Resident and recruited macrophages differentially contribute to cardiac healing after myocardial ischemia
Abstract
Cardiac macrophages are heterogenous in phenotype and functions, which has been associated with differences in their ontogeny. Despite extensive research, our understanding of the precise role of different subsets of macrophages in ischemia/reperfusion (I/R) injury remains incomplete. We here investigated macrophage lineages and ablated tissue macrophages in homeostasis and after I/R injury in a CSF1R-dependent manner. Genomic deletion of a fms-intronic regulatory element (FIRE) in the Csf1r locus resulted in specific absence of resident homeostatic and antigen-presenting macrophages, without affecting the recruitment of monocyte-derived macrophages to the infarcted heart. Specific absence of homeostatic, monocyte-independent macrophages altered the immune cell crosstalk in response to injury and induced proinflammatory neutrophil polarization, resulting in impaired cardiac remodeling without influencing infarct size. In contrast, continuous CSF1R inhibition led to depletion of both resident and recruited macrophage populations. This augmented adverse remodeling after I/R and led to an increased infarct size and deterioration of cardiac function. In summary, resident macrophages orchestrate inflammatory responses improving cardiac remodeling, while recruited macrophages determine infarct size after I/R injury. These findings attribute distinct beneficial effects to different macrophage populations in the context of myocardial infarction.
eLife assessment
Using state-of-the-art fate-mapping models and genetic and pharmacological targeting approaches, this study provides important findings on the distinct functions exerted by resident and recruited macrophages during cardiac healing after myocardial ischemia. Evidence supporting the conclusions are solid with the use of the FIRE mouse model in combination with fate-mapping to target fetal-derived macrophages. This study will be of interest for the macrophage biologists working in the heart but also in others tissues in the context of inflammation.
https://doi.org/10.7554/eLife.89377.4.sa0Introduction
Macrophages are important effectors of innate immunity. They are essential for host defense against infections but are also involved in different cardiovascular diseases. They represent the most abundant immune cell population in healthy cardiovascular tissues (Heidt et al., 2014; Weinberger et al., 2020), where they contribute to organ functions (Hulsmans et al., 2017) and maintenance of tissue homeostasis (Nicolás-Ávila et al., 2020). In cardiovascular diseases such as atherosclerosis and its main sequelae, ischemic stroke and acute myocardial infarction (AMI), macrophage functions are central to both disease development and healing. AMI has remained a leading cause of mortality and morbidity worldwide (Ahmad and Anderson, 2021; Lozano et al., 2012). Although acute survival in this condition has improved through the broad availability of percutaneous coronary intervention, adverse myocardial remodeling, and fibrosis frequently result in heart failure (Gerber et al., 2016). Pathophysiologically, the diminished blood supply to myocardial tissue during AMI leads to acute tissue necrosis, which induces a profound sterile inflammation and triggers complex cascade of immune processes and tissue remodeling (Hilgendorf et al., 2014; Honold and Nahrendorf, 2018; Nahrendorf et al., 2007). Consequently, uncontrolled immune reactions in the course of AMI are associated with impaired wound healing and adverse remodeling and can result in worsened cardiac outcome (Panizzi et al., 2010).
Macrophages play an essential role in cardiac injury, and thus represent a potential therapeutic target (Hilgendorf et al., 2014; Nahrendorf et al., 2007). However, they are an heterogenous population (Kubota et al., 2019; Weinberger and Schulz, 2015; Zaman and Epelman, 2022), and a large body of work has shown that they can have both pro- and anti-inflammatory functions. The differential roles of macrophage populations in AMI have remained incompletely understood. Cardiac macrophages can derive from embryonic and adult hematopoietic progenitors (Epelman et al., 2014). Fate-mapping analyses have identified yolk sac (YS) erythro-myeloid progenitors (EMPs) as a principal source of cardiac macrophages in adult life (Ginhoux et al., 2010). However, limited labeling in inducible cre reporter systems has not allowed for precisely differentiating and quantifying developmental origins of cardiac macrophages. Furthermore, targeting of these macrophages has been challenging (Frieler et al., 2015; Ruedl and Jung, 2018).
In this study, we investigated the cellular identity of cardiac macrophages in association with their developmental paths and their immune responses to ischemia/reperfusion (I/R) injury. By combining lineage tracing with single-cell RNA sequencing, we provide an in-depth analysis of the differential functions of resident and recruited cardiac macrophages. We then harnessed mice with genomic deletion of the fms-intronic regulatory element (FIRE) (Rojo et al., 2019), that allowed us to specifically address populations of resident macrophages in the infarcted heart and compared them to mice, in which both recruited and resident macrophages are depleted by pharmacological inhibtion of the CSF1R-signaling pathway. Using these approaches of selective macrophage depletion, we could attribute different beneficial functions to resident and also to recruited macrophages which impact differently on cardiac remodeling, infarct size, and cardiac outcome (Figure 1).
Results
Absence of resident cardiac macrophages in Csf1r∆FIRE mice
To quantify the contribution of YS EMPs to cardiac resident macrophages, we harnessed constitutive labeling in Tnfsf11aCre;Rosa26fs-eYFP mice (Jacome-Galarza et al., 2019; Mass et al., 2016; Percin et al., 2018). ~80% of cardiac macrophages expressed YFP in hearts of 12-week-old animals, whereas blood monocytes were not labeled (Figure 2A; Figure 2—figure supplement 1). EMP-derived microglia served as control confirming high efficiency of Cre-mediated recombination. In a comparative analysis, we traced fetal and adult definitive hematopoiesis in Flt3CreRosa26fs-eYFP mice (Gomez Perdiguero et al., 2015; Schulz et al., 2012), indicating that ~20% of cardiac macrophages derived from definitive HSC (Figure 2B). Thus, the majority of resident macrophages in the healthy heart is of early embryonic origin.
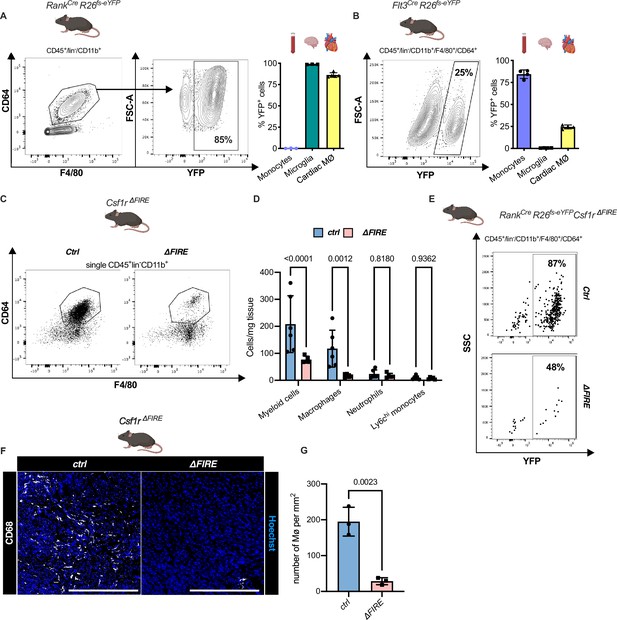
Absence of resident cardiac macrophages in Csf1r∆FIRE mice.
(A) Flow cytometry analysis of 3-month-old Tnfsf11aCreRosa26fs-eYFP mice, showing single/CD45+/lin-(CD11c, Ter119, Tcrß, Nk1.1)/CD11b+ cardiac cells, eYFP expression in macrophages (CD64+/F4/80+) and percentage of eYFP+ blood monocytes, microglia and cardiac macrophages (n = 3–5 each from an independent experiment). (B) Flow cytometry analysis of 3-month-old Flt3CreRosa26fs-eYFP mice, showing macrophage expression of eYFP and percentage of eYFP+ blood monocytes, microglia, and cardiac macrophages (n = 4 each from an independent experiment). (C) Representative flow cytometry analysis of cardiac macrophages in control and ∆FIRE mice. (D) Quantification of myeloid cells by flow cytometry (CD45+/lin−/CD11b+), macrophages (CD45+/lin−/CD11b+/CD64+/F4/80+), neutrophils (CD45+/lin−/CD11b+/CD64−/F4/80−/Ly6g+), and Ly6chi monocytes (CD45+/lin−/CD11b+/CD64−/F4/80−/Ly6g−/Ly6chi) (n = 6 for control and n = 5 for ∆FIRE mice, each single experiments). (E) Representative flow cytometry analysis of cardiac macrophages and their expression of eYFP in Csf1rΔFIRE/+Tnfsf11aCreRosa26fs-eYFP and Csf1rΔFIRE/ΔFIRETnfsf11aCreRosa26fs-eYFP. (F) Representative immunohistological images showing macrophages (CD68+ cells in white and Hoechst in blue) in control and ∆FIRE hearts in 3-month-old mice at baseline conditions (scale bars represent 500 µm). (G) Quantification of macrophages by histology (n = 3 for control and ∆FIRE mice). Either Fisher's Least Significant Difference (LSD) test or unpaired t-test was performed and mean ± standard deviation (SD) is shown.
