Parasite Communication: Learning the language of pathogens
To survive and replicate, pathogens must evade the immune system of the organisms they infect by adapting to the environment inside them. The individual pathogens that manage to do this will survive and multiply, and particularly successful adaptations may lead to the emergence of new strains of the pathogen (Bashey, 2015; Mideo, 2009). Understanding how this happens – including how pathogens communicate with each other, and how they respond to the microbiota of their host (Kalia et al., 2020) – is crucial to efforts to combat the diseases caused by parasites and other pathogens.
Decades of studying host-pathogen interactions has revealed several mechanisms of pathogen virulence, such as variation of surface antigens to avoid immune detection and the release of molecules that neutralize the host immune system and promote pathogen invasion (Casadevall and Pirofski, 2001). Specific structures and organelles that pathogens employ – such as flagella to allow swimming – have also been discovered (Khan and Scholey, 2018).
Cells have been shown to communicate with one another by exchanging membrane-bound ‘extracellular vesicles’ – which contain proteins, lipids and genetic material – with neighbouring cells (van Niel et al., 2018; Raposo and Stahl, 2019). Cells can also communicate via plasma membrane protrusions called ‘filopodia’ which contain bundles of actin filaments and have roles in cellular adherence and migration (Roy and Kornberg, 2015). There are multiple types of filopodia, categorized by their size and origin.
Now, in eLife, Natalia de Miguel (Instituto Tecnológico Chascomús and Escuela de Bio y Nanotecnologías) and colleagues – including Nehuén Salas and Manuela Blasco Pedreros as joint first authors – report that different strains of the protozoan Trichomonas vaginalis, a parasite responsible for sexually-transmitted infections, communicate using extracellular vesicles and a type of filopodia called a cytoneme (Salas et al., 2023). Cytonemes are thin specialized filopodia that can traffic signalling proteins (Roy and Kornberg, 2015).
T. vaginalis is a single-celled parasite which colonizes the human urogenital tract and adheres to epithelial cells. Strains that are highly adherent can form clumps more easily than other strains when cultured, and it has been shown that these strains are more cytotoxic to host cells (Coceres et al., 2015; Lustig et al., 2013). Through live imaging, Salas et al. showed that cytonemes are visible on the surface of T. vaginalis, and that highly adherent strains have more cytonemes than poorly adherent strains. The number of parasites displaying cytonemes also increased when clumps formed, with the individual parasites within the clumps being connected by cytonemes.
In elegant experiments using inserts with a porous membrane that prevents direct contact between parasites – but allows secreted factors through – Salas et al. demonstrate that extracellular vesicles of less adherent strains stimulate the growth of cytonemes in the most adherent strain. Moreover, overexpressing the protein VPS32 – which regulates the biogenesis of small vesicles – stimulated the induction of cytonemes, suggesting their formation is, in part, contact-independent.
These findings raised the question of what signals within extracellular vesicles from poorly adherent strains stimulate cytoneme formation in highly adherent strains. To investigate, Salas et al. compared the proteins expressed in the extracellular vesicles of multiple strains of T. vaginalis, finding differences in protein expression but conservation of the biological processes they are involved in. The strains shared essential components of metabolic processes, signal response, development and locomotion. Furthermore, all strains contained proteins associated with the formation of cytonemes.
Finally, Salas et al. harnessed the ability of T. vaginalis to change from a swimming flagellate form to an amoeboid when it adheres to prostate cells, in order to investigate how communication impacts its behavior during infection (Kusdian et al., 2013). Again, through controlled experiments using porous inserts, they showed that less adherent strains induce the amoeboid morphology and the adhesion of the highly adherent strain to prostate cells. Surprisingly, this parasite-to-parasite communication also doubled the adhesion of a poorly adherent strain to the cells.
