The ORP9-ORP11 dimer promotes sphingomyelin synthesis
Figures
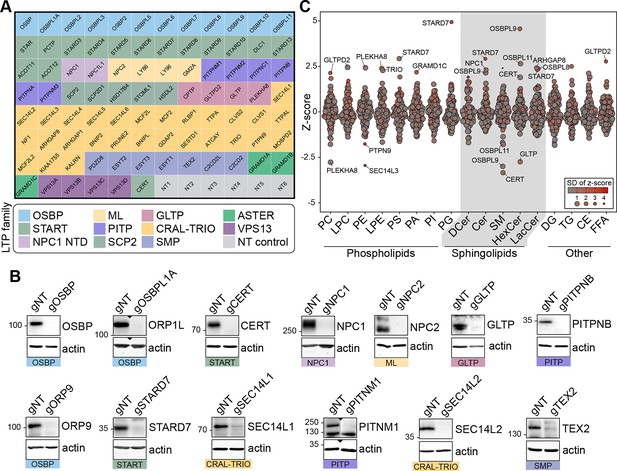
Lipidomics analysis of a lipid transfer protein (LTP) knockout library revealed known and candidate controllers of cellular lipid levels.
(A) Overview of the arrayed gene knockout library targeting LTPs. NT: non-targeting. (B) Western blotting of the selected wells showing the efficiency of the LTP knockout library on MelJuSo cells. gNT: Non-targeting, control cells. (C) Summary plot of the lipidomics analysis: Z-scores calculated for 17 lipid classes are plotted. Each data point represents the mean Z-score of an LTP knockout cell line; size and color of data points represent the standard deviation of Z-scores for three experiments on average. PC: phosphatidylcholine, LPC: lyso-phosphatidylcholine, PE: phosphatidylethanolamine, LPE: lyso-phosphatidylethanolamine, PS: phosphatidylserine, PI: phosphatidylinositol, PA: phosphatidic acid, PG: phosphatidylglycerol, DCer: dihydroxyceramide, Cer: ceramide, SM: sphingomyelin, HexCer: hexosylceramide, LacCer: lactosylceramide, DG: diacylglycerol, TG: triacylglycerol, CE: cholesterol ester, FFA: free fatty acids.
-
Figure 1—source data 1
Raw unedited gels for Figure 1.
- https://cdn.elifesciences.org/articles/91345/elife-91345-fig1-data1-v1.zip
-
Figure 1—source data 2
Uncropped and labeled gels for Figure 1.
- https://cdn.elifesciences.org/articles/91345/elife-91345-fig1-data2-v1.zip
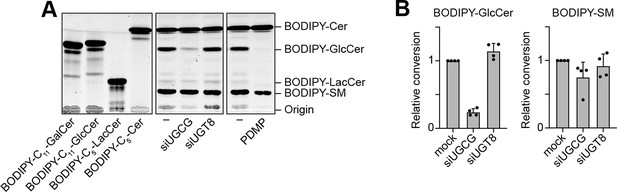
Glucosylceramide is the main hexosylceramide in MelJuSo cells.
(A) MelJuSo cells silenced for glucosylceramide synthase (siUGCG) or galactosylceramide synthase (siUGT8) or treated with the glucosylceramide synthase inhibitor (PDMP) were fed with the fluorescent ceramide analog BODIPY-C5-ceramide. Lipids were isolated and separated by thin-layer chromatography. Note that the fluorescent band corresponding to monohexosylceramide is affected only when glucosylceramide synthesis is perturbed. (B) Quantifications corresponding to panel A. Bars and error bars correspond to mean and standard deviations from four experiments, respectively.
-
Figure 1—figure supplement 1—source data 1
Raw unedited gels for Figure 1—figure supplement 1.
- https://cdn.elifesciences.org/articles/91345/elife-91345-fig1-figsupp1-data1-v1.zip
-
Figure 1—figure supplement 1—source data 2
Uncropped and labeled gels for Figure 1—figure supplement 1.
- https://cdn.elifesciences.org/articles/91345/elife-91345-fig1-figsupp1-data2-v1.zip
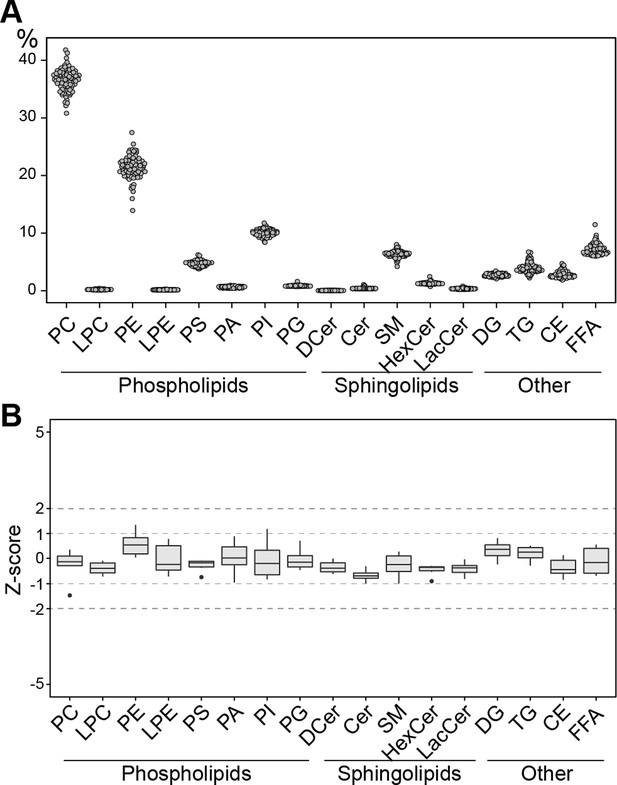
Overview of lipidomics analysis performed for lipid transfer protein (LTP) knockout library.
