing muscle endurance and increasing the number of oxidative fibers.
(A) Experimental design: after familiarization with a cage equipped with a running wheel, LSD1 deletion was induced by tamoxifen injection (days 0–4). Mice were subjected to VWR in the same cage for 38 days (days –3–34). (B) Muscle weight normalized to body weight in sedentary wild type (WT) (n=8) and LSD1-mKO mice (n=7) as well as in VWR-trained WT (n=23) and LSD1-mKO mice (n=22). (C) Immunofluorescence staining of individual fiber types in the whole soleus (Sol) muscles from VWR-trained WT and LSD1-mKO mice. Type I fiber (blue), type IIA fiber (green), and laminin (red) were stained. Magnified images of the tissue sections (scale bars, 100 µm). Representative images (WT and mKO, n=8) are shown. (D) Occupancy of type I fiber based on the cross-sectional area (WT and mKO, n=8). (E) Treadmill performance of WT (n=9) and LSD1-mKO (n=8) mice after VWR. Running distance and time until exhaustion are shown. (F) Succinate dehydrogenase (SDH) staining of the Sol muscles from trained WT and LSD1-mKO mice. Scale bar, 100 μm.
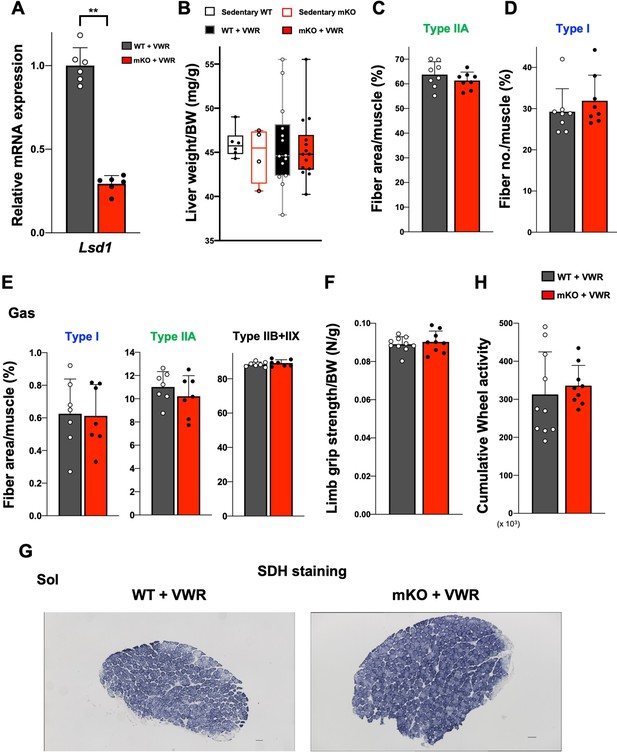
Lysine-specific demethylase-1 (LSD1)-mKO increased the number of oxidative fibers in slow dominant muscle after voluntary wheel running (VWR) training.
(A) LSD1 mRNA expression in soleus (Sol) muscles from wild type (WT) (n=6) and LSD1-mKO (n=6) mice after VWR training. Quantitative reverse transcription-polymerase chain reaction (qRT-PCR) values are normalized to the 36B4 gene and shown as fold differences against WT. (B) Neither the training nor LSD1 genotype affected liver weight. Sedentary WT (n=7) and LSD1-mKO (n=4) mice, and trained WT (n=23) and LSD1-mKO (n=22) mice were analyzed. (C) Occupancy of type IIA fiber based on the cross-sectional area (WT and mKO, n=8). (D) Frequency of type I fiber based on the fiber number (WT and mKO, n=8). (E) Occupancy of each muscle fiber type based on the fiber cross-sectional area in gastrocnemius (Gas) muscles after VWR training (WT, n=7, mKO, n=7). (F) All-limb grip strength in WT (n=10) and LSD1-mKO mice (n=9) after VWR training. (G) Succinate dehydrogenase (SDH) staining of the Sol muscles from trained WT and LSD1-mKO mice. Scale bar, 100 μm. (H) Cumulative running activity of mice in cages with free access to a vertical running wheel for 30 days (days 4–34 in Figure 4A) (WT: n=10, mKO: n=9). Values are mean ± SD. **p<0.01.