To determine the role of resident macrophages in the mouse heart, we generated Tnfsf11aCreRosa26fs-DTR mice that express the avian diphtheria toxin (DT) receptor in the EMP lineage, rendering them susceptible to DT-mediated ablation. Administration of a single DT dose, however, resulted in premature death of all mice (n = 6) within 24 hr (Figure 2—figure supplement 2). The injected mice presented with a systemic inflammatory response syndrome-like phenotype, indicating that acute depletion of EMP-derived macrophages is not viable.
In Csf1r∆FIRE mice, genetic deletion of the FIRE in the first intron of the Csf1r gene leads to a selective absence of tissue macrophages without the developmental defects observed in Csf1r−/− mice (Munro et al., 2020; Rojo et al., 2019). We first confirmed that cardiac macrophages in Csf1r∆FIRE/∆FIRE mice (further termed ∆FIRE) mice were reduced by ~90% and the number of monocytes and neutrophils was not altered (Figure 2C–G). We thus crossed the ∆FIRE animals with the YS EMP fate-mapping line (Tnfsf11aCreRosa26fs-eYFP mice) and showed that the macrophages absent in ∆FIRE animals were EMP-derived macrophages (Figure 2E), thus offering a new model to investigate the role of resident macrophages in cardiac injury.
Changes in the cardiac immune phenotype in Csf1rΔFIRE mice in baseline conditions
To further characterize the macrophage populations absent in healthy hearts of adult ∆FIRE mice, we carried out single-cell RNA-sequencing (scRNA-seq) of CD45+ immune cells in wildtype, ∆FIRE, and Tnfsf11aCreRosa26fs-eYFP mice (Figure 3A, Figure 3—figure supplement 1). We identified the presence of six macrophage clusters in control mice (Figure 3B, Figure 3—figure supplement 1). Two clusters of macrophages expressing homeostatic (e.g. Lyve1, Gas6, Stab1) and antigen presentation-related genes (e.g. Cd74, H2-Ab1) were specifically ablated in ∆FIRE mice (Figure 3C), which largely represent YFP-expressing macrophages in Tnfsf11aCreRosa26fs-eYFP mice (Figure 3D). Specifically, we mapped ~90% of yfp transcripts to the two clusters of homeostatic and antigen-presenting macrophages that were ablated in ∆FIRE. Other clusters were not reduced in ∆FIRE, and also were not quantitatively represented in the YS macrophage lineage tracing model, that is Cx3cr1hi (2% YFP), Ccr2lowLy6clo (5% YFP), and Ccr2hiLy6chi (1% YFP) macrophages within respective clusters (Figure 3D). The specific impact of ∆FIRE on resident macrophages is further supported by the observation, that no changes in gene expression nor cell numbers were observed in Ly6chi monocytes and inflammatory Ccr2hiLy6chi macrophages (Figure 3C, Figure 3—figure supplement 2). Together, ∆FIRE allows for investigating the role of resident cardiac macrophages that are largely of YS origin.
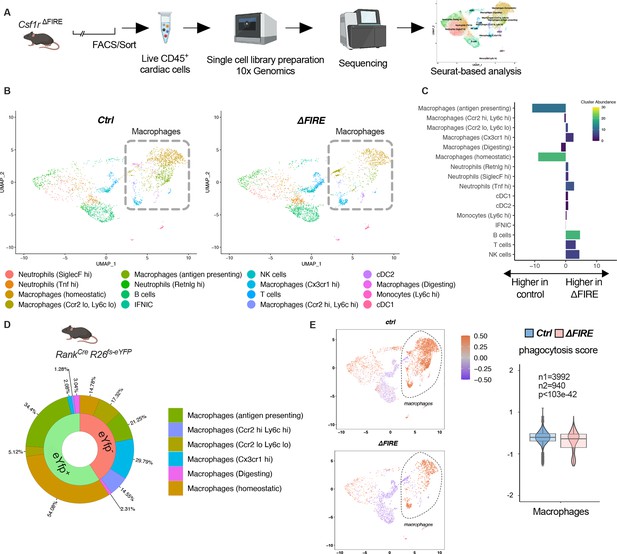
Changes in the cardiac immune phenotype in Csf1rΔFIRE mice in baseline conditions.
(A) Experimental setup to analyze cardiac immune cells using scRNA-seq of sorted CD45+/live cells. (B) UMAPs (Uniform Manifold Approximation and Projection) of control and ∆FIRE in baseline conditions (n = 3 for control and ∆FIRE). (C) Absolute difference (percentage points) in cluster abundance between control and ∆FIRE. (D) Contribution of erythro-myeloid progenitor (EMP)-derived (eYfp expressing) macrophages to the different macrophage clusters analyzed by scRNA-seq analysis of immune cells harvested from a Tnfsf11aCreRosa26fs-eYFP mouse. (E) Phagocytosis score projected on a UMAP displaying control and ∆FIRE immune cell subsets. Violin and box plots show the computed phagocytosis score combined in all macrophage clusters (n1/n2 represents number of cells from control/∆FIRE mice).
Absence of resident macrophages in ∆FIRE was associated with changes in gene expression in non-macrophage clusters such as T- and B-cells and natural killer (NK) cells (Figure 3—figure supplement 3). Furthermore, overall phagocytic capacity, as inferred by expression of phagocytosis-related genes (Amorim et al., 2022), was reduced (Figure 3E). This suggests that absence of resident macrophages is accompanied by distinct changes in immune functions in the homeostatic heart.
Adverse cardiac remodeling in Csf1r∆FIRE mice after I/R injury
To assess the impact of the absence of resident macrophages in cardiac injury, we subjected ∆FIRE mice to I/R injury and investigated remodeling and functional outcome by sequential positron emission tomography (PET) imaging after 6 and 30 days (Figure 4A). Function of the cardiac left ventricle (LV), as determined by ejection fraction (LVEF) and stroke volume (SV), improved in controls in the course of post-I/R remodeling (Figure 4B, C). In contrast, LVEF and SV remained unchanged or worsened in ∆FIRE mice, and longitudinal observations of mice indicated a negative net effect on ejection fraction from day 6 to 30 post I/R. Thus, absence of resident macrophages negatively influenced cardiac remodeling in the course of infarct healing (Figure 4D–G). Infarct size as determined by viability defect (PET) and fibrotic area (histology) was not different after 30 days (Figure 4E–H).
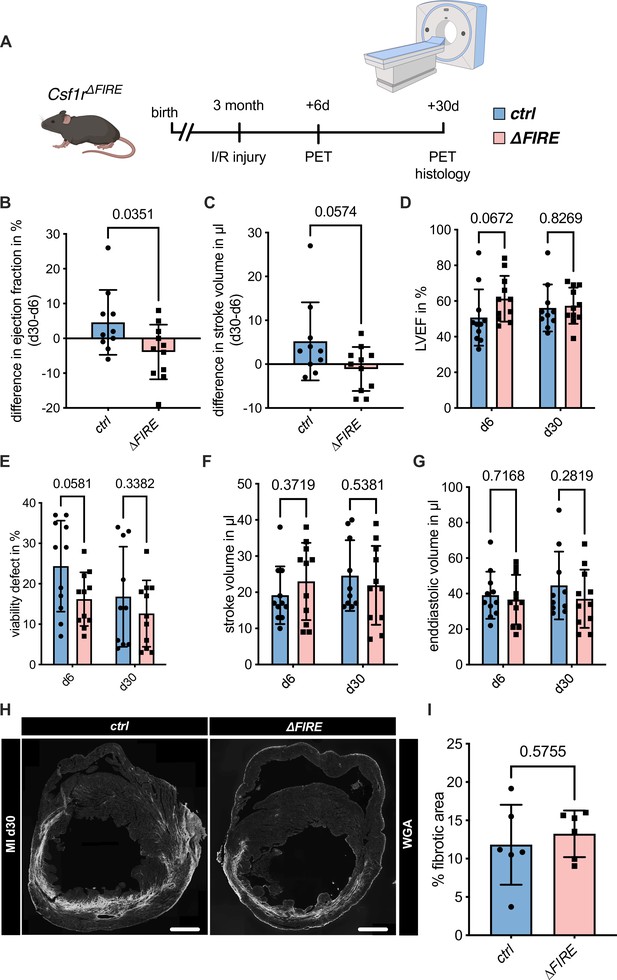
Adverse cardiac remodeling in Csf1r∆FIRE mice after ischemia/reperfusion (I/R) injury.
