The negative adipogenesis regulator Dlk1 is transcriptionally regulated by Ifrd1 (TIS7) and translationally by its orthologue Ifrd2 (SKMc15)
Figures
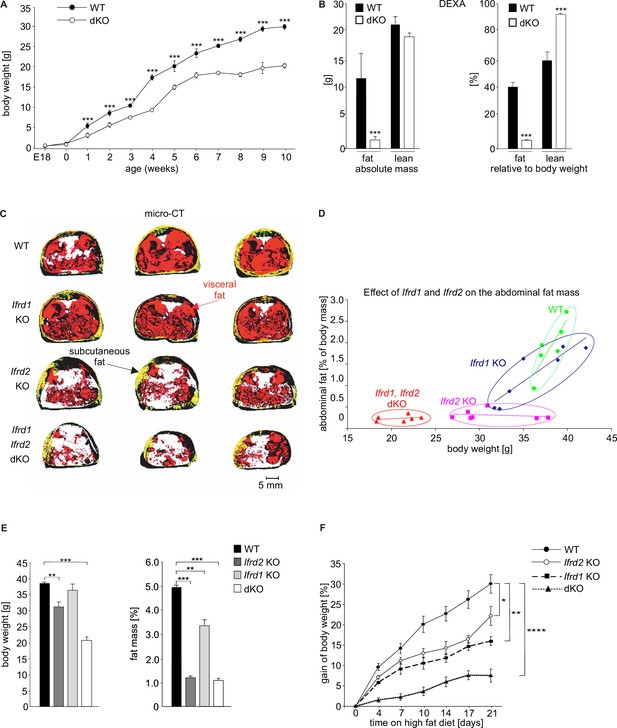
Double knockout (dKO) mice display postnatal growth retardation, less body fat, and are resistant against high-fat diet (HFD)-induced obesity.
(A) Growth curves of wild type (WT) and dKO mice on chow diet (n fluctuate depending on the time point of measurement). (B) Dual-energy X-ray absorptiometry (DEXA) measurements of WT and dKO mice. Left panel depicts the absolute values of fat and lean mass per animal, and the right panel represents values normalized to the total body weight of animals (n = 15). (C) Micro-computed tomography measurements identified a lack of abdominal fat in single and dKO mice. Three-dimensional reconstitution of images of the abdominal fat mass distribution in WT and KO mice. Yellow color represents subcutaneous and red visceral fat mass. Black compartments are shadows resulting as part of the lightning model for the 3D volume rendering. (D) Mass contribution (%) of the abdominal fat in correlation to the total body weight (g). A linear regression is overlaid for each group individually. The regression results for the WT group are y = 0.342x - 11.13 with an R2 of 0.74; for the Ifrd1 KO group y = 0.143x - 3.91 with an R2 of 0.82; for the Ifrd2 KO y = 0.005x + 0.48 with an R2 of 0.04 and; for the dKO y = 0.011x + 0.01 with an R2 of 0.1. ANCOVA for the fat mass as a percentage of the body weight as covariant was performed. (E) Effect of single Ifrd1 or Ifrd2 knockout and Ifrd1 Ifrd2 double knockout on total body weight in (g) and body fat amount normalized to the body weight in (%). (F) Ifrd1 and Ifrd2 knockout reduced the gain of body weight of mice fed with HFD. 7-week-old male WT and dKO mice were caged individually and maintained up to 21 d on HFD. Data shown are mean ± STD, n ≥ 9 per genotype. Data were analyzed applying one-way ANOVA with Holm–Šidák’s multiple-comparisons test. *p<0.05, **p<0.01, ****p<0.0001.
-
Figure 1—source data 1
DEXA and microCT measurements of IFRD1 and IFRD2 double knockout mice.
- https://cdn.elifesciences.org/articles/88350/elife-88350-fig1-data1-v3.xls
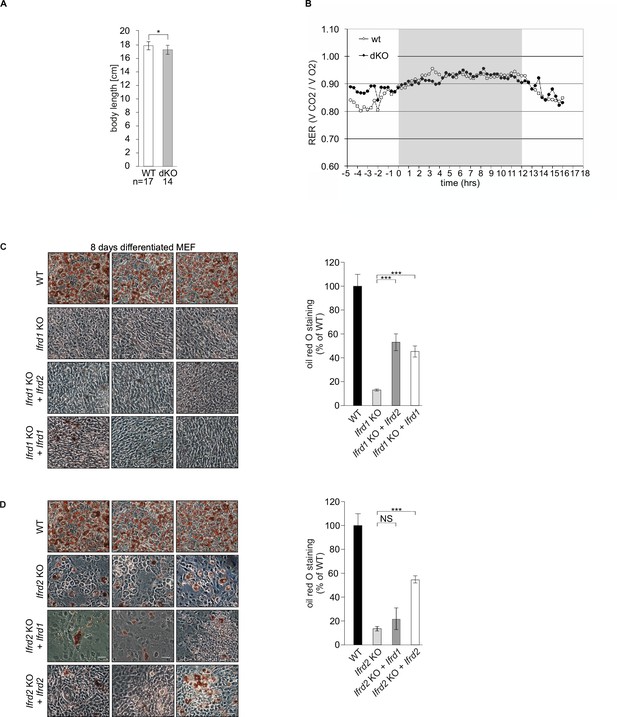
Body length and respiratory exchange of double knockout (dKO) and wild type (WT) mice.
