Energy coupling and stoichiometry of Zn2+/H+ antiport by the prokaryotic cation diffusion facilitator YiiP
Figures
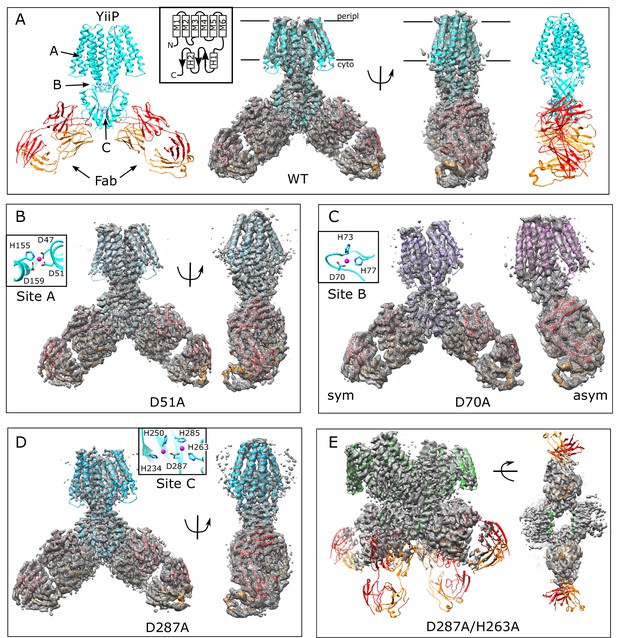
Overview of the cryo-EM structures.
Density maps and corresponding atomic models are shown for each mutant. Fab molecules are colored orange (light chain) and red (heavy chain) with YiiP colored in cyan, blue, purple and green, depending on the mutant. The homo-dimers adopt C2 symmetry for WT (A), D51A (B) and D287A (D) mutants, but a bend between TMD and CTD break this symmetry for D70A (C). The D287A/H263A mutant (E) forms a dimer of dimers in which the Fab molecules are rather disordered. Two conformations were observed for D70A, both of which are shown in panel C: D70A_sym on the left and D70A_asym on the right. Location Zn2+ binding sites, membrane boundaries as well as the topology of the YiiP protomer (inset) are shown in panel A; rectangles and arrows represent α-helices and β-sheets, respectively. Insets in panels B-D show the coordination geometry at the individual sites. Although the resolution was not always sufficient to uniquely define the side chain orientations, the maps are fully consistent with coordination geometry originally defined in the higher resolution X-ray structure (Lu et al., 2009).
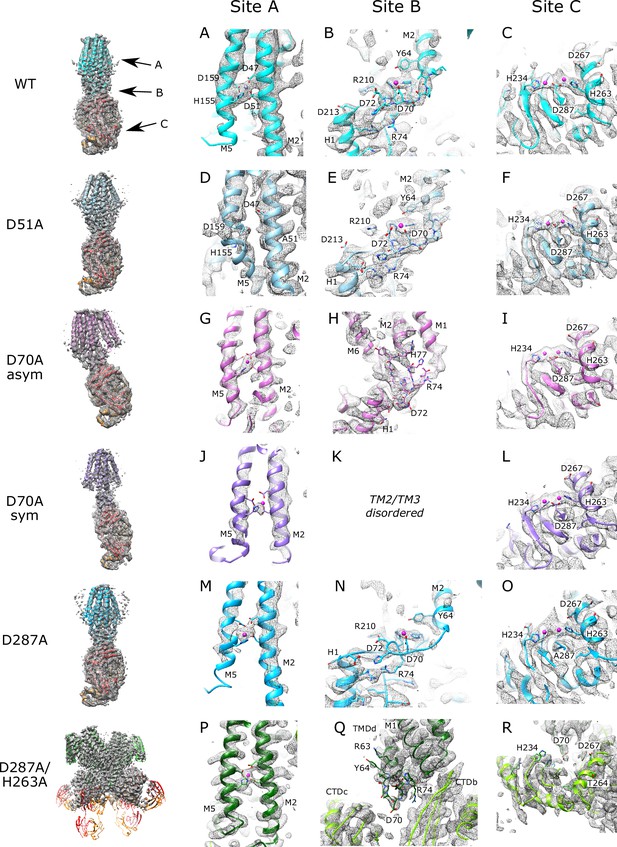
Density at Zn2+ sites in the cryo-EM maps.
An overview of each cryo-EM structure is shown on the left, followed by densities at sites A, B and C, respectively. Contour levels used to display the various maps were as follows. WT: 6 σ for site A, 7 σfor sites B and C, D51A: 5.1 σfor site A, 7 σfor sites B and C, D287A, 6.5 σ for sites A and C, 5.5 σ for site B, D70A_asym: 9 σ, D70A_sym: 9 σ, D287A/H263A: 10 σ. For clarity, side-chains are shown only for selected residues. In D70A_asym, the TM2/TM3 loop forms a Zn2+-free association with the CTD (H1 and C-terminus of M6) in one protomer (panel H) and is disordered in the other protomer (not shown). For D70A_sym, site B is disordered in both protomers and therefore not shown (panel K). In D287A/H263A, one of the TM2/TM3 loops associates with CTD’s from opposing protomers (CTDb, CTDc and TMDd refer to chains B, C and D in panel Q, respectively). The TM2/TM3 loops are also shown in Figure 2—figure supplement 1.
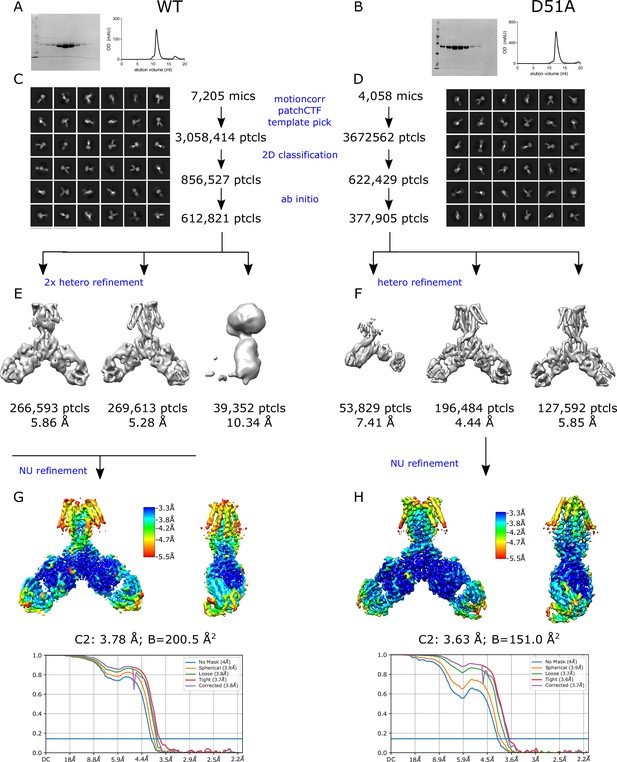
Determination of WT and D51A structures by cryo-EM.
(A,B) SDS-PAGE, left, and elution profile from SEC purification, right. Molecular weight standards are in the first lane at 116, 66, 45, 35, 25, 18.4, 14.4 kDa, with the YiiP monomer running as a single band at 32.5 kDa. (C,D) The cryo-EM workflow starts with correction of beam-induced motion and contrast transfer function (CTF) of movies, followed by template-based particle picking (box size of 350 Å). The particle set was subjected to two rounds of 2D classification and ab initio structure determinations to remove false positives. (E,F) Hetero-refinement jobs were used to look for multiple conformations and to maximize the homogeneity of particles for a final non-uniform refinement job, in this case with C2 symmetry. (G,H) The final structure was characterized by local resolution, represented by the colored surface shown from two orthogonal directions, and the gold-standard Fourier Shell Correlation comparing two independently refined particle sets.