(A) Percentage distribution of 17 lipid classes in LTP knockout cells; each data point represents the mean percentage of an LTP knockout cell line. (B) Box plots of Z-scores of NT control cells. Note that Z-scores for NT control cells do not exceed the absolute value of 2. In boxplots, middle line denotes median, box boundaries denote the first (Q1) and third (Q3) quartiles, and the lower and upper whiskers denote ‘Q1-1.5*IQR’ and ‘Q3+1.5*IQR,’ respectively. IQR: inter-quartile range. PC: phosphatidylcholine, LPC: lysophosphatidylcholine, PE: phosphatidylethanolamine, LPE: lysophosphatidylethanolamine, PS: phosphatidylserine, PA: phosphatidic acid, PG: phosphatidylglycerol, PI: phosphatidylinositol, DCer: dihydroceramide, Cer: ceramide, SM: sphingomyelin, HexCer: hexosyl-ceramide, LacCer: lactosylceramide, DG: diacylglycerol, TG: triacylglycerol, CE: cholesterol ester, and FFA: free fatty acid.
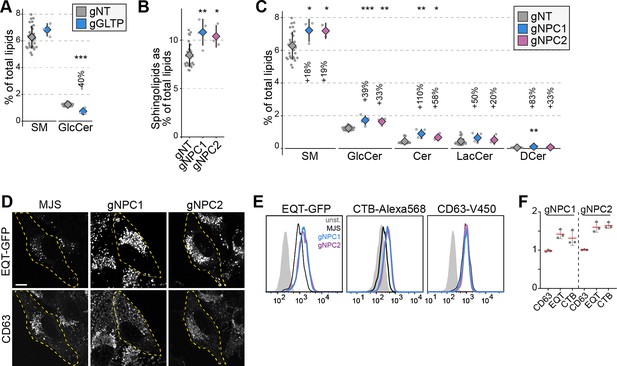
NPC1 and NPC2 knockout cells accumulate sphingomyelin in lysosomes.
(A) GLTP knockouts show decreased glucosylceramide levels compared to control cells. GlcCer: glucosylceramide (B) NPC1 and NPC2 knockout cells have increased sphingolipids levels compared to control cells. (C) Increased sphingolipid levels in NPC1 and NPC2 knockouts are represented in all sphingolipid classes. (D) Immunofluorescence images of NPC1 and NPC2 knockout cells permeabilized and stained with recombinant GFP-EQT show the accumulation of sphingomyelin in the lysosomes compared to the parental MelJuSo (MJS) cells. Pixel intensities of the images were adjusted evenly. Scale bar 10 µm. (E) Flow cytometry analysis of cells stained with EQT-GFP, CTB-Alexa568, and CD63-V450 on the cell surface. Analysis demonstrates the accumulation of sphingomyelin (EQT-GFP) and the glycosphingolipid GM1 (CTB-Alexa568) on the cell surface without affecting the surface protein levels. (F) Mean fluorescence intensities for each staining normalized to control cells from three experiments. Approximately 2000 cells were analyzed per experiment. Red lines and diamonds correspond to mean mean, and the error bars denote in all figures.
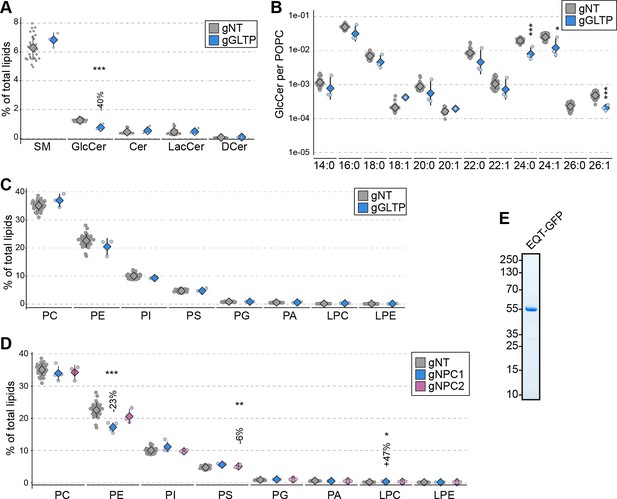
GLTP, NPC1, and NPC2 knockout cells do not display major phospholipid imbalances.
