Diverse evolutionary pathways challenge the use of collateral sensitivity as a strategy to suppress resistance
Figures
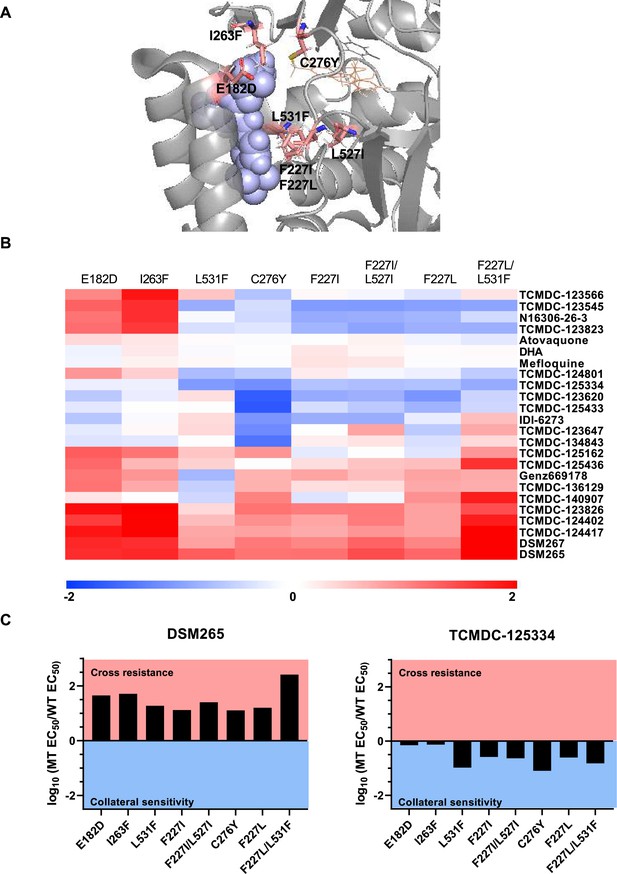
Identifying DHODH inhibitors with broad activity against mutant lines.
We tested several DSM265-resistant parasite lines against a set of 17 compounds identified from a previous screen of GSK libraries (Ross et al., 2018). (A) Crystal structure of DHODH bound to DSM265 (PDB ID: 4RX0). Mutations included in our cross-resistance analysis are highlighted in pink. (B) To visualize patterns of cross-resistance and collateral sensitivity, we calculated the fold change of each mutant line over its wildtype parent, and plotted the log10-transformed values in a heatmap. Hierarchical clustering by Euclidean distance based on both parasite line and compound was performed using MultiExperimentViewer v4.9. Shades of red indicate that a parasite line is resistant to the indicated compound while blue indicates that it is sensitive. The DHODH C276Y, F227L, and F227L/L531F are newly reported, while other mutant lines were reported in our previous cross-resistance analysis (Ross et al., 2018). The wildtype parent for the DHODH F227I, L531F, F227I/L527I, and I263F lines is Dd2. The wildtype parent for the DHODH E182D line is 3D7. The wildtype parent for the DHODH C276Y, F227L, and F227L/L531F lines is 3D7 A10. (C) One compound, TCMDC-125334 stands out in this analysis as being active against all mutant lines tested. Shown is a bar graph of the log10 fold change in EC50 relative to wildtype for all eight mutant lines tested against DSM265 and TCMDC-125334. DSM265 data was previously reported (Mandt et al., 2019). Each dose-response assay was performed with triplicate technical replicates, and average EC50’s were obtained from 4 to 5 independent biological replicates. See also Figure 1—source data 1.
-
Figure 1—source data 1
Individual bioreplicates of EC50 values (nM) obtained from dose-response assays.
- https://cdn.elifesciences.org/articles/85023/elife-85023-fig1-data1-v2.xlsx
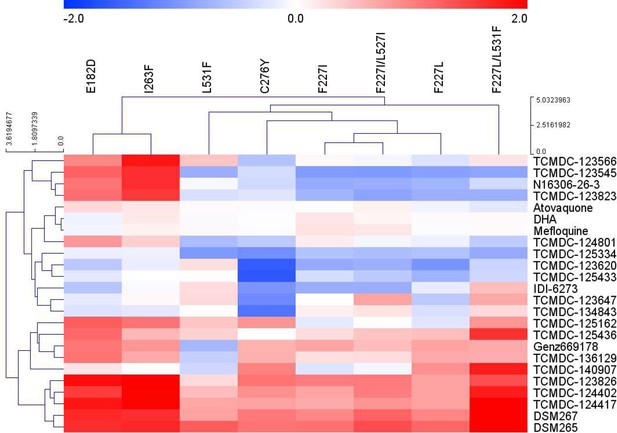
Hierarchical clustering tree of mutant lines and compounds.
Clustering was done using Euclidean distance and average linkage clustering. Data was analyzed and image produced by MultiExperimentViewer (MeV) v. 4.9.0.
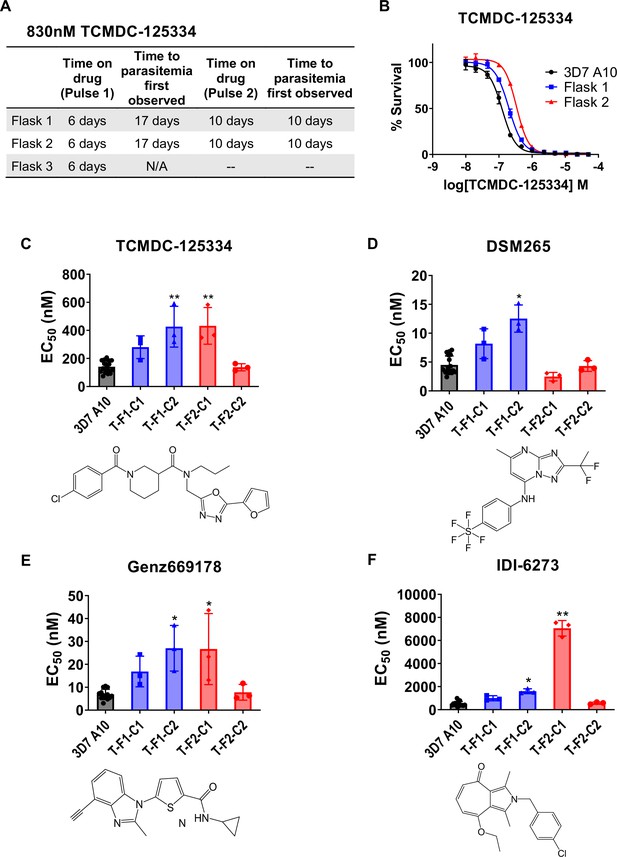
In vitro resistance to TCMDC125334 can be mediated by copy number variation as well as the novel point mutation DHODH I263S.