Ifrd1 and Ifrd2 positively regulate adipocyte differentiation of mouse embryonic fibroblast (MEF) cells. (A) Measurement of whole body length, including the tale of age- and sex-matched mice. n = 14 and 17, respectively. Data shown are mean ± STD, *p<0.01 (B) Indirect calorimetry measurements of WT and dKO animals. No significant differences were found in respiratory exchange ratios (RER) of WT and dKO mice. (C) Oil red O staining of MEFs following 8-day adipocyte differentiation protocol. Ectopic expression of Ifrd1 and/or Ifrd2 induced adipocyte differentiation of Ifrd1 KO MEFs, size bar 20 μm. Quantification of oil red O staining, n = 3. Data shown are mean ± STD, ***p<0.001. (D) Ectopic expression of Ifrd2 induced adipocyte differentiation of Ifrd2 KO MEFs, size bar 20 μm. Quantification of oil red O staining, n = 3. Data shown are mean ± STD, ***p<0.001.
-
Figure 1—figure supplement 1—source data 1
Further characterization of dKO mice.
- https://cdn.elifesciences.org/articles/88350/elife-88350-fig1-figsupp1-data1-v3.pdf

No difference between wild type (WT) and double knockout (dKO) mice in food consumption.
Expression patterns of Ifrd1 and Ifrd2 in WT mouse embryonic fibroblasts (MEFs) during adipocyte differentiation. Ifrd2 knockout strategy. (A) No significant genotype effect on food intake; when adjusted to the body mass, nonsignificantly decreased in dKO mice (4.0 ± 0.4 g in WT vs. 3.4 ± 0.5 g dKO mice). Data shown are mean ± STD. (B) Hepatic lipase serum concentrations of WT (n = 4) and dKO (n = 4) 11-week-old male mice after 3 wk of high-fat diet (HFD). Data shown are mean ± STD. (C) Lipoprotein lipase serum concentrations of WT (n = 4) and dKO (n = 4) 8-week-old male mice following 3 wk of HFD. Data shown are mean ± STD. (D) Ifrd1 expression reaches its maximum on day 3 of MEF adipocyte differentiation. Data shown are mean ± STD. Student’s t-test p<0.01, n = 3. (E) Ifrd2 reaches its maximum expression on day 5 of MEF adipocyte differentiation. Data shown are mean ± STD. Student’s t-test p<0.05, n = 3. All Ct values were normalized to GAPDH. (F) Modification of the Ifrd2 gene locus and characterization of the targeted and null genotype. Homologous recombination with the targeting vector inserts one loxP site and a frt site flanked neomycin resistance gene (PGK–Neo) into intron 1 at position 105515 (AY162905). A second loxP site inserted behind the poly A tail into the 3´ UTR of the SKMc15 gene at position 102082 (AY162905). Further downstream were added 15 additional nucleotides, parts of intron 1, exon 2 (splice site: donor and acceptor) and the CDS of hrGFP (out of Vitality hrGFP Mammalian Expression Vector pIRES-hrGFP-2a; Stratagene). Arrows indicate the orientation of the hrGFP gene and the loxP sites. Southern blot analysis. The fragment detected by the Southern probe, was 9.6 kb in the WT (insert, band V) and 15 kb in the deleted locus (band IV). (G) PCR genotype analysis of Ifrd2 targeted and deleted alleles. I: 426 bp WT Ifrd2 PCR fragment. II: 1772 bp Ifrd2 targeted allele PCR product. III: 1700 bp Ifrd2 GFP screening PCR product can be amplified from the targeted (F) and the deleted allele (-/-), but not from the WT (+/+) locus. (H) Ifrd1 PCR detection in dKO mice. Left panel: detection of 1800 bp Ifrd1 WT allele; right panel: detection of 2100 bp Ifrd1 KO allele. (I) dKO MEFs overexpressing Ifrd1. The overexpression level of Ifrd1 is 14.7-fold in comparison to the endogenous levels in WT MEF cells. (J) dKO MEFs overexpressing Ifrd2. The overexpression level is 10.91-fold in comparison to the endogenous levels in WT MEF cells. (K) Analysis of total cholesterol in plasma. Two-tailed unpaired t-test did not identify any significant difference between WT and Ifrd1 Ifrd2 dKO mice (n = 4 per group, p=0.3258). (L) Analysis of total triglycerides in plasma. Two-tailed unpaired t-test did not identify any significant difference between WT and Ifrd1 Ifrd2 dKO mice (n = 4 per group, p=0.0647). (M) Lipoprotein profiles analysis of pooled plasma of WT and Ifrd1 Ifrd2 dKO mice was performed using fast protein liquid chromatography (n = 4 per group).