(A, B) Lipidomics analysis of GLTP knockouts show lowered glucosylceramide levels that is represented in various acyl chain species. (C) GLTP knockouts do not present altered phospholipid levels. (D) Lipidomics analysis of NPC1 and NPC2 knockout cells do not display major changes in their phospholipid repertoire. Diamonds and error bars denote mean and standard deviations, respectively. (E) Coomassie Blue staining of recombinant EQT-GFP.
-
Figure 2—figure supplement 1—source data 1
Raw unedited gels for Figure 2—figure supplement 1.
- https://cdn.elifesciences.org/articles/91345/elife-91345-fig2-figsupp1-data1-v1.zip
-
Figure 2—figure supplement 1—source data 2
Uncropped and labeled gels for Figure 2—figure supplement 1.
- https://cdn.elifesciences.org/articles/91345/elife-91345-fig2-figsupp1-data2-v1.zip
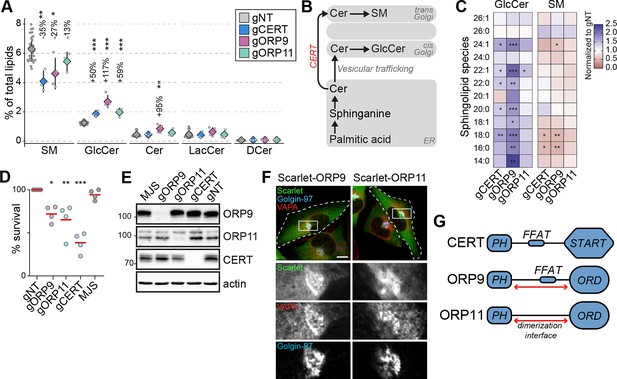
CERT, ORP9, and ORP11 knockout cells demonstrate reduced sphingomyelin levels.
(A) CERT, ORP9, and ORP11 knockouts demonstrate decreased sphingomyelin and increased glucosylceramide levels. Diamonds and error bars denote mean and standard deviations, respectively. (B) Schematic representation of de novo sphingomyelin synthesis pathway of mammalian cells. (C) Decreased sphingomyelin and increased glucosylceramide levels observed in knockout cells are present in various sphingolipid subspecies. (D) Similar to CERT knockouts, ORP9 and ORP11 knockouts are sensitive to methyl-β-cyclodextrin treatment. Red lines correspond to mean values from four experiments. (E) Western blot of knockout cells showing that the loss of ORP9 or ORP11 does not affect CERT protein levels. (F) ORP9 and ORP11 localize at the Golgi apparatus. Scale bar 10 µm. (G) Domain architecture of CERT, ORP9, and ORP11. All proteins contain PH domains for Golgi localization. CERT and ORP9, but not ORP11, contain a FFAT motif for interacting with VAP proteins.
-
Figure 3—source data 1
Raw unedited gels for Figure 3.
- https://cdn.elifesciences.org/articles/91345/elife-91345-fig3-data1-v1.zip
-
Figure 3—source data 2
Uncropped and labeled gels for Figure 3.
- https://cdn.elifesciences.org/articles/91345/elife-91345-fig3-data2-v1.zip
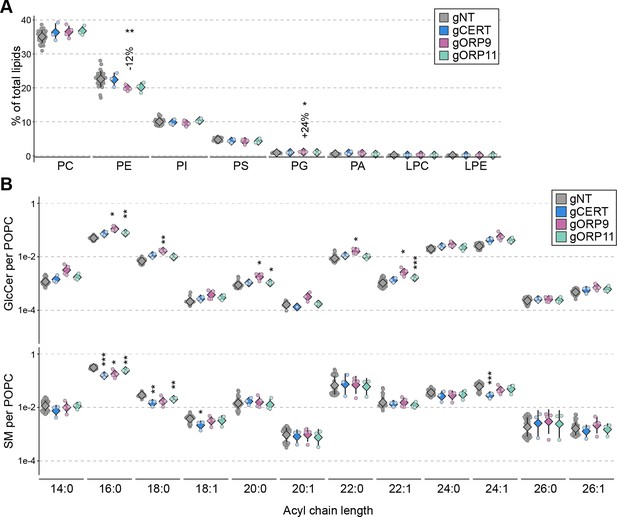
Sphingolipid imbalances in CERT, ORP9, and ORP11 knockouts are represented in multiple acyl chain species.
(A) CERT, ORP9, and ORP11 knockouts do not display major phospholipid imbalances. (B) Reduced sphingomyelin and increased glucosylceramide levels in CERT, ORP9, and ORP11 knockout cells is observed in multiple acyl chain species. Lipid levels are normalized to the POPC (16:0/18:1) levels. Diamonds and error bars denote mean and standard deviations, respectively.