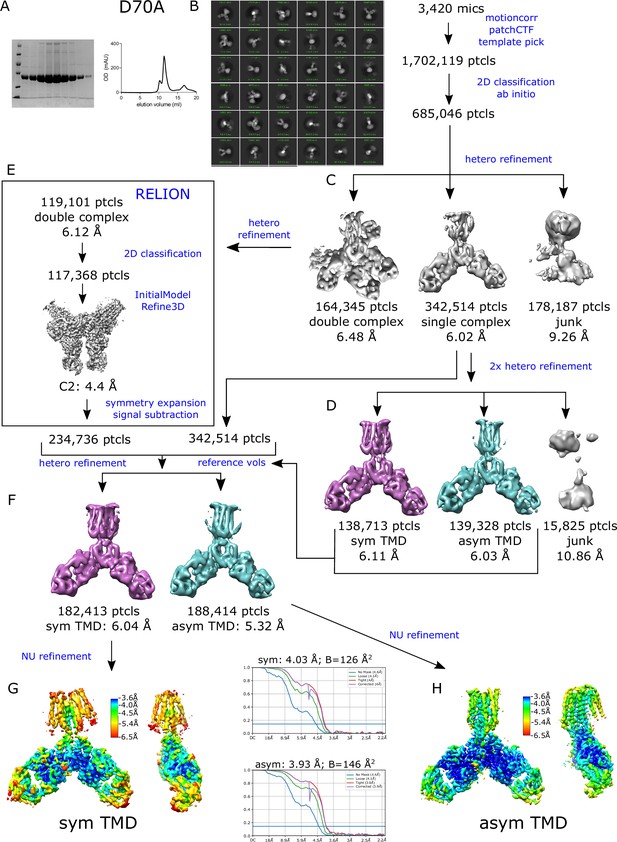
Determination of D70A structures by cryo-EM.
(A) SDS-PAGE and elution profile for SEC purification; molecular weight markers are at 116, 66, 45, 35, 25, 18.4, 14.4 kDa. The workflow for image processing was complex, involving multiple attempts to segregate distinct classes via ab initio and hetero-refinement jobs. This chart is a summary of the final, productive steps leading to structures presented. (B) Initial steps included correction of beam-induced motion and CTF followed by template-based particle picking (box size 350 Å), rounds of 2D classification and ab initio structure determination. (C) Hetero-refinement was used to segregate two major classes: class 1 comprising the expected dimer and class 2 comprising a dimer of dimers. The dimer of dimers involved interactions between Fab domains and thus did not appear to affect the conformations adopted by YiiP. (D) Particles from class 1 were subjected to further hetero-refinement to segregate into an additional two classes: one with symmetric TMD’s (purple) and the other with asymmetric TMD’s (blue) as described in the text. (E) Particles from class 2 (dimer of dimers) were exported to RELION where symmetry expansion and signal subtraction was used to generate a larger set comprising isolated dimers. (F) These symmetry-expanded particles were grouped together with class 1 particles for hetero-refinement using reference volumes with symmetric and asymmetric TMD’s as well as a junk collector (not shown). (G, H) The resulting final classes were then used for non-uniform refinement to produce D70A_sym and D70A_asym structures, each colored according to local resolution and shown from two orthogonal directions. The resolution was also characterized using the gold-standard Fourier Shell Correlation comparing two independently refined particle sets.

Determination of D287A and D287A/H263A structures by cryo-EM.
(A,B) SDS-PAGE and elution profiles from SEC purifications. Images come from a single gel with the molecular weight markers (116, 66, 45, 35, 25, 18.4, 14.4 kDa). Position and presence of the double peak is consistent with the higher order oligomerization seen during image analysis. (C,D) Corrections for motion and CTF were followed by template-based particle picking (box size of 350 Å), two rounds of 2D classification and ab initio structure determination. (E,F) Hetero-refinement was used to select a homogeneous set of particles for non-uniform refinement. (G,H) Final structures are characterized by the gold-standard FSC and by local resolution, illustrated by the plots and by surface coloring, respectively.
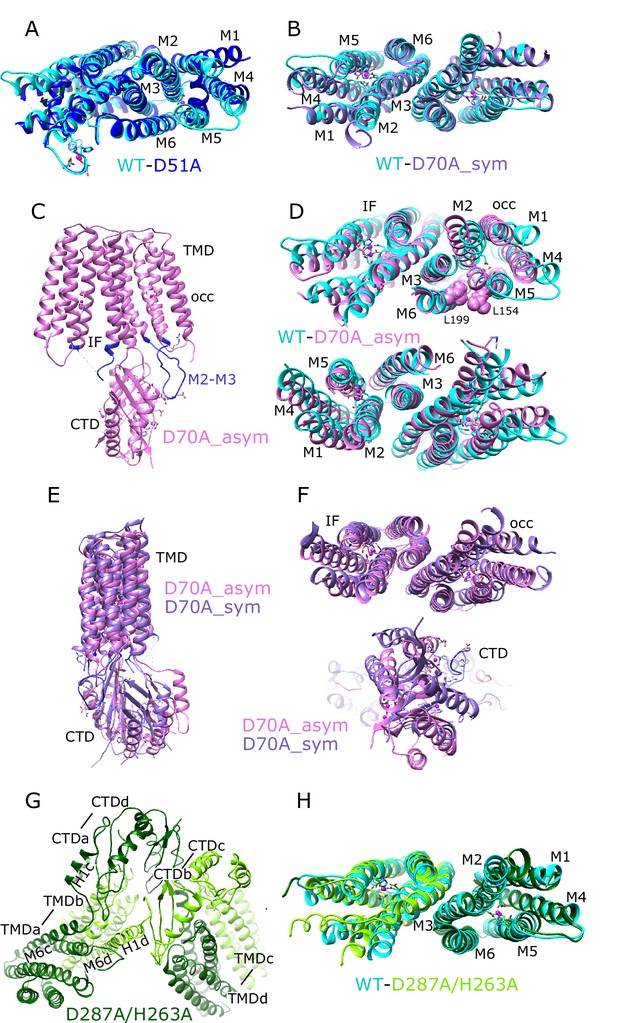
Comparisons of atomic models.