(A) Schematic of the sequential analysis of cardiac function, dimensions, and viability using positron emission tomography 6 and 30 days after I/R injury in control and ∆FIRE with (B, C) showing the intraindividual changes in each parameter from d6 to d30 and (D–G) the individual time points on d6 and d30 (d6: n = 11 for control and ∆FIRE, d30: n = 10 for control and n = 11 for ∆FIRE). (B, D) left ventricular ejection fraction (LVEF), (C, E) percentage of the viability defect, (F) stroke volume, and (G) left ventricular end-diastolic volume. (H) Representative immunohistological images showing the fibrotic area (WGA+ area) in hearts from control and ΔFIRE mice 30 days after I/R injury. Right panel shows the percentage of fibrotic area in the respective groups (n = 6 for each group). Student’s t-test was performed and mean ± standard deviation (SD) is shown.
Recruitment of BM-derived macrophages into infarct zone of Csf1r∆FIRE mice
To gain a deeper understanding of the inflammatory processes taking place in the infarcted heart, we quantified macrophage distribution by immunofluorescence and flow cytometry analysis of ischemic and remote areas after I/R. In ∆FIRE mice, macrophages were largely absent in the remote zone of infarcted hearts (Figure 5A), indicating sustained depletion of resident macrophages. However, macrophages strongly increased in the infarct area and their numbers were not different in both infarct and border zones between ∆FIRE and control mice (Figure 5A). This indicated recruitment and differentiation of ∆FIRE-independent macrophages from the circulation into these regions. Indeed, complementing lineage tracing of bone marrow (BM) hematopoietic stem cells (HSC) in Flt3Cre mice (Figure 5B) and YS EMP in Tnfsf11aCre mice (Figure 5C) proved the recruitment of macrophages from BM HSC (Flt3 GFP+ and Tnfsf11a RFP−, respectively) in the early phase of I/R injury. 30 days after I/R, BM-derived macrophages remained overrepresented in the infarct zone (~75% HSC contribution), and differential contribution of BM HSC declined from the border zone (~50%) to the remote zone (~35%) (Figure 5D). Taken together, recruited BM-derived macrophages represent the main population in the infarct area and their recruitment was unaltered in ∆FIRE mice. This supports the notion that resident macrophages influence cardiac remodeling but recruited macrophages drive infarct size after I/R.
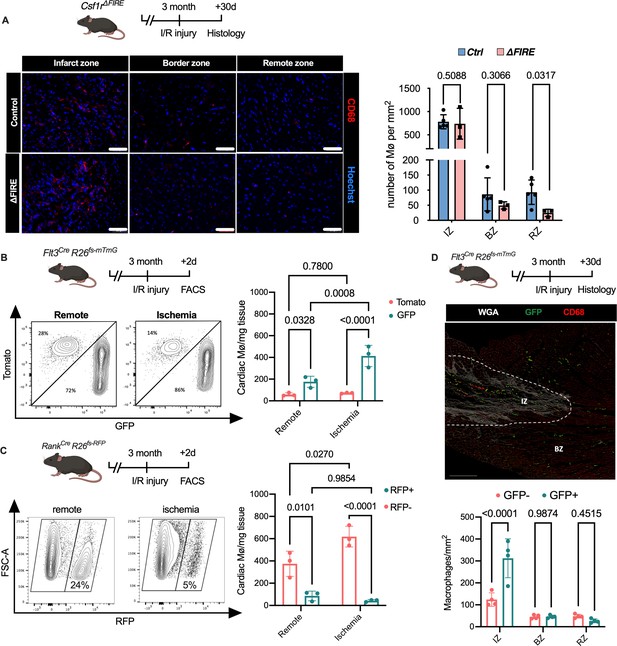
Recruitment of BM-derived macrophages into infarct zone of Csf1r∆FIRE mice.
(A) Representative immunohistology of hearts from ∆FIRE mice 30 days after ischemia/reperfusion (I/R) injury showing macrophages (CD68+ cells in red and Hoechst in blue) in the infarct, border and remote zone. Right panel shows number of cardiac macrophages in the respective area (n = 5 control and n = 3 for ∆FIRE). (B) Flow cytometry analysis of Flt3CreRosa26fs-mT/mG mice 2 days after I/R injury, (left) representative flow cytometry showing expression of tomato and GFP in macrophages in the remote and ischemic myocardium and (right) number of tomato+ and GFP+ cardiac macrophages in the respective area (n = 3, each individual experiment). (C) Flow cytometry analysis of Tnfsf11aCreRosa26RFP mice 2 days after I/R injury, (left) representative flow cytometry showing expression of RFP in macrophages in the remote and ischemic myocardium and (right) number of RFP− and RFP+ cardiac macrophages in the respective area (n = 3, each individual experiment). (D) Histological analysis of Flt3CreRosa26fs-mT/mG mice 30 days after I/R injury in the infarct, border, and remote zones, (left) representative immunohistology of the infarct and border zones and (right) number of GFP− and GFP+ cardiac macrophages in the respective areas (n = 4). Fisher’s Fisher's Least Significant Difference (LSD) test was performed for all experiments and mean ± standard deviation (SD) is shown.
Transcriptional landscape of resident versus recruited macrophages in I/R injury
To address the differential responses of resident and recruited macrophages to I/R injury, we generated BM chimeric mice. We applied an irradiation-independent model using conditional deletion of c-myb to deplete BM hematopoietic cells in CD45.2 mice and replace them with CD45.1 donor HSC (hematopoietic stem cells). Two days after I/R injury, we FACS-sorted recruited (CD45.1+) and resident (CD45.2+) macrophages and carried out bulk RNA sequencing (Figure 6A). The two macrophage populations exhibited profound transcriptional differences after I/R injury. Expression of homeostasis-related genes like Timd4, Lyve1, Cd163, and Retnla was increased in resident CD45.2+ macrophages (Figure 6B, C). Substantiating the differential regulation of macrophage programs, CD45.1+ macrophages increased inflammatory- and host defense-related gene ontology (GO) terms (e.g. myeloid leukocyte-related immunity, killing of cells), whereas CD45.2+ macrophages upregulated development- and homeostasis-related GO terms (e.g. extracellular matrix organization, vasculature development, heart muscle development) (Figure 6D). GO enrichment analysis further predicted that processes involved in innate (e.g. leukocyte migration, regulation of defense response, response to bacterium) as well as the adaptive immunity (e.g. T cell selection, T cell activation, B cell proliferation) were increased in CD45.1+ macrophages after infarction (Figure 6D). Thus, resident and recruited macrophages provide distinct transcriptional changes and inferred functions in response to I/R.
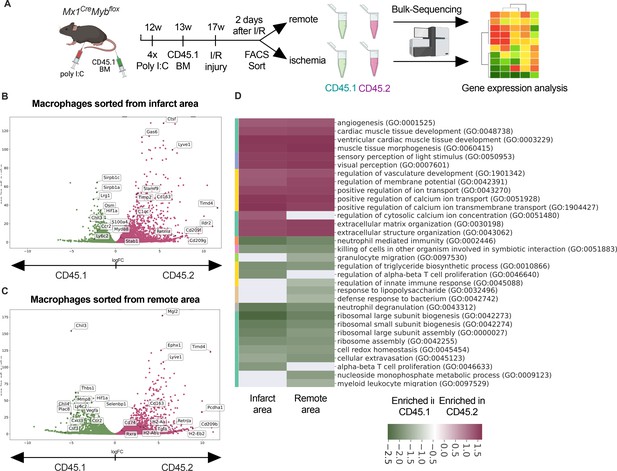
Transcriptional landscape of resident versus recruited macrophages in ischemia/reperfusion (I/R) injury.
(A) Experimental setup to generate non-irradiation BM chimera using CD45.2 Mx1CreMybflox/flox and transplantation of CD45.1 BM. I/R injury was induced 4 weeks after BM transplantation and CD45.1+ and CD45.2+ macrophages were sorted from the remote and ischemic myocardium 2 days after I/R injury and RNA-sequencing was performed on bulk cells (n = 3). Volcano plot showing differential gene expression analysis results of recruited CD45.1 versus resident CD45.2 macrophages in the (B) remote and (C) ischemic zones. (D) Gene ontology enrichment analysis showing specific biological processes enriched in CD45.1 and CD45.2 macrophages in the ischemic and remote zones.