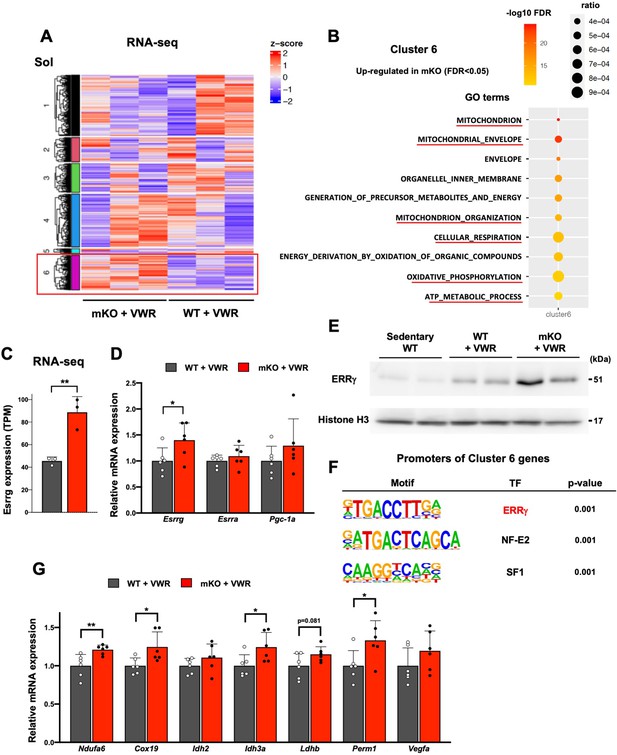
Lysine-specific demethylase-1 (LSD1)-mKO augments the effects of voluntary wheel running (VWR) by increasing the expression of ERRγ and its target genes.
(A) RNA-seq analysis of wild type (WT) and LSD1-mKO soleus (Sol) muscles after voluntary wheel running (VWR) training. K-means clustering identified a group of genes that were upregulated in mKO (cluster 6). (B) RNA-seq analysis of WT and LSD1-mKO Sol muscles after VWR training. The genes upregulated in mKO (cluster 6) were subjected to a gene ontology analysis. (C) The expression of Esrrg in WT (n=3) and LSD1-mKO (n=3) Sol muscles after VWR training from RNA-seq data. (D) The expression of Esrrg and functionally related genes in WT (n=6) and LSD1-mKO (n=6) Sol muscles after VWR training. (E) The expression of ERRγ protein in Sol muscles from sedentary WT, trained WT, and trained LSD1-mKO mice. (F) Motif analyses of promoter regions of the cluster 6 genes. Values are mean ± SD. *p<0.05, **p<0.01. (G) Expression of ERRγ target genes in WT (n=6) and LSD1-mKO (n=6) Sol after VWR training. Quantitative reverse transcription-polymerase chain reaction (qRT-PCR) values are shown as the fold differences against the WT. Full descriptions of gene symbols are provided in the Supplementary file 3. Values are mean ± SD. *p<0.05, **p<0.01.
-
Figure 5—source data 1
Original blots for Figure 5E.