(A) Overlay of the TMD for WT (cyan) and D51A (blue) structures. This view is from the cytoplasmic side of the membrane, tilted slightly to show site A in protomer A. Core helices (M2, M3, M6) are well aligned, but there are substantial displacements of the peripheral helices (M1, M4, M5), presumably due to lack of Zn2+ binding at site A serving to bridge M2 and M5. (B) Overlay of the TMD for WT (cyan) and D70A_sym (purple) shows a very similar configuration of helices and an intact dimeric interface. This view is directly along the two-fold axis from the cytoplasmic side of the membrane. (C) Structure of D70A_asym viewed along the membrane plane showing the asymmetry between the two TMD’s. The protomer on the left (chain B) adopts an IF conformation, whereas the protomer on the right (chain A) adopts a novel occluded conformation that includes a reconfigured TM2/TM3 loop (blue) making a novel interaction with the CTD. (D) Overlay of the TMD for WT (cyan) and D70A_asym (plum) showing the occluded conformation adopted by protomer A (top) and the IF conformation adopted by protomer B (bottom). L199 and L154 (spheres) make van der Waals interactions that appear to stabilize the occluded conformation. These views are from the cytoplasmic side of the membrane, slightly tilted to show the respective protomers. (E–F) Overlays of D70A_asym (plum) and D70A_sym (purple) structures after alignment of core helices TM3 and TM6. Structures are viewed parallel to the membrane plane in E and along the two-fold axis from the cytoplasmic side of the membrane in F. A significant shift in the position of the CTD is apparent in E and bottom panel in F. Despite this shift and substantial conformational changes in the occluded protomer A, the dimer interface in the TMD (TM3 and TM6) is well aligned (F, top panel). (G) Domain swapped dimer of dimers adopted by the D287A/H263A construct. Dimerization of TMD’s involves interaction between one dark-green and one light-green molecule (e.g., TMDa and TMDb, where ‘a’ and ‘b’ refer to chain ID), whereas dimerization of CTD’s involves interaction between either two dark-green or two light-green molecules (e.g., CTDa and CTDd). The linker between M6 and the CTD adopts a long straight helix in chains b and d, but remains an unstructured loop in chains a and c. This view is from the cytoplasm looking toward the membrane surface. (H) Overlay of the TMD for WT (cyan) and D287A/H263A structures viewed along the dimer axis from the cytoplasmic side of the membrane shows a good match, indicating that disruption of site C affects mainly the configuration of the CTD.

Interactions between the TM2/TM3 loop and the CTD.
(A) In the WT structure, the TM2/TM3 loop (blue) contacts the TM6/CTD linker from the opposing protomer with proximity of D72 and R210. (B) In the D70A_asym structure, the Zn2+-free TM2/TM3 loop extends to interact with the H1 helix in the CTD of the opposing protomer. (C) In the D287A/H263A structure, the TM2/TM3 loop extends towards CTD’s of two different protomers (e.g., TM2/TM3 loop from chain B inserts between CTD’s of chains A and D). (D) In the other monomer of D287A/H263A, the TM2/TM3 loop interacts with the TM6/CTD linker of an opposing chain (e.g., the loop from chain A interacts with the linker from chain B). In these chains, the TM6/CTD linker has refolded into one, long continuous helix.
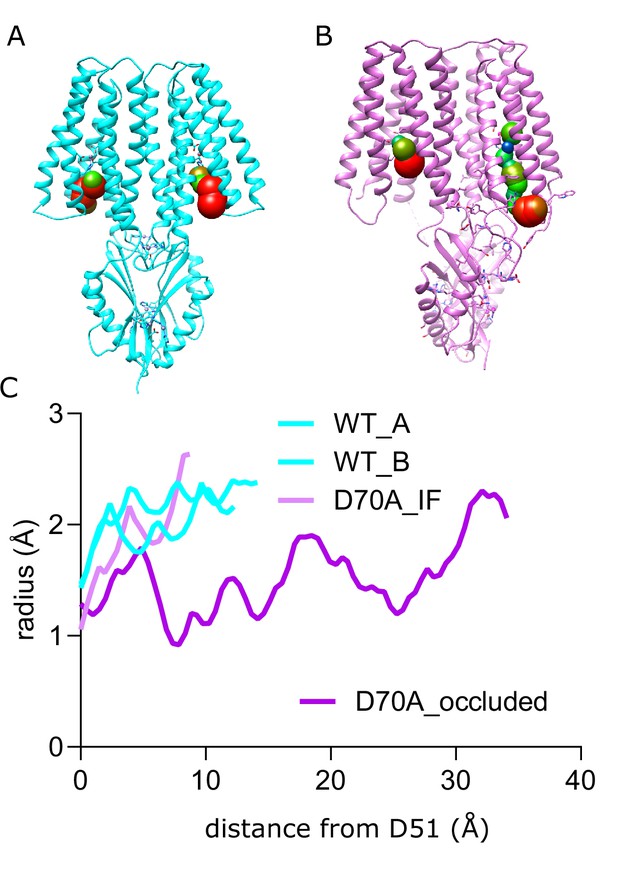
Water accessibility of site A.
Caver Analyst (Jurcik et al., 2018) was used to map cavities starting from Site A and leading towards the cytoplasm. (A) Model of WT YiiP with the cavity depicted a series of spheres, color coded according to the radius. (B) Model of D70A_asym shows an IF conformation on the left and a much constricted cavity in the occluded protomer on the right. (C) Radii are plotted along the contour of the respective cavities shown in panels A and B. This plot shows that the occluded protomer in D70A_asym falls well below the radius of 1.4 Å, typically taken as the limit for water accessibility.
-
Figure 2—figure supplement 2—source data 1
Source data plotted in panel C.
- https://cdn.elifesciences.org/articles/87167/elife-87167-fig2-figsupp2-data1-v1.xlsx
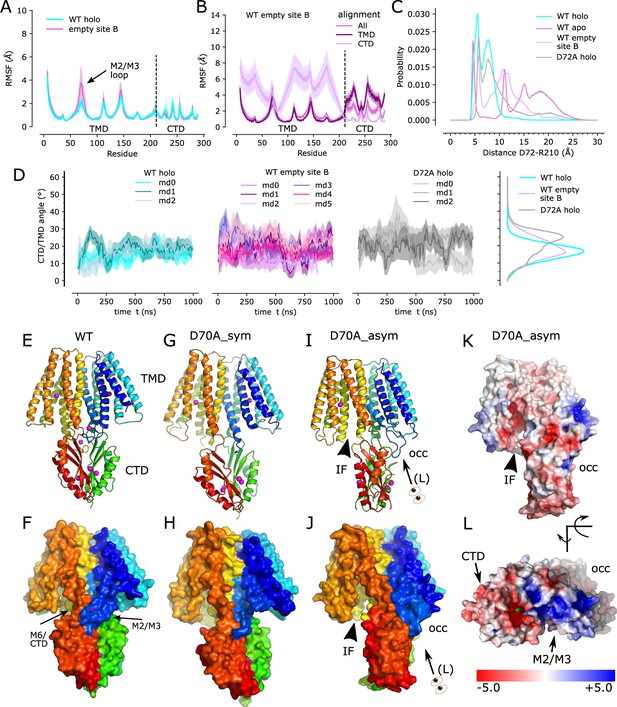
Zn2+ removal from site B in the TM2/TM3 loop.