Altered inflammatory patterns and immune cell communication in Csf1r∆FIRE mice
To evaluate the immune response of resident and recruited macrophages to I/R injury in ∆FIRE mice, we interrogated the transcription profile of CD45+ cells from the infarct area (Figure 7A). In contrast to the absence of homeostatic and antigen-presenting macrophage clusters in healthy hearts of ∆FIRE mice (Figure 3B, C), there were less differences in immune cell clusters between ∆FIRE and control mice 2 days after I/R (Figure 7). Abundance of homeostatic macrophages was also reduced at this time point, however, other clusters including Ccr2hiLy6chi inflammatory macrophages were not altered, which is in line with our histological findings.
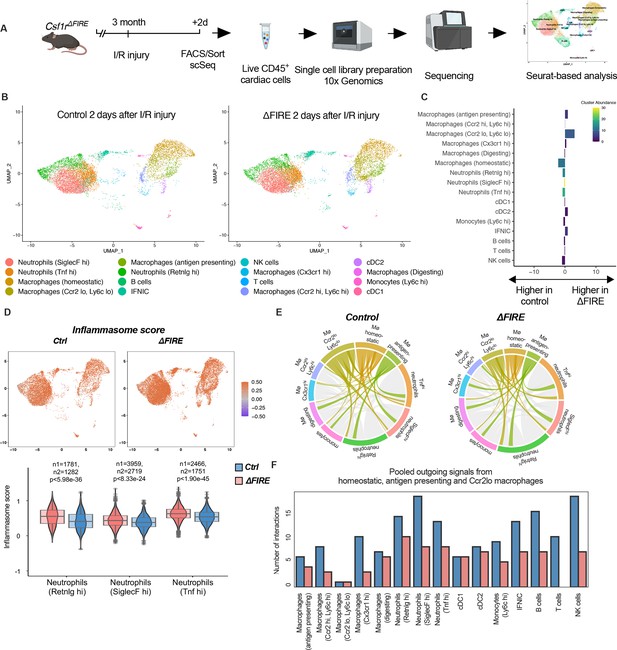
Altered inflammatory patterns and immune cell communication in Csf1r∆FIRE mice.
(A) Experimental setup to analyze transcriptional changes in cardiac immune cells on a single-cell level 2 days after ischemia/reperfusion (I/R) injury in ΔFIRE mice. (B) UMAPs of control and ∆FIRE 2 days after I/R injury (n = 2 for control and ∆FIRE). (C) Absolute difference (percentage points) in cluster abundance between control and ∆FIRE. (D) Inflammasome score projected on a UMAP displaying control and ∆FIRE immune cell subsets after I/R injury. Violin and box plots show the computed inflammasome score in neutrophil clusters (n1/n2 represents number of cells from control/ ∆FIRE mice). (E) Ligand–receptor interactions of antigen-presenting, Ccr2lo ly6clo and homeostatic macrophages (highlighted) with other immune cell clusters. Shown are the aggregated communication scores (width of interactions) for all cell types. Only communication scores larger than 6 are considered. (F) Number of interactions (with communication score >6) outgoing from homeostatic, antigen-presenting and Ccr2lo macrophages to other immune cell clusters.
∆FIRE was associated with some changes in gene expression in cardiac non-macrophage immune cells. Across different clusters, including lymphocyte and neutrophil clusters, expression of anti-inflammatory genes like Chil3 (Ym1) and Lcn2 was reduced. Vice versa, expression of Bcl-family genes like Blc2a1a and Bcl2a1d, which are associated with apoptosis and inflammatory pathways, was higher in ∆FIRE mice (Figure 7—figure supplements 1–3). Other upregulated genes were related to antigen presentation (e.g.Cd74, H2-Ab1), as identified in the Ccr2loLy6clo, homeostatic and Ccr2hiLy6chi macrophage clusters (Figure 7—figure supplements 1 and 2). Furthermore, we interrogated inflammatory gene expression in neutrophils, which are abundant first responders to myocardial infarction. We found that a computed score summing up inflammasome activation (Amorim et al., 2022) was increased in all neutrophil clusters in ∆FIRE mice (Figure 7D). Thus, the absence of cardiac macrophages was associated with altered inflammatory properties of non-macrophage immune cells in the infarcted heart.
Altered intercellular crosstalk of macrophages is a hallmark of cardiac inflammation. We therefore assessed ligand–receptor (LR) interactions between immune cell populations after I/R injury. Indeed, the number of LR interactions with neutrophils and lymphocytes, as well as the strength of the macrophage-emitted communication signals was markedly reduced in homeostatic, antigen-presenting, and Ccr2loLy6clo macrophage clusters (Figure 7E, F, Figure 7—figure supplement 4). In contrast, immune cell communication in BM-derived macrophage clusters (e.g. Ccr2hiLy6chi macrophage cluster, Figure 7—figure supplement 5) was not different to control mice. Taken together, deficiency in resident macrophages in I/R injury altered the intercellular immune crosstalk and induced a proinflammatory signature in for example cardiac neutrophils. However, transcriptional profiles of BM-derived inflammatory macrophages were largely unaltered. Together with our histological analysis showing the dominance of recruited BM-derived macrophages in the early phase of I/R, this potentially explained the limited impact of ∆FIRE on functional outcome after I/R injury.
Ablation of resident and recruited macrophages severely impacts on cardiac healing after I/R injury
To test this hypothesis, we determined the effect of combined ablation of resident and recruited macrophages. We therefore exposed mice to continuous treatment with the CSF1R-inhibitor PLX5622 (Figure 8A). In healthy hearts, inhibitor treatment resulted in the absence of cardiac macrophages within 7 days (Figure 8—figure supplement 1). We then subjected mice to I/R injury and investigated outcome by sequential PET imaging and histology (Figure 8A). Treatment with PLX5622 diminished macrophage numbers in both remote and infarct areas in the early phase (day 2) after injury. Recruitment of other myeloid cells for example neutrophils was not altered in this context (Figure 8B). This effect was pronounced in the chronic phase (day 30) after I/R injury, in which macrophages were largely absent in remote, border, and infarct zones (Figure 8C, D). Absence of resident and recruited macrophages was associated increased infarct size, as determined by fibrosis area (WGA histology) as well as viability defect (PET), and resulted in deterioration of cardiac function (Figure 8E–I). Specifically, LVEF was reduced 6 days after I/R, and remained strongly impaired at 30 days (Figure 8E).
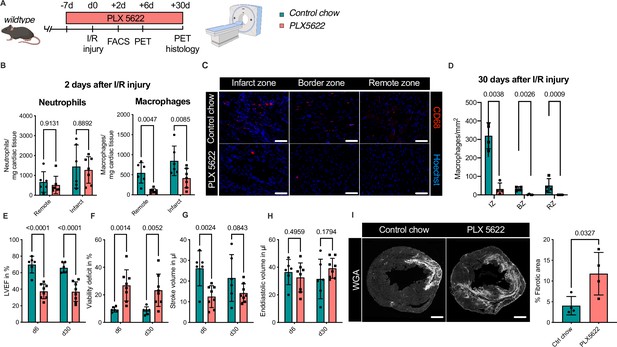
Ablation of resident and recruited macrophages severely impacts on cardiac healing after ischemia/reperfusion (I/R) injury.
(A) Schematic of the analysis of cardiac function and infarct size in mice treated with PLX5622 7 days prior and 30 days after I/R injury. (B) Number of cardiac macrophages and neutrophils in the remote and ischemic myocardium 2 days after I/R injury in mice fed control chow (n = 6) or PLX5622 (n = 7). (C) Representative immunohistology of hearts 30 days after I/R injury showing macrophages (CD68+ cells in red and Hoechst in blue) in the infarct, border, and remote zones and (D) number of cardiac macrophages in the respective area (n = 4 for control chow and n = 4 for PLX5622). (E) Left ventricular ejection fraction (LVEF), (F) viability deficit, (G) stroke volume, and (H) end-diastolic volume measured using positron emission tomography 6 and 30 days after I/R injury (n = 6 for control chow, n = 8 for PLX5622). (I) Representative immunohistological images showing the fibrotic area (WGA+ area) in hearts 30 days after I/R injury from mice fed control chow or PLX5622. Percentage of fibrotic area in the respective groups (n = 4 for each group). Student’s t-test or Fisher’s Fisher's Least Significant Difference (LSD) test was performed and mean ± standard deviation (SD) is shown.