(A) Protocol for in vitro selection with TCMDC-125334. Parasite populations in three independent 25 mL culture flasks were exposed to 830 nM TCMDC-125334, then allowed to recover in the absence of compound. Resistant parasites emerged after two rounds of treatment within the indicated timeframe. (B) In vitro dose-response curve of bulk populations recovered after the second pulse of compound treatment. Error bars show standard deviation of three technical replicates. (C–E) Resistant populations were cloned by limiting dilutions. Shown are the average EC50 and standard deviation for three biological replicates of dose-response phenotype of two representative clones from each flask for TCMDC-125334 (C), DSM265 (D), Genz669178 (E), and IDI-6273 (F). Each dose-response assay was performed with triplicate technical replicates, and average EC50’s were obtained from 3 to 4 independent biological replicates. Compound structures for TCMDC-125334, DSM265, Genz669178, and IDI-6273 are displayed below their corresponding graphs. The parasite lines are each designated with a unique identifier; for example, T-F1-C1 was selected with TCDC-125334 (T) came from flask 1 (F1), and is designated as ‘C1’ for ‘Clone 1’. DHODH genotypes for representative clones are as follows: T-F1-C1: 2x dhodh copy number variation (CNV); T-F1-C2: 3x dhodh CNV; T-F2-C1: DHODH I263F; T-F2-C1: WT. Statistical significance was determined by a Kruskal-Wallis test, with post hoc multiple comparisons (Dunn’s) of each clone to 3D7 A10. *p≤0.05; **p≤0.01. See also Table 1, Supplementary file 1b, and Table 1—source data 1.
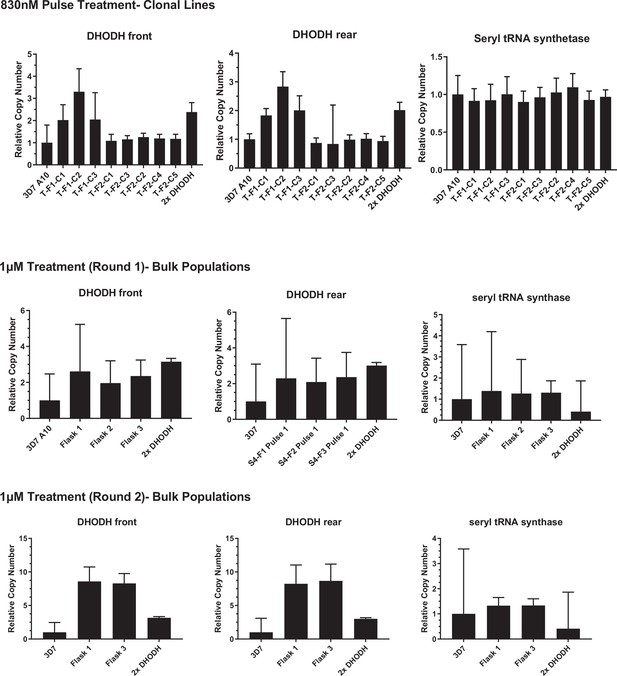
Copy number variation of the dhodh locus in 3D7 A10 parasites selected with TCMDC-125334.
Copy number was detected by quantitative PCR and calculated using the ΔΔCT method, normalizing to the 3D7 A10 parent and the 18s rRNA target. DHODH front and DHODH rear refer to two amplicons within the dhodh gene. Seryl tRNA synthase is shown as a control. 2x DHODH is control gDNA isolated from parasites with previously confirmed copy number duplication. Error bars represent minimum and maximum values of four technical replicates.
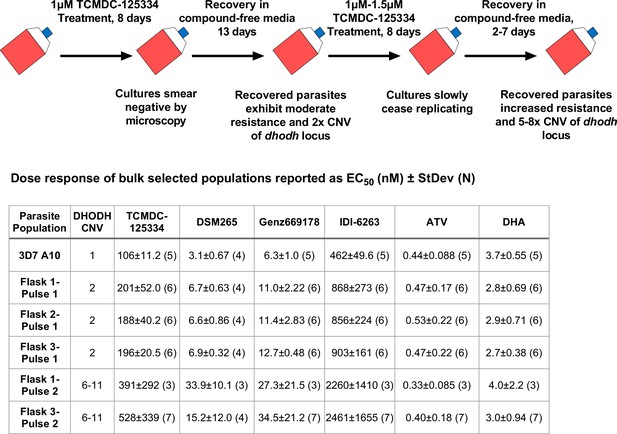
Treatment with 1 µM TCMDC-125334 selects for moderately resistant parasite populations with copy number variation at the dhodh locus.
Schematic of selection protocol. Genetic characterization revealed that parasite populations exhibited 2x copy number variation of the dhodh locus after the first round of treatment with TCMDC-125334, and five- to eight-fold copy number variation of the dhodh locus after the second round of treatment (see Figure 2). Table displays dose response of parasite populations selected with TCMDC-125334. Shown are average EC50’s with standard deviation and sample number (N) defined as number of individual bioreplicates. See also Figure 2—figure supplement 2—source data 1.
-
Figure 2—figure supplement 2—source data 1
Whole-genome sequencing analysis of bulk selected populations.
Status column indicates whether the mutation is present at a clonal allele frequency or mixed allele frequency since the samples were bulk populations.
- https://cdn.elifesciences.org/articles/85023/elife-85023-fig2-figsupp2-data1-v2.xlsx
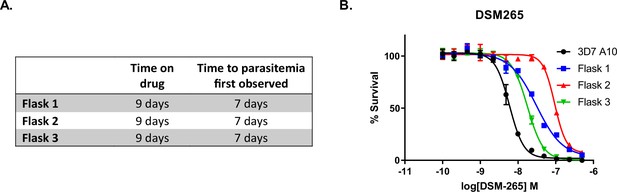
Control selection with DSM265.