(A) Per-residue RMSF of the WT, holo structure with Zn2+ present (cyan) and absent (purple) at site B demonstrates a notable increase in fluctuations in the TM2/TM3 loop. The dashed line indicates the boundary between TMD and CTD. (B) Per-residue RMSF for simulations with empty site B using three different alignment schemes: the entire molecule ("All"), transmembrane domain ("TMD"), or C-terminal domain ("CTD"). Analogous data for the WT, holo structure have previously been published (Figure 3i in Lopez-Redondo et al., 2021). (C) Distributions of distance between Cα atoms from Asp72 in one chain and Arg210 in the opposite chain during simulations of the WT, holo structure, WT apo structure with Zn2+ absent from all sites, WT structure with site B empty, and the D72A mutant with Zn2+ present at all three sites. The sharp peak at ~5 Å from the holo structure suggests a salt bridge that is less stable in the D72A mutant and disrupted when Zn2+ is absent from site B. (D) Angle between the TMD and CTD in simulations in the presence (cyan) and absence (purple) of Zn2+ at site B; the D72A mutant in the holo state is also shown on the right. The distribution of angles, on the far right, highlight greater mobility either when site B is empty or with the D72A mutation. (E,F) Structure of the WT, holo YiiP dimer showing global C2 symmetry about a vertical axis and juxtaposition of the TM2/3 loop (blue) with the TM6/CTD linker from the opposing protomer (orange-to-red). Rainbow colors progress from blue to red moving from the N-terminus of one protomer to the C-terminus of the other protomer. (G,H) D70A_sym structure shows a kink between TMD and CTD and disordering of the TM2/3 loop. Both protomers are in the IF state. (I,J) D70A_asym structure showing further twisting of the CTD and asymmetry of the TMD’s. The TM2/3 loop is disordered in the protomer on the left (chain B), but adopts a novel interaction with the CTD in the protomer on the right (chain A). (K,L). Electrostatic surface of D70A_asym showing a negatively charged cavity leading to site A on the left, but an occluded cavity with positive charge on the right. Note that L is at an oblique angle looking down on the M2-M3 loop. Profiles in panels A, B and D represent the average over both protomers and all three simulations, with the mean shown as a solid line and the error band indicating standard deviation.
-
Figure 3—source data 1
Source data for Figure 3 panel A.
RMSFs, and their standard deviations. TMD parts were calculated with alignment on TMD residue CA atoms, while CTD parts with alignment on CTD residue CA atoms.
- https://cdn.elifesciences.org/articles/87167/elife-87167-fig3-data1-v1.csv
-
Figure 3—source data 2
Source data for Figure 3 panel B.
RMSFs using three different alignment schemes, and their standard deviations.
- https://cdn.elifesciences.org/articles/87167/elife-87167-fig3-data2-v1.csv
-
Figure 3—source data 3
Source data for Figure 3 panel C.
The distributions of the distances between R210 and D72.
- https://cdn.elifesciences.org/articles/87167/elife-87167-fig3-data3-v1.csv
-
Figure 3—source data 4
Source data for Figure 3 panel D.
Angles between the TMD and CTD.
- https://cdn.elifesciences.org/articles/87167/elife-87167-fig3-data4-v1.csv
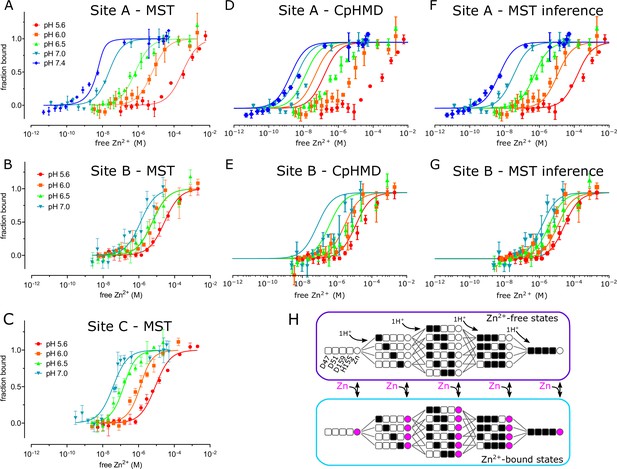
pH dependence of Zn2+ binding.
MST was used to experimentally measure Zn2+ binding affinity at each site (panels A, B and C). These data were combined with a thermodynamic model, represented in panel H, with parameters derived either from CpHMD (panels D and E) or MST inference (panels F and G). (A) For MST studies of site A, the D70A/D287A/H263A construct was used with Zn2+ buffered either with NTA (pH 7.0 and 7.4) or with citrate (pH 5.6, 6.0, and 6.5). Curves represent the law of mass action with Kd values listed in Table 2 together with their 95% confidence intervals. The relatively poor fit at pH 7.4 may reflect the fact that the Kd (1 nM) is lower than the minimum protein concentration (8 nM) supported by the assay, thus moving the system from the binding to the titration regime (Jarmoskaite et al., 2020). (B). For MST studies of site B, the D51A/D287A/H263A construct was used and Zn2+ was buffered with citrate. (C) For MST studies of site C, the D51A/D70A construct was used and Zn2+ was buffered with NTA at pH 7 or with citrate at the other pH’s. (D,E) Curves represent predictions of a thermodynamic model with pKa values for either site A residues (Asp47, Asp51, His153, Asp157) or site B residues (D70, H73, H77) taken from CpHMD simulations. These pKa values are listed in Table 4 and the distributions of protonation states are shown in Figure 4—figure supplements 5 and 6. Symbols represent experimental MST data as in A and illustrate a poor fit using these parameters. Thermodynamic modeling was not possible for site C because of instability of the CTD in CpHMD simulations in the absence of Zn2+. (F,G). Curves represent predictions of the MST inference algorithm with corresponding pKa values listed in Table 4. Again, symbols represent experimental MST data and show the excellent fit using these refined parameters. (H) Schematic representation of the microscopic thermodynamic model for site A. Titratable residues (D47, D51, D159, H155) are represented either as black squares for the protonated state, or white squares for the deprotonated state. Binding of one Zn2+ ion to the site is indicated by a filled magenta circle. Transitions are only possible between states connected by lines (protonation/deprotonation) or corresponding states connected by double-sided arrows (Zn2+ binding/release). An analogous model for site B comprised D70, H73 and H77 (not depicted). MST data was collected in triplicate from three independent titrations. Protein used for these titrations represent a single biological replicate, although qualitatively similar results were obtained from three to five independent protein preparations derived from at least two bacterial cultures.
-
Figure 4—source data 1
Source data and fitting for the plots in of site A in panel A.
- https://cdn.elifesciences.org/articles/87167/elife-87167-fig4-data1-v1.xlsx
-
Figure 4—source data 2
Source data and fitting for the plots in of site B in panel B.
- https://cdn.elifesciences.org/articles/87167/elife-87167-fig4-data2-v1.xlsx
-
Figure 4—source data 3
Source data and fitting for the plots in of site C in panel C.
- https://cdn.elifesciences.org/articles/87167/elife-87167-fig4-data3-v1.xlsx

Measuring Zn2+ affinity by MST.
(A–D) SEC elution profiles for YiiP constructs. WT protein is included for comparison but was not analyzed by MST. Elution volumes of the peak are indicated on each plot, which are almost identical for all constructs. (E–H) Raw data from MST for the titrations at pH 7. The blue rectangles correspond to the time window used for initial fluorescence levels and the red rectangles correspond to the time window used for the thermophoresis rates. Each plot includes 48 traces that represent data from 16 ligand concentrations used for the titration, each of which were measured in triplicate. Titrations were done with Zn2+ in panels E, F, and G, whereas Mg2+ was used as a control for panel H. (I) SEC profiles of the D287A mutant in the presence and absence of Fab. The shift in position of the main peak in the presence of Fab is consistent with a complex between the homodimer and two Fab’s and the secondary peak at 9.9 ml is consistent with the dimer of dimers (4 YiiP +4 Fab) seen during cryo-EM processing (Figure 1—figure supplement 4). (J) SEC profiles of the D287A/H263A mutant in the presence and absence of Fab. Although the position of the peak in the absence of Fab is consistent with the YiiP homodimer seen with other mutants, the position of the main peak in the presence of Fab is consistent with a dimer of dimers and the secondary peak with even higher order oligomers.
-
Figure 4—figure supplement 1—source data 1
Source data plotted in panels A-D.