Taken together, sole absence of resident macrophages had limited negative impact on cardiac remodeling. Absence of both resident and recruited macrophages resulted in a significant increase in infarct size and deterioration of left ventricular function after I/R injury, highlighting a beneficial effect of recruited macrophages in cardiac healing.
Discussion
Macrophages are key players in cardiac homeostasis and disease (Bajpai et al., 2019; Bajpai et al., 2018; Dick et al., 2019; Epelman et al., 2014; Hulsmans et al., 2017; Nahrendorf et al., 2007; Nicolás-Ávila et al., 2020; Panizzi et al., 2010; Sager et al., 2016). The precise understanding of their developmental origin, their functions, and their regulation could enable the identification of macrophage-targeted strategies to modify inflammation in the heart. In cardiac repair after myocardial infarction, macrophages have both positive and negative effects. They are critical for tissue repair, angiogenesis, and inflammation regulation, but their actions need to be carefully balanced to prevent excessive inflammation, scar tissue formation, and adverse remodeling. This study sheds light on the differential role of resident and recruited macrophages in cardiac remodeling and outcome after AMI.
BM-derived recruited macrophages represent a small population in the healthy heart but are recruited in vast numbers to the injured myocardium after I/R injury. These recruited cells exhibit substantially different transcriptional profiles in comparison to their resident counterpart, and show proinflammatory properties. Resident macrophages remain present in the remote and border zones and display a reparative gene expression profile after I/R injury. In comparison to recruited macrophages, resident macrophages expressed higher levels of genes related to homeostatic functions (e.g. Lyve1, Timd4, Cd163, Stab1). These markers have recently been associated with self-renewing tissue macrophages that are maintained independently of BM contribution (Dick et al., 2022). Biological processes associated with cardiac healing are upregulated in resident macrophages (e.g. regulation of vascular development, regulation of cardiomyocyte development, extracellular matrix organization). Transcriptional profiles of recruited macrophages show enhanced inflammatory biological processes also in macrophages harvested from the remote area, underlining their potentially detrimental influence on remote cardiac injury.
Two recent studies addressed the role of resident macrophages using DT-mediated macrophage depletion and reported impaired cardiac remodeling in chronic myocardial infarction (Dick et al., 2019) and after I/R injury (Bajpai et al., 2019). However, DT-mediated cell ablation is known to induce neutrophil recruitment and tissue inflammation (Frieler et al., 2015; Oh et al., 2017; Ruedl and Jung, 2018; Sivakumaran et al., 2016). This inflammatory preconditioning of cardiac tissue after DT-depletion is likely to impact on cardiac remodeling and influence assessment of tissue macrophage functions. Genomic deletion of FIRE in the Csf1r gene results in the near-absence of resident cardiac macrophages but circumvents the inflammatory stimulus of DT-induced depletion. ∆FIRE specifically diminished homeostatic and antigen-presenting macrophages. Substantiating the specificity to resident cardiac macrophages in this genetic model, the amount of macrophages recruited to the infarct area after I/R injury was not affected in ∆FIRE mice. Furthermore, scRNA-seq analysis revealed that gene expression of monocytes as well as Ccr2hiLy6chi and Cx3cr1hi Mф, representing mainly recruited immune cells, was mostly unchanged in ∆FIRE compared to control mice. The specific targeting of the resident macrophage population introduces an interesting in vivo model for studying their role in MI without the need for inflammation-prone conditional deletion of macrophages (Frieler et al., 2015).
Ablation of resident macrophages altered macrophage crosstalk to non-macrophage immune cells, especially lymphocytes and neutrophils. This was characterized by a proinflammatory gene signature, such as neutrophil expression of inflammasome-related genes and a reduction in anti-inflammatory genes like Chil3 and Lcn2 (Gordon and Martinez, 2010; Guo et al., 2014; Parmar et al., 2018). Interestingly, inflammatory polarization of neutrophils have also been associated with poor outcome after ischemic brain injury (Cuartero et al., 2013). Clinical trials in myocardial infarction patients show a correlation of inflammatory markers with extent of the myocardial damage (Sánchez et al., 2006) and with short- and long-term mortality (Mueller et al., 2002).
Our study provides evidence that the absence of resident macrophages negatively influences cardiac remodeling in the late postinfarction phase in ∆FIRE mice indicating their biological role in myocardial healing. In the early phase after I/R injury, absence of resident macrophages had no significant effect on infarct size or LV function. These observations potentially indicate a protective role in the chronic phase after myocardial infarction by modulating the inflammatory response, including adjacent immune cells like neutrophils or lymphocytes.
Deciphering in detail the specific functions of resident macrophages is of considerable interest but requires both cell-specific and temporally controlled depletion of respective immune cells in injury, which to our knowledge is not available at present. These experiments could be important to tailor immune-targeted treatments of myocardial inflammation and postinfarct remodeling.
Depletion of macrophages by pharmacological inhibition of CSF1R induced the absence of both resident and recruited macrophages, allowing us to determine cardiac outcome in juxtaposition to the ∆FIRE mice. Continuous CSF1R inhibition induced the absence of macrophages also in the infarcted area and had deleterious effect on infarct size and LV function. In line with our findings, depletion of macrophages by anti-CSF1R treatment was associated with worsened cardiac function in a model of pressure overload induced heart failure (Revelo et al., 2021). Controversially, a recent study in which monocytes were depleted using DT-injections in Ccr2DTR mice reported beneficial effects on cardiac outcome after MI in mice (Bajpai et al., 2019). An explanation of this controversy might be the timing and duration of macrophage depletion. Bajpai et al., 2019 depleted recruited macrophages only in the initial phase of myocardial infarction which improved cardiac healing, while depletion of macrophages over a longer period of time, as shown in our study, is detrimental for cardiac repair. Furthermore, other immune cells including neutrophils express CCR2 and may therefore be affected directly (Talbot et al., 2015; Xu et al., 2017). In line with this, Ccr2-deficient mice exhibit reduced acute recruitment of neutrophils to the brain after I/R injury, which was associated with reduced infarct size and brain edema (Dimitrijevic et al., 2007).
Taken together our study underlines the heterogeneity of cardiac macrophages and the importance of ontogeny therein. Resident macrophages, which mainly derive from YS EMPs, govern cardiac homeostasis in the healthy heart, and contribute positively to cardiac healing after I/R injury by orchestrating anti-inflammatory programming of other cardiac immune cells. However, recruited macrophages contribute to the healing phase after AMI and their absence defines infarct size and cardiac outcome.
Materials and methods
Mice
Tnfsf11aCre (Jacome-Galarza et al., 2019; Percin et al., 2018), Flt3Cre (Benz et al., 2008), Mybfl/fl (Emambokus et al., 2003), Mx1Cre (Kühn et al., 1995) (from The Jackson Laboratory (JAX), Stock No: 003556), Csf1rΔFIRE (Rojo et al., 2019), Rosa26fs-DTR (Buch et al., 2005) (JAX Stock No: 007900), Rosa26fs-mT/mG (Muzumdar et al., 2007) (JAX Stock No: 007676), Rosa26fs-eYFP (Srinivas et al., 2001) (JAX Stock No: 006148), and Rosa26fs-RFP (JAX Stock No: 034720) mice have been previously described. PCR genotyping was performed according to protocols described previously. Animals were aged between 10 and 16 weeks. We have complied with all relevant ethical regulations. Animal studies were approved by the local regulatory agency (Regierung von Oberbayern, Munich, Germany, record numbers ROB-55.2-2532.Vet_02-19-17 and ROB-55.2-2532.Vet_02-19-1; Care and Use Committee of the Institut Pasteur (CETEA), dap190119).
FIRE mice were kept on a CBA/Ca background. Experiments in which reporter mice were necessary (Csf1rΔFIRETnfsf11aCreRosa26fs-eYFP) the background was mixed with a C57Bl6 background. All experiments evaluating cardiac function and outcome after infarction in FIRE mice were performed on mice kept with a CBA/Ca background.
For fate-mapping analysis of Flt3+ precursors, Flt3Cre males (the transgene is located on the Y chromosome) were crossed to Rosa26fs-eYFP or Rosa26fs-mT/mG female reporter mice and only the male progeny was used. For all other experiments, the offspring of both sexes was used for experiments.
BM transplantation was enabled by conditional deletion of the transcription factor myb as previously described (Stremmel et al., 2018). In brief, we induced BM ablation by four injections of polyI:C into CD45.2; Mx1CreMybflox/flox every other day, and then transplanted BM from congenic C57BL/6 CD45.1 (Ly5.1; JAX Stock No: 006584). We confirmed the success (chimerism of above 90%) of the BM transplantation after 4 weeks.