- https://cdn.elifesciences.org/articles/84618/elife-84618-fig5-data1-v1.zip
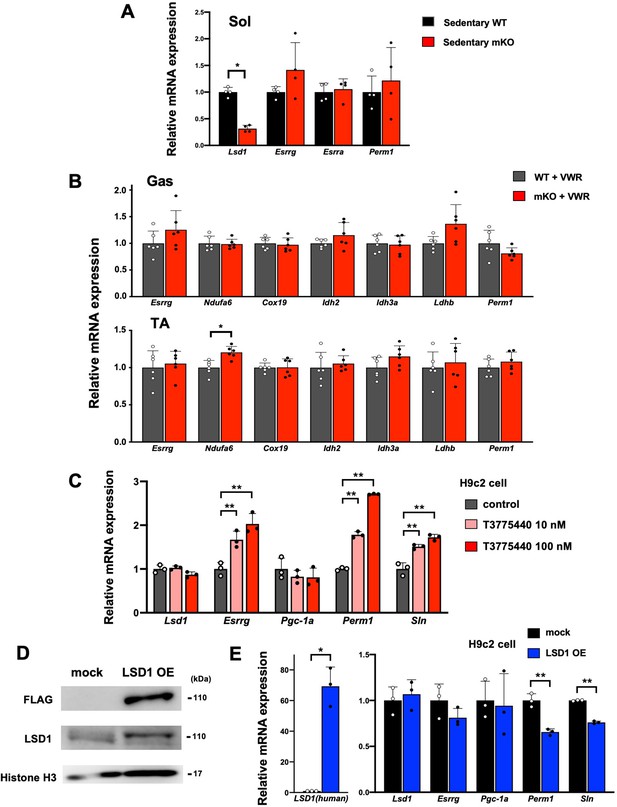
Expression of Esrrg and its target genes remain unaffected in lysine-specific demethylase-1 (LSD1)-mKO gastrocnemius (Gas) and tibialis anterior (TA) muscles after voluntary wheel running (VWR).
(A) The expression of Esrrg and functionally related genes in sedentary wild type (WT) (n=4) and LSD1-mKO (n=4) soleus (Sol) muscles. (B) The expression of Esrrg and its target genes in the Gas and TA muscles after VWR training (WT: n=6, mKO: n=6). Quantitative reverse transcription-polymerase chain reaction (qRT-PCR) values are shown as the fold differences against the WT. (C) The expression of Esrrg and its target genes in the H9c2 cells treated with phosphate buffered saline (PBS) (control) (n=3) or T3775440 (10 and 100 nM, n=3). Cells were treated with the drug during the differentiation. qRT-PCR values are shown as the fold differences against the control. (D) Forced expression of FLAGx3-hLSD1 in H9c2 cells. (E) The expression of Esrrg and its target genes in the mock (n=3) or LSD1-overexpressing (n=3) H9c2 cells. Full descriptions of gene symbols are provided in the Supplementary file 3. Values are mean ± SD. *p<0.05, **p<0.01.
-
Figure 5—figure supplement 1—source data 1
Original blots for Figure 5—figure supplement 1D.
- https://cdn.elifesciences.org/articles/84618/elife-84618-fig5-figsupp1-data1-v1.zip
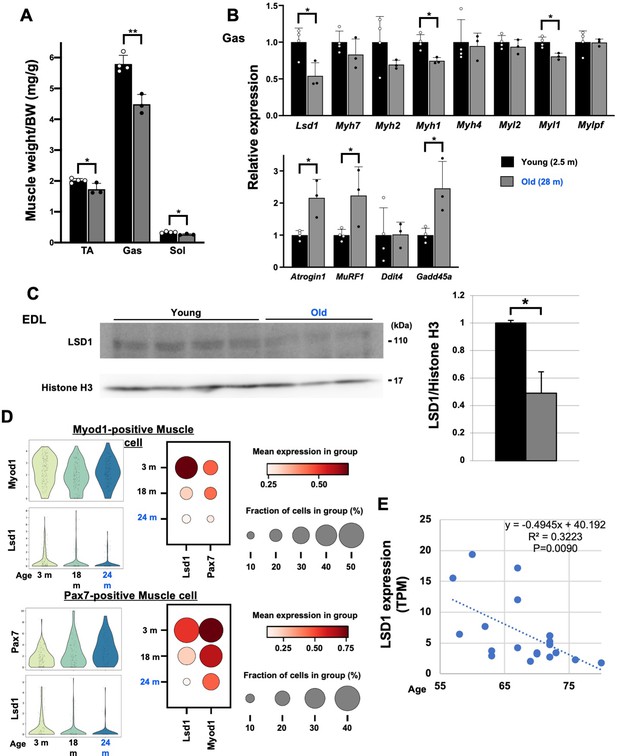
Lysine-specific demethylase-1 (LSD1) expression is decreased in the aged muscles in mice and humans.