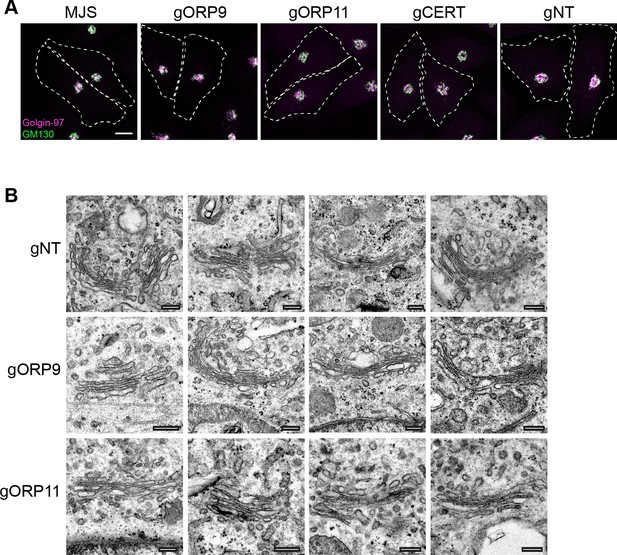
CERT, ORP9, and ORP11 knockouts do not display defects in Golgi morphology.
(A) Immunofluorescence staining for cis and trans-Golgi markers, GM130, and Golgin-97 respectively, do not show any changes in ORP9, ORP11, and CERT knockouts. Scale bar 10 µm. (B) Electron microscopy images for ORP9 and ORP11 knockouts do not display any changes in Golgi morphology. Scale bar 250 nm.
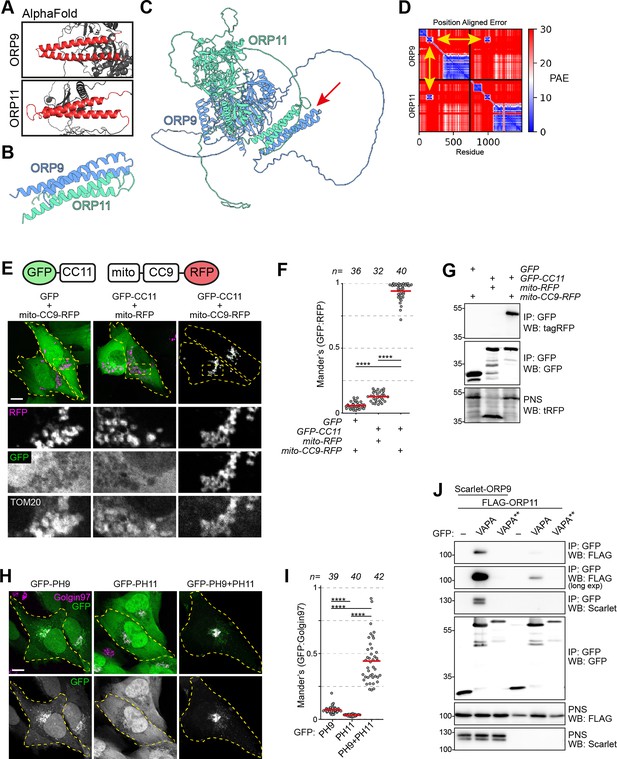
ORP9 dimerization is critical for ER localization of ORP11.
(A) AlphaFold revealing coiled coils in ORP9 and ORP11. (B) AlphaFold-Multimer shows that coiled coils of ORP9 and ORP11 interacting with each other. (C) ColabFold protein complex prediction for full length ORP9 and ORP11 shows the dimerization via coiled coils (arrow). (D) Position aligned error for panel C. Note that the interaction of coils has a lower score (yellow arrows). (E) Coiled coils of ORP9 and ORP11 is sufficient to define their localization as ORP11 coiled coils colocalizes with the mitochondria-targeted ORP9 coiled coils at mitochondria. Scale bar: 10 µm. (F) Colocalization analysis of immunofluorescent images corresponding to panel E. Red lines correspond to mean values from three experiments; n is the number of analyzed cells. (G) Co-immunoprecipitation confirming that the coils of ORP9 and ORP11 interact with each other. (H) Immunofluorescence images of PH domain localizations. Compared to individual PH domains of ORP9 and ORP11, ORP9-ORP11 chimera demonstrates better affinity towards the Golgi. Scale bar: 10 µm. (I) Colocalization analysis of immunofluorescent images corresponding to panel H. Red lines correspond to mean values from 3 experiments; n is the number of analyzed cells. (J) Co-immunoprecipitation analysis of VAPA, ORP9, and ORP11 from over-expressing cells. Despite lacking a FFAT motif, ORP11 interacts with the ER-resident VAPA protein. This interaction is facilitated by the FFAT motif of ORP9 as a VAPA mutant unable to interact with FFAT motifs (VAPA**) was unable to co-precipitate ORP9 or ORP11.
-
Figure 4—source data 1
Raw unedited gels for Figure 4.
- https://cdn.elifesciences.org/articles/91345/elife-91345-fig4-data1-v1.zip
-
Figure 4—source data 2
Uncropped and labeled gels for Figure 4.
- https://cdn.elifesciences.org/articles/91345/elife-91345-fig4-data2-v1.zip
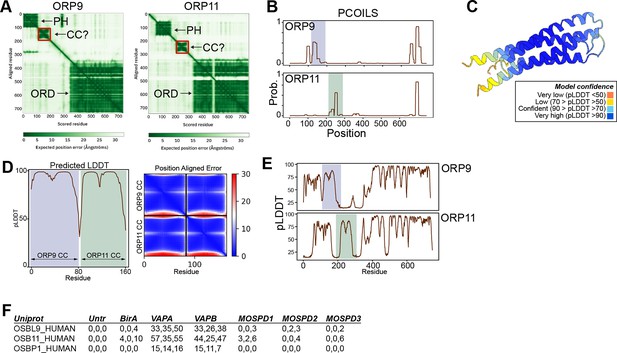
ORP9 and ORP11 interact with each other via their coiled coils.
