ERK3/MAPK6 dictates CDC42/RAC1 activity and ARP2/3-dependent actin polymerization
Figures
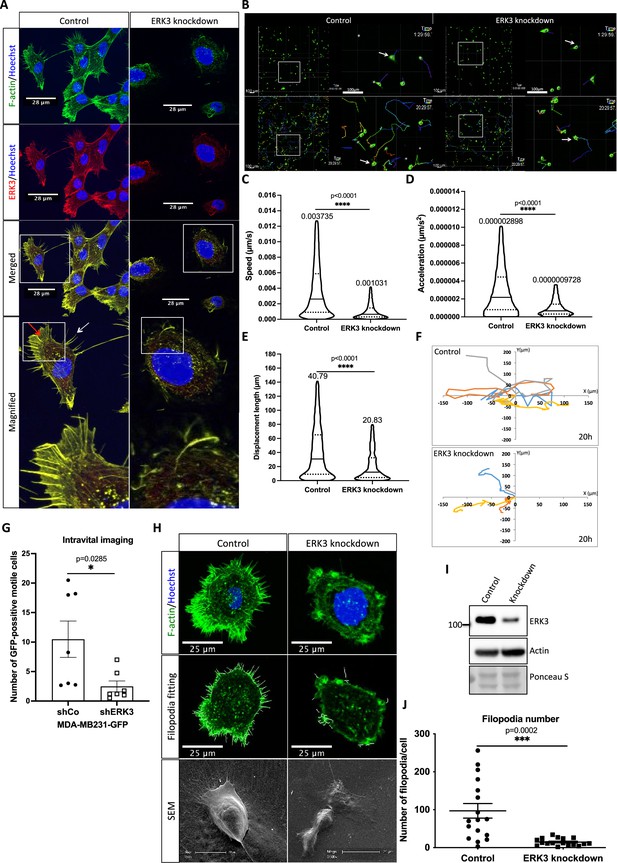
ERK3 localizes to the F-actin-rich protrusions and regulates mammary epithelial cells motility.
(A) Confocal analyses of control (shCo) and ERK3 knockdown (shERK3) human mammary epithelial cells (HMECs) co-stained with anti-F-actin (green) and anti-ERK3 antibodies (red). Hoechst staining (blue) was used to visualize cell nuclei. Scale bars 28 µm. Higher magnification images of the boxed areas are shown on the right. Red and white arrows exemplify lamellipodia and filopodia, respectively. (B–F) Single-cell tracking analyses of control (shCo) and ERK3 knockdown (shERK3) MDA-MB231-GFP cells. (B) Representative images of the cell migration assay were taken at the start (top panel) and end point (20 hr) (lower panel) of the full track length. Random fields were selected, and boxed areas were magnified on the right. Arrows indicate exemplified cell tracking at the beginning and at the end of the tracking. (C–E) Violin plots present cell distribution according to the analyzed motility parameters, calculated as described in the ‘Materials and methods’ section. Plotted are median (solid line) and 25%/75% quartiles (dashed lines). (C) Speed (µm/s), (D) acceleration (µm/s2), and (E) displacement length (µm) of 14,659 single cells (n = 14659) are depicted. Significance was determined using nonparametric Mann–Whitney test. *p<0.0332, **p<0.0021, ***p<0.0002, ****p<0.0001. Analyses of the migration tracks length and average speed are depicted in Figure 1—figure supplement 1A and B. (F) Cell migration patterns of four randomly selected control and ERK3 knockdown cells were plotted as x–y trajectories over the 20 hr tracking. (G) Quantification of the intravital imaging of the control (shCo) and ERK3 knockdown (shERK3) orthotopic mammary tumors (MDA-MB231-GFP). The number of GFP-positive motile cells was quantified as described in the ‘Materials and methods’ section and is presented as mean ± SEM from seven animals (n = 7) per condition. Please see representative Videos 5 and 6. Chemotactic responses of the ERK3-depleted cells were assessed in the presence of EGF and are presented in Figure 1—figure supplement 1C–F. ERK3 protein kinetics and stability in response to EGF are depicted in Figure 1—figure supplement 2. (H–J) Depletion of ERK3 alters leading edge protrusions in primary mammary epithelial cells. (H) Confocal F-actin (green)/Hoechst (blue) and SEM images analyses of control and ERK3 knockdown HMECs are presented after 4 hr starvation. For the confocal analyses, exemplified filopodia fitting was included in the middle panel. (I) Western blot analyses presenting ERK3 knockdown efficiency and total levels of β-actin. Ponceau S was used as a loading control. (J) Filopodia number was quantified as described in the ‘Materials and methods’ section and is presented as mean ± SEM of 17 single cells (n = 17); *p<0.0332, **p<0.0021, ***p<0.0002, ****p<0.0001, unpaired t-test. Effect of S189 phosphorylation of ERK3 on F-actin assembly is presented in Figure 1—figure supplement 3.
-
Figure 1—source data 1
Prism and Excel file for Figure 1C–E.
- https://cdn.elifesciences.org/articles/85167/elife-85167-fig1-data1-v3.zip
-
Figure 1—source data 2
Excel file for Figure 1F.
- https://cdn.elifesciences.org/articles/85167/elife-85167-fig1-data2-v3.zip
-
Figure 1—source data 3
Prism and Excel file for Figure 1G.
- https://cdn.elifesciences.org/articles/85167/elife-85167-fig1-data3-v3.zip
-
Figure 1—source data 4
Full membrane scans for western blot images for Figure 1I.
- https://cdn.elifesciences.org/articles/85167/elife-85167-fig1-data4-v3.zip
-
Figure 1—source data 5
Prism and Excel file for Figure 1J.
- https://cdn.elifesciences.org/articles/85167/elife-85167-fig1-data5-v3.zip
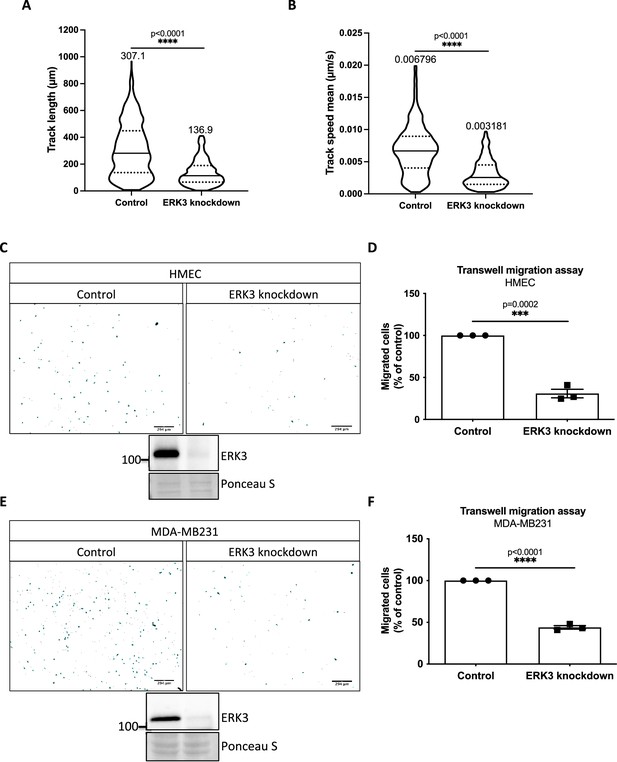
ERK3 regulates mammary epithelial cells motility.
(A, B) Tracks analyses depicting (A) length (µm) and (B) mean speed (µm/s) of 605 random cells (n = 605); *p<0.0332, **p<0.0021, ***p<0.0002, **** p<0.0001, Mann–Whitney test. Mean values for each parameter are indicated on top of the violin plots. (C–F) Chemotactic responses of control vs. ERK3-depleted (C, D) human mammary epithelial cells (HMECs) and (E, F) MDA-MB231 cells toward EGF. (C) and (E) depict representative ImageJ images of the analyzed Hoechst-stained migrated cells and immunoblots of ERK3 knockdown validation. Ponceau S was used as a loading control. (D) and (F) present comparisons of the chemotactic rate between control and ERK3 knockdown (D) HMECs and (F) MDA-MB231 cells. Quantification of three (n = 3) independent experiments is presented as mean ± SEM percentage of the control; *p<0.0332, **p<0.0021, ***p<0.0002, ****p<0.0001, unpaired t-test.
-
Figure 1—figure supplement 1—source data 1
Prisma file and original movie files for Figure 1—figure supplement 1A and B.
- https://cdn.elifesciences.org/articles/85167/elife-85167-fig1-figsupp1-data1-v3.zip
-
Figure 1—figure supplement 1—source data 2
Full membrane scans for western blot images for Figure 1—figure supplement 1C and E.
- https://cdn.elifesciences.org/articles/85167/elife-85167-fig1-figsupp1-data2-v3.zip
-
Figure 1—figure supplement 1—source data 3
Prism and Excel file for Figure 1—figure supplement 1D.
- https://cdn.elifesciences.org/articles/85167/elife-85167-fig1-figsupp1-data3-v3.zip
-
Figure 1—figure supplement 1—source data 4
Prism and Excel file for Figure 1—figure supplement 1F.
- https://cdn.elifesciences.org/articles/85167/elife-85167-fig1-figsupp1-data4-v3.zip
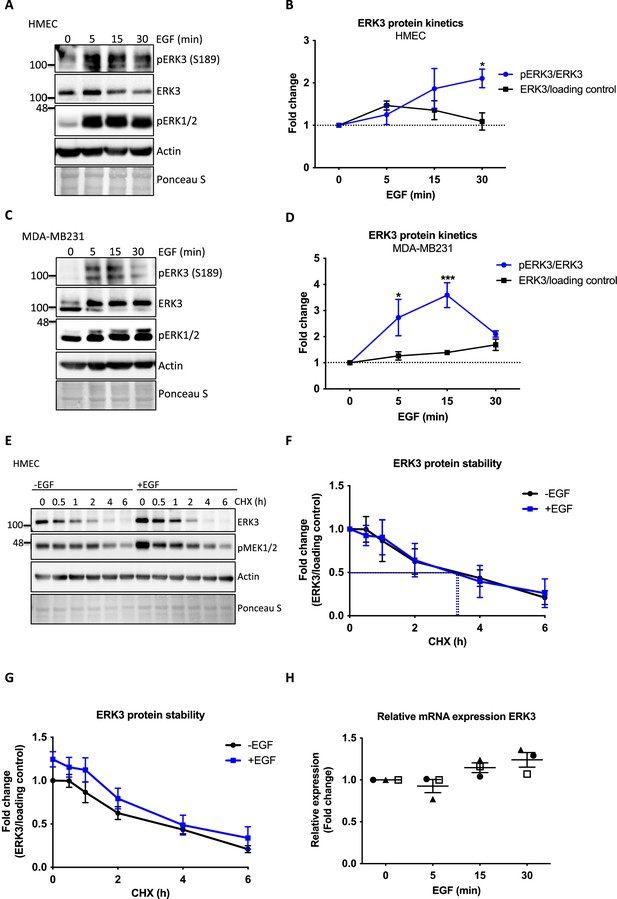
Effect of EGF on chemotactic responses and ERK3 protein kinetics in human mammary epithelial cells (HMECs).
