Entorhinal Cortex: Use it or lose it
Alzheimer’s disease is a neurodegenerative disorder that affects approximately 55 million people globally (Gauthier et al., 2021). One serious symptom of the disease is a tendency to become disorientated both in space and in time. Researchers attribute this, in part, to impairments in the medial temporal lobe of the brain, which includes the hippocampus and the entorhinal cortex. These two regions are involved in the formation and retrieval of memories; in particular, they are important for making spatial memories for navigation, including memories of place, time, head direction and environmental borders (see for example review by Knierim, 2015).
To encode new memories and retrieve past ones, neurons in the network formed by the entorhinal cortex and the hippocampus have to maintain their plasticity – that is, their ability to form new connections between cells and remove ones which are rendered obsolete – throughout life. In mouse models of Alzheimer’s disease, however, the role of hippocampal and entorhinal cells in learning and memory is impaired (Rechnitz et al., 2021; Ying et al., 2022). As these regions are highly interconnected, early-stage corruption in the entorhinal region is further amplified downstream in the hippocampus. This leads to a vicious cycle that may impact behavior and cause spatial and temporal disorientation.
The specific mechanisms leading to a deterioration of the brain’s neural networks in Alzheimer’s disease are unclear. Similarly, it is unknown how this leads to memory loss. However, the impairment of neural networks within the entorhinal cortex often appears early in the progression of the disease (Stranahan and Mattson, 2010). Now, in eLife, Joanna Jankowsky and colleagues at Baylor College – including Rong Zhao, Stacy Grunke and Caleb Wood as joint first authors – report on how a neuronal population in layer II of the entorhinal cortex contributes to this impairment (Zhao et al., 2022). Their experiments show that blocking the activity of these neurons ultimately leads to increased cell death in this population.
Zhao et al. used a mouse model thought to undergo the same disruption to cell activity observed in Alzheimer’s disease. However, in this model, the impairment is not induced by the amyloid-beta plaques or neurofibrillary tangles characteristic of the disease. This is interesting because, even though it had previously been established that neurons die during Alzheimer’s disease, this was usually attributed to a vicious cycle of increased amyloid release driven by increased synchronization between cells causing hyperactivity (Busche and Konnerth, 2015; Zott et al., 2019). Instead, Zhao et al. show that, in their mouse model, cell deterioration and death in the entorhinal cortex are driven by silencing of specific neuronal activity.
Zhao et al. also demonstrate that, in their mouse model, a competitive process between active and inactive cells in the entorhinal cortex precedes neurodegeneration. This type of competition is similar to what is seen in infantile neuronal plasticity during development, when neural circuits are refined by selecting neurons and neural pathways depending on their levels of activity. Following this refinement process, which was previously thought to end soon after birth, some neurons degenerate while others persist. The extension of infantile neuronal plasticity in entorhinal cortex cells into adulthood may act as a double-edged sword: on the one hand, the plasticity allows these cells to form and modulate memories throughout life; on the other hand, the cells are more vulnerable to malfunction and death through competition.
Sensory information from multiple modalities (i.e. tactile, olfactory, auditory etc.) converges into layers II and III of the entorhinal cortex, which form the major input sources for two regions in the hippocampus, called the dentate gyrus and CA3. These areas of the brain are part of a pathway that ultimately terminates in another hippocampal region known as CA1 (Witter et al., 2000). It is therefore suggested that the cell impairment observed in the entorhinal cortex by Zhao et al. resembles an isolated deficit that can occur in Alzheimer’s disease. This impairment potentially forms an early seed to the later deterioration of neural networks and brain regions downstream, mainly in the hippocampus (Figure 1; Cacucci et al., 2008; Rechnitz et al., 2021; Roy et al., 2016). This may lead to the memory deficits and disorientation observed in Alzheimer’s patients.
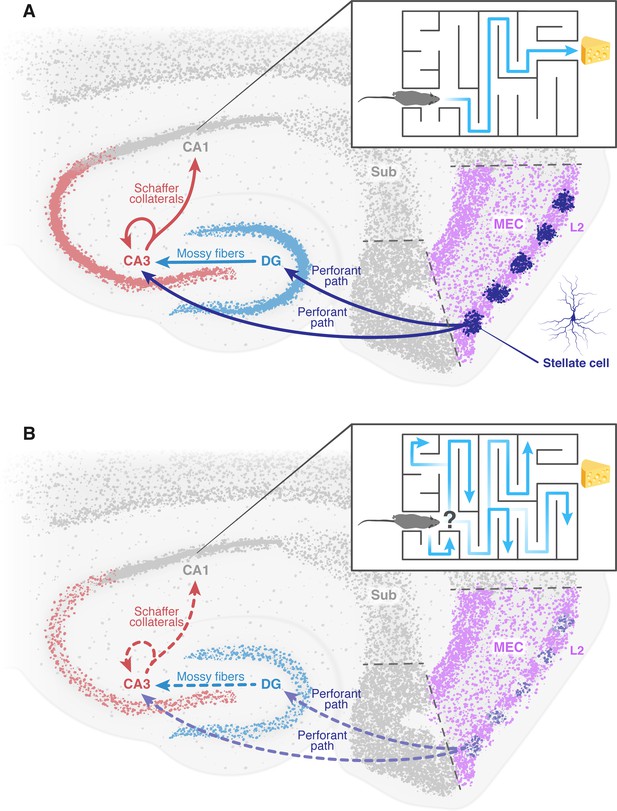
The deterioration of neural networks following impairment of the entorhinal cortex may drive disorientation in Alzheimer’s disease.