(A) AlphaFold prediction of ORP9 and ORP11 reveals secondary structures between their PH and ORD domains. (B) PCOILS predicts coiled coils in the same of both proteins. Residues corresponding to coiled coils are highlighted. (C) AlphaFold-multimer/ColabFold prediction for ORP9-ORP11 coils dimerization. (D) Predicted local distance difference test (pLDDT) and position-aligned error plots for panel C. (E) Predicted local distance difference test for Figure 4C. (F) Unique peptide counts from BioID experiments performed with VAPA, VAPB, MOSPD1, MOSPD2, and MOSPD3 from Cabukusta et al., 2020. Both ORP9 and ORP11, despite latter lacking a FFAT motif, detected in BioID experiments with VAPA and VAPB.
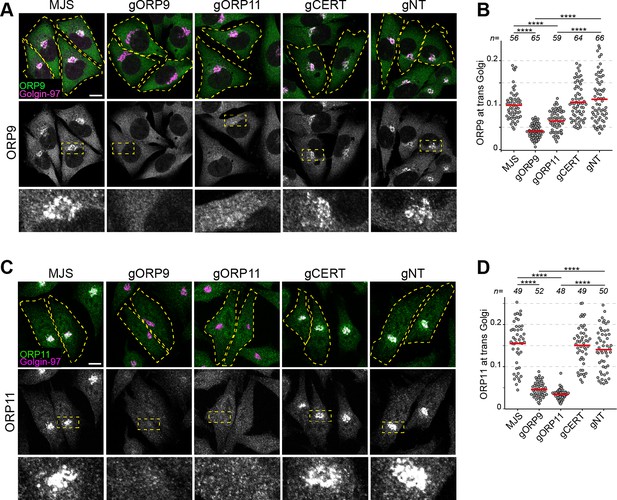
ORP9 and ORP11 dimerization is critical for their Golgi localization.
(A) Immunofluorescence images of cells stained for ORP9. ORP9 fails to localize at the Golgi as efficiently in ORP11 knockout cells. (B) Colocalization analysis of immunofluorescent images corresponding to panel A. Red lines correspond to mean values from three experiments; n is the number of analyzed cells. (C) Immunofluorescence images of cells stained using an ORP11 antibody. Similar to ORP9, ORP11 fails to localize at the Golgi as efficiently in ORP9 knockout cells. Note that the effect of ORP9 loss on ORP11 localization is more dramatic than vice versa. (D) Colocalization analysis of immunofluorescent images from panel C. Red lines correspond to mean values from three experiments; n is the number of analyzed cells. All scale bars are 10 µm.
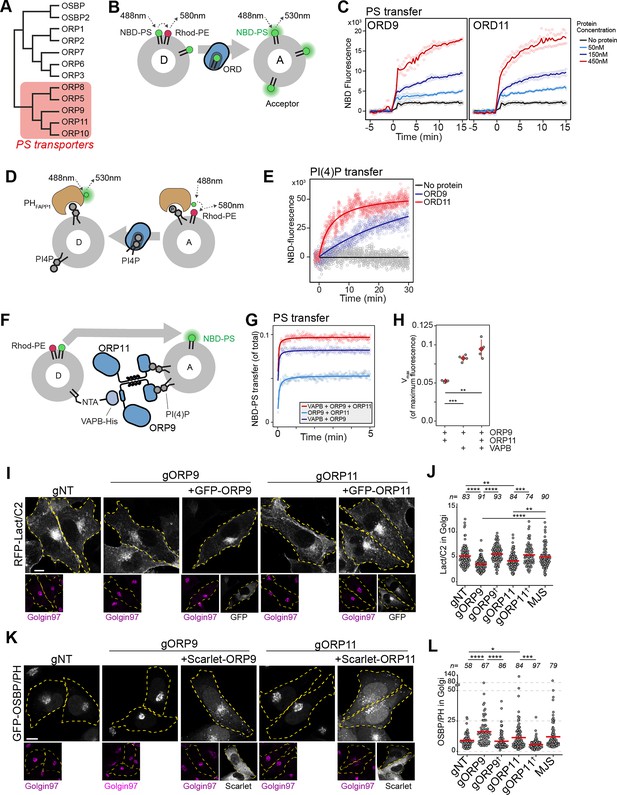
ORP9 and ORP11 are essential for phosphatidylserine (PS) and phosphatidylinositol-4-phosphate (PI(4)P) levels in the Golgi apparatus.