(A, B) HMECs and (C, D) MDA-MB231 cells were stimulated with EGF at 0, 5, 15, and 30 min. Phosphorylation status of ERK3 at S189 and total protein levels were assessed. Phosphorylation levels of canonical ERK1/2 were used as a validation of EGF treatment. Actin and Ponceau S staining were used as loading controls. (A, C) Representative western blot analyses. Graphs in (B) and (D) depict changes in the phosphorylation and expression of ERK3 protein after normalization with total protein levels or internal loading control, respectively. Each time point was normalized to unstimulated cells (0). Mean fold changes ± SEM from three independent experiments (n = 3) are presented; *p<0.0332, **p<0.0021, ***p<0.0002, ****p<0.0001, two-way ANOVA, Bonferroni post-test. (E–H) Effect of EGF on ERK3 protein stability and expression. (E–G) ERK3 protein stability was assessed by CHX chase at 0, 0.5, 1, 2, 4, and 6 hr in the control (-EGF) and EGF pre-treated (+EGF) HMECs. (E) Representative western blot analyses of ERK3 levels. Phosphorylation of MEK1/2 was used as a positive control for EGF treatment. Actin and Ponceau S were used as loading controls. (F, G) ERK3 protein stability was quantified and is presented as fold change (F) with respect to the untreated cells (-EGF, 0 hr) and (G) with respect to the respective 0 hr time points of the untreated cells (-EGF) and EGF-stimulated (+EGF) cells. Data are presented as mean fold change ± SEM from three independent experiments (n = 3). (H) Quantitative RT-PCR analysis of ERK3 expression was assessed in HMECs upon 0, 5, 15, and 30 min of EGF treatment. Each biological replicate was measured in triplicates. Log2 fold change in gene expression is presented as mean ± SEM of three independent experiments (n = 3); *p<0.0332, **p<0.0021, ***p<0.0002, ****p<0.0001, one-way ANOVA, Tukey’s post-test.
-
Figure 1—figure supplement 2—source data 1
Full membrane scans for western blot images for Figure 1—figure supplement 2A, C, and E.
- https://cdn.elifesciences.org/articles/85167/elife-85167-fig1-figsupp2-data1-v3.zip
-
Figure 1—figure supplement 2—source data 2
Prism and Excel file for Figure 1—figure supplement 2B.
- https://cdn.elifesciences.org/articles/85167/elife-85167-fig1-figsupp2-data2-v3.zip
-
Figure 1—figure supplement 2—source data 3
Prism and Excel file for Figure 1—figure supplement 2D.
- https://cdn.elifesciences.org/articles/85167/elife-85167-fig1-figsupp2-data3-v3.zip
-
Figure 1—figure supplement 2—source data 4
Prism and Excel file for Figure 1—figure supplement 2F and G.
- https://cdn.elifesciences.org/articles/85167/elife-85167-fig1-figsupp2-data4-v3.zip
-
Figure 1—figure supplement 2—source data 5
Prism and Excel file for Figure 1—figure supplement 2H.
- https://cdn.elifesciences.org/articles/85167/elife-85167-fig1-figsupp2-data5-v3.zip
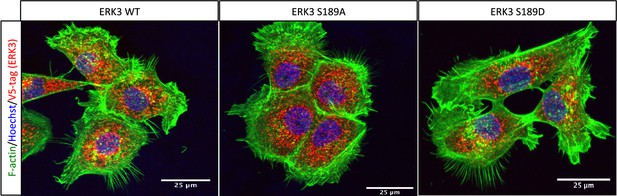
Effect of overexpressed S189 mutants on F-actin.
ERK3-depleted human mammary epithelial cells (HMECs) (shERK3, 3′ UTR) were transfected with V5-tagged wild type ERK3 (ERK3 WT) or S189 phosphorylation mutants (ERK3 S189A/ERK3 S189D). Expression of the exogenous ERK3 was determined by staining with V5-tag antibody (red). Cells were additionally co-stained with phalloidin (green) and Hoechst (blue) to visualize actin cytoskeleton and nuclei, respectively.
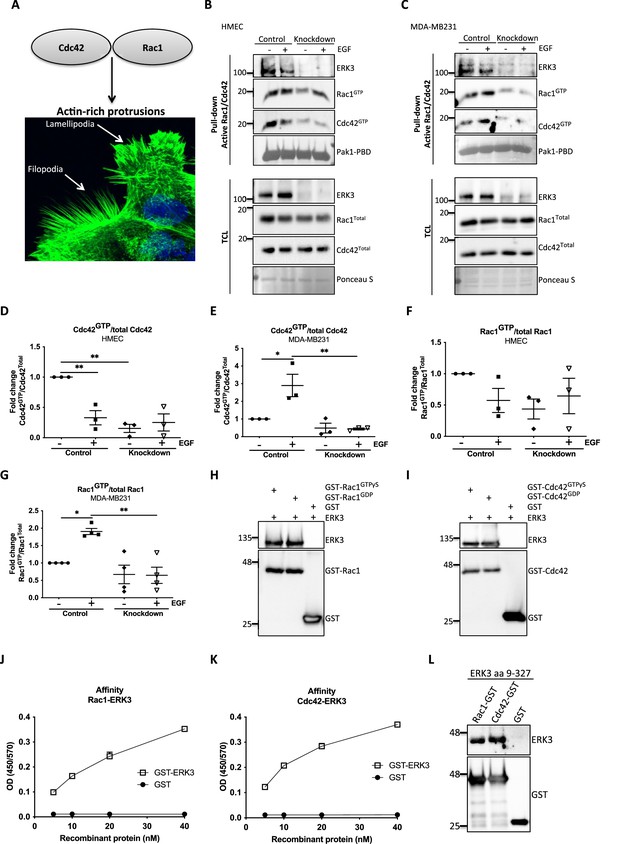
ERK3 binds RAC1 and CDC42 and regulates their activity.
(A) Confocal image of phalloidin-stained actin-rich protrusions in human mammary epithelial cells (HMECs), filopodia and lamellipodia. (B–G) In vivo pull-down of active RAC1 and CDC42 from control and ERK3-depleted (knockdown) (B) HMECs and (C) MDA-MB231 cells in the presence and absence of EGF. Levels of active (GTP-bound) CDC42 and RAC1 as well as the total protein expression were assessed and quantified. ERK3 knockdown efficiency was verified in the total cell lysate (TCL) as well as the co-immunoprecipitation of ERK3 with active RAC1 and CDC42. Relative levels of (D, E) active CDC42 and (F, G) RAC1 were calculated with respect to the total protein levels and are presented as mean ± SEM from minimum three (n = 3) independent experiments; *p<0.0332, **p<0.0021, ***p<0.0002, ****p<0.0001, one-way ANOVA, Tukey’s post-test. ERK3-dependent regulation of the RAC1 and CDC42 activity was assessed in multiple cell types and data are presented in Figure 2—figure supplements 1 and 2. (H, I) In vitro interaction between ERK3 and GDP/GTP bound (H) RAC1 and (I) CDC42 was assessed, GST was used as a negative control. Pull-down efficiency was assessed with GST antibody and levels of bound ERK3 were verified. The nucleotide-loading status of the purified RAC1 and CDC42 proteins is presented in Figure 2—figure supplement 3. (J, K) Concentration-dependent binding affinity of ERK3-GST protein to (J) RAC1 and (K) CDC42 was determined. Interacting ERK3/GST proteins were used at 5, 10, 20, and 40 nM concentrations. Data from three independent experiments run in triplicates are presented as mean ± SEM. (L) ERK3 kinase domain (aa 9–327) binds to RAC1 and CDC42. Representative analysis of the in vitro GST pull-down of RAC1 and CDC42 and the interaction with the ERK3 kinase domain recombinant protein. Binding affinity of ERK3 (aa 9–327) with PAK1-PBD was verified and is presented in Figure 2—figure supplement 4.
-
Figure 2—source data 1
Full membrane scans for western blot images for Figure 2B, C, H, I, and L.
- https://cdn.elifesciences.org/articles/85167/elife-85167-fig2-data1-v3.zip
-
Figure 2—source data 2
Prism and Excel file for Figure 2D.
- https://cdn.elifesciences.org/articles/85167/elife-85167-fig2-data2-v3.zip
-
Figure 2—source data 3
Prism and Excel file for Figure 2E.
- https://cdn.elifesciences.org/articles/85167/elife-85167-fig2-data3-v3.zip
-
Figure 2—source data 4
Prism and Excel file for Figure 2F.
- https://cdn.elifesciences.org/articles/85167/elife-85167-fig2-data4-v3.zip
-
Figure 2—source data 5
Prism and Excel file for Figure 2G.
- https://cdn.elifesciences.org/articles/85167/elife-85167-fig2-data5-v3.zip
-
Figure 2—source data 6
Prism and Excel file for Figure 2J.
- https://cdn.elifesciences.org/articles/85167/elife-85167-fig2-data6-v3.zip
-
Figure 2—source data 7
Prism and Excel file for Figure 2K.
- https://cdn.elifesciences.org/articles/85167/elife-85167-fig2-data7-v3.zip
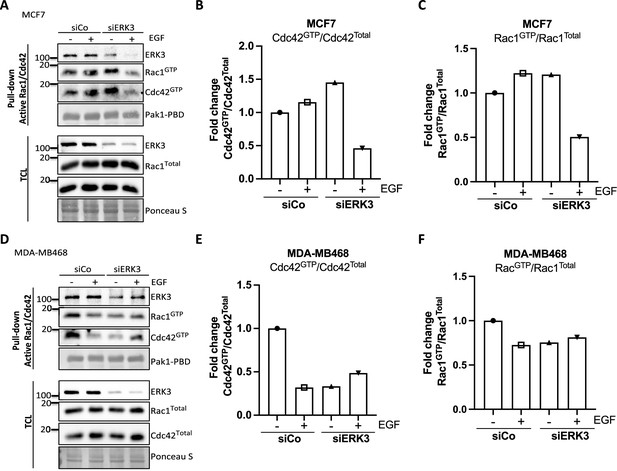
Pull-down of active RAC1 and CDC42 from control and ERK3-depleted (siERK3 knockdown) breast cancer cells.
The siRNA-mediated ERK3 knockdown was introduced in (A–C) MCF7 and (D–F) MDA-M468 cells. 48 hr post-transfection, cells were serum-starved, then treated with EGF (100 ng/ml) for 15 min and subjected to active RAC1/CDC42 pull-down assay. Levels of active (GTP-bound) CDC42 and RAC1 as well as co-precipitating ERK3 was assessed in the pulldown. The total protein levels and ERK3 knockdown efficiency were monitored in the total cell lysate (TCL). Relative levels of active CDC42 (B, E) and RAC1 (C, F) were calculated with respect to the total protein levels and are presented as mean fold change after normalization with the control (siCo).
-
Figure 2—figure supplement 1—source data 1
Full membrane scans for western blot images for Figure 2—figure supplement 1A and D.
- https://cdn.elifesciences.org/articles/85167/elife-85167-fig2-figsupp1-data1-v3.zip
-
Figure 2—figure supplement 1—source data 2
Prism and Excel file for Figure 1—figure supplement 1B and C.
- https://cdn.elifesciences.org/articles/85167/elife-85167-fig2-figsupp1-data2-v3.zip
-
Figure 2—figure supplement 1—source data 3
Prism and Excel file for Figure 1—figure supplement 1E and F.