-
Figure 1—figure supplement 2—source data 1
Supplementary information for the Figure 1.
- https://cdn.elifesciences.org/articles/88350/elife-88350-fig1-figsupp2-data1-v3.zip
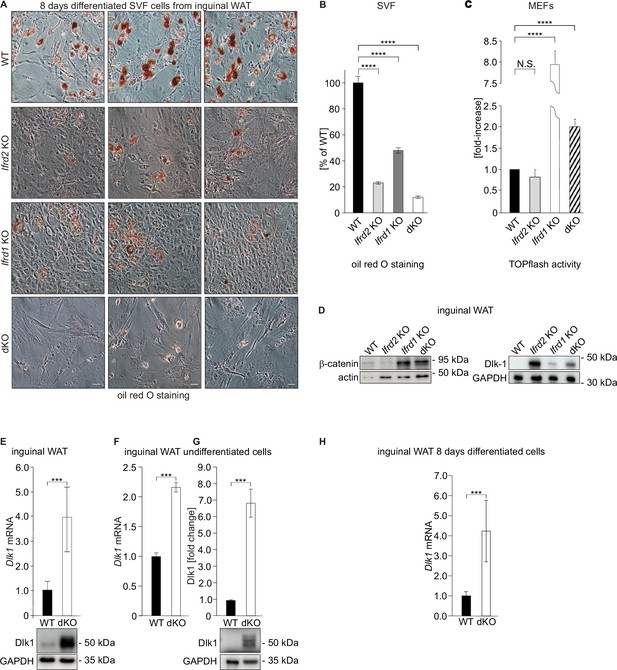
Adipocyte differentiation, Dlk1 levels, and Wnt signaling pathway are significantly affected in double knockout (dKO) mice.
(A) Representative oil red O staining of inguinal stromal vascular fraction (SVF) cells following 8 d of the adipocyte differentiation protocol. Scale bar is 20 µm. (B) Quantification of oil red O staining from three independent adipocyte differentiation experiments shown in panel (A). Ordinary one-way ANOVA ****p<0.0001. (C) Wnt signaling activity was measured in mouse embryonic fibroblasts (MEFs) transiently co-transfected with the Tcf reporter plasmid pTOPflash and β-galactosidase expression vectors. Transfection efficiency was normalized using the β-galactosidase values. The experiment was repeated three times. Data shown are mean ± STD. Ordinary one-way ANOVA Holm–Šídák’s multiple-comparisons test ****p<0.0001. (D) Left top panel: western blot analysis of β-catenin amounts in inguinal WAT samples. Representative western blot from three biological repetitions is shown. Right top panel: Dlk1 is significantly upregulated both in Ifrd1, Ifrd2 single KO and in dKO mice. Bottom panels: actin western blots were used for normalization of sample loading. (E) Dlk1 mRNA expression measured by qPCR in inguinal white adipose tissue (WAT) samples (top). Values were normalized on GAPDH, n = 3. Error bars indicate standard deviations. A representative western blot image detecting Dlk1 and GAPDH proteins (bottom). (F) Dlk1 mRNA expression detected in undifferentiated cells isolated from inguinal WAT SVF cells. Normalized on GAPDH. Error bars indicate standard deviations, ***p<0.001. (G) Dlk1 protein western blot analysis in undifferentiated cells isolated from inguinal WAT SVF cells. Mean of three biological repeats; inset is one representative western blot. ***p<0.001. (H) Dlk1 mRNA expression detected in 8 d adipocyte-differentiated cells isolated from inguinal WAT SVF cells. Normalized on GAPDH. Error bars indicate standard deviations, ***p<0.001.
-
Figure 2—source data 1
b-catenin and Dlk1 protein levels in inguinal WAT.