(A) Phylogenetic tree of human OSBP-related domains showing that ORP9 and ORP11 belong to the PS transporter branch. (B) Schematic representation of FRET-based PS transfer assay. Rhodamine in the donor vesicles quenches NBD fluorescence unless NBD-labeled lipid is transferred to acceptor liposomes. (C) FRET-based lipid transfer assay using the OSBP-related domains of ORP9 and ORP11, ORD9, and ORD11, respectively, demonstrate the PS trafficking ability between vesicles in vitro. (D) Graphical representation of PI(4)P transfer assay that utilizes the NBD-labeled PH domain of FAPP1. PI(4)P transfer by a protein leads to increase NBD-fluorescence by dequenching. (E) PI(4)P transfer assay using ORP9-ORD and ORP11-ORD (ORD9 and ORD11, respectively). (F) Schematic representation of in vitro reconstitution of membrane contact sites for lipid transfer assays. (G) PS transfer assay at in vitro reconstituted membrane contact sites formed by the VAPB-ORP9-ORP11 trimer. (H) Vmax quantifications of PS transfer assays from panel E. (I) The PS sensor Lact-C2 localizes less prominently to the Golgi in ORP9 and ORP11 knockout cells. (J) Golgi quantification of the PS sensor RFP-LactC2 indicates reduced PS levels in this organelle. This phenotype could be rescued by reconstitution of the missing protein in knockout cells (shown with a dagger). Red lines correspond to mean values from three experiments; n is the number of analyzed cells. (K) The PI(4)P sensor OSBP-PH localizes more prominently to the Golgi area in ORP9 and ORP11 knockout cells. (I) Golgi quantification of the PI(4)P sensor confirms the accumulation of PI(4)P at this organelle. This phenotype was rescued by reconstitution of the missing protein in knockout cells (shown with a dagger). Red lines correspond to mean values from three experiments; n is the number of analyzed cells.
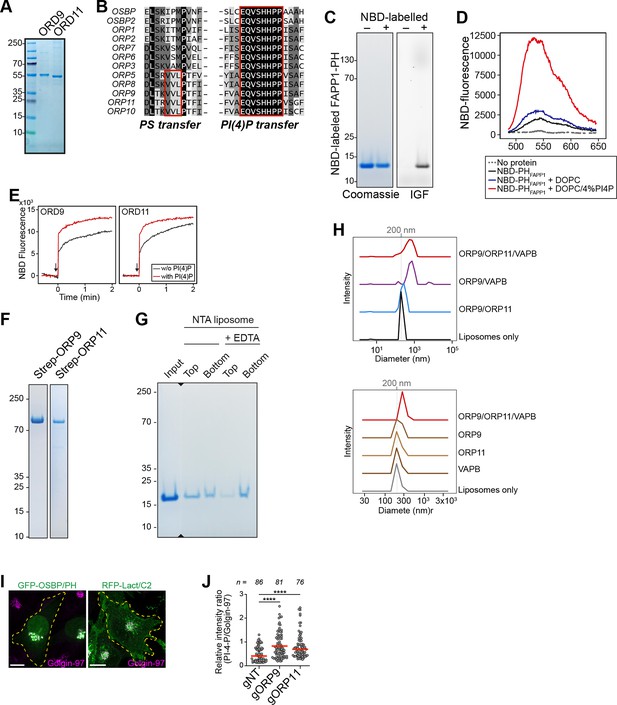
ORP9 and ORP11 are needed for maintaining phospholipid levels in the Golgi.
(A) Coomassie Blue staining of recombinant OSBP-related domains of ORP9 and ORP11. (B) ORP9 and ORP11 contain the critical residues for phosphatidylserine (PS) transfer. (C) Coomassie and in-gel fluorescence images of unlabeled and NBD-labeled PH domains of FAPP1 (D) Emission spectrum of NBD-labeled FAPP1PH in the presence of empty (DOPC) and phosphatidylinositol-4-phosphate (PI(4)P)-loaded liposomes. (E) PS transfer activity of ORD9 or ORD11 is promoted by addition of PI(4)P to acceptor liposomes. (F) Coomassie staining of recombinant full-length ORP9 and ORP11. (G) Liposome floatation assay shows the tethering of the soluble VAPB-His onto DGS-NTA-decorated liposomes. (H) Liposome tethering assay demonstrates liposome tethering by the ORP9-ORP11-VAPB complex. (I) Intracellular localization of GFP-OSBP-PH and RFP-Lact-C2 in MelJuSo cells. (J) Golgi quantification of a PI(4)P-specific antibody reveals accumulation of PI(4)P in ORP9 and ORP11 knockout cells. Red lines correspond to mean from two experiments. n is the number of analyzed cells.
-
Figure 6—figure supplement 1—source data 1
Raw unedited gels for Figure 6—figure supplement 1.
- https://cdn.elifesciences.org/articles/91345/elife-91345-fig6-figsupp1-data1-v1.zip
-
Figure 6—figure supplement 1—source data 2
Uncropped and labeled gels for Figure 6—figure supplement 1.