- https://cdn.elifesciences.org/articles/85167/elife-85167-fig2-figsupp1-data3-v3.zip
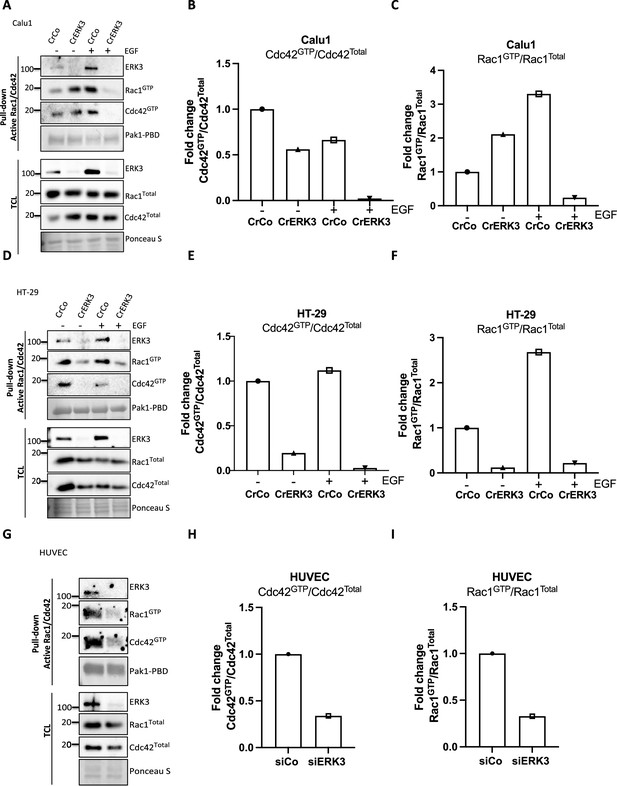
ERK3 binds Rac1 and Cdc42 and regulates their activity.
Pull-down of active RAC1 and CDC42 from CRISPR control and CRISPR ERK3 (A–C) Calu1 and (D–F) HT-29 cells. Serum-starved cells were treated with EGF (100 ng/ml) for 15 min and subjected to active RAC1/CDC42 pull-down assay. (G–I) HUVECs were cultured on 0.2% gelatin-coated plates and transfected with siERK3. 48 hr post-transfection, cells were subjected to active RAC1/CDC42 pull-down assay. Levels of active (GTP-bound) CDC42 and RAC1 as well as co-precipitating ERK3 was assessed in the pulldown. The total protein levels and ERK3 knockdown efficiency were monitored in the total cell lysate (TCL). Relative levels of active CDC42 (B, E, H) and RAC1 (C, F, I) were calculated with respect to the total protein levels and are presented as mean fold change after normalization with the control.
-
Figure 2—figure supplement 2—source data 1
Full membrane scans for western blot images for Figure 2—figure supplement 2A, D, and G.
- https://cdn.elifesciences.org/articles/85167/elife-85167-fig2-figsupp2-data1-v3.zip
-
Figure 2—figure supplement 2—source data 2
Prism and Excel file for Figure 2—figure supplement 2A and C.
- https://cdn.elifesciences.org/articles/85167/elife-85167-fig2-figsupp2-data2-v3.zip
-
Figure 2—figure supplement 2—source data 3
Prism and Excel file for Figure 2—figure supplement 2E and F.
- https://cdn.elifesciences.org/articles/85167/elife-85167-fig2-figsupp2-data3-v3.zip
-
Figure 2—figure supplement 2—source data 4
Prism and Excel file for Figure 2—figure supplement 2H and I.
- https://cdn.elifesciences.org/articles/85167/elife-85167-fig2-figsupp2-data4-v3.zip
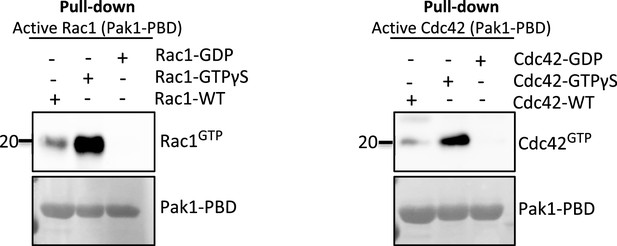
In vitro pull-down of purified native and GTP/GTP-loaded RAC1 and CDC42 proteins using PAK1-PBD-fusion beads as described in the ‘Materials and methods’ section.
Levels of active (GTP-bound) levels of RAC1 (left panel) and CDC42 (right panel) were assessed by western blot analyses.
-
Figure 2—figure supplement 3—source data 1
Full membrane scans for western blot images for Figure 2—figure supplement 3.
- https://cdn.elifesciences.org/articles/85167/elife-85167-fig2-figsupp3-data1-v3.zip
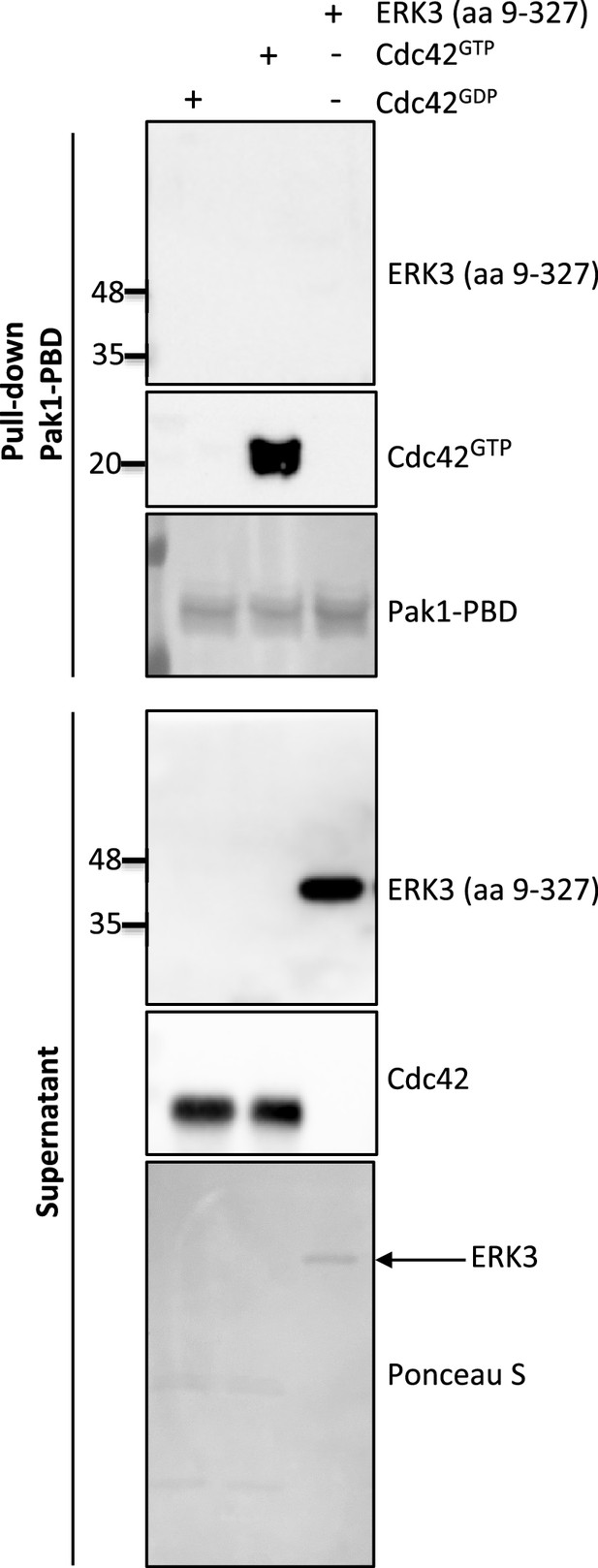
Western blot analyses of in vitro pull-down assay assessing binding affinity of ERK3 (aa 9–327) and PAK1-PBD.
CDC42 was pre-loaded with GTPγ or GDP as described in the ‘Materials and methods’ section and used as positive and negative control for the pull-down, respectively. Pre-loaded CDC42 or ERK3 (aa 9–327) recombinant proteins were incubated with the PAK1-PBD beads as described in the ‘Materials and methods’ section. Levels of active RhoGTPase were detected using a CDC42-specific antibody. PAK1-PBD protein was detected by Ponceau S staining. Supernatant obtained after the pull-down was analyzed to verify the presence of ERK3 and CDC42 used for the assay. The marked ERK3 protein was also detected with Ponceau S.
-
Figure 2—figure supplement 4—source data 1
Full membrane scans for western blot images for Figure 2—figure supplement 4.
- https://cdn.elifesciences.org/articles/85167/elife-85167-fig2-figsupp4-data1-v3.zip
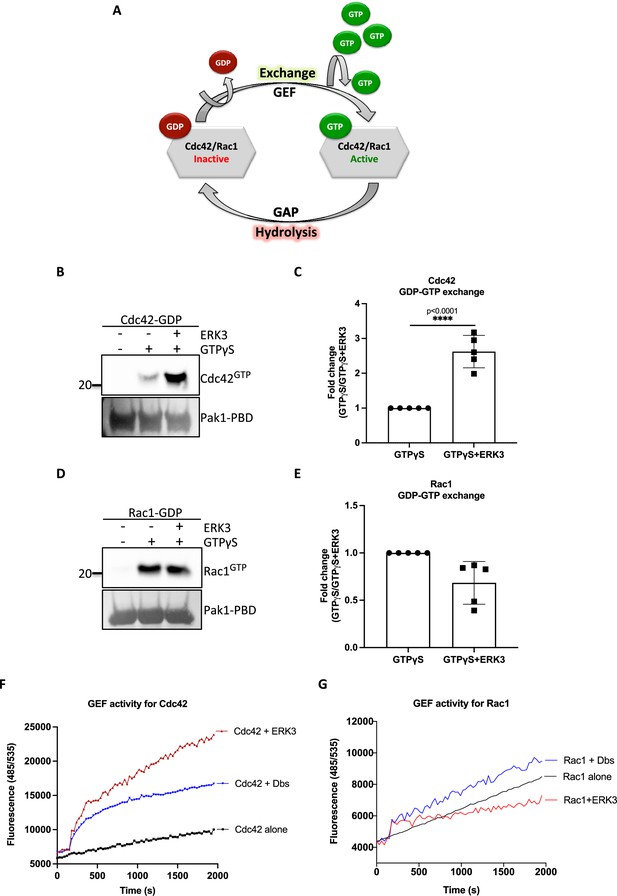
ERK3 kinase domain binds to RAC1 and CDC42 and facilitates nucleotide exchange on CDC42.
(A) Schematic representation of the GDP-GTP nucleotide exchange on CDC42 and RAC1. Guanine exchange factor (GEF) facilitates the GDP-GTP exchange on the RhoGTPases, resulting in the GTP-bound form of the CDC42 or RAC1. GTPase-activating protein (GAP) stimulates GTP hydrolysis. (B–E) Role of ERK3 in the GDP-GTP exchange was assessed in the in vitro assays as described in the 'Materials and methods' section for (B, C) CDC42 and (D–, E) RAC1. (B, D) Representative western blot analyses of active RAC1 and CDC42 pull-down, respectively, using PAK1-PBD fusion beads. Levels of active RhoGTPases were detected using RAC1- and CDC42-specific antibodies. Levels of PAK1-PBD protein were detected by Ponceau S staining and used for the quantification presented in (C) and (E). Fold change in GTP-loading was calculated by normalization of the signal obtained for the samples with GTPγS in the presence of ERK3 with samples incubated with GTPγS alone and is presented as mean ± SEM from (D) five (n = 5) independent experiments; *p<0.0332, **p<0.0021, ***p<0.0002, ****p<0.0001, unpaired t-test. (F, G) In vitro RhoGEF activity assay was performed to assess guanine nucleotide exchange activity on (F) CDC42 and (G) RAC1 in the presence and absence of recombinant ERK3 protein (9–327 aa). After six initial readings, Dbs-GEF or ERK3 protein were added at 0.5 µM final concentration. GEF activity was expressed as mean relative fluorescence units (RFUs) from at least three independent experiments. RhoGEF Dbs protein was used as a positive control. In vitro GEF activity of full-length ERK3 vs. ERK3 kinase domain towards CDC42 was compared as is presented in Figure 3—figure supplement 1A.