(A) Muscle weight in young (2.5 months of age, n=4) and old (28 months of age, n=3) male C57BL/6J mice. (B) The expression of fiber type-specific genes and atrophy-associated genes in the gastrocnemius (Gas) muscles in young and old mice. Quantitative reverse transcription-polymerase chain reaction (qRT-PCR) values are shown as fold differences against the young mice. (C) Expression of LSD1 protein in the EDL muscles from the young and old mice. Band densities were quantified by densitometry and normalized to histone H3. Values are shown as fold differences against the young. (D) Decreased expression of Lsd1 in aged muscles. Using single-cell RNA-seq data (flow cytometry) published by the Tabula Muris Senis (Tabula Muris Consortium, 2020), Lsd1 expression was analyzed in Myod1-positive and Pax7-positive muscle cells from young to old mice (3–24 months of age). (E) The expression of LSD1 in skeletal muscles of 55- to 80-year-old men and women. RNA-seq data by Tumasian et al., 2021, was analyzed. Values are mean ± SD. R2: coefficient of determination. *p<0.05, **p<0.01.
-
Figure 6—source data 1
Original blots for Figure 6C.
- https://cdn.elifesciences.org/articles/84618/elife-84618-fig6-data1-v1.zip
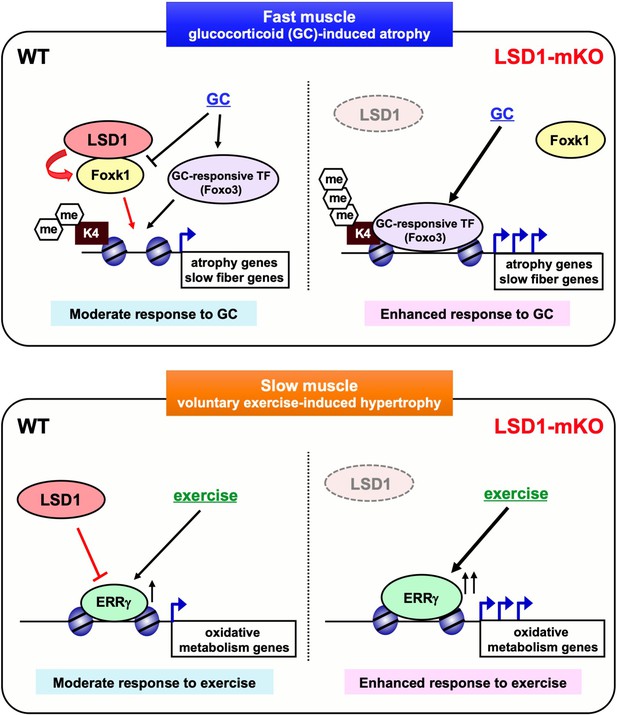
Lysine-specific demethylase-1 (LSD1) serves as an ‘epigenetic barrier’ that defines stress sensitivities in the skeletal muscle.
LSD1 attenuates glucocorticoid (GC)-induced atrophy in the fast fiber-dominant muscles, in collaboration with Foxk1, an anti-autophagic transcription factor. On the other hand, LSD1 attenuates endurance exercise-induced hypertrophy in the slow fiber-dominant muscles, by inhibiting ERRγ, a transcription factor that promotes oxidative metabolism genes. The loss of LSD1 remarkably sensitized the muscles to GC and endurance exercise.