(A) In healthy mice, the tri-synaptic pathway begins in the entorhinal cortex (MEC, pink) and terminates in the CA1 region (grey) of the hippocampus. A prominent population of stellate cells (dark blue) can be found in layer 2 (L2, pink) of the entorhinal cortex. First, signals travel from layer 2 of the entorhinal cortex through the perforant path (dark blue arrow) to the dentate gyrus (DG, light blue) and the CA3 (red). Signals then travel from the dentate gyrus to the CA3 through the mossy fibers (light blue arrow). From the CA3, signals are transmitted through axons known as Schaffer collaterals (red arrows) to the CA1. This pathway is known to be essential for the formation of spatial memory and navigation. The inset shows how a healthy mouse, in which this pathway is working correctly, can navigate a maze. (B) In mice models of Alzheimer’s disease, the results of Zhao et al. suggest that the stellate cells in the entorhinal cortex degenerate and corrupt the information transmitted through the tri-synaptic pathway (dashed arrows), leading to the disruption of the neural network downstream, neuronal loss and ultimately disorientation. The inset shows how an Alzheimer’s disease model mouse is unable to navigate a maze. MEC: medial entorhinal cortex; Sub: subiculum.
Figure credit: Dafna Antes.
The findings of Zhao et al. hold promise for potential new treatment strategies targeting the cell population in layer II of the entorhinal cortex early on in the Alzheimer's disease. These interventions have the potential to slow down the cascade of events leading to the onset of the condition.
References
-
Anatomical organization of the parahippocampal-hippocampal networkAnnals of the New York Academy of Sciences 911:1–24.https://doi.org/10.1111/j.1749-6632.2000.tb06716.x
Article and author information
Author details
Publication history
Copyright
© 2023, Rechnitz and Derdikman
This article is distributed under the terms of the Creative Commons Attribution License, which permits unrestricted use and redistribution provided that the original author and source are credited.
Metrics
-
- 2,045
- views
-
- 140
- downloads
-
- 2
- citations
Views, downloads and citations are aggregated across all versions of this paper published by eLife.
Download links
Downloads (link to download the article as PDF)
Open citations (links to open the citations from this article in various online reference manager services)
Cite this article (links to download the citations from this article in formats compatible with various reference manager tools)
Further reading
-
- Developmental Biology
- Neuroscience
Williams syndrome (WS; OMIM#194050) is a rare disorder, which is caused by the microdeletion of one copy of 25–27 genes, and WS patients display diverse neuronal deficits. Although remarkable progresses have been achieved, the mechanisms for these distinct deficits are still largely unknown. Here, we have shown that neural progenitor cells (NPCs) in WS forebrain organoids display abnormal proliferation and differentiation capabilities, and synapse formation. Genes with altered expression are related to neuronal development and neurogenesis. Single cell RNA-seq (scRNA-seq) data analysis revealed 13 clusters in healthy control and WS organoids. WS organoids show an aberrant generation of excitatory neurons. Mechanistically, the expression of transthyretin (TTR) are remarkably decreased in WS forebrain organoids. We have found that GTF2IRD1 encoded by one WS associated gene GTF2IRD1 binds to TTR promoter regions and regulates the expression of TTR. In addition, exogenous TTR can activate ERK signaling and rescue neurogenic deficits of WS forebrain organoids. Gtf2ird1-deficient mice display similar neurodevelopmental deficits as observed in WS organoids. Collectively, our study reveals critical function of GTF2IRD1 in regulating neurodevelopment of WS forebrain organoids and mice through regulating TTR-ERK pathway.
-
- Cell Biology
- Neuroscience
The assembly and maintenance of neural circuits is crucial for proper brain function. Although the assembly of brain circuits has been extensively studied, much less is understood about the mechanisms controlling their maintenance as animals mature. In the olfactory system, the axons of olfactory sensory neurons (OSNs) expressing the same odor receptor converge into discrete synaptic structures of the olfactory bulb (OB) called glomeruli, forming a stereotypic odor map. The OB projection neurons, called mitral and tufted cells (M/Ts), have a single dendrite that branches into a single glomerulus, where they make synapses with OSNs. We used a genetic method to progressively eliminate the vast majority of M/T cells in early postnatal mice, and observed that the assembly of the OB bulb circuits proceeded normally. However, as the animals became adults the apical dendrite of remaining M/Ts grew multiple branches that innervated several glomeruli, and OSNs expressing single odor receptors projected their axons into multiple glomeruli, disrupting the olfactory sensory map. Moreover, ablating the M/Ts in adult animals also resulted in similar structural changes in the projections of remaining M/Ts and axons from OSNs. Interestingly, the ability of these mice to detect odors was relatively preserved despite only having 1–5% of projection neurons transmitting odorant information to the brain, and having highly disrupted circuits in the OB. These results indicate that a reduced number of projection neurons does not affect the normal assembly of the olfactory circuit, but induces structural instability of the olfactory circuitry of adult animals.