-
Figure 3—source data 1
Full membrane scans for western blot images for Figure 3B and D.
- https://cdn.elifesciences.org/articles/85167/elife-85167-fig3-data1-v3.zip
-
Figure 3—source data 2
Prism and Excel file for Figure 3C.
- https://cdn.elifesciences.org/articles/85167/elife-85167-fig3-data2-v3.zip
-
Figure 3—source data 3
Prism and Excel file for Figure 3E.
- https://cdn.elifesciences.org/articles/85167/elife-85167-fig3-data3-v3.zip
-
Figure 3—source data 4
Prism and Excel file for Figure 3F.
- https://cdn.elifesciences.org/articles/85167/elife-85167-fig3-data4-v3.zip
-
Figure 3—source data 5
Prism and Excel file for Figure 3G.
- https://cdn.elifesciences.org/articles/85167/elife-85167-fig3-data5-v3.zip
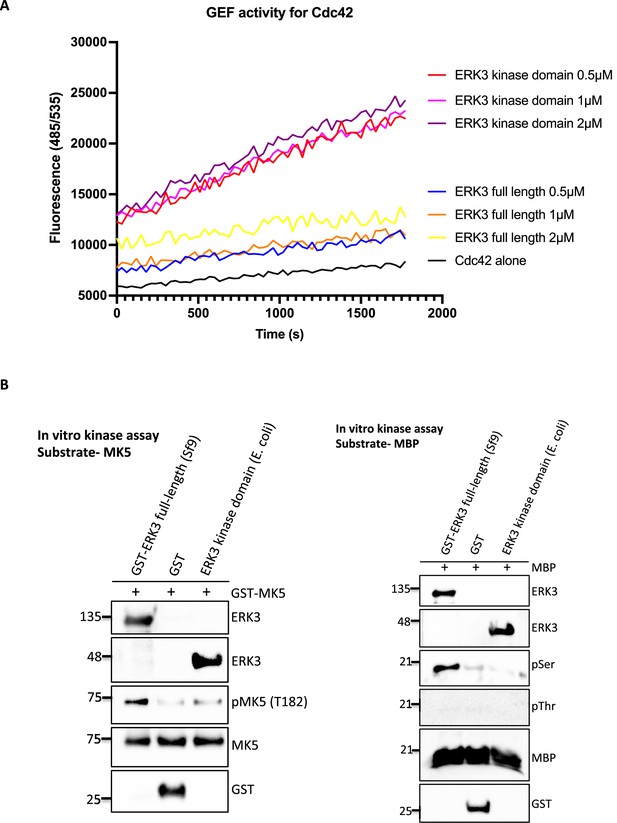
The ERK3 kinase domain regulates GEF activity of Cdc42.
(A) GEF activity of full-length ERK3 vs. ERK3 kinase domain toward CDC42 was assessed in vitro. ERK3 proteins were used at 0.5, 1, and 2 µM final concentration. GEF activity was expressed as mean relative fluorescence units (RFUs). (B) In vitro kinase assays were performed using full-length GST-ERK3 or kinase domain ERK3 (aa 9–327) and MK5 (left panel) or MBP (right panel) as a substrate. All reactions contain 1:4 kinase to substrate ratio. Kinase activity of ERK3 proteins was assessed using the specific phospho-MK5 (T182) antibody in case of MK5 and phospho-Ser/phospho-Thr antibody for MBP. Total protein levels of ERK3 were determined, GST control was included for the full-length GST-ERK3 and total levels of respective substrates MK5 (left panel) MBP (right panel).
-
Figure 3—figure supplement 1—source data 1
Prism and Excel file for Figure 3—figure supplement 1.
- https://cdn.elifesciences.org/articles/85167/elife-85167-fig3-figsupp1-data1-v3.zip
-
Figure 3—figure supplement 1—source data 2
Full membrane scans for the western blot images for Figure 3—figure supplement 1B.
- https://cdn.elifesciences.org/articles/85167/elife-85167-fig3-figsupp1-data2-v3.zip
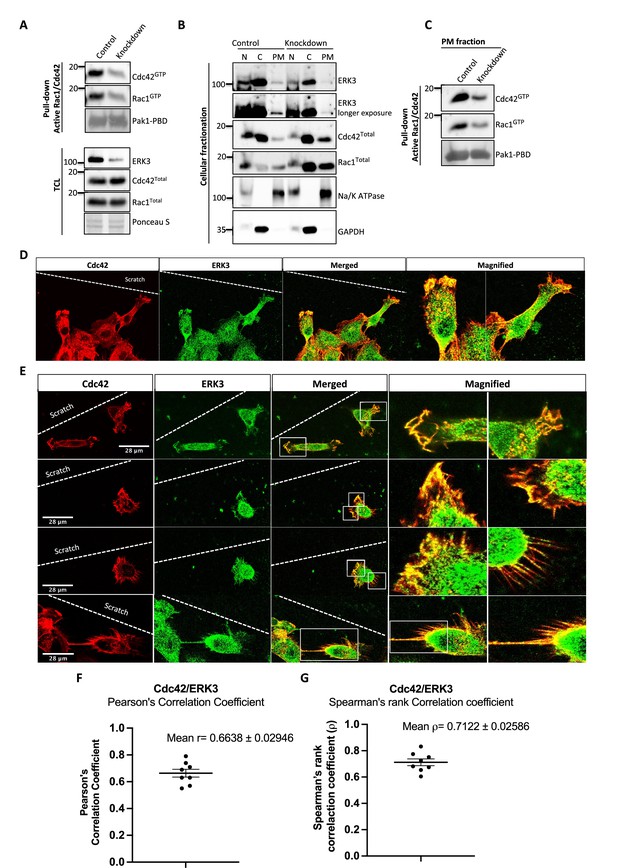
ERK3 colocalizes with CDC42 in polarized cells and regulates activity of both CDC42 and RAC1 at the plasma membrane (PM).
(A–C) Cell fractionation for control (siCo) and ERK3-depleted HMECs (siERK3). Fractionation was performed using Minute Plasma Membrane Protein Isolation and Cell Fractionation Kit (Cat# SM-005, Invent Biotechnologies) according to the manufacturer’s instruction. (A) Total cell lysate control was used to determine knockdown efficiency and activation status of RAC1 and CDC42 using active RAC1/CDC42 pull-down according to the manufacturer’s instructions (Cat# 16118/19, Thermo Fisher) and as described in the 'Materials and methods' section. (B) Cellular fractionation was performed. Expression levels of ERK3, RAC1, and CDC42 were assessed in nuclear (N), cytosolic (C), and PM fractions. Na/K ATPases and GAPDH were used as controls for the analyzed fractions. (C) Active RAC1/CDC42 pull-down was performed from the isolated PM fraction. (D–G) Colocalization of ERK3 and CDC42 in polarized cells. Human mammary epithelial cells (HMECs) were seeded and cultured on cover slips. When cells became around 70% confluent, scratch wounds were introduced to the cover slip using a 200 µl tip, medium was exchanged to supplement-free, and cells were cultured for additional 6 hr. Afterward, cells were fixed and subjected to IF staining as described in the ‘Materials and methods’ section with anti-CDC42 (red) (secondary antibody: anti-mouse Cy3; Cat# A10521, Thermo Fisher Scientific) and anti-ERK3 (green) (secondary antibody: Alexa Fluor 488; Cat# A11008, Thermo Fisher Scientific) antibodies. Merged images show the colocalization of both proteins in yellow. Magnification of the boxed regions is shown on the right for better visualization. In (D), a group of cells at the scratch site is presented and ERK3-CDC42 colocalization is marked with red arrows at the cell protrusions and with white arrows at the cell body. (E) Images representing ERK3-CDC42 colocalization at a single-cell level at the scratch site. Scale bars 28 µm. (F, G) Colocalization of ERK3 and CDC42 was analyzed as described in the ‘Materials and methods’ section and values for (F) Pearson’s correlation coefficient as well as the (G) Spearman’s rank correlation coefficient are presented for eight randomly selected cells (n = 8). Scores above 0 indicate a tendency towards colocalization with a perfect colocalization with a score of 1.
-
Figure 4—source data 1
Full membrane scans for western blot images for Figure 4A–C.
- https://cdn.elifesciences.org/articles/85167/elife-85167-fig4-data1-v3.zip
-
Figure 4—source data 2
Prism and Excel file for Figure 4F and G.
- https://cdn.elifesciences.org/articles/85167/elife-85167-fig4-data2-v3.zip
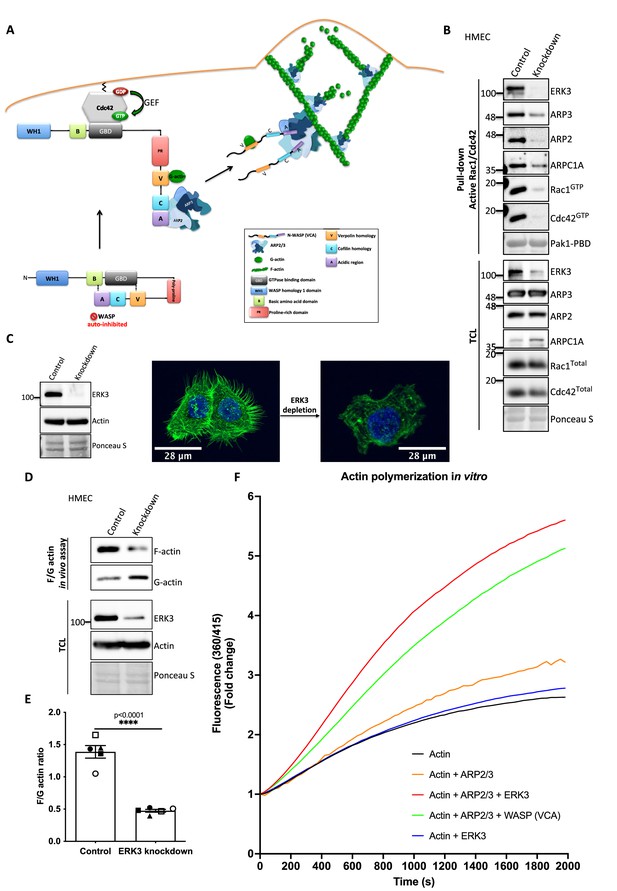
ERK3 acts as a nucleation-promoting factor (NPF) in ARP2/3-dependent actin polymerization in vitro and in vivo.