- https://cdn.elifesciences.org/articles/88350/elife-88350-fig2-data1-v3.zip
-
Figure 2—source data 2
Dlk1 mRNA levels in inguinal WAT.
- https://cdn.elifesciences.org/articles/88350/elife-88350-fig2-data2-v3.zip
-
Figure 2—source data 3
Dlk1 protein levels in inguinal WAT cells.
- https://cdn.elifesciences.org/articles/88350/elife-88350-fig2-data3-v3.zip
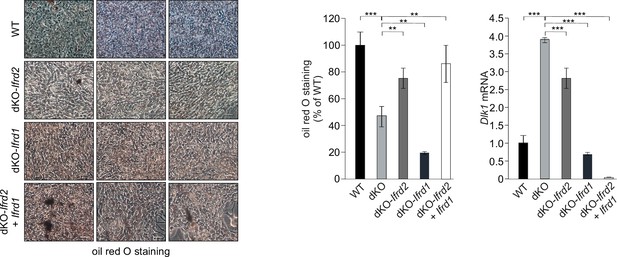
Ifrd1 and Ifrd2 positively regulate adipocyte differentiation of mouse embryonic fibroblast (MEF) cells and inhibit Dlk1 expression.
Ectopic expression of Ifrd1 and/or Ifrd2 induced adipocyte differentiation of double knockout (dKO) MEFs, size bar 20 μm. Quantification of oil red O staining, n = 3, ***p<0.001. Ectopic expression of Ifrd1 and/or Ifrd2 inhibited Dlk1 mRNA expression detected in dKO MEF cells. Normalized on GAPDH. Error bars indicate standard deviations, Wild type (WT) MEF values were set as 1. Data shown are mean ± STD, ***p<0.001.
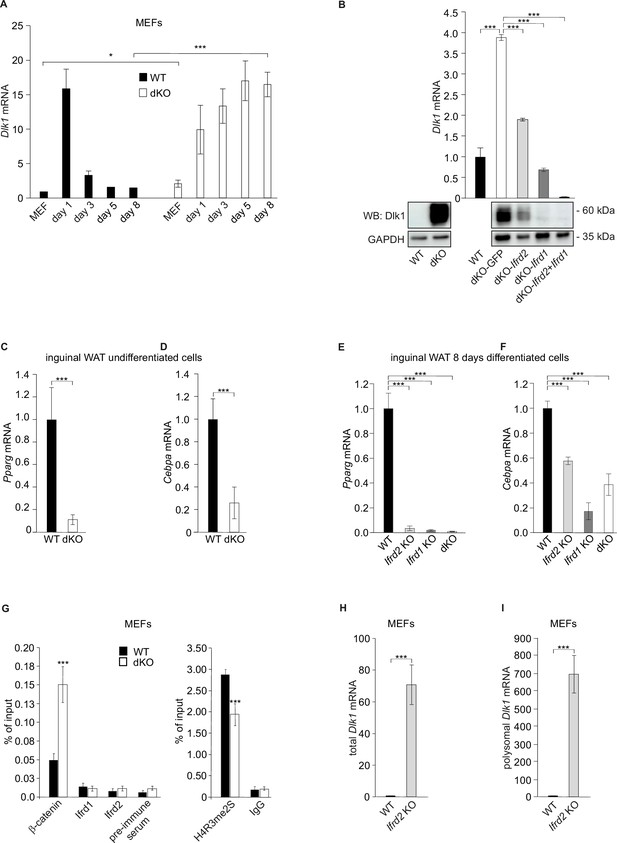
Ifrd1 and Ifrd2 regulate Dlk1 and thereby affect adipocyte regulators expression.