(A) Table describing selection procedure. Three 25 mL flasks of approximately 108 parasites were treated with 30 nM of DSM265 for 9 days. In all three flasks, parasitemia was observed 7 days post drug treatment. (B) Dose-response assay of in vitro selected bulk populations. Error bars show standard deviation of three technical replicates. See also Figure 2—figure supplement 3—source data 1.
-
Figure 2—figure supplement 3—source data 1
Whole-genome sequencing analysis of bulk selected populations.
Status column indicates whether the mutation is present at a clonal allele frequency or mixed allele frequency since the samples were bulk populations.
- https://cdn.elifesciences.org/articles/85023/elife-85023-fig2-figsupp3-data1-v2.xlsx
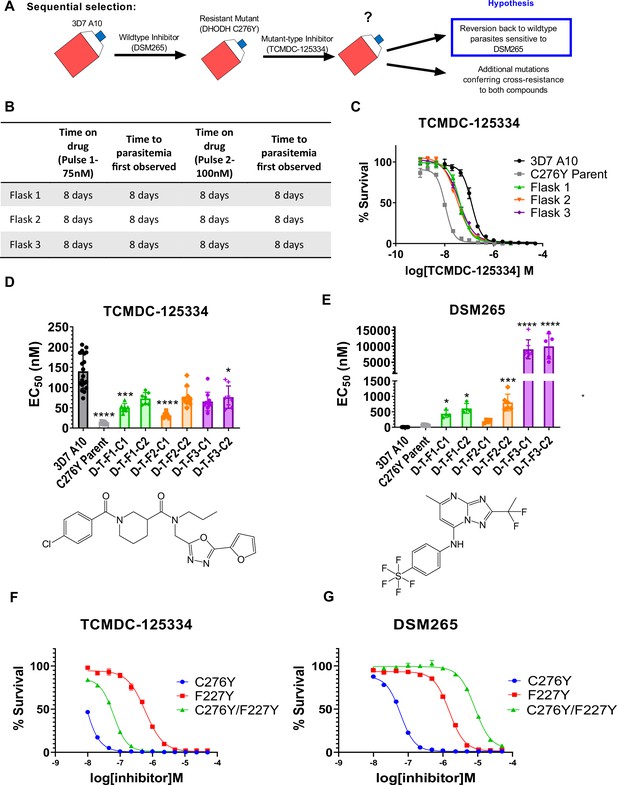
Mutant parasites can overcome collateral sensitivity by acquiring additional genetic changes that confer high-level resistance to wildtype inhibitors.
(A) General schematic for sequential selection. (B) Protocol for in vitro selection. DHODH C276Y mutant parasites in three independent 25 mL culture flasks were exposed to 40 nM TCMDC-125334, then allowed to recover. The time on compound and time to recovery are indicated. After two rounds of treatment and recovery, resistant parasites are observed in all three flasks. (C) In vitro dose-response curve of selected bulk populations. Error bars show standard deviation of technical replicates. (D–E) Resistant populations were cloned by limiting dilution. Shown is the average EC50 of two representative clones from each flask for TCMDC-125334 (C), and DSM265 (D), with chemical structures illustrated below. Bar graphs represent average EC50 with error bars depicting standard deviation. Individual biological replicates are also shown. Each dose-response assay was performed with triplicate technical replicates, and average EC50’s were obtained from 3 to 8 independent biological replicates. The parasite lines are each designated with a unique identifier; for example, D-T-F1-C1 was selected first with DSM265 (D), then from TCMDC-125334 (T), came from flask 1 (F1), and is designated as ‘C1’ for ‘Clone 1’. DHODH genotypes for representative clones are as follows: D-T-F1-C1: DHODH C276Y+3x dhodh copy number variation (CNV); D-T-F1-C2: DHODH C276Y+4x dhodh CNV; D-T-F2-C1: DHODH C276Y+2x dhodh CNV; D-T-F2-C2: DHODH C276Y+4x dhodh CNV; D-T-F3-C1: DHODH C276Y/F227Y; D-T-F3-C2: DHODH C276Y/F227Y. Statistical significance was determined by a Kruskal-Wallis test, with post hoc multiple comparisons (Dunn’s) of each clone to 3D7 A10. In cases where the EC50 could not be determined with range of concentrations tested, the maximum concentration that achieved >50% growth was used as a stand-in value. *p≤0.05; ***p≤0.001; ****p≤0.0001. See also Table 2, Supplementary file 1d, and Table 2—source data 1. (F–I) The DHODH C276Y/F227Y double mutant exhibits a resistant phenotype intermediate to single mutations. The dose-response phenotypes of the DHODH C276Y/F227Y line selected in this study (clone C276Y T-F3-C3), the DHODH C276Y line previously selected with DSM265, and the DHODH F227Y line previously selected with DSM267 (Mandt et al., 2019) were characterized. Shown is a representative dose-response curve for DSM265 (F) and TCMDC-125334 (G) for illustration. Error bars show standard deviation of technical replicates within a single assay.
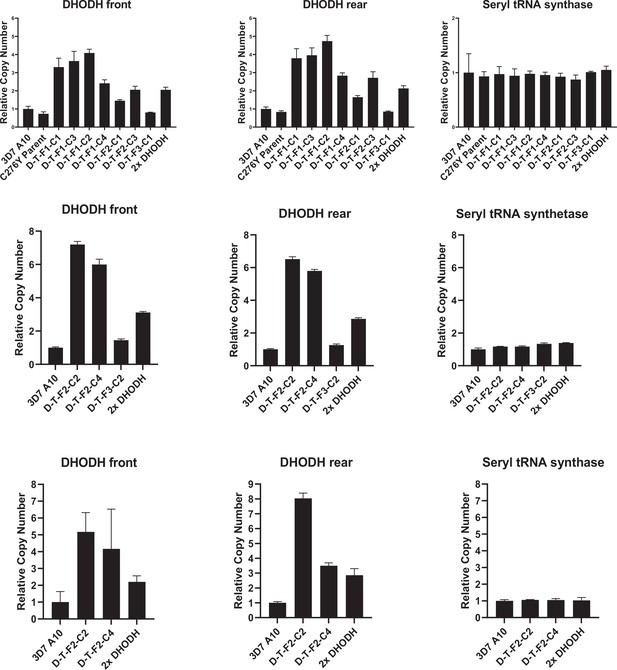
Copy number variation of the dhodh locus in DHODH C276Y parasites selected with TCMDC-125334.