To deplete macrophages, we used the selective CSF1R-inhibitor PLX5622. Control and PLX5622 (300 ppm formulated in AIN-76A standard chow, Research Diets, Inc) chows were kindly provided by Plexxikon Inc (Berkeley, CA). For depletion of embryonic macrophages, Tnfsf11aCreRosa26fs-DTR mice were injected intraperitoneally with 0.02 mg/kg body weight DT (Sigma-Aldrich) at the time points mentioned in the figure and corresponding legend. Mice were closely monitored by veterinarians and according to score sheets that were approved by the regulatory agency. Single DT application in Tnfsf11aCreRosa26fs-DTR mice let to rapid deterioration of their health (without infarction or other interventions), and consequently experiments needed to be aborted according to regulations; experimental outcomes were then documented as premature death as indicated.
Ischemia–reperfusion (I/R) injury
Request a detailed protocolI/R injury was carried out as previously described (Novotny et al., 2018). In brief, mice were anesthetized using 2% isoflurane and intraperitoneal injection of fentanyl (0.05 mg/kg), midazolam (5.0 mg/kg), and medetomidine (0.5 mg/kg), and then intubated orally (MiniVent Ventilator model nr. 845, Harvard Apparatus) and ventilated (volume of 150 µl at 200 /min). After lateral thoracotomy, the left anterior descending artery (LAD) was ligated with an 8–0 prolene suture producing an ischemic area in the apical LV. To induce the reperfusion injury, the suture was removed after 60 min and reperfusion was confirmed by observing the recoloring of the LV. Postoperative analgesia was performed by injection of Buprenorphin (0.1 mg/kg) twice per day for 3 days. After 2, 6, or 30 days after I/R injury organs were harvested after cervical dislocation.
Organ harvest
Request a detailed protocolMice were anesthetized using 2% isoflurane and organ harvest was performed after cervical dislocation. Blood was harvested with a heparinized syringe (2 ml) by cardiac puncture. After perfusion with 20 ml of ice-cold phosphate-buffered saline (PBS) hearts were excised and kept in PBS on ice until further tissue processing. For flow cytometry, hearts were divided into the ischemia (tissue distal of the LAD ligation) and remote area (tissue proximal of the LAD ligation). For histological examinations of tissues, hearts were incubated in 4% paraformaldehyde for 30 min followed by an incubation in 30% sucrose solution (Sigma-Aldrich) for 24 hr. Afterwards, hearts were mounted onto a heart slicing device and cut transversally into three equal parts (termed levels 1, 2, and 3) and stored in Tissue-Tek (Sakura Finetek Germany GmbH) at −80°C.
Immunohistology
Request a detailed protocolCryosections (10–12 µm) of heart tissue were fixed with 4% paraformaldehyde for 10 min. Blocking and permeabilization were performed with 0.5% Saponin and 10% goat serum for 1 hr. Primary antibodies were added and incubated for 2–18 hr (see Table S1 - Supplementary file 1). Slides were washed with PBS and secondary antibodies were added and incubated for 1 hr (see Table S1 - Supplementary file 1). WGA-staining (Wheat Germ Agglutinin Alexa Fluor 647 conjugated antibody, Thermo Fisher Scientific) was used to locate and measure the infarct area and nuclei were stained with 4′,6-diamidino-2-phenylindole (DAPI). Finally, slides were washed one more time and Fluorescence Mounting Medium was used to cover the stained sample.
Heart samples were evaluated using an Axio Imager M2 (Carl Zeiss) and blinded picture analysis was performed using ZEN Imaging and Axiovision SE64 Rel. 4.9.1 (Carl Zeiss). For the evaluation of cell numbers, six individual high-resolution images from each respective anatomical region (infarct area, border zone, and remote zone) were analyzed for each animal. To measure infarct size the heart was cut into three parts and the infarcted area was measured as WGA+ area in sections from each part.
Flow cytometry
Request a detailed protocol100 µl of heparinized blood was used for FACS analysis. Erythrocytes were lysed with 1% ammonium chloride. After washing with PBS, the cell suspension was resuspended in purified rat anti-mouse CD16/CD32 (BD Pharmingen) and incubated for 15 min at 4°C. Following this, cells were incubated with FACS antibodies for 15 min at 4°C (Supplementary file 1).
Heart tissue was dissected into remote and ischemic tissue as described above and minced into small pieces using forceps and a scalpel. When comparing baseline and I/R injury in FACS analysis, basal heart tissue was used for comparison with remote tissue and apical heart tissue for comparison with ischemia tissue. After enzymatic digestion (Collagenase XI 1200 U/mg, Collagenase I 125 U/mg, Hyaluronidase 500 U/mg, DNase I 1836 U/mg; Sigma-Aldrich) for 30 min at 37°C cells were washed and incubated with purified anti-CD16/32 (FcγRIII/II; dilution 1/50) for 10 min. Thereafter, cells were incubated with FACS antibodies (Supplementary file 1) for 30 min at 4°C. FACS analysis was performed on a BD Fortessa or a BeckmanCoulter Cytoflex flow cytometer and gating strategies are shown in Figure 2—figure supplement 1. Data were analyzed using FlowJo (version 10.0.8r1).
Cell sorting
Request a detailed protocolCell sorting was performed on a MoFlo Astrios (Beckman Coulter) to obtain cardiac macrophages from CD45.2; Mx1CreMybflox/flox after BM transplantation of CD45.1 BM (n = 3 for 2 days after I/R injury) for bulk sequencing, or all cardiac immune cells (CD45+ cells) of Csf1rΔFIRE and Tnfsf11aCreRosa26fs-eYFP mice (n = 3 for Csf1r∆FIRE/+ and Csf1r∆FIRE/∆FIRE in baseline conditions, n = 1 for Tnfsf11aCreRosa26fs-eYFP in baseline conditions; n=2 for Csf1r∆FIRE/+ and Csf1r∆FIRE/∆FIRE 2 days after I/R injury) for single-cell analysis. CD45+ cells were enriched by using magnetic beads and MS columns (CD45 MicroBeads; Miltenyi Biotec). Cells were then sorted as single/live/CD45+ cells for single-cell sequencing from baseline hearts and from the ischemic myocardium 2 days after I/R injury. Bulk sequencing was performed on single/live/CD45+/lin−/CD11b+/F4/80+/CD64+/CD45.1+ or CD45.2+ cells from the remote and the ischemic myocardium 2 days after I/R injury. Dead cells were identified with SYTOX Orange Dead Cell Stain.
Bulk sequencing and analysis
Request a detailed protocolFor each sample, ~1000 macrophages were sorted into 75 µl of RLT buffer (QIAGEN, containing 1% beta-mercaptoethanol), vortexed for 1 min and immediately frozen (−80°C). RNA extraction (RNeasy Plus Micro Kit, QIAGEN), cDNA generation (SMART-Seq v4 Ultra Low Input RNA Kit, Takara Bio), and library preparation (Nextera XT DNA Library Prep Kit, Illumina) were performed according to the manufacturer’s specifications. Sequencing was performed on a HiSeq4000 system (Ilumina).
The obtained reads were trimmed using bbduk from the BBMap (https://sourceforge.net/projects/bbmap/) v38.87 collection using parameters “ktrim = r k=23 mink = 11 hdist = 1 tpe tbo”. The trimmed reads were aligned with Hisat2 2.2.1 against the Ensembl release 102 reference mouse genome (Yates et al., 2020).
Gene expression was quantified using the featureCounts (Liao et al., 2014) application from the subread package (v2.0.1) and with parameters ‘--primary -O -C -B -p -T 8 --minOverlap 5’. Differential expression analysis was performed using DESeq2 (v1.30.0) (Love et al., 2014). A gene ontology set enrichment analysis was performed using the ClusterProfiler R package (v3.18.1). The visualization of the respective clustermaps was layouted using the ForceAtlas2 implementation of the python fa2 (https://pypi.org/project/fa2/) package for networkx (v2.5) (https://networkx.org/).
On the library-size normalized count data, the pymRMR (Peng et al., 2005, https://pypi.org/project/pymrmr/) package was used to derive the top 100 discriminatory genes (Mutual Information Quotient method) for subsequently calculating the UMAP 2D-embedding (McInnes et al., 2020, https://pypi.org/project/umap-learn/) for all samples (umap-learn package v0.5.0rc1, 3 neighbors) (McInnes et al., 2018, https://pypi.org/project/pymrmr/).