(A) Dlk1 mRNA expression measured in mouse embryonic fibroblasts (MEFs) treated with the adipocyte differentiation cocktail for given times. Wild type (WT) MEF values were set as 1. *p<0.05, ***p<0.001. (B) Dlk1 mRNA levels (top) and protein levels (bottom) in double knockout (dKO) MEFs were downregulated following ectopic co-expression of Ifrd1 and Ifrd2. mRNA expression levels were analyzed by RT qPCR in stably transfected cells following 8-day adipocyte protocol differentiation. WT MEF values were set as 1, n = 3; error bars indicate standard deviations. ***p<0.001 (C) Pparg mRNA expression detected in undifferentiated cells isolated from inguinal white adipose tissue (WAT) stromal vascular fraction (SVF) cells. Normalized on GAPDH. Error bars indicate standard deviations, ***p<0.001. (D) Cebpa mRNA expression detected in undifferentiated cells isolated from inguinal WAT SVF cells. Normalized on GAPDH. Error bars indicate standard deviations, ***p<0.001. (E) Pparg mRNA expression detected in 8-day adipocyte-differentiated cells isolated from inguinal WAT SVF cells. Normalized on GAPDH. Error bars indicate standard deviations, ***p<0.001. (F) Cebpa mRNA expression detected in 8-day adipocyte-differentiated cells isolated from inguinal WAT SVF cells. Normalized on GAPDH. Error bars indicate standard deviations, ***p<0.001. (G) Recruitment of indicated proteins to regulatory regions of the Dlk1 promoter in WT and KO MEFs was analyzed by chromatin immunoprecipitation (ChIP) at day 8 of the adipocyte differentiation. Values are expressed as the percentage of immunoprecipitated chromatin relative to input and are the mean of triplicates. ChIP analysis identified increased specific β-catenin binding to its Dlk1 regulatory element in dKO samples. Pre-immune serum and IgG were used as background controls. n = 3, data shown are mean ± STD, Student’s t-test ***p<0.001. (H) Dlk1 mRNA expression detected in undifferentiated MEF cells. Normalized on GAPDH. Error bars indicate standard deviations, ***p<0.001. (I) Real-time qPCR detection of Dlk1 RNA in polyribosomes. Normalized on GAPDH. Error bars indicate standard deviations, ***p<0.001.
-
Figure 3—source data 1
Supplementary information for the Figure 3 - WB images.
- https://cdn.elifesciences.org/articles/88350/elife-88350-fig3-data1-v3.zip
-
Figure 3—source data 2
qPCR measurements for Figure 3G.
- https://cdn.elifesciences.org/articles/88350/elife-88350-fig3-data2-v3.zip
-
Figure 3—source data 3
Supplementary information for the Figure 3.
- https://cdn.elifesciences.org/articles/88350/elife-88350-fig3-data3-v3.zip

Dlk1 inhibits adipocyte differentiation of double knockout (dKO) mouse embryonic fibroblasts (MEFs).
Cells from which the conditioned medium was transferred during the differentiation. Oil red O staining (panels on the left) after 8 d of adipocyte differentiation. Western blot detection of Dlk1 protein in differentiated cells.
-
Figure 3—figure supplement 1—source data 1
WB images for the Figure 3—figure supplement 1.
- https://cdn.elifesciences.org/articles/88350/elife-88350-fig3-figsupp1-data1-v3.zip
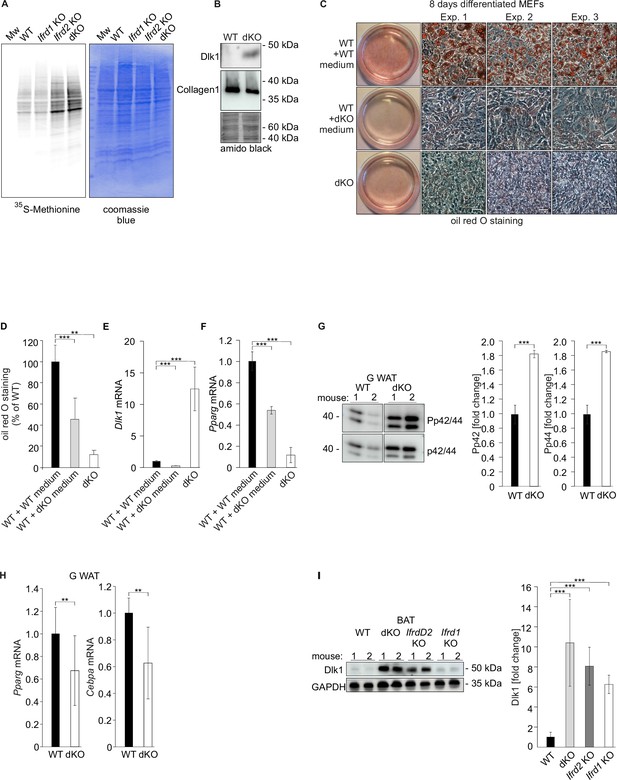
Ifrd2 inhibits Dlk1 translation.