Copy number was detected by quantitative PCR and calculated using the ΔΔCT method, normalizing to the 3D7 A10 parent and the 18s rRNA target. DHODH front and DHODH rear refer to two amplicons within the dhodh gene. Seryl tRNA synthase is shown as a control. 2x DHODH is control gDNA isolated from parasites with previously confirmed copy number duplication. Error bars represent minimum and maximum values of four technical replicates.
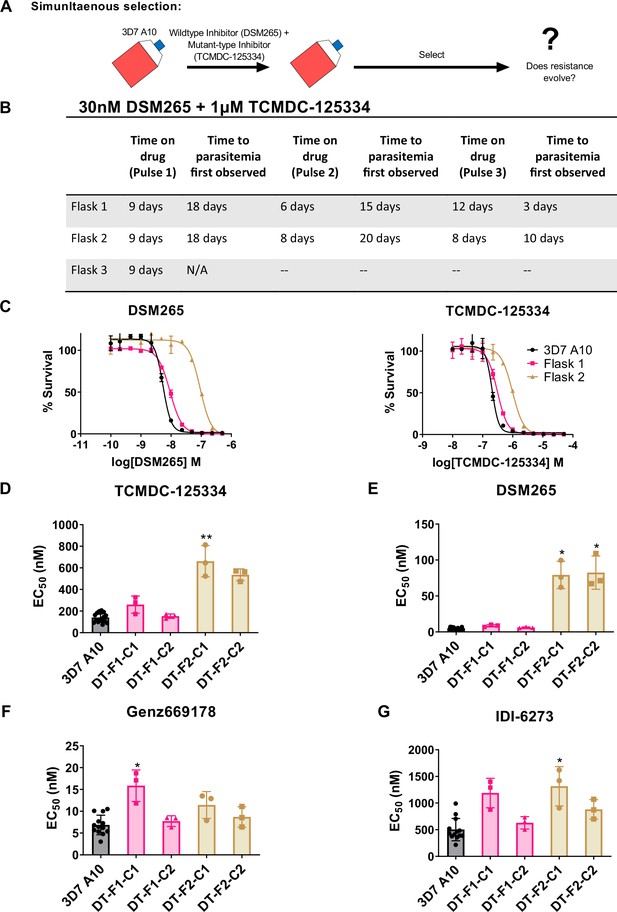
Resistance to the DSM265 + TCMDC-125334 combination arose after three rounds of treatment, with cross-resistance to both compounds conferred by the novel DHODH V532A mutation.
(A) General schematic for simultaneous selection. (B) Protocol for in vitro selection with TCMDC-125334 and DSM265. Parasite populations in three independent 25 mL culture flasks were exposed to combination treatment, then allowed to recover. The time on compound and time to recovery are indicated. After three rounds of treatment and recovery, resistant parasites are observed in flasks 1 and 2. (C) In vitro dose-response curve of bulk populations from flasks 1 and 2. Error bars show standard deviation of technical replicates. (D–G) Resistant populations were cloned by limiting dilutions. Shown is the average EC50 of two representative clones from each flask for TCMDC-125334 (D), DSM265 (E), Genz669178 (F), and IDI6273 (G). Bar graphs represent average EC50 with error bars depicting standard deviation. Individual biological replicates are also shown. Each dose-response assay was performed with triplicate technical replicates, and average EC50’s were obtained from 3 independent biological replicates. The parasite lines are each designated with a unique identifier; for example, DT-F1-C1 was selected with DSM255 and TCDC-125334 (DT) came from flask 1 (F1), and is designated as ‘C1’ for ‘Clone 1’. Statistical significance was determined by a Kruskal-Wallis test, with post hoc multiple comparisons (Dunn’s) of each clone to 3D7 A10. *p≤0.05; **p≤0.01. See also Table 3, Supplementary file 1e, and Table 3—source data 1.
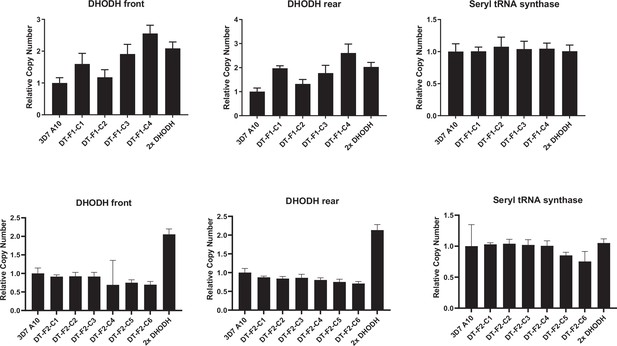
Copy number variation of the dhodh locus in 3D7 A10 parasites selected with DSM265 + TCMDC-125334 simultaneously.
Copy number was detected by quantitative PCR and calculated using the ΔΔCT method, normalizing to the 3D7 A10 parent and the 18s rRNA target. DHODH front and DHODH rear refer to two amplicons within the dhodh gene. Seryl tRNA synthase is shown as a control. 2x DHODH is control gDNA isolated from parasites with confirmed copy number duplication. Error bars represent minimum and maximum values of four technical replicates.
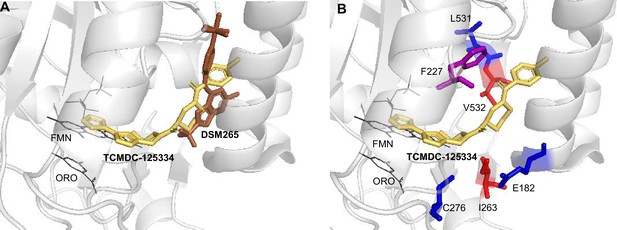
Molecular docking of TCMDC-125334 with PfDHODH.