- https://cdn.elifesciences.org/articles/87167/elife-87167-fig4-figsupp1-data1-v1.xlsx
-
Figure 4—figure supplement 1—source data 2
Source data for the MST titrations of Site A with Zn shown in panel E.
- https://cdn.elifesciences.org/articles/87167/elife-87167-fig4-figsupp1-data2-v1.xlsx
-
Figure 4—figure supplement 1—source data 3
Source data for the MST titrations of Site B with Zn shown in panel F.
- https://cdn.elifesciences.org/articles/87167/elife-87167-fig4-figsupp1-data3-v1.xlsx
-
Figure 4—figure supplement 1—source data 4
Source data for the MST titrations of Site C with Zn shown in panel G.
- https://cdn.elifesciences.org/articles/87167/elife-87167-fig4-figsupp1-data4-v1.xlsx
-
Figure 4—figure supplement 1—source data 5
Source data for the MST titrations of Site A with Mg shown in panel H.
- https://cdn.elifesciences.org/articles/87167/elife-87167-fig4-figsupp1-data5-v1.xlsx
-
Figure 4—figure supplement 1—source data 6
Source data plotted in panels I-J.
- https://cdn.elifesciences.org/articles/87167/elife-87167-fig4-figsupp1-data6-v1.xlsx

Walk of replica simulations in CpHMD pH-replica exchange (REX) through pH space.
Each panel shows how one replica simulation (Rep:0 to Rep:29) changes its current pH state over the course of the simulation (Frame number), as indicated by the ‘pH replica’ on the ordinate. The pH replicas range from pH 1.5 (pH replica 0)–11.5 (pH replica 29). Multiple replicas sample most of the pH range and many move across the especially relevant range between pH replica 9 (pH 4) and pH replica 22 (pH 9), indicating that the REX procedure samples pH space well.
-
Figure 4—figure supplement 2—source data 1
Source data documenting the state transitions of each replica simulation.
- https://cdn.elifesciences.org/articles/87167/elife-87167-fig4-figsupp2-data1-v1.csv
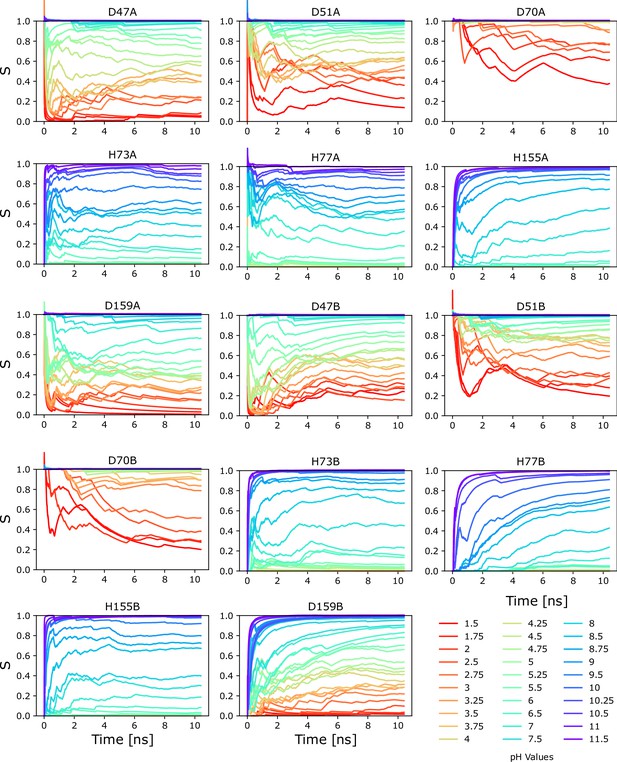
Convergence of the deprotonated fraction for titratable residues in CpHMD simulations.
Residues in site A (D47, D51, H155, D159) and site B (D70, H73, H77) from both protomers (A and B) are shown. The instantaneous deprotonated fraction S is plotted as a function of simulation time and pH value, sampled across all replicas in the replica exchange simulation. The deprotonated fractions generally converge to a stable value after about 8 ns with similar behavior in protomers A and B, thus indicating sufficient sampling during the CpHMD simulations.
-
Figure 4—figure supplement 3—source data 1
Source data for changes of the proton-bound fractions of specified residues at different pH over the course of the simulation.
Data for individual residues - D47A, D51A, D70A, H73A, H77A, H155A, D159A, D47B, D51B, D70B, H73B, H77B, H155B, and D159B - are listed in the respective tabs.
- https://cdn.elifesciences.org/articles/87167/elife-87167-fig4-figsupp3-data1-v1.xlsx

Titration curves for titratable residues in CpHMD simulations.
Residues in site A (D47, D51, H155, D159) and site B (D70, H73, H77) are shown from both protomers (A and B). The unprotonated fraction S from the end of the CpHMD simulations is shown as a function of pH (black points). The Hill equation (generalized Henderson-Hasselbalch equation) is fitted to the data (black line). The estimated pKa is indicated as a red line at S=0.5. As described in the text, H73/H77 were considered to be coupled and were analyzed in aggregate; the two pKas for the combined system (H73AH77A and H73BH77B) are represented by the pair of red lines in the last two panels.
-
Figure 4—figure supplement 4—source data 1
Source data for titration curves of the residues in binding sites A and B.
- https://cdn.elifesciences.org/articles/87167/elife-87167-fig4-figsupp4-data1-v1.csv
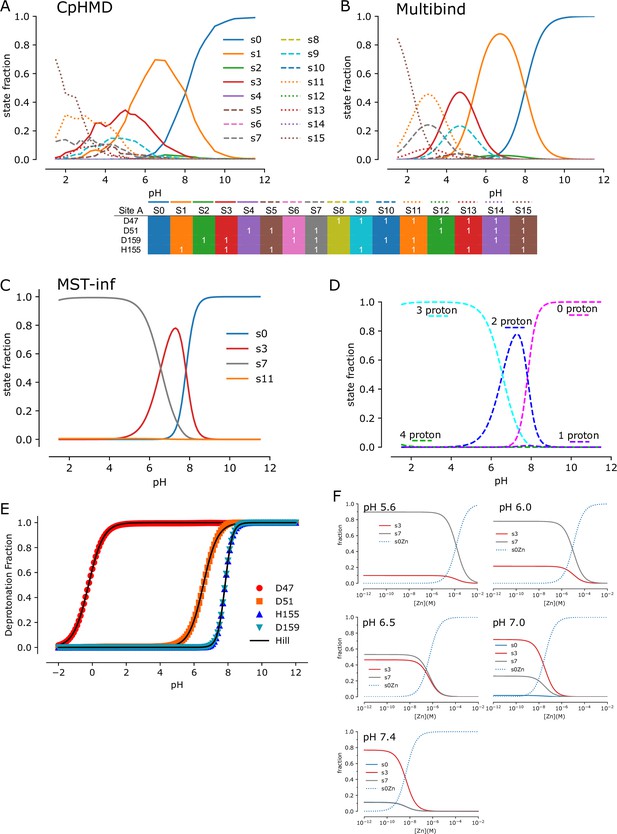
Site A Protonation and Zn2+-binding states by CpHMD and MST inference.