Double knockout (dKO)-secreted Dlk1 inhibits adipocyte differentiation through MEK/ERK signaling. (A) Translational analysis – in vivo metabolic staining of mouse embryonic fibroblasts (MEFs) with 35S-methionine. Equal numbers of cells were seeded and 24 hr later treated as explained in the ‘Methods’ section. Identical volumes of cell lysates were separated by SDS-PAGE, gel was dried and analyzed by a phosphorimager. Equal loading was documented by the Coomassie blue staining of the gel. (B) dKO MEFs secrete Dlk1 protein into the cell culture medium. Identical volumes of media from wild type (WT) and dKO MEF cells 8 d treated with the adipocyte differentiation cocktail were analyzed by western blot. Collagen I was present in both samples in similar amounts. Equal loading of samples was evaluated by amido black staining of the membrane. (C) dKO MEFs-conditioned medium inhibited adipocyte differentiation in WT MEFs. MEFs were treated 8 d with the adipocyte differentiation cocktail. WT MEFs treated with the dKO-conditioned medium (replaced three times every 2 d) showed reduced differentiation. Images representing three biological repeats were cropped, and space bars represent always 20 µm. (D) Quantification of oil red O staining from three independent adipocyte differentiation experiments. Data shown are mean ± STD, ordinary one-way ANOVA p=0.0016. Student’s t-test, **p<0.01, ***p<0.001. (E) Dlk1 mRNA levels in WT and dKO MEFs treated 8 d with the adipocyte differentiation cocktail or in WT MEFs treated with the dKO-conditioned medium, ***p<0.001. (F) Pparg mRNA expression levels in same cells as shown in panels (D) and (E), ***p<0.001. (G) Representative western blots of phospho-p44, phospho-p42, p44, and p42 in gonadal white adipose tissue (WAT) of WT and dKO mice. Normalization on p44 and p42; n = 3. Error bars indicate standard deviations. WT values were set as 1, ***p<0.001. (H) Pparg and Cebpa mRNA expression in gonadal WAT. Normalization on GAPDH; n = 3. Error bars indicate standard deviations. WT values were set as 1, **p<0.01. (I) Dlk1 protein is upregulated in brown adipose tissues (BAT) of Ifrd1, Ifrd2 single knockout and in dKO mice. Western blot analysis was performed on five samples of each genotype. Normalization on GAPDH. Error bars indicate standard deviations, ***p<0.001.
-
Figure 4—source data 1
Original images of the Figure 4A.
- https://cdn.elifesciences.org/articles/88350/elife-88350-fig4-data1-v3.zip
-
Figure 4—source data 2
Original images of the Figure 4B.
- https://cdn.elifesciences.org/articles/88350/elife-88350-fig4-data2-v3.zip
-
Figure 4—source data 3
Original images of the Figure 4G.
- https://cdn.elifesciences.org/articles/88350/elife-88350-fig4-data3-v3.zip
-
Figure 4—source data 4
Original images of the Figure 4I.
- https://cdn.elifesciences.org/articles/88350/elife-88350-fig4-data4-v3.zip
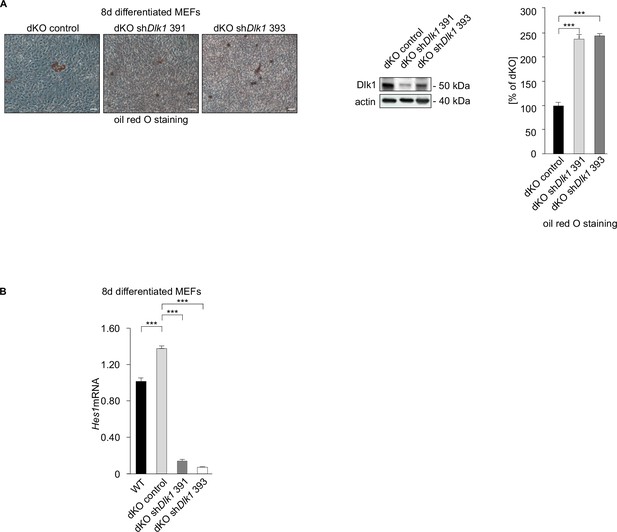
Dlk1 inhibits adipocyte differentiation of double knockout (dKO) mouse embryonic fibroblasts (MEFs) and regulates Hes1 expression.
(A) Oil red O staining (left panel) of dKO control and shDlk1 MEFs following 8-day adipocyte differentiation protocol. Dlk1 knockdown induced adipocyte differentiation of dKO MEFs, size bar 20 μm. Western blot documenting (middle panel) the knockdown efficiency of two independent shRNA constructs targeting Dlk1. Quantification of oil red O staining, n = 3. Data shown are mean ± STD, ***p<0.001. (B) Hes1 mRNA expression detected in 8-day differentiated MEF cells. Normalized on GAPDH. Error bars indicate standard deviations, WT MEF values were set as 1. Data shown are mean ± STD, ***p<0.001.
-
Figure 4—figure supplement 1—source data 1
Original images of the Figure 4—figure supplements 1 and 2.