(A) TCMDC-125334 docks within the inhibitor binding pocket of DHODH. Shown is overlay of TCMDC-125334 docked in combined structure, and DSM265 from the 4XR0 structure. (B) Mutations conferring cross-resistance or collateral sensitivity are in close proximity to TCMDC-125334. Key residues discussed in the study are highlighted. Residues conferring cross-resistance are shown in red, while residues conferring collateral sensitivity are in blue. The F227 residue is colored purple, as the F227L and F227I substitutions confer collateral sensitivity while the F227Y substitution confers resistance. Structure labeled ORO is the orotic acid product. FMN is the flavin mononucleotide cofactor.
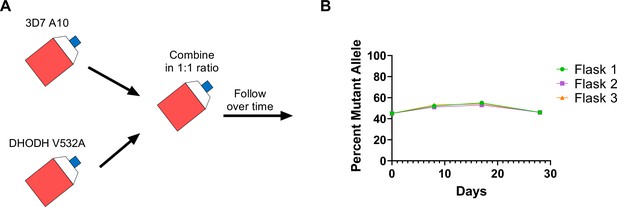
The DHODH V532A mutation does not confer a fitness cost in in vitro competitive growth assays.
(A) Schematic of competitive growth experiments. Synchronized 3D7 parent and DHODH V532A mutant parasites at 1% ring-stage parasitemia were mixed at equal ratios. The mixed culture was then split into three independent flasks, and followed over time. Mixed cultures were grown for 4 weeks, and genomic DNA was collected every 7–9 days. (B) The percent mutant allele was calculated at each timepoint based on whole-genome sequencing reads (Supplementary file 1f).
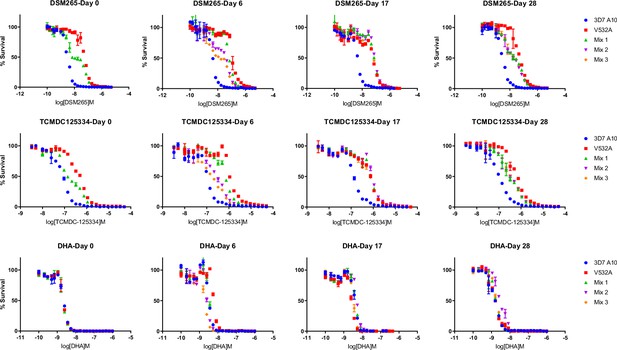
Co-cultured parasites in competition assay retain an intermediate phenotype.
3D7 parent and DHODH V532A mutant parasites at 1% ring-stage parasitemia were mixed at equal ratio and separated into three independent flasks. Mixed cultures were grown for 4 weeks. Dose-response assays were conducted at day 0, day 17, and day 28. Upper and middle panels show the dose response for DSM265 and TCMDC-125334, respectively, which differ between the 3D7 A10 wildtype and DHODH V532A strains. The bottom panel shows the dose response for dihydroartemisinin (DHA) as a control. Error bars show standard deviation of three technical replicates.
Tables
DHODH genotype and corresponding dose-response phenotype for representative in vitro TCMDC-125334 selected lines.
Clone ID | DHODH mutation(s)* | DHODH CNV | TCMDC-125334 EC50 (nM) | DSM265EC50 (nM) | IDI-6273 EC50 (nM) | Genz669178 EC50 (nM) |
---|---|---|---|---|---|---|
3D7 A10 | WT | 1 | 140±42.6 | 4.5±1.6 | 502±209 | 6.9±2.2 |
T-F1-C1 | WT | 2 | 280±81.3 | 8.2±2.6 | 993±220 | 16.9±6.70 |
T-F1-C2 | WT | 3 | 426±146** | 12.5±2.36* | 1597±212* | 27.0±10.0* |
T-F2-C1 | I263S | 1 | 432±130.8** | 2.5±0.74 | 7057±671** | 26.7±15.5* |
T-F2-C2 | WT | 1 | 137±25.1 | 4.3±0.94 | 572±91.5 | 7.79±3.42 |
Overall p-value (approximate) | 0.0004 | 0.0013 | 0.0007 | 0.0013 | ||
Kruskall-Wallis statistic | 28.52 | 25.54 | 27.05 | 25.50 |
-
The parasite lines are each designated with a unique identifier; for example, T-F1-C1 was selected with TCDC-125334 (T) came from flask 1 (F1), and is designated as ‘C1’ for ‘Clone 1’. Data is shown as mean EC50 ± standard deviation. Statistical significance was determined by a Kruskal-Wallis test, with post hoc multiple comparisons (Dunn’s) of each clone to 3D7 A10. *p≤0.05; **p≤0.01. Overall statistics are reported for each comparison group. Each dose-response assay was performed with triplicate technical replicates, and average EC50’s were obtained from 3 to 4 independent biological replicates.
-
*
Variants identified by whole-genome sequencing.
-
Table 1—source data 1
Individual bioreplicates of EC50 values (nM) obtained from dose-response assays.
- https://cdn.elifesciences.org/articles/85023/elife-85023-table1-data1-v2.xlsx
-
Table 1—source data 2
Copy number variation (CNV) analysis based on whole-genome sequencing.
Denoised log2 copy ratios were calculated across the genic intervals of the full genome for all sequenced samples. Values were calculated using the GATK4.0 CNV detection pipeline.
- https://cdn.elifesciences.org/articles/85023/elife-85023-table1-data2-v2.xlsx
-
Table 1—source data 3
Homozygous variants identified from in vitro selections by whole-genome sequencing.
Each entry represents a single clonal mutation that is present in the drug-selected clone but not in the matched parent line (i.e. arose during the drug selection process).
- https://cdn.elifesciences.org/articles/85023/elife-85023-table1-data3-v2.xlsx
DHODH genotype and corresponding dose-response phenotype for representative in vitro selection of DHODH C276Y parent with TCMDC-125334.