Single-cell RNA sequencing and analysis
Request a detailed protocolAfter sorting, cells were proceeded for single-cell capture, barcoding and library preparation using Chromium Next GEM single cell 3′ (v3.1, 10× Genomics) according to the manufacturer’s specifications. Pooled libraries were sequenced on an Illumina HiSeq1500 sequencer (Illumina, San Diego, USA) in paired-end mode with asymmetric read length of 28 + 91 bp and a single indexing read of 8 bp.
The reads of heart1 sample (Tnfsf11aCreRosa26fs-eYFP) were demultiplexed using Je-demultiplex-illu (Girardot et al., 2016) and mapped against a customized mouse reference genome (GRCm38.p6, Gencode annotation M24) including eYfp sequence using CellRanger (v3.1.0, 10× Genomics).
The six mouse samples 20133-0001 to 20133-0006 (baseline condition of Csf1r∆FIRE/+ and Csf1r∆FIRE/∆FIRE), were processed using Cellranger 4.0.0 using the 2020 A mm10 reference.
The four mouse samples (MUC13956-13959, infarct condition of Csf1r∆FIRE/+ and Csf1r∆FIRE/∆FIRE) were sequenced with four technical sequencing replicates and pooled using the cellranger (v4.0.0, 10× Genomics) pipeline. Cellranger 4.0.0 was called with default parameters and the 2020 A mm10 reference for gene expression.
Finally, all 11 samples were integrated using Seurat 4.0.0 (on R 4.0.1) (Stuart and Satija, 2019). The samples were processed, and cells were filtered to contain between 200 and 6000 features, have at least 1000 molecules detected (nCount_RNA >1000), have below 15% mtRNA content (^MT) and below 40% ribosomal RNA content (^Rps|^Rpl). After this filtering a total of 35,759 cells remained.
After performing SCTransform (Hafemeister and Satija, 2019) on the samples, the SCTransform vignette for integrating the datasets was followed (with 2000 integration features). For dimensionality reduction, PCA was performed using default parameters, and UMAP and Neighbour-Finding was run on 50 PCs. Clustering was performed at a resolution of 0.8. A total of 18 clusters was identified using this approach. Cluster markers were calculated using the t-test in the FindMarkers function. Subsequently, cell types were initially predicted using the cPred cell-type prediction (https://github.com/mjoppich/scrnaseq_celltype_prediction, copy archived at Joppich, 2023a). Upon manual curation, further fine-grained cell-type annotations were made. Differential comparisons were performed against several subgroups of the dataset. These comparisons were performed using the t-test in the FindMarkers function of Seurat. Differential results are visualized using the EnhancedVolcano library (Blighe and Lewis, 2021).
For the analysis of cell–cell interactions we downloaded the LR pairs from Jin et al., 2021 from the Lewis Lab GitHub repository (https://github.com/LewisLabUCSD/Ligand-Receptor-Pairs, copy archived at Armingol, 2024). For each interaction (LR pair for a cluster pair) the communication score is calculated as the expression product Armingol et al., 2021 of the mean normalized expressions exported from the Seurat object. This ensures that little expression of either ligand or receptor in only one cluster results in a relatively low communication score, and only good expression of ligand and receptor will result in a high communication score. The direction of an interaction is fixed from ligand to receptor. The single LR communication scores were then aggregated (sum) such that only interactions with a score greater 6 were taken into account.
Gene module scores for inflammasome, reactive oxygen species (ROS), and phagocytosis gene sets were calculated using Seurat’s AddModuleScore function. All scripts, including the ones for creating the visualizations of bulk and scRNA-seq data, are available online through https://github.com/mjoppich/myocardial_infarction, copy archived at Joppich, 2023b.
In vivo PET imaging
Request a detailed protocolElectrocardiogram (ECG)-gated PET images were performed on days 6 and 30 after I/R injury of the LAD using a dedicated small-animal PET scanner (Inveon Dedicated PET, Preclinical Solutions, Siemens Healthcare Molecular Imaging, Knoxville, TN, USA), as previously described (Brunner et al., 2012). Anaesthesia was induced with isoflurane (2.5%), delivered via a face mask in pure oxygen at a rate of 1.2 l/min and maintained with isoflurane (1.5%). Approximately 15 MBq 2-deoxy-2-[18F]fluoro-D-glucose ([18F]-FDG) (~100 μl) were administered through a teil vein catheter and slowly flushed immediately afterwards with 50 μl saline solution. Body temperature was monitored using a rectal thermometer and maintained within the normal range using a heating pad. After placing animals within the aperture of the PET scanner ECG electrodes (3 M, St. Paul, MN, USA) were placed on both forepaws and the left hind paw and ECG was recorded using a dedicated physiological monitoring system (BioVet; Spin Systems Pty Ltd, Brisbane, Australia) (Todica et al., 2018). The PET emission acquisition (list-mode) was initiated 30 min after [18F]-FDG injection and lasted 15 min (Brunner et al., 2012; Gross et al., 2016). For scatter and attenuation correction and additional 7-min long transmission scan was performed using a Co-57 source.
The accuracy of the ECG trigger signal was verified retrospectively using in-house software programmed in MATLAB (The Mathworks, Natick, USA) and in C programming language and erroneous trigger events were removed when needed, as previously described (Böning et al., 2013). Further processing of the data was performed using the Inveon Acquisition Workplace (Siemens Medical Solutions, Knoxville, TN). As previously described, data were reconstructed as a static image or as a cardiac gated image with 16 bins in a 128 × 128 matrix with a zoom of 211% using an OSEM 3D algorithm with 4 and a MAP 3D algorithm with 32 iterations (Brunner et al., 2012). The reconstructed data were normalized, corrected for randoms, dead time, and decay as well as attenuation and scatter.
PET images were analyzed using the Inveon Research Workplace in a blinded manner (Siemens Medical Solutions, Knoxville, TN). Infarct sizes were determined from static reconstructed images using QPS (Cedars-Sinai, Los Angeles, CA, USA). Hereby, datasets were compared to a normative database and the viability defect was calculated as percentage of the left ventricular volume, as described previously (Lehner et al., 2014a; Lehner et al., 2014b). Left ventricular function volumes (end-diastolic volume (EDV), end-systolic volume (ESV), and stroke volume (SV)), as well as the LVEF, were determined from ECG-gated images using QGS (Cedars-Sinai, Los Angeles, CA, USA), as described previously (Brunner et al., 2012; Croteau et al., 2003).
Statistical analysis
Request a detailed protocolStudent’s t-test or Fisher’s Fisher's Least Significant Difference (LSD) test was used (Prism GraphPad). Welch’s correction for unequal variances was used when applicable. A p-value of <0.05 was considered significant. The Shapiro–Wilk test was used to test normality. Data are presented as mean ± standard deviation.
Data availability
Sequencing data have been deposited in GEO under accession codes GSE263544, GSE263545.
-
NCBI Gene Expression OmnibusID GSE263544. Resident and recruited macrophages differentially contribute to cardiac healing after myocardial ischemia [bulk RNA-seq].
-
NCBI Gene Expression OmnibusID GSE263545. Resident and recruited macrophages differentially contribute to cardiac healing after myocardial ischemia [scRNA-seq].
References
-
Deciphering cell-cell interactions and communication from gene expressionNature Reviews. Genetics 22:71–88.https://doi.org/10.1038/s41576-020-00292-x
-
SoftwareLigand-receptor-pairs, version swh:1:rev:c0ce8167c20c2f5d1a3d309e04514da9a178c41cSoftware Heritage.
-
The stream of precursors that colonizes the thymus proceeds selectively through the early T lineage precursor stage of T cell developmentThe Journal of Experimental Medicine 205:1187–1199.https://doi.org/10.1084/jem.20072168
-
Quantitative gated PET for the assessment of left ventricular function in small animalsJournal of Nuclear Medicine 44:1655–1661.
-
FDG-PET reveals improved cardiac regeneration and attenuated adverse remodelling following Sitagliptin + G-CSF therapy after acute myocardial infarctionEuropean Heart Journal. Cardiovascular Imaging 17:136–145.https://doi.org/10.1093/ehjci/jev237
-
Lipocalin 2 is a regulator of macrophage polarization and NF-κB/STAT3 pathway activationMolecular Endocrinology 28:1616–1628.https://doi.org/10.1210/me.2014-1092
-
Resident and monocyte-derived macrophages in cardiovascular diseaseCirculation Research 122:113–127.https://doi.org/10.1161/CIRCRESAHA.117.311071
-
Inference and analysis of cell-cell communication using CellChatNature Communications 12:1088.https://doi.org/10.1038/s41467-021-21246-9
-
SoftwareMyocardial infarction, version swh:1:rev:001d5870172113d2b3f9bb675719aed1a23ef006Software Heritage.