- https://cdn.elifesciences.org/articles/88350/elife-88350-fig4-figsupp1-data1-v3.zip
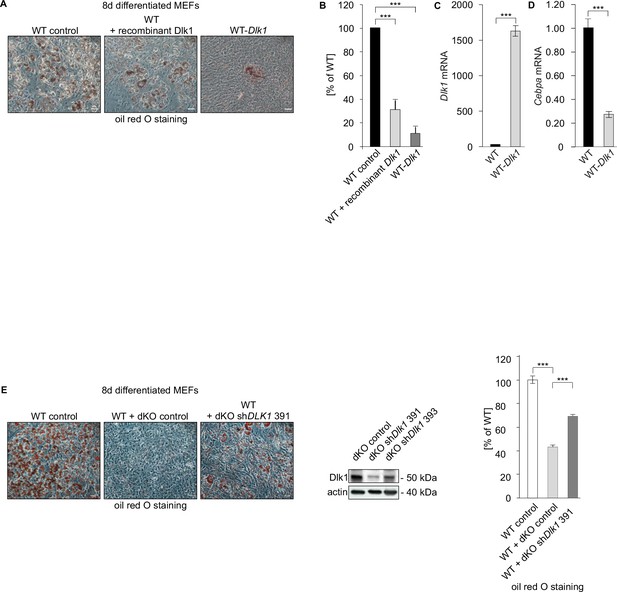
Dlk1, adipocyte differentiation of double knockout (dKO) mouse embryonic fibroblast (MEF) cells, and Cebpa expression.
dKO MEFs-secreted Dlk1 inhibits adipocyte differentiation of wild type (WT) MEF cells. (A) Oil red O staining (left panel) of 8-day adipocyte-differentiated WT control MEFs, WT MEFs treated with a recombinant Dlk1 or expressing ectopic Dlk1. (B) Quantification of oil red O staining shown in panel (A), n = 3. Data shown are mean ± STD, ***p<0.001. (C) Dlk1 mRNA expression detected in 8-day differentiated MEF cells. (D) Cebpa mRNA expression detected in 8-day differentiated MEF cells. Normalized on GAPDH. Error bars indicate standard deviations, WT MEF values were set as 1. Data shown are mean ± STD, ***p<0.001. (E) Oil red O staining of WT control MEFs (left image) following 8-day adipocyte differentiation protocol. WT cells differentiated in conditioned medium from dKO MEFs (middle image) and WT MEFs treated with conditioned medium from dKO MEFs expressing Dlk1 shRNA construct (right image). Size bar 20 μm. Western blot documenting (middle panel) the knockdown efficiency of shRNA constructs targeting Dlk1. Quantification of oil red O staining, n = 3. Data shown are mean ± STD, ***p<0.001.
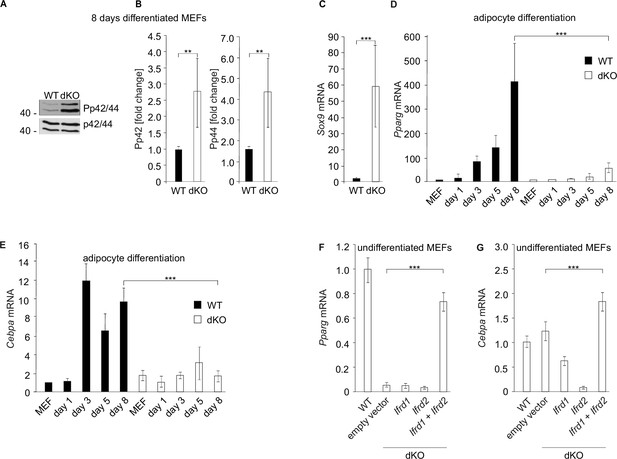
MAPK signaling and Sox9 expression are induced while adipocyte differentiation regulatory genes are downregulated in double knockout (dKO) mouse embryonic fibroblasts (MEFs).
(A) Representative western blots of phospho-p44, phospho-p42, p44, and p42 from 8-day adipocyte differentiation cocktail-treated MEFs. (B) Quantitative analysis of western blot data. Normalization on p44 and p42; n = 3. Error bars indicate standard deviations. Wild type (WT) values were set as 1; **p<0.01. (C) Sox9 expression was measured by qPCR in 8-day adipocyte-differentiated WT and dKO MEFs. Sox9 values were normalized on GADPH expression. WT MEF values were set as 1, n = 3. Data shown are mean ± STD. Student’s t-test ***p<0.001. (D) Pparg and (E) Cebpa mRNA levels were downregulated in dKO adipocyte-differentiated MEFs. Gene expression was measured by qPCR during the treatment with the adipocyte differentiation cocktail. Values were normalized on GADPH expression. WT MEF values were set as 1, n = 3. Data shown are mean ± STD, *** p<0.001. (F) Ectopic co-expression of Ifrd1 and Ifrd2 in undifferentiated MEFs significantly increased levels of adipogenic genes Pparg and (G) Cebpa. Data shown are mean ± STD, Student’s t-test ***p<0.001.