(A) Schematic overview of CDC42-WASP-stimulated ARP2/3-dependent actin polymerization based on the cited literature. The process involves ARP2/3 complex, WASP (VCA) as nucleation promoting factor, filamentous actin (F-actin), and monomeric actin (G-actin). In the initial step, CDC42 is activated by GEF-catalyzed exchange of GDP to GTP. Active CDC42 (CDC42-GTP) binds to the GTP-binding domain (GBD) on WASP, thereby displacing the VCA domain. While the V-verpolin-like motif binds actin monomer (G-actin), C-central and A-acidic domains bind and activate the ARP2/3 complex. Conformational changes induced by the binding of the ARP2/3 complex promote its binding to the actin filament, which is strengthened by the additional interaction of the ARP2/3 complex with WASP (VCA)-G-actin. Further conformational changes will secure the ARP2/3 complex on the filament and allow its binding to the actin monomer and the polymerization of the newly nucleated filament. Actin polymerizes at the fast-growing/barbed end, elongating toward the plasma membrane and the ARP2/3 complex would cross-link newly polymerizing filament to the existing filament. (B) ERK3 co-precipitates with active RAC1 and CDC42 in complex with ARP2/3. Active RAC1/CDC42 pull-down was performed using control and ERK3 knockdown human mammary epithelial cells (HMECs). Levels of the active RAC1 and CDC42 were assessed as well as the co-immunoprecipitation levels of ERK3, ARP2, ARP3, and ARPC1A. Levels of the total protein expression were evaluated in the total cell lysates (TCL) and Ponceau S staining was used as a loading control. (C–F) ERK3 regulates F-actin levels in vitro and in vivo. (C) Western blot analyses of control (CRISPR Co) and ERK3-depleted (CRISPR ERK3) HMECs are presented alongside with representative confocal images of F-actin staining. (D, E) In vivo analysis of F- and G-actin levels in HMECs upon ERK3 knockdown. (D) Representative western blot analyses of the enriched F- and G-actin fractions as well as the ERK3 knockdown validation and total actin levels in the TCL are presented. (E) F- and G-actin levels were quantified, and ratios were calculated from five (n = 5) independent experiments and are presented as mean ± SEM; *p<0.0332, **p<0.0021, ***p<0.0002, ****p<0.0001, unpaired t-test. Analyses of ERK3-dependent regulation of F-actin levels in cancerous MDA-MB231 cells is presented in Figure 5—figure supplement 1. Cellular colocalization between endogenous ERK3 and the ARP2/3 was assessed in the absence of CDC42 and is presented in Figure 5—figure supplement 2. (F) Effect of full-length ERK3 on ARP2/3-dependent pyrene actin polymerization was assessed using a pyrene actin polymerization assay. Polymerization induced by the VCA domain of WASP that served as a positive control (green) as well as the ARP2/3 (orange) and ERK3 protein alone (blue) are shown for reference. Actin alone (black) was used to establish a baseline of polymerization. Fluorescence at 360/415 was measured over time and is presented as mean fold change from at least three independent experiments after normalization to the first time point within the respective group. ARP2/3-dependent actin polymerization was measured in the presence of both ERK3 and WASP (VCA) domain, and the results are depicted in Figure 5—figure supplement 3.
-
Figure 5—source data 1
Full membrane scans for western blot images for Figure 5B and C and Figure 4D.
- https://cdn.elifesciences.org/articles/85167/elife-85167-fig5-data1-v3.zip
-
Figure 5—source data 2
Prism and Excel file for Figure 5E.
- https://cdn.elifesciences.org/articles/85167/elife-85167-fig5-data2-v3.zip
-
Figure 5—source data 3
Prism and Excel file for Figure 5F.
- https://cdn.elifesciences.org/articles/85167/elife-85167-fig5-data3-v3.zip
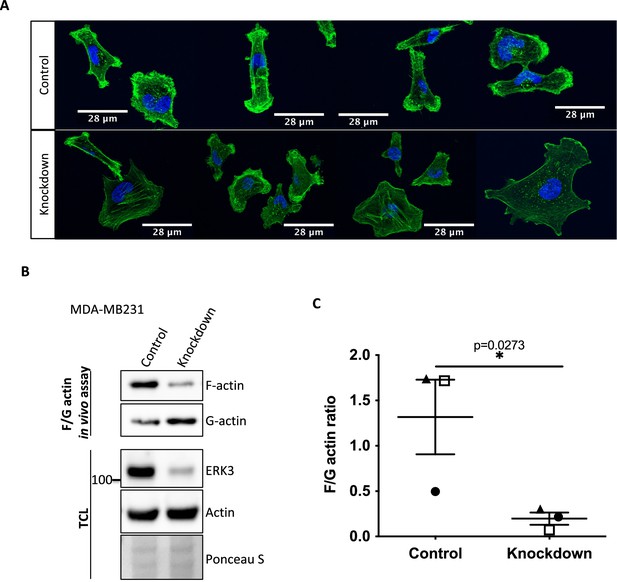
ERK3 regulates F-actin levels in MDA-MB231 cells.
F-actin distribution and levels were determined in control (shCo) and ERK3 knockdown (shERK3) MDA-MB231 cells. (A) Representative confocal images of the phalloidin green-stained F-actin merged with Hoechst staining (blue) of the nuclei. (B, C) Levels of F- and G-actin were quantified using F/G actin in vivo assay. (B) Representative western blot of the F- and G-actin fractions as well as the total cell lysate (TCL) evaluation of the ERK3 knockdown efficiency and total actin levels. Ponceau S was used as loading control.
-
Figure 5—figure supplement 1—source data 1
Full membrane scans for western blot images for Figure 5—figure supplement 1B.
- https://cdn.elifesciences.org/articles/85167/elife-85167-fig5-figsupp1-data1-v3.zip
-
Figure 5—figure supplement 1—source data 2
Prism and Excel file for Figure 5—figure supplement 1C.
- https://cdn.elifesciences.org/articles/85167/elife-85167-fig5-figsupp1-data2-v3.zip
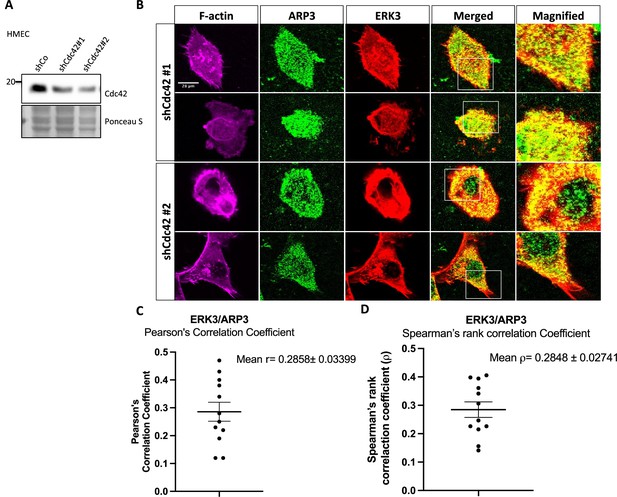
ERK3 and ARP3 (ARP2/3) colocalization in CDC42-knockdown cells.
Human mammary epithelial cells (HMECs) were transfected with two shRNAs targeting CDC42 (shCDC42#1/#2) as described in the 'Materials and methods' section. Afterward, cells were subjected to either (A) western blot analyses to validate to CDC42 knockdown or (B) IF staining as described in the 'Materials and methods' section and confocal imaging to determine localization of ERK3 and ARP3 in the CDC42 knockdown cells. F-actin was visualized using rhodamine phalloidin to assess cell morphology. (C, D) Graphs present (C) Pearson’s correlation coefficient and (D) Spearman’s rank correlation coefficient values obtained from the colocalization analyses of ERK3 and ARP3 as mean ± SEM from twelve randomly selected cells (n = 12).
-
Figure 5—figure supplement 2—source data 1
Full membrane scans for western blot images for Figure 5—figure supplement 2A.
- https://cdn.elifesciences.org/articles/85167/elife-85167-fig5-figsupp2-data1-v3.zip
-
Figure 5—figure supplement 2—source data 2
Prism and Excel file for Figure 5—figure supplement 2C and D.
- https://cdn.elifesciences.org/articles/85167/elife-85167-fig5-figsupp2-data2-v3.zip
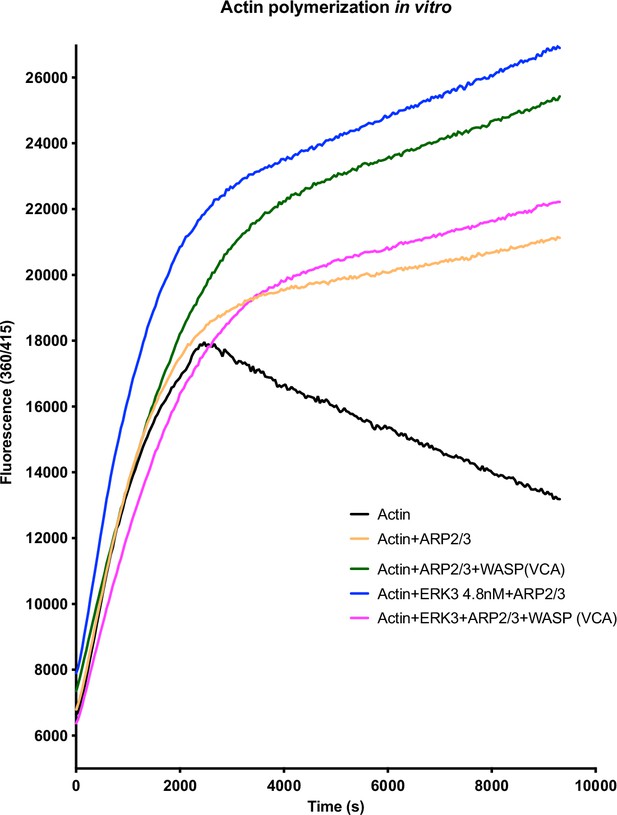
ARP2/3-dependent pyrene actin polymerization in vitro was measured in the presence of full-length ERK3 (4.8 nM) or WASP (VCA) domain (400 nM), as well as in the presence of both proteins at the indicated concentrations.
Fluorescence at 360/415 was measured over time.
-
Figure 5—figure supplement 3—source data 1
Prism and Excel file for Figure 5—figure supplement 3.
- https://cdn.elifesciences.org/articles/85167/elife-85167-fig5-figsupp3-data1-v3.zip
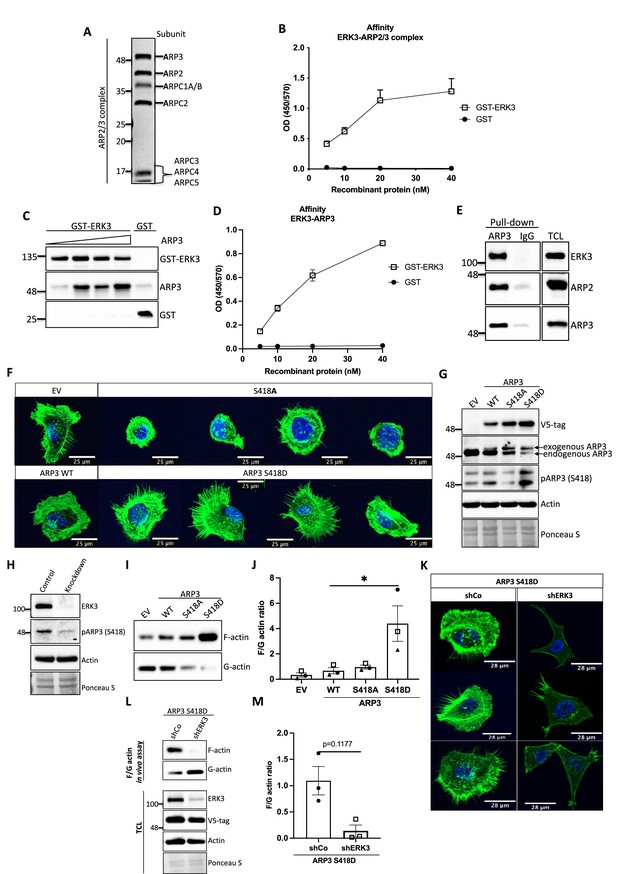
ERK3-dependent regulation of the ARP2/3 complex.