-
Matrix metalloproteinase-12 produced by Ly6Clow macrophages prolongs the survival after myocardial infarction by preventing neutrophil influxJournal of Molecular and Cellular Cardiology 131:41–52.https://doi.org/10.1016/j.yjmcc.2019.04.007
-
UMAP: uniform manifold approximation and projectionJournal of Open Source Software 3:861.https://doi.org/10.21105/joss.00861
-
The healing myocardium sequentially mobilizes two monocyte subsets with divergent and complementary functionsThe Journal of Experimental Medicine 204:3037–3047.https://doi.org/10.1084/jem.20070885
-
Impaired infarct healing in atherosclerotic mice with Ly-6C(hi) monocytosisJournal of the American College of Cardiology 55:1629–1638.https://doi.org/10.1016/j.jacc.2009.08.089
-
Lipocalin 2 plays an important role in regulating inflammation in retinal degenerationJournal of Immunology 200:3128–3141.https://doi.org/10.4049/jimmunol.1701573
-
Feature selection based on mutual information: criteria of max-dependency, max-relevance, and min-redundancyIEEE Transactions on Pattern Analysis and Machine Intelligence 27:1226–1238.https://doi.org/10.1109/TPAMI.2005.159
-
Cardiac resident macrophages prevent fibrosis and stimulate angiogenesisCirculation Research 129:1086–1101.https://doi.org/10.1161/CIRCRESAHA.121.319737
-
DTR-mediated conditional cell ablation-Progress and challengesEuropean Journal of Immunology 48:1114–1119.https://doi.org/10.1002/eji.201847527
-
Kinetics of C-reactive protein release in different forms of acute coronary syndromeRevista Espanola de Cardiologia 59:441–447.https://doi.org/10.1016/S1885-5857(06)60792-5
-
Inducible disruption of the c-myb gene allows allogeneic bone marrow transplantation without irradiationJournal of Immunological Methods 457:66–72.https://doi.org/10.1016/j.jim.2018.03.016
-
Integrative single-cell analysisNature Reviews. Genetics 20:257–272.https://doi.org/10.1038/s41576-019-0093-7
-
CCR2 expression in neutrophils plays a critical role in their migration into the joints in rheumatoid arthritisArthritis & Rheumatology 67:1751–1759.https://doi.org/10.1002/art.39117
-
Myocardial infarction: a critical role of macrophages in cardiac remodelingFrontiers in Physiology 6:107.https://doi.org/10.3389/fphys.2015.00107
-
CCR2 dependent neutrophil activation and mobilization rely on TLR4-p38 axis during liver ischemia-reperfusion injuryAmerican Journal of Translational Research 9:2878–2890.
Article and author information
Author details
Funding
Deutsches Zentrum für Herz-Kreislaufforschung (81Z0600204)
- Christian Schulz
Deutsches Zentrum für Herz-Kreislaufforschung (81X2600252)
- Tobias Weinberger
Deutsches Zentrum für Herz-Kreislaufforschung (81X2600256)
- Maximilian Fischer
Deutsche Forschungsgemeinschaft (SCHU 2297/1-1)
- Christian Schulz
Deutsche Forschungsgemeinschaft (SFB 1123 project Z02)
- Ralf Zimmer
Deutsche Forschungsgemeinschaft (SFB 1123 project A07)
- Christian Schulz
Deutsche Forschungsgemeinschaft (SFB TRR332, project A06)
- Christian Schulz
LMUexcellent
- Tobias Weinberger
European Society of Cardiology (Research Grant 2021)
- Tobias Weinberger
Friedrich-Baur Stiftung
- Tobias Weinberger
Deutsche Stiftung für Herzforschung
- Maximilian Fischer
Chinese Scholarship Council
- Jiahui Fang
- Lulu Liu
The funders had no role in study design, data collection, and interpretation, or the decision to submit the work for publication.
Acknowledgements
We thank Nicole Blount, Beate Jantz, Michael Lorenz, and Sebastian Helmer for excellent technical assistance. DZHK (German Centre for Cardiovascular Research) and the BMBF (German Ministry of Education and Research) (grants 81Z0600204 to CS, 81X2600252 to TW and 81X2600256 to MF). The Deutsche Forschungsgemeinschaft SCHU 2297/1-1 and collaborative research centers 1123 project Z02 (MJ, RZ) and A07 (CS), and CRC TRR332 project A6 (CS). LMUexcellent (TW). ESC Research Grant 2021 (TW). Friedrich-Baur Stiftung (TW). Deutsche Stiftung für Herzforschung (MF). Chinese Scholarship Council (CSC) to JF and LL.
Ethics
Animal studies were approved by the local regulatory agency (Regierung von Oberbayern, Munich, Germany, record numbers ROB-55.2-2532.Vet_02-19-17 and ROB-55.2-2532.Vet_02-19-1; Care and Use Committee of the Institut Pasteur (CETEA), dap190119).
Version history
- Sent for peer review: June 9, 2023
- Preprint posted: June 19, 2023 (view preprint)
- Preprint posted: August 2, 2023 (view preprint)
- Preprint posted: January 19, 2024 (view preprint)
- Preprint posted: March 4, 2024 (view preprint)
- Version of Record published: May 22, 2024 (version 1)
- Version of Record updated: May 29, 2024 (version 2)
Cite all versions
You can cite all versions using the DOI https://doi.org/10.7554/eLife.89377. This DOI represents all versions, and will always resolve to the latest one.
Copyright
© 2023, Weinberger et al.
This article is distributed under the terms of the Creative Commons Attribution License, which permits unrestricted use and redistribution provided that the original author and source are credited.
Metrics
-
- 1,667
- views
-
- 158
- downloads
-
- 1
- citations
Views, downloads and citations are aggregated across all versions of this paper published by eLife.
Download links
Downloads (link to download the article as PDF)
Open citations (links to open the citations from this article in various online reference manager services)
Cite this article (links to download the citations from this article in formats compatible with various reference manager tools)
Further reading
-
- Developmental Biology
- Structural Biology and Molecular Biophysics
The receptor tyrosine kinase ROR2 mediates noncanonical WNT5A signaling to orchestrate tissue morphogenetic processes, and dysfunction of the pathway causes Robinow syndrome, brachydactyly B, and metastatic diseases. The domain(s) and mechanisms required for ROR2 function, however, remain unclear. We solved the crystal structure of the extracellular cysteine-rich (CRD) and Kringle (Kr) domains of ROR2 and found that, unlike other CRDs, the ROR2 CRD lacks the signature hydrophobic pocket that binds lipids/lipid-modified proteins, such as WNTs, suggesting a novel mechanism of ligand reception. Functionally, we showed that the ROR2 CRD, but not other domains, is required and minimally sufficient to promote WNT5A signaling, and Robinow mutations in the CRD and the adjacent Kr impair ROR2 secretion and function. Moreover, using function-activating and -perturbing antibodies against the Frizzled (FZ) family of WNT receptors, we demonstrate the involvement of FZ in WNT5A-ROR signaling. Thus, ROR2 acts via its CRD to potentiate the function of a receptor super-complex that includes FZ to transduce WNT5A signals.
-
- Cell Biology
- Developmental Biology
The generation of distinct cell fates during development depends on asymmetric cell division of progenitor cells. In the central and peripheral nervous system of Drosophila, progenitor cells respectively called neuroblasts or sensory organ precursors use PAR polarity during mitosis to control cell fate determination in their daughter cells. How polarity and the cell cycle are coupled, and how the cell cycle machinery regulates PAR protein function and cell fate determination is poorly understood. Here, we generate an analog sensitive allele of CDK1 and reveal that its partial inhibition weakens but does not abolish apical polarity in embryonic and larval neuroblasts and leads to defects in polarisation of fate determinants. We describe a novel in vivo phosphorylation of Bazooka, the Drosophila homolog of PAR-3, on Serine180, a consensus CDK phosphorylation site. In some tissular contexts, phosphorylation of Serine180 occurs in asymmetrically dividing cells but not in their symmetrically dividing neighbours. In neuroblasts, Serine180 phosphomutants disrupt the timing of basal polarisation. Serine180 phosphomutants also affect the specification and binary cell fate determination of sensory organ precursors as well as Baz localisation during their asymmetric cell divisions. Finally, we show that CDK1 phosphorylates Serine-S180 and an equivalent Serine on human PAR-3 in vitro.