Tables
Reagent type (species) or resource | Designation | Source or reference | Identifiers | Additional information |
---|---|---|---|---|
Strain, strain background (Mus musculus) | Lsd1-floxed mice (B6.129-Kdm1atm1.1Sho/J) | Jackson Laboratories | Strain #:023969 RRID:IMSR_JAX:023969 | |
Strain, strain background (Mus musculus) | Tg(ACTA1-cre/Esr1*)2Kesr/J | Jackson Laboratories | Strain #:025750 RRID:IMSR_JAX:025750 | |
Strain, strain background (Mus musculus) | Lsd1flox/flox; TgACTA1-CreERT2/+ conditional knockout mice (LSD1-mKO mice) | This paper | N/A | See ‘Animal studies’ in Methods |
Strain, strain background (Mus musculus) | C57BL6/J mice | Charles River Laboratories | N/A | |
Cell line (Mus musculus) | C2C12 | RIKEN | RCB0987 | |
Cell line (Ruttus norvegicus) | H9c2 | ATCC | CRL-1446 | |
Antibody | Rabbit polyclonal anti-LSD1 | abcam | ab17721 | WB (1:500), ChIP (5 μg), Co-IP (3 μg) |
Antibody | Rabbit polyclonal anti-Foxk1 | abcam | ab18196 | WB (1:1000), ChIP (5 μg), Co-IP (3 μg) |
Antibody | Rabbit monoclonal anti-ERR gamma | abcam | ab128930 | WB (1:1000) |
Antibody | Mouse monoclonal anti-MHC type I | DHSB | BA-F8 | IHC (1:50) |
Antibody | Mouse monoclonal anti-MHC type IIA | DHSB | SC-71 | IHC (1:50) |
Antibody | Rabbit polyclonal anti-Laminin | Sigma-Aldrich | L9393 | IHC (1:500) |
Antibody | Rabbit polyclonal anti-Akt | Cell Signaling Technology | #9272 | WB (1:1000) |
Antibody | Rabbit polyclonal anti-Phospho-Akt | Cell Signaling Technology | #9271 | WB (1:1000) |
Antibody | Rabbit polyclonal anti-LC3 | MBL | PM036 | WB (1:1000) |
Antibody | Mouse monoclonal anti- Phospho-4E-BP1 (Thr37/46) | Cell Signaling Technology | #2855 | WB (1:1000) |
Antibody | Rabbit polyclonal anti-4E-BP1 | Cell Signaling Technology | 9454 | WB (1:1000) |
Antibody | Mouse monoclonal anti- SQSTM1(p62) | Santa Cruz | sc-28359 | WB (1:1000) |
Antibody | Rabbit polyclonal anti-GAPDH | Santa Cruz | sc-25778 | WB (1:6000) |
Antibody | Rabbit polyclonal anti-trimethyl-Histone H3 (Lys4) | Millipore | 07-473 | ChIP (3 μg) |
Antibody | Rabbit polyclonal anti-Histone H3 | abcam | ab1791 | WB (1:5000) |
Recombinant DNA reagent | Control Double Nickase Plasmid | Santa Cruz Biotechnology | sc-437281 | |
Recombinant DNA reagent | FOXK1 Double Nickase Plasmid | Santa Cruz Biotechnology | sc-437282-NIC | |
Recombinant DNA reagent | pENTR4-H1 | RIKEN BRC DNA BANK | RDB04395 | |
Recombinant DNA reagent | pCAG-HIVgp | RIKEN BRC DNA BANK | RDB04394 | |
Recombinant DNA reagent | pCMV-VSV-G-RSV-Rev | RIKEN BRC DNA BANK | RDB04393 | |
Recombinant DNA reagent | CS-RfA-EVBsd | RIKEN BRC DNA BANK | RDB06090 | |
Recombinant DNA reagent | CS-RfA-EVBsd shFoxk1 | This paper | N/A | See ‘Construction of lentiviral expression vectors and their transduction’ in Methods |
Recombinant DNA reagent | CS-RfA-EVBsd shGL3 | This paper | N/A | See ‘Construction of lentiviral expression vectors and their transduction’ in Methods |
Recombinant DNA reagent | CSII-EF-3xFLAG-hLSD1-Bsd | This paper | N/A | A lentiviral construct used for the forced expression of human LSD1 |
Sequence-based reagent | Primers for qRT-PCR | This paper | N/A | See Supplementary file 3 |
Sequence-based reagent | See Table S2 for primers for ChIP-qPCR | This paper | N/A | See Supplementary file 4 |
Commercial assay or kit | NEBNext Ultra DNA Library Prep Kit | New England Biolabs | E7370S | |