(A) Coomassie-stained 10% SDS-PAGE gel with 1 mg of the ARP2/3 protein complex (Cytoskeleton) presenting all the subunits. (B) Binding of increasing concentrations of recombinant GST-ERK3 to the ARP2/3 complex was measured by ELISA as described in the 'Materials and methods' section. (C) The interaction between GST-ERK3 and ARP3 was measured in vitro using GST-pull-down assay as described in the 'Materials and methods' section. (D) Binding affinity of the recombinant GST-ERK3 protein and ARP3 was assessed by ELISA as described in the 'Materials and methods' section and mean absorbance (Abs) ± SEM from three independent experiments is presented. (E) Co-immunoprecipitation (IP) of ARP2/3 protein complex and ERK3 was performed in HMECs using ARP3 antibody. Levels of precipitated ARP3 as well as co-IP of ARP2 and ERK3 were assessed. IgG control was included to determine the specificity of the interaction. Total cell lysate (TCL) was included to present expression levels of the verified interacting partners. Ponceau S staining was used as a loading control. (F, G) Actin phenotype of the human mammary epithelial cells (HMECs) was validated upon stable overexpression of the ARP3 non-phosphorylatable (S418A) and the phospho-mimicking (S418D) mutant, respectively. Wild type (WT) ARP3 was used as a control for the mutants and empty vector (EV) served negative control for the overexpression itself. (F) F-actin expression and organization in the negative (S418A) and phospho-mimicking (S418D) ARP3 mutant was visualized by green phalloidin and merged with the Hoechst staining of the nuclei. Four representative confocal images are presented. Images of EV-transfected and ARP3 WT-overexpressing HMECs are presented as controls. (G) Western blot validation of the overexpression efficiency and phosphorylation of ARP3 at S418. Anti-V5-tag antibody was used to detect levels of exogenous ARP3 WT, S418A, and S418D. Expression levels of the endogenous ARP3 were assessed as well as the phosphorylation at S418, total actin was validated. Ponceau S staining was used as a loading control. (H) Detection of the S418 phosphorylation of ARP3 in CRISPR ERK3 HMECs presented in Figure 5C and D. (I, J) Effect of the ARP3 mutant overexpression on F-actin levels was quantified using F/G actin in vivo assay. (I) Representative western blot analyses of F- and G-actin levels detected in fractions obtained from EV, ARP3 WT, S418A, S418D HMECs. (J) Quantification of the F/G actin ratios was performed for three (n = 3) independent experiments and is presented as mean ± SEM; *p<0.0332, **p<0.0021, ***p<0.0002, ****p<0.0001, one-way ANOVA, Tukey’s post-test. (K–M) Effect of ERK3 depletion on dense F-actin phenotype of the ARP3 S418D-overexpressing HMECs. HMECs stably overexpressing ARP3 S418D were transduced with lentiviral particles targeting ERK3 (shERK3) and stable knockdown was established as described in the 'Materials and methods' section. Cells were further subjected to analyses of the F-actin levels. (K) IF staining with Oregon Green Phalloidin 488 to visualize F-actin levels and organization. Scale bars 28 µm. (L, M) Effect of the ERK3 knockdown on F-actin levels was quantified in the ARP3 S418D mutant overexpressing HMECs using F/G actin in vivo assay. (L) Representative western blot analyses of F/G actin levels. ARP3 S418D-(V5-tagged) overexpression and ERK3 knockdown efficiency were validated in TCL. Actin and Ponceau S staining were used as loading controls. (M) Calculated ratios of F/G actin are presented as mean ± SEM from three (n = 3) independent experiments; *p<0.0332, **p<0.0021, ***p<0.0002, ****p<0.0001, paired t-test. Colocalization of endogenous ERK3 with endogenous and exogenous ARP3 mutant (S418D) was verified, and further effect of the ERK3 depletion on the RAC1 and CDC42 activity was assessed in ARP3 S418D-overexpressing HMECs and presented in Figure 6—figure supplement 2.
-
Figure 6—source data 1
Full membrane scans for western blot images for Figure 6A, C, E, G, I, and L.
- https://cdn.elifesciences.org/articles/85167/elife-85167-fig6-data1-v3.zip
-
Figure 6—source data 2
Prism and Excel file for Figure 6B.
- https://cdn.elifesciences.org/articles/85167/elife-85167-fig6-data2-v3.zip
-
Figure 6—source data 3
Prism and Excel file for Figure 6D.
- https://cdn.elifesciences.org/articles/85167/elife-85167-fig6-data3-v3.zip
-
Figure 6—source data 4
Prism and Excel file for Figure 6J.
- https://cdn.elifesciences.org/articles/85167/elife-85167-fig6-data4-v3.zip
-
Figure 6—source data 5
Prism and Excel file for Figure 6M.
- https://cdn.elifesciences.org/articles/85167/elife-85167-fig6-data5-v3.zip
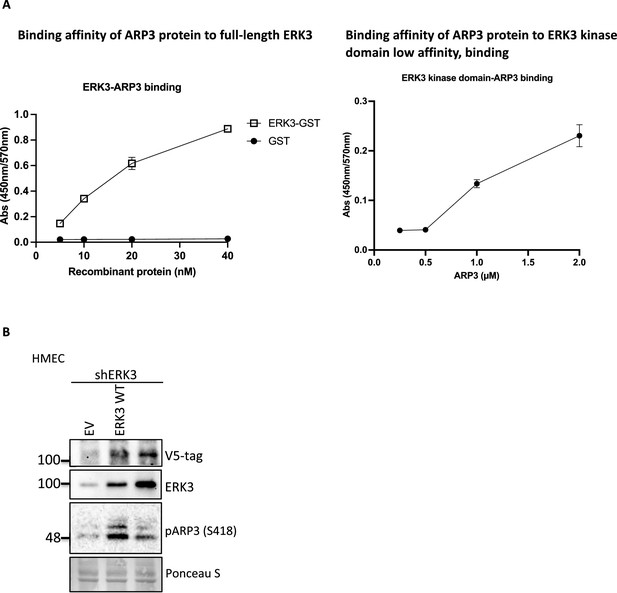
Binding affinities of ARP3 to full-length ERK3 or ERK3 kinase domain and phosphorylation of ARP3 by wild type or kinase-dead ERK3.
(A) ARP3 binding affinity of the recombinant full-length GST-ERK3 protein and kinase domain only was assessed by ELISA as described in the 'Materials and methods' section, and the mean absorbance (Abs) ± SEM from three independent experiments is presented. (B) Detection of the S418 phosphorylation of ARP3 in ERK3-knockdown HMECs (shERK3) complemented with wild type (WT) ERK3 and the kinase dead version (ERK3 KD).
-
Figure 6—figure supplement 1—source data 1
Prism and Excel file for Figure 6—figure supplement 1A.
- https://cdn.elifesciences.org/articles/85167/elife-85167-fig6-figsupp1-data1-v3.zip
-
Figure 6—figure supplement 1—source data 2
Full membrane scans for western blot images for Figure 6—figure supplement 1B.
- https://cdn.elifesciences.org/articles/85167/elife-85167-fig6-figsupp1-data2-v3.zip
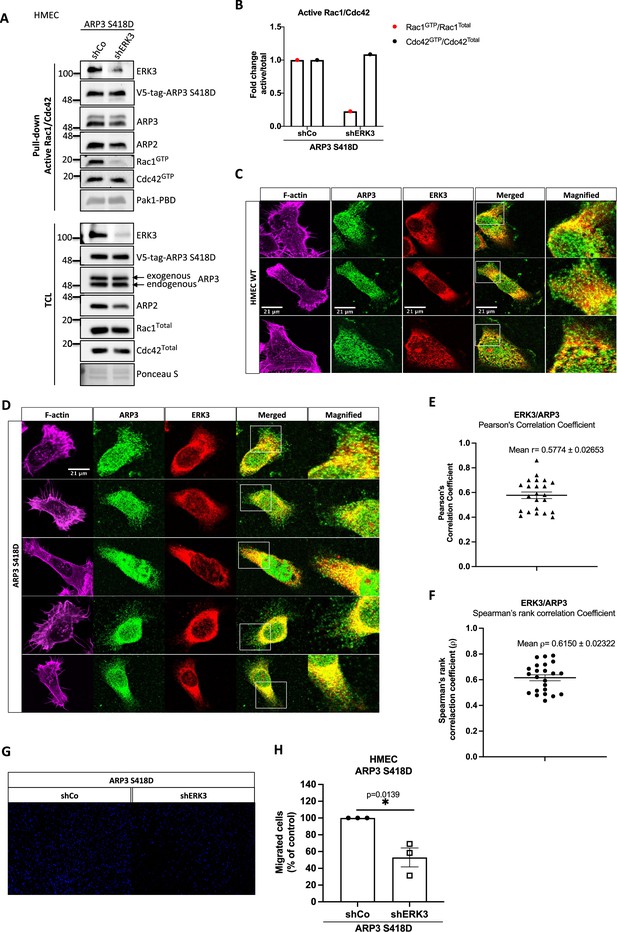
Effect of ERK3 knockdown on ARP2/3 interactions, Cdc42 and Rac1 activity, and migration of ARP3 S418D-overexpressing HMECs.
(A, B) Active RAC1/CDC42 pull-down according to the manufacturer’s protocol (Cat# 16118/19, Thermo Fisher) and ‘Materials and methods’ section. (A) Levels of active (GTP-bound) CDC42 and RAC1 as well as the total protein levels were assessed. Knockdown of ERK3 was validated by an ERK3 antibody, exogenous ARP3 (S418D) was detected using a V5-tag antibody, and total expression of ARP3 in the cells was determined using an ARP3 antibody. Detection of ARP2 was used as an additional control for the detection of the ARP2/3 complex in both active RAC1/CDC42 pull-down and total cell lysate (TCL). (B) Relative levels of active CDC42 and RAC1 were calculated with respect to the total protein levels and are presented as mean fold change after normalization with the control (shCo). (C, D) IF staining of ERK3 (secondary antibody: anti-mouse Alexa Fluor 647; Cat# A21235, Thermo Fisher Scientific) and ARP3 (secondary antibody: Alexa Fluor 488; Cat# A11008, Thermo Fisher Scientific) in (C) WT and (D) ARP3 S418D-overexpressing human mammary epithelial cells (HMECs). F-actin was visualized using rhodamine phalloidin to assess cell morphology. Scale bar: 21 µm. (E, F) Graphs present (E) Pearson’s correlation coefficient and (F) Spearman’s rank correlation coefficient values obtained from the colocalization analyses of ERK3 and ARP3 as mean ± SEM from 23 randomly selected cells (n = 23). (G, H) Effect of the ERK3 knockdown on the directional migration of ARP3 S418D-overexpressing HMECs was assessed and quantified using the Transwell system as described in the 'Materials and methods' section. Cells were seeded in the inserts in medium without supplements for 1 hr prior the beginning of the assay. Complete medium was used as a chemoattractant in the lower chamber. (G) Representative images of the analyzed inserts. (H) Percentage of the migrated ARP3 S418D shERK3 cells compared to the control (shCo) is presented as mean ± SEM from three (n = 3) independent experiments; *p<0.0332, **p<0.0021, ***p<0.0002, ****p<0.0001, t-test.