The experiments provide solid evidence of the participation of extracellular vesicles in communications between parasites, as well as the presence of specific membranous structures that allow this communication. The finding that parasites of different strains communicate with one another raises fundamental questions related to parasitism and the pathology of Trichomonas. Why do poorly adherent strains have a greater effect on the formation of cytonemes by adherent strains than their own strain? Do the secreted extracellular vesicles signal the presence of another strain, alerting nearby parasites to enhance their adherence in order to outcompete competitors? Future work should also investigate the role of microbiota and infections that often occur alongside Trichomonas, such as Mycoplasma (Margarita et al., 2020), in parasite communication and behavior.
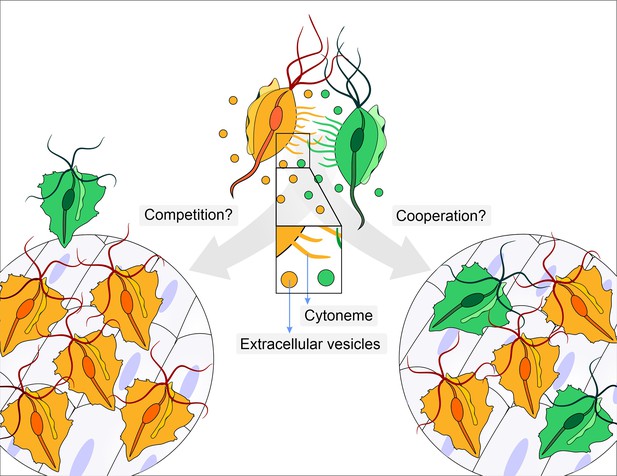
Does parasite communication result in competition or cooperation?
Different strains of Trichomonas vaginalis (yellow and green) communicate with one another through the release of extracellular vesicles and the formation of membrane protrusions called cytonemes (depicted in inset). This communication can lead to increased adherence of the parasites to epithelial cells. It is not clear whether this communication leads to competition (left), where one strain (yellow) enhances its adherence in order to outcompete a less adherent strain (green), or whether it leads to cooperation (right) where a less adherent strain (green) becomes more adherent after contact with a highly adherent strain (yellow).
References
-
Within-host competitive interactions as a mechanism for the maintenance of parasite diversityPhilosophical Transactions of the Royal Society of London. Series B, Biological Sciences 370:20140301.https://doi.org/10.1098/rstb.2014.0301
-
Host-pathogen interactions: the attributes of virulenceThe Journal of Infectious Diseases 184:337–344.https://doi.org/10.1086/322044
-
Microbiota in vaginal health and pathogenesis of recurrent vulvovaginal infections: a critical reviewAnnals of Clinical Microbiology and Antimicrobials 19:5.https://doi.org/10.1186/s12941-020-0347-4
-
Assembly, functions and evolution of archaella, flagella and ciliaCurrent Biology 28:R278–R292.https://doi.org/10.1016/j.cub.2018.01.085
-
Trichomonas vaginalis contact‐dependent cytolysis of epithelial cellsInfection and Immunity 81:1411–1419.https://doi.org/10.1128/IAI.01244-12
-
Impact of symbiosis between Trichomonas vaginalis and Mycoplasma hominis on vaginal dysbiosis: a mini reviewFrontiers in Cellular and Infection Microbiology 10:179.https://doi.org/10.3389/fcimb.2020.00179
-
Parasite adaptations to within-host competitionTrends in Parasitology 25:261–268.https://doi.org/10.1016/j.pt.2009.03.001
-
Extracellular vesicles: a new communication paradigmNature Reviews Molecular Cell Biology 20:509–510.https://doi.org/10.1038/s41580-019-0158-7
-
Shedding light on the cell biology of extracellular vesiclesNature Reviews Molecular Cell Biology 19:213–228.https://doi.org/10.1038/nrm.2017.125
Article and author information
Author details
Publication history
- Version of Record published: June 15, 2023 (version 1)
Copyright
© 2023, Rossi and Ramirez
This article is distributed under the terms of the Creative Commons Attribution License, which permits unrestricted use and redistribution provided that the original author and source are credited.