Clone ID | DHODH mutation(s)* | DHODH CNV | TCMDC-125334 EC50 (nM) | DSM265EC50 (nM) | IDI-6273 EC50 (nM) | Genz669178 EC50 (nM) |
---|---|---|---|---|---|---|
3D7 A10 | WT | 1 | 140±42.6 | 4.5±1.6 | 502±209 | 6.9±2.2 |
C276Y Parent | C276Y | 1 | 12.40±3.089**** | 63.9±19.84 | 41.7±12.7**** | 22.0±6.68 |
D-T-F1-C1 | C276Y | 3–4 | 50.3±10.4*** | 439.3±115.96* | 185±36.1 | >106 |
D-T-F1-C2 | C276Y | 4 | 73.0±14.7 | 611±159* | 345±64.1 | >106 |
D-T-F2-C1 | C276Y | 2 | 31.1±6.89**** | 196±56.6 | 138±44.0* | 117±30.0 |
D-T-F2-C2 | C276Y | 4 | 77.2±25.1 | 801±270 | 326±155 | ND |
D-T-F3-C1 | C276Y/F227Y | 1 | 65.6±22.9* | 9042±2985**** | 163±36.3** | 69.7±14.5 |
D-T-F3-C2 | C276Y/F227Y | 1 | 76.3±27.7 | 9960±3923**** | 183±84.3 | 97±54.5 |
Overall p-value (approximate) | <0.0001 | <0.0001 | <0.0001 | <0.0001 | ||
Kruskall-Wallis statistic | 38.12 | 47.36 | 45.55 | 54.32 |
-
The parasite lines are each designated with a unique identifier; for example, D-T-F1-C1 was selected first with DSM265 (D), then from TCMDC-125334 (T), came from flask 1 (F1), and is designated as ‘C1’ for ‘Clone 1’. Data is shown as mean EC50 ± standard deviation. Statistical significance was determined by a Kruskal-Wallis test, with post hoc multiple comparisons (Dunn’s) of each clone to 3D7 A10. *p≤0.05; **p≤0.01; ***p≤0.001; ****p≤0.0001. Overall statistics are reported for each comparison group. Each dose-response assay was performed with triplicate technical replicates, and average EC50’s were obtained from 3 to 8 independent biological replicates. WT = wildtype. ND indicates that the EC50 could not be determined; parasites were resistant to the range of doses tested as indicated by lack of complete kill at the highest dose of 500 nM. A representative set of clones were tested at higher concentrations.
-
*
Variants identified by whole-genome sequencing.
-
Table 2—source data 1
Individual bioreplicates of EC50 values (nM) obtained from dose-response assays.
Data is organized in tabs by figure/table of main manuscript.
- https://cdn.elifesciences.org/articles/85023/elife-85023-table2-data1-v2.xlsx
-
Table 2—source data 2
Copy number variation (CNV) analysis based on whole-genome sequencing.
Denoised log2 copy ratios were calculated across the genic intervals of the full genome for all sequenced samples. Values were calculated using the GATK4.0 CNV detection pipeline.
- https://cdn.elifesciences.org/articles/85023/elife-85023-table2-data2-v2.xlsx
-
Table 2—source data 3
Homozygous variants identified from in vitro selections by whole-genome sequencing.
Each entry represents a single clonal mutation that is present in the drug-selected clone but not in the matched parent line (i.e. arose during the drug selection process).
- https://cdn.elifesciences.org/articles/85023/elife-85023-table2-data3-v2.xlsx
Resistance phenotype of C276Y and F227Y single mutants and C276Y/F227Y double mutant compared to expected phenotype under additive epistasis.
DHODH mutations | TCMDC-125334 EC50 (fold change) | DSM265 EC50 (fold change) | IDI-6273 EC50 (fold change) | Genz669178EC50 (fold change) |
---|---|---|---|---|
3D7 A10 | 140±42.6 | 4.5±1.6 | 502±209 | 6.9±2.2 |
C276Y | 12.40±3.09 (0.089) | 63.9±19.8 (14.2) | 41.7±12.7 (0.083) | 22.0±6.68 (3.2) |
F227Y | 408±17.6 (2.9) | 998±50.0 (221) | 2210±289 (4.4) | 18.8±3.39 (2.7) |
C276Y/F227Y (D-T-F3-C1) | 65.6±22.9 (0.46) | 9042±2985 (2009) | 163±36.3 (0.32) | 69.7±14.5 (10.1) |
Expected fold change for C276Y/F227Y (additive assumption) | 0.26 | 3138 | 0.37 | 8.64 |
-
EC50 (nM) of each line is reported, with fold change relative to 3D7 A10 in parentheses. The expected fold change of the C276Y double mutant based on an assumption of additive epistasis is reported for comparison.
-
Table 3—source data 1
Individual bioreplicates of EC50 values (nM) obtained from dose-response assays.
- https://cdn.elifesciences.org/articles/85023/elife-85023-table3-data1-v2.xlsx
DHODH genotype and corresponding dose-response phenotype for representative in vitro TCMDC-125334 + DSM265 selected lines.
Clone ID | DHODH mutation(s) | DHODH CNV | TCMDC-125334 EC50 (nM) | DSM265EC50 (nM) | IDI-6273 EC50 (nM) | Genz669178 EC50 (nM) |
---|---|---|---|---|---|---|
3D7 A10 | WT | 1 | 140±42.6 | 4.5±1.6 | 502±209 | 6.9±2.2 |
DT-F1-C1 | WT* | 2 | 260±79.5 | 8.6±1.6 | 1190±276 | 15.9±3.61* |
DT-F1-C2 | WT† | 1 | 153±21.0 | 6.1±0.88 | 628±117 | 7.74±1.23 |
DT-F2-C1 | V532A* | 1 | 661±145** | 79.2±19.0* | 1320±368* | 11.4±3.08 |
DT-F2-C2 | V532A* | 1 | 536±54.4 | 82.4±23.1* | 881±185 | 8.69±2.35 |
Overall p-value (approximate) | <0.0001 | <0.0001 | 0.0019 | 0.0091 | ||
Kruskall-Wallis statistic | 35.72 | 35.80 | 27.85 | 23.50 |
-
The parasite lines are each designated with a unique identifier; for example, DT-F1-C1 was selected with DSM255 and TCDC-125334 (DT) came from flask 1 (F1), and is designated as ‘C1’ for ‘Clone 1’. Data is shown as mean EC50 ± standard deviation. Statistical significance was determined by a Kruskal-Wallis test, with post hoc multiple comparisons (Dunn’s) of each clone to 3D7 A10. *p≤0.05; **p≤0.01. Overall statistics are reported for each comparison group. Each dose-response assay was performed with triplicate technical replicates, and average EC50’s were obtained from 3 independent biological replicates. WT = wildtype.