- https://cdn.elifesciences.org/articles/91345/elife-91345-fig6-figsupp1-data2-v1.zip
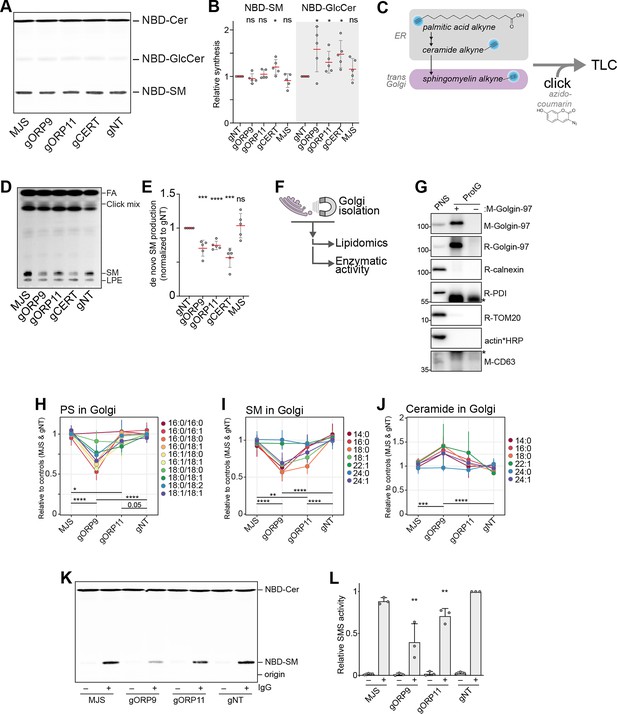
ORP9 and ORP11 are needed for de novo sphingomyelin synthesis in the Golgi apparatus.
(A) Thin-layer chromatography readout of the enzymatic activity assay performed in lysates reveals the unreduced sphingomyelin synthesis capacity of knockout cells. (B) Quantification of the enzymatic activity assays corresponding to panel A. Note the increased GlcCer production capacity in the knockout cells. Red lines and error bars correspond to mean and standard deviation from five experiments, respectively. (C) Graphic representation of experiments using palmitic acid alkyne for monitoring de novo sphingomyelin synthesis. Alkyne-modified sphingolipids are ‘clicked’ with azido-coumarin before thin-layer chromatography (TLC) analysis. (D) TLC readout of de novo sphingomyelin synthesis assay in intact cells using palmitic acid alkyne. Knockout cells demonstrate reduced conversion of palmitic acid to sphingomyelin. FA: fatty acid, SM: sphingomyelin, LPE: lyso-O-phosphatidylethanolamine (E) Quantification of de novo sphingomyelin synthesis assay from panel C. Red lines and error bars correspond to mean and standard deviation from five experiments, respectively. (F) Simplified representation of Golgi-targeted lipidomics and enzymatic activity assays. (G) Western blot confirming the immunomagnetic isolation of trans-Golgi membranes. Cross-reactivity to Protein G and/or Golgin-97 IgG were labeled with asterisks. (H–J) Lipidomics analysis of Golgi isolates shows lowered PS levels in the Golgi of ORP9 and ORP11 knockout cells. Same Golgi fractions have reduced sphingomyelin but increased ceramide levels. Each colored line corresponds to a lipid species. Data points and the error bars correspond to mean and standard deviations from four experiments. (K) TLC readout of enzymatic activity assay performed in isolated Golgi from knockout cells. Golgi of ORP11 and especially that of ORP9 demonstrate lowered capacity to synthesize sphingomyelin. (L) Quantification of sphingomyelin synthesis activity assay performed in Golgi isolates corresponding to panel K. Bars and error bars denote mean and standard deviations from three experiments, respectively.
-
Figure 7—source data 1
Raw unedited gels for Figure 7.
- https://cdn.elifesciences.org/articles/91345/elife-91345-fig7-data1-v1.zip
-
Figure 7—source data 2
Uncropped and labeled gels for Figure 7.
- https://cdn.elifesciences.org/articles/91345/elife-91345-fig7-data2-v1.zip
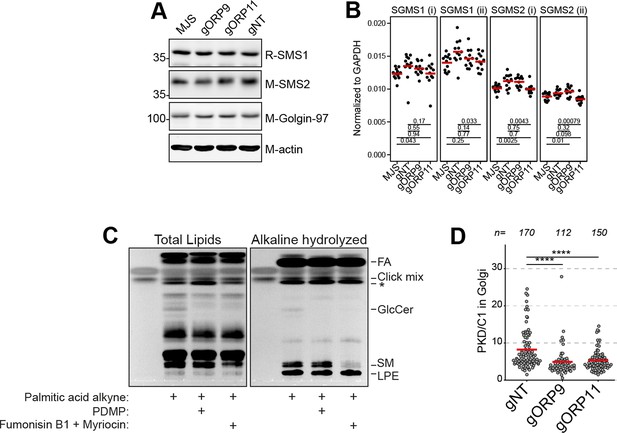
Loss of ORP9 or ORP11 does not affect sphingomyelin synthase levels.
(A) Western blot analysis showing that the protein two human sphingomyelin synthases, SMS1 and SMS2, are not affected in ORP9 and ORP11 knockouts. (B) qRT-PCR shows that mRNA levels of SMS1 and SMS2 are not affected dramatically in knockout cells. Two primer sets per protein each was used (shown with roman numbers). Red lines correspond to mean values. (C) Thin-layer chromatography analysis visualizing the conversion of palmitic acid alkyne to sphingomyelin. To define the band corresponding to sphingomyelin, cells were treated with PDMP or with a cocktail of fumonisin B1 and myriocin prior to alkaline hydrolysis. (D) Golgi quantification of the DAG sensor, mCherry-C1ab, indicates reduced DAG levels in this organelle. Red lines correspond to mean from three experiments. n is the number of analyzed cells.