Metrics
-
- 1,054
- views
-
- 39
- downloads
-
- 0
- citations
Views, downloads and citations are aggregated across all versions of this paper published by eLife.
Download links
Downloads (link to download the article as PDF)
Open citations (links to open the citations from this article in various online reference manager services)
Cite this article (links to download the citations from this article in formats compatible with various reference manager tools)
Further reading
-
- Microbiology and Infectious Disease
The target of rapamycin (TOR) signaling pathway is highly conserved and plays a crucial role in diverse biological processes in eukaryotes. Despite its significance, the underlying mechanism of the TOR pathway in Aspergillus flavus remains elusive. In this study, we comprehensively analyzed the TOR signaling pathway in A. flavus by identifying and characterizing nine genes that encode distinct components of this pathway. The FK506-binding protein Fkbp3 and its lysine succinylation are important for aflatoxin production and rapamycin resistance. The TorA kinase plays a pivotal role in the regulation of growth, spore production, aflatoxin biosynthesis, and responses to rapamycin and cell membrane stress. As a significant downstream effector molecule of the TorA kinase, the Sch9 kinase regulates aflatoxin B1 (AFB1) synthesis, osmotic and calcium stress response in A. flavus, and this regulation is mediated through its S_TKc, S_TK_X domains, and the ATP-binding site at K340. We also showed that the Sch9 kinase may have a regulatory impact on the high osmolarity glycerol (HOG) signaling pathway. TapA and TipA, the other downstream components of the TorA kinase, play a significant role in regulating cell wall stress response in A. flavus. Moreover, the members of the TapA-phosphatase complexes, SitA and Ppg1, are important for various biological processes in A. flavus, including vegetative growth, sclerotia formation, AFB1 biosynthesis, and pathogenicity. We also demonstrated that SitA and Ppg1 are involved in regulating lipid droplets (LDs) biogenesis and cell wall integrity (CWI) signaling pathways. In addition, another phosphatase complex, Nem1/Spo7, plays critical roles in hyphal development, conidiation, aflatoxin production, and LDs biogenesis. Collectively, our study has provided important insight into the regulatory network of the TOR signaling pathway and has elucidated the underlying molecular mechanisms of aflatoxin biosynthesis in A. flavus.
-
- Microbiology and Infectious Disease
The microbiota is a key determinant of the physiology and immunity of animal hosts. The factors governing the transmissibility of viruses between susceptible hosts are incompletely understood. Bacteria serve as food for Caenorhabditis elegans and represent an integral part of the natural environment of C. elegans. We determined the effects of bacteria isolated with C. elegans from its natural environment on the transmission of Orsay virus in C. elegans using quantitative virus transmission and host susceptibility assays. We observed that Ochrobactrum species promoted Orsay virus transmission, whereas Pseudomonas lurida MYb11 attenuated virus transmission relative to the standard laboratory bacterial food Escherichia coli OP50. We found that pathogenic Pseudomonas aeruginosa strains PA01 and PA14 further attenuated virus transmission. We determined that the amount of Orsay virus required to infect 50% of a C. elegans population on P. lurida MYb11 compared with Ochrobactrum vermis MYb71 was dramatically increased, over three orders of magnitude. Host susceptibility was attenuated even further in the presence of P. aeruginosa PA14. Genetic analysis of the determinants of P. aeruginosa required for attenuation of C. elegans susceptibility to Orsay virus infection revealed a role for regulators of quorum sensing. Our data suggest that distinct constituents of the C. elegans microbiota and potential pathogens can have widely divergent effects on Orsay virus transmission, such that associated bacteria can effectively determine host susceptibility versus resistance to viral infection. Our study provides quantitative evidence for a critical role for tripartite host-virus-bacteria interactions in determining the transmissibility of viruses among susceptible hosts.