Commercial assay or kit | NEBNext Poly(A) mRNA Magnetic Isolation Module | New England Biolabs | E7490S | |
Chemical compound, drug | Tamoxifen | Sigma-Aldrich | T5648 | |
Chemical compound, drug | Dexamethasone | Sigma-Aldrich | D4902 | |
Chemical compound, drug | Insulin | nacalai tesque | 19251-24 | |
Chemical compound, drug | Streptozotocin | Wako | 197-15153 | |
Chemical compound, drug | T-3775440 hydrochloride | MedChemExpress | HY-103085 | |
Chemical compound, drug | FuGENE 6 Transfection Reagent | Promega | E2691 | |
Chemical compound, drug | Puromycin dihydrochloride | Sigma-Aldrich | P8833 | |
Chemical compound, drug | Blasticidin S | FUJIFILM Wako Chemicals | 029-18701 | |
Chemical compound, drug | Can Get Signal immunostain Solution A | TOYOBO | NKB-501 | |
Chemical compound, drug | malinol | Muto Pure Chemicals | 2009-1 | |
Chemical compound, drug | Can Get Signal Solution 1 | TOYOBO | NKB-201 | |
Chemical compound, drug | Can Get Signal Solution 2 | TOYOBO | NKB-301 | |
Chemical compound, drug | TRIzol RNA Isolation Reagent | Thermo Fisher Scientific | 15596026 | |
Chemical compound, drug | ReverTra Ace qPCR RT Master Mix | TOYOBO | FSQ-201 | |
Chemical compound, drug | THUNDERBIRD SYBR qPCR Mix | TOYOBO | QPS-201 | |
Software, algorithm | BZ-X Analyzer software | KEYENCE | N/A | |
Software, algorithm | Homer v4.9.1 | Heinz et al., 2010 | http://homer.ucsd.edu/homer/ | |
Software, algorithm | STAR | Dobin et al., 2013 | https://github.com/alexdobin/STAR; Dobin, 2023 | |
Software, algorithm | Edge R | Robinson et al., 2010 | http://bioconductor.org/packages/release/bioc/html/edgeR.html | |
Software, algorithm | Heatmapper | Babicki et al., 2016 | http://www.heatmapper.ca/expression/ | |
Software, algorithm | DEseq2 | Love et al., 2014 | https://bioconductor.org/packages/release/bioc/html/DESeq2.html | |
Software, algorithm | ComplexHeatmap | Gu et al., 2016 | https://github.com/jokergoo/ComplexHeatmap; Gu, 2022 | |
Software, algorithm | Molecular Signature Database v7.4 | Subramanian et al., 2005 | https://www.gsea-msigdb.org/gsea/msigdb/ | |
Software, algorithm | HOMER V4.9.1 | Heinz et al., 2010 | http://homer.ucsd.edu/homer/ | |
Software, algorithm | JMP (version 10.0.2d1) | SAS Institute Inc | N/A | |
Software, algorithm | GraphPad Prism version 8.4.3 | GraphPad Software | N/A | |
Software, algorithm | Integrative Genomics Viewer (IGV) | James T et al., 2011 | https://software.broadinstitute.org/software/igv/ |
Additional files
-
Supplementary file 1
Top 50 differentially regulated genes in dexamethasone (Dex)-treated lysine-specific demethylase-1 (LSD1)-mKO mice.
- https://cdn.elifesciences.org/articles/84618/elife-84618-supp1-v1.xlsx
-
Supplementary file 2
Cluster 6 genes in Figure 5A.
- https://cdn.elifesciences.org/articles/84618/elife-84618-supp2-v1.xlsx
-
Supplementary file 3
Primers used in this study.
- https://cdn.elifesciences.org/articles/84618/elife-84618-supp3-v1.xlsx
-
Supplementary file 4
Chromatin immunoprecipitation (ChIP) primers used in this study.
- https://cdn.elifesciences.org/articles/84618/elife-84618-supp4-v1.xlsx
-
Supplementary file 5
Full uncropped blots.
- https://cdn.elifesciences.org/articles/84618/elife-84618-supp5-v1.pdf
-
MDAR checklist
- https://cdn.elifesciences.org/articles/84618/elife-84618-mdarchecklist1-v1.pdf