-
*
DHODH genotype determined by whole-genome sequencing.
-
†
DHODH genotype determined by Sanger sequencing.
-
Table 4—source data 1
Individual bioreplicates of EC50 values (nM) obtained from dose-response assays.
- https://cdn.elifesciences.org/articles/85023/elife-85023-table4-data1-v2.xlsx
-
Table 4—source data 2
Copy number variation (CNV) analysis based on whole-genome sequencing.
Denoised log2 copy ratios were calculated across the genic intervals of the full genome for all sequenced samples. Values were calculated using the GATK4.0 CNV detection pipeline.
- https://cdn.elifesciences.org/articles/85023/elife-85023-table4-data2-v2.xlsx
-
Table 4—source data 3
Homozygous variants identified from in vitro selections by whole-genome sequencing.
Each entry represents a single clonal mutation that is present in the drug-selected clone but not in the matched parent line (i.e. arose during the drug selection process). Note we conducted whole-genome sequencing for two representative clones from each flask. The others were characterized via Sanger sequencing of the dhodh locus (see Source Data 4).
- https://cdn.elifesciences.org/articles/85023/elife-85023-table4-data3-v2.xlsx
-
Table 4—source data 4
Sanger sequencing of in vitro selected clones selected with DSM265 + TCMDC-125334.
- https://cdn.elifesciences.org/articles/85023/elife-85023-table4-data4-v2.zip
Reagent type (species) or resource | Designation | Source or reference | Identifiers | Additional information |
---|---|---|---|---|
Gene (Plasmodium falciparum) | dhodh; Pfdhodh | PlasmoDB | PF3D7_0603300 | |
Strain, strain background (Plasmodium falciparum) | 3D7 A10 | Goldberg lab at Washington University, St. Louis, MO, USA | 3D7 A10; 3D7_A10 | Cowell et al., 2018 |
Cell line (Plasmodium falciparum) | T-F1-C1 | This paper | Isolated from selection of 3D7 A10 parasites with TCMDC-125334; available upon request | |
Cell line (Plasmodium falciparum) | T-F1-C2 | This paper | Isolated from selection of 3D7 A10 parasites with TCMDC-125334; available upon request | |
Cell line (Plasmodium falciparum) | T-F1-C3 | This paper | Isolated from selection of 3D7 A10 parasites with TCMDC-125334; available upon request | |
Cell line (Plasmodium falciparum) | T-F2-C1 | This paper | Isolated from selection of 3D7 A10 parasites with TCMDC-125334; available upon request | |
Cell line (Plasmodium falciparum) | T-F2-C2 | This paper | Isolated from selection of 3D7 A10 parasites with TCMDC-125334; available upon request | |
Cell line (Plasmodium falciparum) | T-F2-C3 | This paper | Isolated from selection of 3D7 A10 parasites with TCMDC-125334; available upon request | |
Cell line (Plasmodium falciparum) | T-F2-C4 | This paper | Isolated from selection of 3D7 A10 parasites with TCMDC-125334; available upon request | |
Cell line (Plasmodium falciparum) | T-F2-C5 | This paper | Isolated from selection of 3D7 A10 parasites with TCMDC-125334; available upon request | |
Cell line (Plasmodium falciparum) | C276Y Parent | Mandt et al., 2019 | S1-F1-C1 | |
Cell line (Plasmodium falciparum) | D-T-F1-C1 | This paper | Isolated from selection of C276Y parent parasites with TCMDC-125334; available upon request | |
Cell line (Plasmodium falciparum) | D-T-F1-C2 | This paper | Isolated from selection of C276Y parent parasites with TCMDC-125334; available upon request | |
Cell line (Plasmodium falciparum) | D-T-F1-C3 | This paper | Isolated from selection of C276Y parent parasites with TCMDC-125334; available upon request | |
Cell line (Plasmodium falciparum) | D-T-F1-C4 | This paper | Isolated from selection of C276Y parent parasites with TCMDC-125334; available upon request | |
Cell line (Plasmodium falciparum) | D-T-F2-C1 | This paper | Isolated from selection of C276Y parent parasites with TCMDC-125334; available upon request | |
Cell line (Plasmodium falciparum) | D-T-F2-C2 | This paper | Isolated from selection of C276Y parent parasites with TCMDC-125334; available upon request | |
Cell line (Plasmodium falciparum) | D-T-F2-C3 | This paper | Isolated from selection of C276Y parent parasites with TCMDC-125334; available upon request | |
Cell line (Plasmodium falciparum) | D-T-F2-C4 | This paper | Isolated from selection of C276Y parent parasites with TCMDC-125334; available upon request | |
Cell line (Plasmodium falciparum) | D-T-F3-C1 | This paper | Isolated from selection of C276Y parent parasites with TCMDC-125334; available upon request | |
Cell line (Plasmodium falciparum) | D-T-F3-C2 | This paper | Isolated from selection of C276Y parent parasites with TCMDC-125334; available upon request | |
Cell line (Plasmodium falciparum) | D-T-F3-C3 | This paper | Isolated from selection of C276Y parent parasites with TCMDC-125334; available upon request | |
Cell line (Plasmodium falciparum) | DT-F1-C1 | This paper | Isolated from selection of 3D7 A10 parasites with DSM265 and TCMDC-125334; available upon request | |
Cell line (Plasmodium falciparum) | DT-F1-C2 | This paper | Isolated from selection of 3D7 A10 parasites with DSM265 and TCMDC-125334; available upon request | |