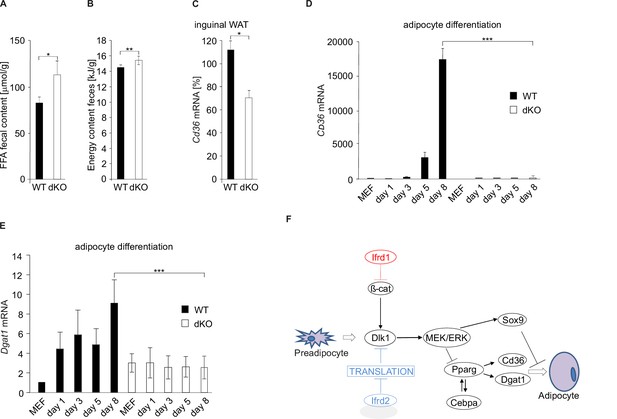
Free fatty acid (FFA) uptake is inhibited in double knockout (dKO) mice.
(A) FFA concentrations in feces of wild type (WT) and dKO mice. Feces were collected every second day, and the composition of excreted lipids was determined by capillary gas chromatography. Data shown are mean ± STD, *p<0.05. (B) Energy content of dried egested feces samples was determined by bomb calorimetry. Data shown are mean ± STD, **p<0.01. (C) qPCR analysis of Cd36 mRNA levels in inguinal white adipose tissue (WAT). Data shown are mean ± STD, *p<0.05. (D) qPCR analysis of Cd36 in mouse embryonic fibroblasts (MEFs) differentiating into adipocytes. Relative expression levels were normalized on GAPDH expression. WT MEF values were set as 1. Data shown are mean ± STD, ***p<0.001. (E) Dgat1 mRNA levels were downregulated in dKO adipocyte-differentiated MEFs. Data shown are mean ± STD, ***p<0.001. (F) Proposed model of Ifrd1 and Ifrd2 molecular mechanisms of action during adipocyte differentiation. Two parallel mechanisms leading to a deficiency in adipocyte differentiation: Ifrd1-regulated Wnt signaling affected Dlk1 transcription and Ifrd2 acting as a translational inhibitor also contributed to the regulation of adipogenesis.
Tables
Double knockout (dKO) mice do not differ from wild type mice in any metabolic parameter besides the body weight.
A 21-hr indirect calorimetry trial monitoring gas exchange (oxygen consumption and carbon dioxide production), activity (distance and rearing), and food intake. The genotype effects were statistically analyzed using one-way ANOVA. Food intake and energy expenditure were analyzed using a linear model including body mass as a co-variate.
Parameter | ANOVA (genotype)* LM (body mass as co-variate) | |||
---|---|---|---|---|
Wild type | dKO | Genotype | Body mass | |
n = 6 | n = 7 | |||
Mean ± STDEV | Mean ± STDEV | p Value | p Value | |
Body mass (g) | 31.1 ± 2.6 | 20.4 ± 1.9 | <0.0001 | n/a |
Body temperature (°C) | 36.56 ± 0.6 | 36.71 ± 0.5 | 0.6515 | n/a |
* Food intake (g) | 4.0 ± 0.4 | 3.4 ± 0.5 | 0.5869 | 0.1277 |
* Mean VO2 (ml/hr) | 107.28 ± 8.41 | 81.12 ± 5.69 | 0.6441 | 0.0241 |
* Min VO2 (ml/hr) | 78.83 ± 8.26 | 57.71 ± 8.86 | 0.9047 | 0.1219 |
* Max VO2 (ml/hr) | 144.33 ± 6.98 | 107.29 ± 7.59 | 0.1944 | 0.0082 |
Mean RER | 0.89 ± 0.01 | 0.90 ± 0.02 | 0.3754 | n/a |
Mean dist D (cm 20 min–1) | 928 ± 173 | 874 ± 219 | 0.6399 | n/a |
Mean Z (rearing 20 min–1) | 116 ± 34 | 96 ± 25 | 0.2444 | n/a |
-
RER, respiratory exchange ratio.
Statistical analysis of results presented in Figure 1F.
Holm-Šidák's multiple comparisons test | Summary | Adjusted P Value |
---|---|---|
WT vs. TIS7 KO | ** | 0,0096 |
WT vs. SKMc15 KO | * | 0,0308 |
WT vs. dKO | **** | <0,0001 |