(A). Populations of protonated states obtained directly from CpHMD simulations for site A, in the absence of Zn2+. Data for protomer A and protomer B were combined. Color coded definitions of individual states are shown below with a ‘1’ indicating the protonated state and absence of a number the unprotonated state of each corresponding residue. (B) Populations of protonated states from an inverse Multibind model based on inferring microscopic pKa values directly from the CpHMD populations in (A). (C) Population of protonated states resulting from MST inference, which relies on the MC method to refine microscopic model parameters based on experimental MST data. Populations represent the Zn2+-free states, although the model incorporates the whole range of Zn2+ bound states [see (F)]. Only states S0, S3, S7, S11 have appreciable occupancy (>0.0001 maximum probability), although all states contribute to aggregated state probabilities in D and E. (D) MST inference results represented as populations of aggregated states defined by the total number of protons bound in the absence of Zn2+. (E) Deprotonated fraction of each site A residue as a function of pH, averaged over all possible states of the MST inference model in the absence of Zn2+. Data points (symbols) were generated from the model; then the Hill-Langmuir equation was fit to the generated data to obtain an effective per-residue pKa (listed in Table 4). (F) Population of states derived from the MST inference model as a function of Zn2+ concentration at various pH values. S0Zn (dotted line) denotes the fully deprotonated, Zn2+-bound state, which is the only populated Zn2+-bound state predicted by the MST inference.
-
Figure 4—figure supplement 5—source data 1
Source data for values plotted in panel A.
- https://cdn.elifesciences.org/articles/87167/elife-87167-fig4-figsupp5-data1-v1.csv
-
Figure 4—figure supplement 5—source data 2
Source data listing pKa values deduced from CpHMD simulations.
- https://cdn.elifesciences.org/articles/87167/elife-87167-fig4-figsupp5-data2-v1.csv
-
Figure 4—figure supplement 5—source data 3
Source data listing pKa and Kd values deduced from MST-inference method.
Panels B-F were derived from these data using Multibind package available at https://github.com/Becksteinlab/yiip_analysis, (Fan, 2023).
- https://cdn.elifesciences.org/articles/87167/elife-87167-fig4-figsupp5-data3-v1.csv

Site B Protonation and Zn2+-binding states by CpHMD and MST inference.
(A). Populations of protonated states obtained directly from CpHMD simulations for site B, in the absence of Zn2+. Data for protomer A and protomer B were combined. Color coded definitions of individual states are shown below with a ‘1’ indicating the protonated state and absence of a number the unprotonated state of each corresponding residue. (B) Populations of protonated states from an inverse Multibind model based on inferring microscopic pKa values directly from the CpHMD populations in (A). (C) Population of protonated states resulting from MST inference. For this analysis, a symmetry restraint was imposed on H73 and H77 as described in the text. Populations are shown in the absence of Zn2+ , although the model incorporates the whole range of Zn2+ concentrations [see (G)]. (D) MST inference results represented as populations of aggregated states defined by the total number of protons bound in the absence of Zn2+. (E) Deprotonated fraction of each site B residue as a function of pH, averaged over all possible states of the MST inference model in the absence of Zn2+. Data points were generated from the model and the Hill-Langmuir equation was fit to D70 data (solid black line) to obtain an effective per-residue pKa; data for H73 and H77 are not correctly modelled by a Hill fit (not plotted). (F) H73 and H77 were treated as a coupled system and the total deprotonated fraction as a function of pH was fit with the ‘coupled titration model’ (solid black line), resulting in two effective pKa values. (G) Population of states from the MST inference mode as a function of Zn2+ concentration at various pH values. Unlike site A, multiple Zn2+-bound states (S0Zn, S1Zn, S2Zn, S3Zn) are populated at lower pH values.
-
Figure 4—figure supplement 6—source data 1
Source data for values plotted in panel A.
- https://cdn.elifesciences.org/articles/87167/elife-87167-fig4-figsupp6-data1-v1.csv
-
Figure 4—figure supplement 6—source data 2
Source data listing pKa values deduced from CpHMD simulations.
- https://cdn.elifesciences.org/articles/87167/elife-87167-fig4-figsupp6-data2-v1.csv
-
Figure 4—figure supplement 6—source data 3
Source data listing pKa and Kd values deduced from MST-inference method.
Panels B-G were derived from these data using Multibind package available at https://github.com/Becksteinlab/yiip_analysis, (Fan, 2023).
- https://cdn.elifesciences.org/articles/87167/elife-87167-fig4-figsupp6-data3-v1.csv
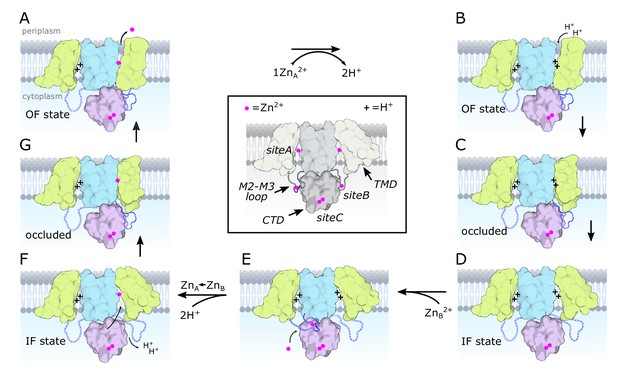
Transport cycle for YiiP.
According to the alternating access paradigm, YiiP toggles between the OF and IF states via an intermediate occluded state. We assume that these changes occur independently in each protomer based on asymmetry seen in structures of soYiiP and Znt8 (Xue et al., 2020). Thus, our model depicts changes in the right-hand protomer while the left-hand protomer remains in a resting IF state. Although these intermediate states are informed by current and past structural work, they do not precisely conform to solved structures but represent hypothetical states that we believe to exist under physiological conditions. (A) Zn2+ is released to the periplasm. The TM2/TM3 loop is depicted interacting with the CTD in a Zn2+-free, extended conformation, as seen in our D70A_asym structure. (B) The release of Zn2+ is promoted by the low pH of the periplasm and results in protonation of two residues in site A, or potentially three residues at lower pH. (C–D) The protonated form transitions to the IF state via an occluded state. In the IF state, the Zn2+-free TM2/TM3 loop is released by the CTD and becomes disordered. (E) Zn2+ is recruited to site B, inducing an ordered conformation of the TM2/TM3 loop that folds onto the CTD enabling interaction of Asp72 and Arg210. (F) Zn2+ is transferred from a relatively low affinity site B to the much higher affinity site A via a negatively charged access channel, thus displacing two protons. (G) This transfer induces a Zn2+-bound, occluded conformation in which the CTD tilts toward the occluded protomer and interacts with the TM2/TM3 loop in its Zn2+-free, extended conformation. Features of the model are illustrated in the middle, boxed panel with desaturated colors. Zn2+ ions are depicted as magenta spheres and protons with a ‘+’. The CTD is pink with two Zn2+ ions constitutively bound at site C. The scaffolding membrane helices (TM3 and TM6) are blue and the transport domain (TM1,2,4,5) is yellow. The TM2/TM3 loop is blue and depicted with dashed lines in the disordered state. Created with biorender.com.