Cell line (Plasmodium falciparum) | DT-F1-C3 | This paper | Isolated from selection of 3D7 A10 parasites with DSM265 and TCMDC-125334; available upon request | |
Cell line (Plasmodium falciparum) | DT-F1-C4 | This paper | Isolated from selection of 3D7 A10 parasites with DSM265 and TCMDC-125334; available upon request | |
Cell line (Plasmodium falciparum) | DT-F2-C1 | This paper | Isolated from selection of 3D7 A10 parasites with DSM265 and TCMDC-125334; available upon request | |
Cell line (Plasmodium falciparum) | DT-F2-C2 | This paper | Isolated from selection of 3D7 A10 parasites with DSM265 and TCMDC-125334; available upon request | |
Cell line (Plasmodium falciparum) | DT-F2-C3 | This paper | Isolated from selection of 3D7 A10 parasites with DSM265 and TCMDC-125334; available upon request | |
Cell line (Plasmodium falciparum) | DT-F2-C4 | This paper | Isolated from selection of 3D7 A10 parasites with DSM265 and TCMDC-125334; available upon request | |
Cell line (Plasmodium falciparum) | DT-F2-C5 | This paper | Isolated from selection of 3D7 A10 parasites with DSM265 and TCMDC-125334; available upon request | |
Cell line (Plasmodium falciparum) | DT-F2-C6 | This paper | Isolated from selection of 3D7 A10 parasites with DSM265 and TCMDC-125334; available upon request | |
Sequence-based reagent | DHODH F1A | Mandt et al., 2019 | PCR primer | GTGTGATAGATAGCTCCAGTCG |
Sequence-based reagent | DHODH R1B | Mandt et al., 2019 | PCR primer | CGTTTGGCCCCTTGGGGTTATGG |
Sequence-based reagent | DHODH F2A | Mandt et al., 2019 | PCR primer | TTGATGGTGAAATATGTCATGACCTT |
Sequence-based reagent | DHODH R2A | Mandt et al., 2019 | PCR primer | CCAAGGGCTTCTTTTTTGTTGTATTAAACC |
Sequence-based reagent | DHODH F3A | Mandt et al., 2019 | PCR primer | GTCACATGATGAAAGATGCTAAGG |
Sequence-based reagent | DHODH R3B | Mandt et al., 2019 | PCR primer | CGCACTTATGTGTCGCCCG |
Sequence-based reagent | DHODH Front-F | Guler et al., 2013 | qPCR primer | TCCATTCGGTGTTGCTGCAGGATTTGAT |
Sequence-based reagent | DHODH Front-R | Guler et al., 2013 | qPCR primer | TCTGTAACTTTGTCACAACCCATATTA |
Sequence-based reagent | DHODH Rear-F | Guler et al., 2013 | qPCR primer | GTGTTAGCGGAGCAAAACTAAAAG |
Sequence-based reagent | DHODH Rear-R | Guler et al., 2013 | qPCR primer | ATAATTGACAAACTGAAGCACCTG |
Sequence-based reagent | Seryl t-RNA Synthetase-F | Guler et al., 2013 | qPCR primer | GGAACAATTCTGTATTGCTTTACC |
Sequence-based reagent | Seryl t-RNA Synthetase-R | Guler et al., 2013 | qPCR primer | AAGCTGCGTTGTTTAAAGCTC |
Sequence-based reagent | 18s Ribosomal RNA | Guler et al., 2013 | qPCR primer | ACAATTCATCATATCTTTCAATCGGTA |
Sequence-based reagent | 18s Ribosomal RNA | Guler et al., 2013 | qPCR primer | GCTGACTACGTCCCTGCCC |
Commercial assay or kit | Nextera XT DNA Library Preparation Kit | Illumina | Cat # FC-131–1024 | |
Commercial assay or kit | DNeasy Blood & Tissue Kit | QIAGEN | Cat # 69556 | |
Commercial assay or kit | LookOut Mycoplasma PCR Detection Kit | Sigma | Cat # MP0035 | |
Commercial assay or kit | Phusion High-Fidelity PCR Master Mix | New England BioLabs | Cat No: M0531S | |
Commercial assay or kit | DNA/RNA Shield | Zymo Research | Cat No: R1200-25 | |
Commercial assay or kit | SYBR Green Master Mix | Applied Biosystems | Cat No: A46109 | |
Chemical compound, drug | IDI-6273 | ChemDiv | ChemDiv4861-0080 | |
Chemical compound, drug | Genz669178 | Genzyme, a Sanofi Company | ||
Chemical compound, drug | DSM265 | Laboratory of Margaret Phillips, University of Texas Southwestern, Dallas, TX, USA | Phillips et al., 2015 | |
Chemical compound, drug | Atovaquone | Sigma-Aldrich | CAS No: 95233-18-4; Product No: A7986 | |
Chemical compound, drug | Dihydroartemisinin | Sigma-Aldrich | CAS No: 71939-50-9; Product No: 1200520 | |
Chemical compound, drug | TCMDC-125334 | Ross et al., 2018 | Additional material ordered from MolPort: MolPort-004-150-355 | |
Software, algorithm | HaplotypeCaller | Genome Analysis Toolkit (GATK) | See also: Cowell et al., 2018 | |
Software, algorithm | Platypus pipeline | https://sourceforge.net/projects/platypusmga/ ; Manary et al., 2014 | See also: Cowell et al., 2018 | |
Software, algorithm | Plasmodium CNV analysis pipeline | rwillia2001 /plasmodium_cnv_analysis — Bitbucket | Cowell et al., 2018 | |
Software, algorithm | Prism v9 | GraphPad | ||
Software, algorithm | CDD Vault | Collaborative Drug Discovery Inc. | ||
Software, algorithm | MultiExperimentViewer (MeV) version 4.9.0 | https://sourceforge.net/projects/mev-tm4/ | ||
Software, algorithm | Applied Biosystems QuantStudio Real-Time PCR Software | Thermo Fisher Scientific | ||
Software, algorithm | Flare v.4.0.3.40719 | Cresset-GroupTM | ||
Software, algorithm | PyMOL v. 4.6.0 | Intel |
Additional files
-
Supplementary file 1
Supplementary tables with additional experimental information for ‘Diverse evolutionary pathways pose a challenge to the use of collateral sensitivity as a strategy to suppress resistance’.
- https://cdn.elifesciences.org/articles/85023/elife-85023-supp1-v2.docx
-
MDAR checklist
- https://cdn.elifesciences.org/articles/85023/elife-85023-mdarchecklist1-v2.docx