-
Figure 6—figure supplement 2—source data 1
Full membrane scans for western blot images for Figure 6—figure supplement 2A.
- https://cdn.elifesciences.org/articles/85167/elife-85167-fig6-figsupp2-data1-v3.zip
-
Figure 6—figure supplement 2—source data 2
Prism and Excel file for Figure 6—figure supplement 2B.
- https://cdn.elifesciences.org/articles/85167/elife-85167-fig6-figsupp2-data2-v3.zip
-
Figure 6—figure supplement 2—source data 3
Prism and Excel file for Figure 6—figure supplement 2E, F.
- https://cdn.elifesciences.org/articles/85167/elife-85167-fig6-figsupp2-data3-v3.zip
-
Figure 6—figure supplement 2—source data 4
Prism and Excel file for Figure 6—figure supplement 2H.
- https://cdn.elifesciences.org/articles/85167/elife-85167-fig6-figsupp2-data4-v3.zip
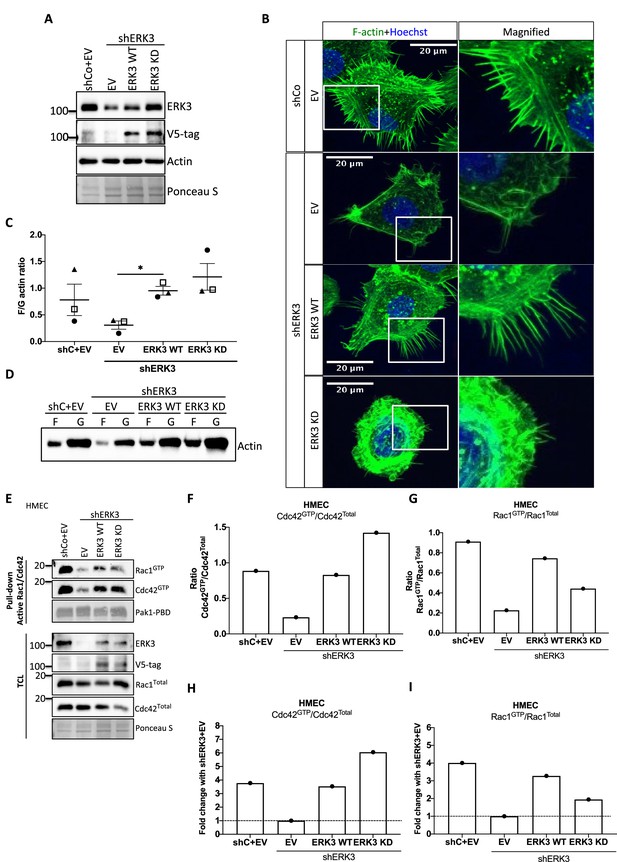
Kinase dead (KD) mutant of ERK3 inhibits actin-rich protrusions with no negative effect on F-actin levels.
(A) Western blot validation of the ERK3 levels and expression of the V5-tagged ERK3 constructs is presented as well as the total actin levels. Ponceau S was used as a loading control. (B) Confocal analyses of control (shCo EV) and ERK3-depleted (shERK3) human mammary epithelial cells (HMECs) reconstituted with wild type (ERK3 WT) or KD (ERK3 KD) (K49A/K50A) mutant of ERK3 after 4 hr starvation. Merged images of F-actin (green, phalloidin) and Hoechst are shown on the left representing cell phenotype and magnified regions of the cell edges on the right show the actin distribution. (C, D) Levels of F-actin in HMECs analyzed by phalloidin staining were quantified using a F/G actin in vivo assay. (C) Calculated ratios of F/G actin are depicted as mean ± SEM from three (n = 3) independent experiments; *p<0.0332, **p<0.0021, ***p<0.0002, ****p<0.0001, one-way ANOVA, Tukey’s post-test. (D) Representative western blot analyses of F- and G-actin levels. (E–I) Active RAC1 and CDC42 pull-down assays were performed from control (shCo) and ERK3-depleted (shERK3, 3′ UTR) HMEC reconstituted with either WT or KD ERK3 as described in the 'Materials and methods' section. PAK1-PBD was used to capture the active form of CDC42 and RAC1 in the respective cell lysates. Levels of active (GTP-bound) CDC42 and RAC1, as well as the total protein expression were assessed, ERK3 knockdown efficiency and overexpression was verified in the total cell lysate (TCL) using ERK3 antibody and V5-tag antibody to detect the exogenous WT and KD version of ERK3. (E) Western blot analyses are presented. (F, G) Relative levels of active CDC42 and RAC1 were calculated with respect to the total protein levels and are presented as ratio of GTP/Total RhoGTPases. (H, I) Additionally, the ratio of GTP/total RAC1 and CDC42 was normalized to the control cells (shERK3+EV) and is presented as fold change in activation.
-
Figure 7—source data 1
Full membrane scans for western blot images for Figure 7A, D, and E.
- https://cdn.elifesciences.org/articles/85167/elife-85167-fig7-data1-v3.zip
-
Figure 7—source data 2
Prism and Excel file for Figure 7C.
- https://cdn.elifesciences.org/articles/85167/elife-85167-fig7-data2-v3.zip
-
Figure 7—source data 3
Prism and Excel file for Figure 7F–I.
- https://cdn.elifesciences.org/articles/85167/elife-85167-fig7-data3-v3.zip
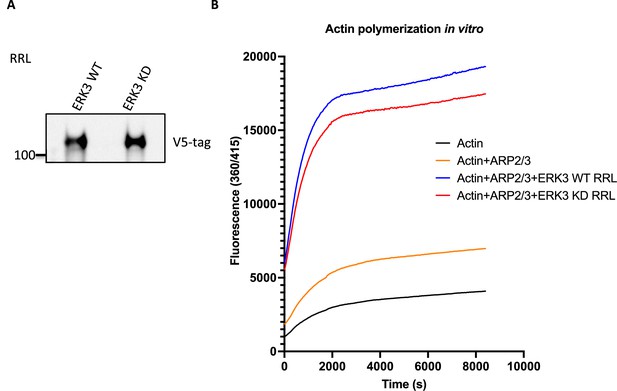
Effect of RRL-expressed wildtype or kinase-dead ERK3 on ARP2/3-dependent pyrene actin polymerization.
(A) Western blot analysis of the RRL-expressed ERK3 proteins. (B) ARP2/3-dependent pyrene actin polymerization in vitro was assessed in the presence of wild type (WT) or kinase dead (KD) ERK3 proteins expressed in rabbit reticulocyte lysates (RRL). The baseline of actin alone was measured in the presence of the same volume of the control RRL as for the expressed proteins. Fluorescence at 360/415 was measured over time, and results are shown as mean fluorescence from three experiments.
-
Figure 7—figure supplement 1—source data 1
Full membrane scans for western blot images for Figure 7—figure supplement 1A.
- https://cdn.elifesciences.org/articles/85167/elife-85167-fig7-figsupp1-data1-v3.zip
-
Figure 7—figure supplement 1—source data 2
Prism and Excel file for Figure 7—figure supplement 1B.
- https://cdn.elifesciences.org/articles/85167/elife-85167-fig7-figsupp1-data2-v3.zip
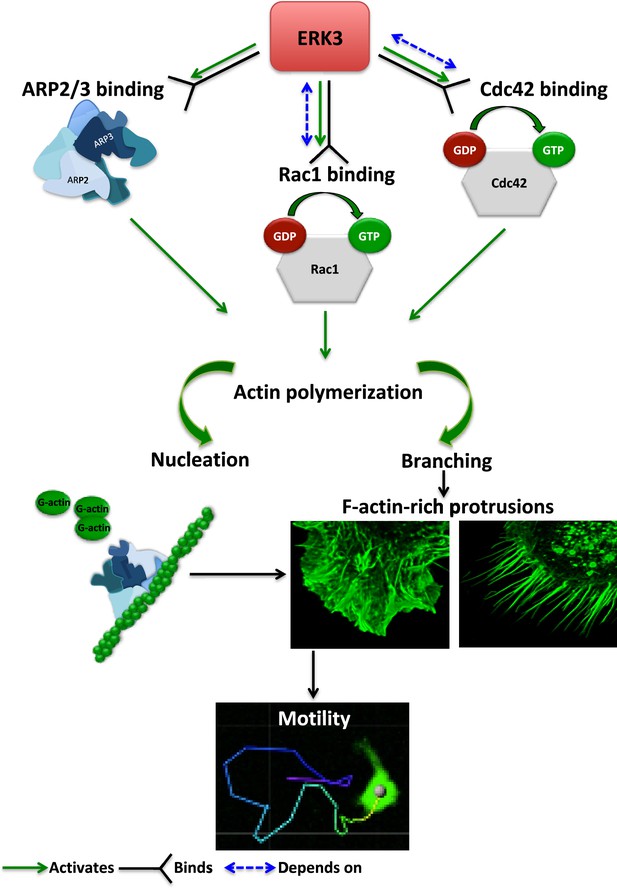
Schematic summary of the ERK3-dependent mechanisms regulating actin cytoskeleton and cell motility.
ERK3 directly binds and activates the ARP2/3 protein complex as well as the CDC42 and RAC1 Rho GTPases. Activation of the ARP2/3 complex and RAC1/CDC42 is required for nucleation of the new actin filaments, elongation, and branching into the lamellipodia and filopodia. ERK3 regulates actin-rich protrusions, which play a direct role in cell motility.

Western Blot analyses are presented to demonstrate the different expression efficiency of ERK3 WT (full-length) and truncated ERK3 (aa 1-340, kinase domain only) constructs with either N-Tap Flag tag (left panel) or C-Tap V5-tag (right panel).
1 µg cDNA was used for the transfection in 6-well plates as described in the methods section. Western Blot analyses show the total cell lysates used for the active Rac1/Cdc42 pull-down on the same membranes using two different antibodies (anti-Flag or anQ-V5, respectively).

ERK3 peptides (aa 180-190) are presented from the MassSpec analyses using the recombinant kinase domain of ERK3 (top panel) or full-length ERK3 (bottom panel).
Arrows indicate S189. Yellow marking indicates the identified peptide, and post-translational modifications are marked in green (Scaffold).
Videos
Movies representing motility of control (Videos 1 and 2) and ERK3-knockdown (Videos 3 and 4) MDA-MB231 cells.
Movies representing motility of control (Videos 1 and 2) and ERK3-knockdown (Videos 3 and 4) MDA-MB231 cells.
Movies representing motility of control (Videos 1 and 2) and ERK3-knockdown (Videos 3 and 4) MDA-MB231 cells.
Movies representing motility of control (Videos 1 and 2) and ERK3-knockdown (Videos 3 and 4) MDA-MB231 cells.
Representative videos following the motility of the control (shCo) and ERK3 knockdown (shERK3) GFP-expressing MDA-MB231 cells.