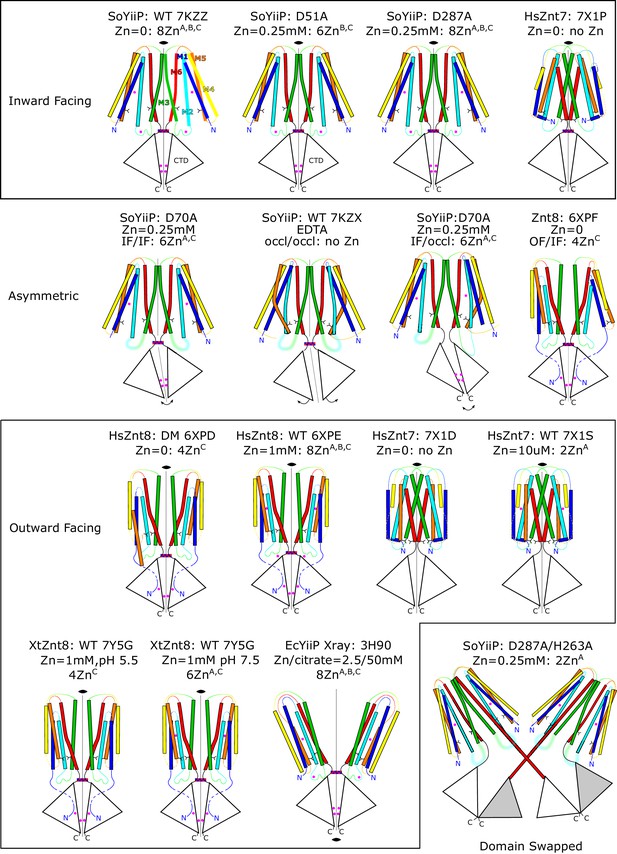
Summary of structures from CDF transporters.
Cartoon representations of most structures so far determined. The structures have been grouped according to conformational state: symmetric IF, symmetric OF and asymmetric states. PDB accession codes are indicated for previously published structures together with concentrations of Zn2+ present in the solution and the number of ions observed in the structures. Accession codes for current work are listed in Table 1. Although the sites been named differently for Znt7 and Znt8, the A,B,C nomenclature has been used to simplify the comparison. The helices in the TMD are shown in rainbow colors starting with blue for TM1 and red for TM6. CTD’s are represented as a triangle. Zn2+ ions as magenta spheres. Hydrophobic residues forming the so-called hydrophobic gate (Leu154 and Leu199 in SoYiiP) are shown as sticks. Disordered in TM2/TM3 loops is indicated by a blurred line.
Tables
Structure determination of YiiP mutants.
Data set | WT | Site AD51A | Site B*D70A asymTMD | Site B*D70A symTMD | Site CD287A | Site C2D287A/H263A | |
---|---|---|---|---|---|---|---|
Deposition PDB EMDB | 8F6E EMD-28881 | 8F6F EMD-28882 | 8F6H EMD-28883 | 8F6I EMD-28884 | 8F6J EMD-28885 | 8F6K EMD-28886 | |
Data collection and processing | |||||||
Magnification | 81,000 | 81,000 | 81,000 | 81,000 | 81,000 | 81,000 | |
Voltage (kV) | 300 | 300 | 300 | 300 | 300 | 300 | |
Electron exposure (e–/Å2) | 50 | 50 | 50 | 50 | 50 | 50 | |
Defocus range (μm) | 1.0–3.0 | 0.75–2.75 | 0.7–3.0 | 0.7–3.0 | 0.7–3.0 | 0.7–2.5 | |
Pixel size (Å) | 1.068 | 1.079 | 1.079 | 1.079 | 1.079 | 1.079 | |
Symmetry imposed | C2 | C2 | C1 | C1 | C2 | C2 | |
Initial particle images (no.) | 3,058,414 | 3,672,562 | 1,702,119 | 1,702,119 | 1,664,097 | 2,982,749 | |
Final particle images (no.) | 536,206 | 196,484 | 188,414 | 182,413 | 252,599 | 300,844 | |
Map resolution FSC threshold (Å) B factor (Å2) Resolution range (Å) | 3.78 0.143 200.5 3.0–5.5 | 3.63 0.143 151.0 3.0–5.2 | 3.93 0.143 146.1 3.4–5.8 | 4.03 0.143 126.0 3.4–6.9 | 3.68 0.143 150.9 3.0–5.5 | 3.46 0.143 135.2 3.0–5.2 | |
Model Refinement | |||||||
Model composition Non-hydrogen atoms Protein residues Ligands | 10478 1366 8 | 10348 1344 6 | 10421 1358 6 | 10377 1351 6 | 10472 1366 8 | 8714 1132 4 | |
RMS deviations Bond lengths (Å) Bond angles (°) | 0.003 0.557 | 0.003 0.535 | 0.002 0.509 | 0.002 0.517 | 0.003 0.536 | 0.002 0.499 | |
Validation MolProbity score Clashscore Rotamer outliers (%) CaBLAM outliers (%) Rama-Z score | 1.70 5.44 0.00 2.66 0.77 | 1.68 6.31 0.09 3.05 0.37 | 1.72 5.78 0.00 2.60 0.05 | 1.75 7.11 0.00 3.40 0.20 | 1.77 6.37 0.00 3.64 0.50 | 1.96 10.28 0.00 4.21 0.45 | |
Ramachandran plot Favored (%) Allowed (%) Disallowed (%) | 94.19 5.44 0.37 | 95.27 4.57 0.15 | 93.99 5.86 0.15 | 94.86 4.99 0.15 | 93.59 6.04 0.37 | 93.51 5.96 0.53 | |
Model vs. Data CC (mask) | 0.75 | 0.83 | 0.79 | 0.80 | 0.81 | 0.73 |
-
*
Both D70A structures arose from the same set of micrographs and initial particle picks. 2 Fab molecules were not included for the refinement of D287A/H263A due to poor density in this region of the map.
Binding affinity for individual Zn2+ sites measured by MST* or deduced by the MST inference algorithm†.
site A D70A/ D287A/H263A (μM) | site A/C D70A/D287A (μM) | site B D51A/ D287A/H263A (μM) | site C D51A/D70A (μM) | site C1 D51A/ D70A/H263A (μM) | site C2 D51A/ D70A/H234A (μM) | |
---|---|---|---|---|---|---|
pH 5.6 | 302±107 (149) | 27.1±2.8 (19.5) | 8.10±1.55 | |||
pH 6.0 | 6.11±1.32 (10.8) | 6.30±1.94 | 5.54±2.0 (5.38) | 0.692±0.28 | ||
pH 6.5 | 0.654±0.173 (0.503) | 5.73±1.75 (1.36) | 0.088±0.032 | |||
pH 7.0 | 0.0163±0.0041 (0.0325) | 0.0012±0.0018 | 1.18±0.6 (0.503) | 0.033±0.0087 | 0.153±0.048 | 0.223±0.039 |
pH 7.4 | 0.001±0.001 (0.0048) |
-
*
Values correspond to Kd as determined by applying the law of mass action to the data together with their 68% confidence intervals.
-
†
Values deduced by MST inference are shown in parentheses.
Binding affinity and Hill coefficients derived from MST data*.
site A D70A/ D287A/H263A (μM) | site B D51A /D287A/H263A (μM) | site C D51A/D70A (μM) | site C1 D51A/ D70A/H263A (μM) | site C2 D51A /D70A/H234A (μM) | |
---|---|---|---|---|---|
pH 5.6 | 1490±2,460 n=0.46 | 28.4±3.54 n=0.94 | 7.50±2.20 n=0.9 | ||
pH 6.0 | 6.34±1.67 n=0.81 | 5.68±1.57 n=1.60 | 1.39±0.382 n=0.78 | ||
pH 6.5 | 0.622±0.198 n=0.54 | 27.9±34.3 n=0.38 | 0.133±0.0359 n=0.66 | ||
pH 7.0 | 0.0247±0.0057 n=1.0 | 4.23±13.0 n=0.45 | 0.0486±0.0038 n=2.9 | 0.116±0.0119 n=2.96 | 0.212±0.0328 n=1.2 |
pH 7.4 | 0.0039±0.0009 n=0.56 |
-
*
Values correspond to EC50 and the Hill coefficient (n) as determined by fitting the Hill equation to the data together with their 68% confidence intervals.
pKa values of residues determined by CpHMD simulations (Hill-Langmuir) and MST inference.