Representative videos following the motility of the control (shCo) and ERK3 knockdown (shERK3) GFP-expressing MDA-MB231 cells.
Tables
Reagent type (species) or resource | Designation | Source or reference | Identifiers | Additional information |
---|---|---|---|---|
Cell line (Homo sapiens) | Immortalized human mammary epithelial cells hTERT-HME1 (ME16C) (HMECs) | ATCC | ATCC CRL-4010 | |
Cell line (H. sapiens) | MDA-MB231 | DSMZ | Cat# ACC 732 | |
Cell line (H. sapiens) | MDA-MB231-GFP | Gift from Prof. Dr. Mohamed Bentires-Alj (University of Basel, Department of Biomedicine) | ||
Cell line (H. sapiens) | 293T cells | Gift from Dr. Andreas Ernst (Goethe-University Frankfurt am Main, IBC2) | ||
Sequence-based reagent-shRNA | shRNA: ERK3 (MAPK6) NM_002748.x-3734s1c1 CCGGGCTGTCCACGTACTTAATTTACTCGAGTAAATTAAGTACGTGGACAGCTTTTT | MISSION shRNA Human Library (Sigma) | TRCN0000001568 | |
Sequence-based reagent-shRNA | shCDC42#1 NM_001791.2-471s1c1 | MISSION shRNA Human Library (Sigma) | TRCN0000047628 | Sequence: CCGGCCCTCTACTATTGAGAAACTTCTCGAGAAGTTTCTCAATAGTAGAGGGTTTTTG |
Sequence-based reagent-shRNA | shCDC42CDC42#2 NM_001791.2-193s1c1 | MISSION shRNA Human Library (Sigma) | TRCN0000047629 | Sequence: CCGGCGGAATATGTACCGACTGTTTCTCGAGAAACAGTCGGTACATATTCCGTTTTTG |
Sequence-based reagent-siRNA | siRNA: ERK3 (MAPK6), Hs_MAPK6_5: 5′- AGUUCAAUUUGAAAGGAAATT-3′ | QIAGEN | Cat# SI00606025 | |
Sequence-based reagent-CRISPR/Cas9 | CrispR/Cas gRNA sequences targeting ERK3 (MAPK6) (CrispR ERK3) | Sigma, Doench et al., 2016 | Designed by Rule Set 2 of Azimuth 2.0 as described previously | #1 5′-CACCGAGCCAATTAACAGACGATGT-3′ #2 5′-CACCGATACTTGTAACTACAAAACG-3′ #3 5′-CACCGCTGCTGTTAACCGATCCATG-3′ |
Recombinant DNA reagent-cDNA | ERK3 K49A K50A kinase dead mutant | Site-directed mutagenesis | Primers sequence: frw_5′ GCAATTGTCCTTACTGATCCCCAGAGTGTC, rev_5′ CGCGATGGCTACTCTTTTGTCACAGTC | |
Recombinant DNA reagent-cDNA | ARP3 pENTR223 | Harvard | HsCD00375598 | |
Recombinant DNA reagent-cDNA | ARP3 S418A | Eurofins | Cat# #11104144057-1-5 | |
Recombinant DNA reagent-cDNA | ARP3 S418D | Eurofins | Cat# #11104144057-1-6 | |
Recombinant DNA reagent-cDNA | pGEX-2T-RAC1-WT | Addgene | Addgene plasmid# 12977 | |
Recombinant DNA reagent-cDNA | pGEX-2T-CDC42-WT | Addgene | Addgene plasmid #12969 | |
Recombinant DNA reagent-cDNA | pGEX2TK-PAK1 (70–117) containing the p21-binding domain (PBD) | Addgene | Addgene plasmid# 12217 | |
Sequence-based reagent | qRT-PCR primers, human ERK3 (MAPK6) | Sigma | Frw_5′ ATGGATGAGCCAATTTCAAG, Rv_5′ CTGACAATCATGATACCTTTCC | |
Sequence-based reagent | qRT-PCR primers, human 18S | Sigma | Cat# 88-8086 | Frw_5′ AGAAACGGCTACCACATCCA, Rv_5′ CACCAGACTTGCCCTCCA |
Commercial assay or kit | Cell Fractionation Kit | Invent Biotechnologies | Cat# SM-005 | |
Commercial assay or kit | RAC1 pull-down and detection kit | Thermo Fisher Scientific | Cat# 16118 | |
Commercial assay or kit | CDC42 pull-down and detection kit | Thermo Fisher Scientific | Cat# 16119 | |
Commercial assay or kit | TNT T7 Quick Coupled transcription/Translation System | Promega | Cat# TM045 | |
Sequence-based reagent | qRT-PCR primers, human ERK3 (MAPK6) | Sigma | Frw_5′ ATGGATGAGCCAATTTCAAG, Rv_5′ CTGACAATCATGATACCTTTCC | |
Sequence-based reagent | qRT-PCR primers, human 18S | Sigma | Cat# 88-8086 | Frw_5′ AGAAACGGCTACCACATCCA, Rv_5′ CACCAGACTTGCCCTCCA |
Commercial assay or kit | Cell Fractionation Kit | Invent Biotechnologies | Cat# SM-005 | |
Commercial assay or kit | RAC1 pull-down and detection kit | Thermo Fisher Scientific | Cat# 16118 | |
Commercial assay or kit | CDC42 pull-down and detection kit | Thermo Fisher Scientific | Cat# 16119 | |
Commercial assay or kit | TNT T7 Quick Coupled transcription/Translation System | Promega | Cat# TM045 | |
Commercial assay or kit | RhoGEF Exchange Assay | Cytoskeleton | Cat# BK100 | |
Commercial assay or kit | G-actin/F-actin in vivo assay | Cytoskeleton | Cat# BK037 | |
Commercial assay or kit | Actin polymerization biochem kit | Cytoskeleton | Cat# BK003 | |
Commercial assay or kit | Two-chamber transwell system | Corning | Cat# 3422 | |
Antibody | Anti-beta-Actin HRP conjugated (mouse monoclonal) | Abcam | Cat# ab49900 | WB 1:40,000 |
Antibody | Anti-GAPDH antibody (mouse monoclonal) | GeneTex | Cat# GTX627408 | WB 1:1000 |
Antibody | HRP-conjugated secondary antibody for rabbit IgG (goat) | Invitrogen | Cat# A16096 | WB 1:40,000 |
Antibody | HRP-conjugated secondary antibody for rabbit IgG (goat) | Invitrogen | Cat# 32460 | WB 1:2000 |
Antibody | HRP-conjugated secondary antibody for mouse IgG (sheep) | GE Healthcare Life Sciences | Cat# NA9310 | WB 1:20,000 |
Antibody | Anti-human ERK3 (mouse monoclonal) | R&D | Cat# MAB3196 | IF 1:400 |
Antibody | Anti-phospho-ERK3 (pSer189) (rabbit polyclonal) | Sigma | Cat# SAB4504175 | 1:500 |
Antibody | Anti-ARPC1A (rabbit polyclonal) | Sigma | Cat# HPA004334 | 1:500 |
Antibody | Anti-phospho-p44/42 MAPK (ERK1/2) (Thr202/Tyr204) (rabbit polyclonal) | Cell Signaling Technology | Cat# 9101L | WB 1:1000 |
Antibody | Anti-V5 Tag (mouse monoclonal) | Invitrogen | Cat # R960-25 | 1:1000 |
Antibody | Anti-GST (rabbit polyclonal) | Cell Signaling Technology | Cat# 2622S | 1:1000 |
Antibody | Anti-normal rabbit IgG (rabbit polyclonal) | Cell Signaling Technology | Cat# 2729 | Used as a control for IP |
Antibody | Anti-GST (B-14) (mouse monoclonal) | Santa Cruz Biotechnology | Cat# sc-138 | WB 1:1000 |
Antibody | Anti-RAC1 (mouse monoclonal) | BD Transduction Laboratories | Cat# 610651 | WB 1:1000 |
Antibody | Anti-CDC42 (mouse monoclonal) | BD Transduction Laboratories | Cat# 610929 | WB 1:1000 |
Antibody | ARP3 (rabbit monoclonal) | Abcam | Cat# ab151729 | WB 1:1000 |
Antibody | ARP2 (rabbit monoclonal) | Abcam | Cat# ab128934 | WB 1:1000 |
Antibody | Anti-ERK3 (mouse monoclonal) | R&D | Cat# MAB3196 | 1:250 |
Antibody | Anti-rabbit IgG-Alexa 488 (goat) | Thermo Fisher Scientific | Cat# A11008 | IF, working concentration: 5 µg/ml |
Antibody | Anti-mouse IgG-Cyanine3 (goat) | Thermo Fisher Scientific | Cat# A10521 | IF, working concentration:: 5 µg/ml |
Chemical compound, drug | Hoechst 33342 | Invitrogen | Cat# H3570 | 10 µg/ml |
Chemical compound, drug | Oregon Green 488 Phalloidin | Invitrogen | Cat# O7466 | 1:50 |
Chemical compound, drug | Human EGF recombinant protein (human) | Invitrogen | Cat# RP-10927 | 100 ng/ml |
Chemical compound, drug | Cycloheximide (CHX) | Sigma | Cat# C-7698 | 100 µg/ml |
Peptide, recombinant protein | ERK3 (MAPK6) kinase domain (aa 9–327) (human) | Crelux | Customized | |
Peptide, recombinant protein | ERK3 (MAPK6) recombinant protein (human) | Aviva Systems Biology | Cat# OPCA01714 | |
Peptide, recombinant protein | ERK3 (MAPK6) protein full-length (human) | SignalChem | Cat# M31 | |
Peptide, recombinant protein | WASP Protein VCA Domain (human) | Cytoskeleton | Cat# VCG03 | |
Peptide, recombinant protein | ARP2/3 Protein Complex: Porcine Brain | Cytoskeleton | Cat# RP01P | |
Software, algorithm | ImageJ | RRID:SCR_003070 | RRID:SCR_003070 https://imagej.net/ | |
Software, algorithm | ImageJ Coloc2 Plugin | Self-modified version as described by French et al., 2008. | Self-modified version of ImageJ; RRID:SCR_003070 | |
Software, algorithm | Fiji | RRID:SCR_003070 | Fiji (RRID:SCR_002285) | |
Software, algorithm | Imaris version 9.3.1 | RRID:SCR_003070 | Imaris, RRID: SCR_007370 | |
Software, algorithm | ImageJ | RRID:SCR_003070 | RRID:SCR_003070 https://imagej.net/ | |
Software, algorithm | ImageJ Coloc2 Plugin | Self-modified version as described by French et al., 2008. | Self-modified version of ImageJ; RRID:SCR_003070 | |
Software, algorithm | Fiji | RRID:SCR_003070 | Fiji (RRID:SCR_002285) |
Additional files
-
MDAR checklist
- https://cdn.elifesciences.org/articles/85167/elife-85167-mdarchecklist1-v3.pdf
-
Supplementary file 1
Excel file with the identified phosphopeptides of ARP3.
Phosphorylation of the ARP2/3 protein complex subunits by full-length ERK3 was detected by in vitro kinase assay and in-gel digestion of the respective ARP2/3 complex proteins followed by subsequent LC-MS analysis using a timsTOF Pro coupled to a nanoElute nanoflow liquid chromatography system. MS acquisition was performed in DDA-PASEF mode.
- https://cdn.elifesciences.org/articles/85167/elife-85167-supp1-v3.xlsx