CpHMD (Hill-Langmuir)* | MST inference† | ||
---|---|---|---|
Site A | Asp47 | 3.84±0.18 | 0.20±0.51 |
Asp51 | 2.92±0.12 | 6.51±0.21 | |
His155 | 7.97±0.15 | 7.82±0.15 | |
Asp159 | 4.87±0.18 | 7.83±0.15 | |
Site B | Asp70 | 2.06±0.46 | 1.12±0.57 |
His73 | 8.08±0.46 | 12.37±0.61‡ | |
His77 | 8.12±0.13 | 5.26±0.47‡ |
-
*
For CpHMD(Hill-Langmuir), CpHMD data for protomer A and B were collected and analyzed independently. The value is the mean over the protomer A and B data and the error estimate is the average of the absolute deviations from the mean.
-
†
For MST inference, error estimates were obtained from 50 independent replicates of the MC sampling process. The value is the mean over the 50 replicates and shown with the standard deviation.
-
‡
Titration curves for His73 and His77 from the MST inference model cannot be fit individually with a simple Hill equation (c.f. Supp. Figs. 11e & f). We therefore consider these two residues as a coupled system with two effective pKa values as shown.
Reagent type (species) or resource | Designation | Source or reference | Identifiers | Additional information |
---|---|---|---|---|
Gene (Shewanella oneidensis) | fieF, SO_4475, | This study | Q8E919 | See Coudray et al., 2013 |
Strain, strain background (Escherichia coli) | BL21-CodonPlus (DE3)-RIPL | Agilent | Part Number: 230280 | |
Strain, strain background (Escherichia coli) | strain 55244 | ATCC | 27C7 | |
Recombinant DNA reagent | pET-YiiP (plasmid) | This study, Lopez-Redondo et al., 2021 | See Coudray et al., 2013 | |
Recombinant DNA reagent | pET_Fab2r (plasmid) | Lopez-Redondo et al., 2021 | ||
Sequence-based reagent | D51A_F | This study | PCR primer | GATTCTTTTGCCGCTACGCTCGCCTCG |
Sequence-based reagent | D51A_R | This study | PCR primer | CGAGGCGAGCGTAGCGGCAAAAGAATC |
Sequence-based reagent | D70A_F | This study | PCR primer | GTCCCTGCTGCTCATGACCACAGATACGGCC |
Sequence-based reagent | D70A_R | This study | PCR primer | GTGGTCATGAGCAGCAGGGACAATGGCATAAC |
Sequence-based reagent | D287A_F | This study | PCR primer | GATTATTCACCAAGCTCCCGTGCAAG |
Sequence-based reagent | D287A_R | This study | PCR primer | CTTGCACGGGAGCTTGGTGAATAATC |
Sequence-based reagent | H263A_F | This study | PCR primer | CGAAGCCGCTAGCATTACCGATACAACAGGGC |
Sequence-based reagent | H263A_R | This study | PCR primer | CGAAGCCGCTAGCATTACCGATACAACAGGGC |
Peptide, recombinant protein | Fab2R | This study, Lopez-Redondo et al., 2021 | See Methods and materials | |
Peptide, recombinant protein | Tobacco Etch Virus (TEV) protease. | This study | Previously synthesized recombinantly in E. coli cells. | |
Chemical compound, drug | N,N,N’,N’-tetrakis(2-pyridinylmethyl)–1,2-ethanediamine [TPEN] | Sigma-Aldrich | CAS number: 16858-02-9 | |
Chemical compound, drug | Alexa Fluor 488 NHS Ester (Succinimidyl Ester) | Invitrogen Life Technologies | Catalog number: A20000 | |
Chemical compound, drug | n-Decyl-β-D-Maltopyranoside | anatrace | D322LA | |
Software, algorithm | cryoSPARC | Structura Biotechnology | RRID:SCR_016501 | |
Software, algorithm | RELION | Scheres, 2012 | https://www3.mrc-lmb.cam.ac.uk/relion/index.php/Main_Page | |
Software, algorithm | Chimera | Pettersen et al., 2004 | RRID:SCR_004097 | |
Software, algorithm | PyMOL | Schrodinger | RRID:SCR_000305 | |
Software, algorithm | PHENIX | Adams et al., 2010 | RRID:SCR_014224 | |
Software, algorithm | COOT | Emsley et al., 2010; | RRID:SCR_014222 | |
Software, algorithm | PRISM | GraphPad | RRID:SCR_002798 | |
Software, algorithm | CAVER | https://caver.cz/ | ||
Software, algorithm | NAMDINMATOR | Kidmose et al., 2019 | https://namdinator.au.dk/ | |
Software, algorithm | MAXCHELTOR | Bers et al., 1994 | https://somapp.ucdmc.ucdavis.edu/pharmacology/bers/maxchelator/webmaxc/webmaxcS.htm | |
Software, algorithm | MO.Affinity Analysis software v2.3 | Nanotemper | ||
Software, algorithm | CHARMM c42a2 with PHMD | Brooks et al., 2009; Khandogin and Brooks, 2005 | https://charmm-gui.org | |
Software, algorithm | CpHMD-Analysis | Huang et al., 2021 Wallace and Shen, 2011 | https://github.com/Hendejac/CpHMD-Analysis | |
Software, algorithm | CHARMM-GUI | Jo et al., 2008; Jo et al., 2009; Lee et al., 2016 | ||
Software, algorithm | GROMACS 2021.1 | Abraham et al., 2015 | ||
Software, algorithm | Multibind | Kenney and Beckstein, 2023 | https://github.com/Becksteinlab/multibind; Beckstein and Kenney, 2023 | |
Others | Monolith standard treated Capillaries | Nanotemper | K022 | https://shop.nanotempertech.com/en/monolith-capillaries-1000-count-17 |
Others | C-Flat 1.2/1.3-4Cu-50 grids | Protochips, Inc | https://www.emsdiasum.com/c-flat-family-of-holey-carbon-gold-grids-for-stem-and-cryo-tem |
MD simulations.
Simulation | Length | N of replicas | Total simulation time | Software |
---|---|---|---|---|
Fixed charge apo* | 1000 ns | 3 | 3000 ns | GROMACS |
Fixed charge holo* | 1000 ns | 3 | 3000 ns | GROMACS |
Fixed charge empty site B | 1000 ns | 6 | 6000 ns | GROMACS |
Fixed charge D72A | 1000 ns | 3 | 3000 ns | GROMACS |
CpHMD apo | 12 ns | 30 (pHREX) | 360 ns | CHARMM |
-
*
Fixed charge” simulations were repeated three times. The CpHMD simulations were performed as coupled pH-replica exchange simulations. *Simulations for apo and holo YiiP were taken from our previous work (Lopez-Redondo et al., 2021).
MST convergence criteria.
Target | Binding site | RMSD cutoff |
---|---|---|
CpHMD microstate probability distributions | Site A | 0.0382 |
CpHMD microstate probability distributions | Site B | 0.0447 |
MST bound fraction | Site A | 0.0760 |
MST bound fraction | Site B | 0.0995 |