-
Figure 7—figure supplement 1—source data 1
Raw unedited gels for Figure 7—figure supplement 1.
- https://cdn.elifesciences.org/articles/91345/elife-91345-fig7-figsupp1-data1-v1.zip
-
Figure 7—figure supplement 1—source data 2
Uncropped and labeled gels for Figure 7—figure supplement 1.
- https://cdn.elifesciences.org/articles/91345/elife-91345-fig7-figsupp1-data2-v1.zip
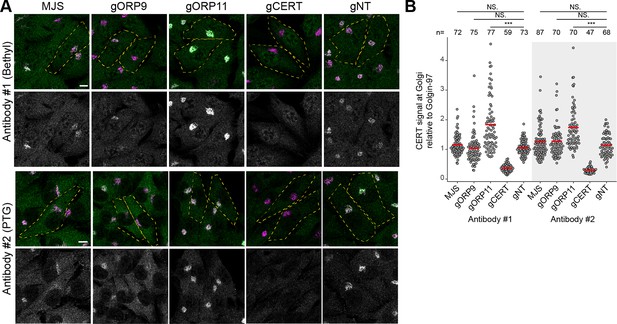
CERT is not less present in the Golgi of ORP9 and ORP11 knockouts.
(A) Immunofluorescent staining of ORP9, ORP11, and CERT knockout cells using two antibodies against CERT. Note that antibodies fail to stain the Golgi in CERT knockouts. (B) Quantifications corresponding to panel A. Loss of ORP9 or ORP11 do not reduce CERT localization at the Golgi. Note that loss of ORP11 increases CERT localization at the Golgi, shown by two antibodies. Red lines correspond to mean from three experiments. n is the number of analyzed cells.
-
Figure 7—figure supplement 2—source data 1
Raw unedited gels for Figure 7—figure supplement 2.
- https://cdn.elifesciences.org/articles/91345/elife-91345-fig7-figsupp2-data1-v1.zip
-
Figure 7—figure supplement 2—source data 2
Uncropped and labeled gels for Figure 7—figure supplement 2.
- https://cdn.elifesciences.org/articles/91345/elife-91345-fig7-figsupp2-data2-v1.zip
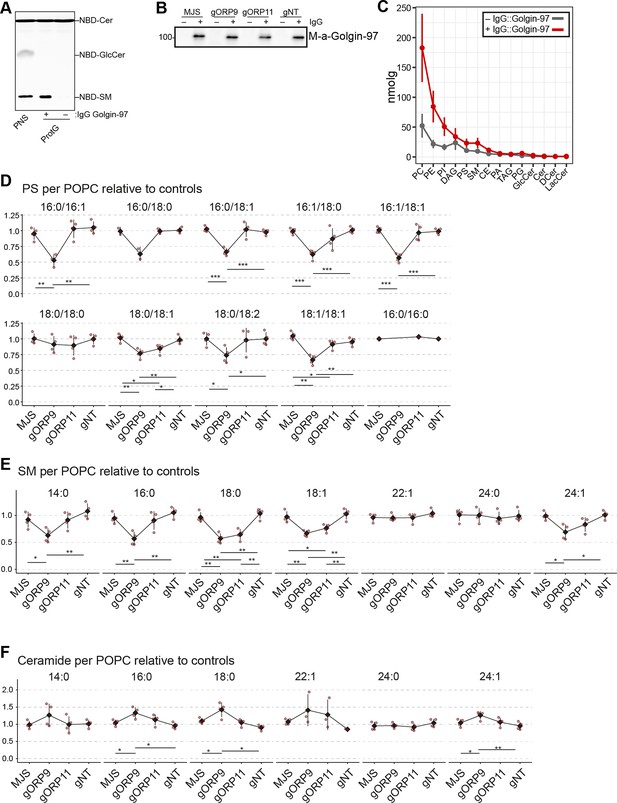
Immunomagnetic isolation yields to intact Golgi membranes.
(A) Sphingomyelin synthase enzymatic activity assay performed on immunomagnetic Golgi isolates shows that the isolated membranes are intact and enzymatically active. Sphingomyelin synthases contain six transmembrane helices and require intact membrane for activity (Tafesse et al., 2006 ‘The Multigenic Sphingomyelin Synthase Family’ J Biol Chem Volume 281, Issue 40). (B) Western blotting of immunomagnetic Golgi isolations from knockout cells using Golgin-97 antibody. (C) Amount of lipids isolated from samples in the absence or presence of the trans-Golgi specific antibody Golgin-97. The plot is based on 16 data points obtained from four experiments. Data points and error bars correspond to mean and standard deviations, respectively. (D) Distribution of phosphatidylserine (PS) species. (E) Distribution of sphingomyelin species. (F) Distribution of ceramide species Diamonds and error bars denote mean and standard deviations from four experiments in panels D-F, respectively.
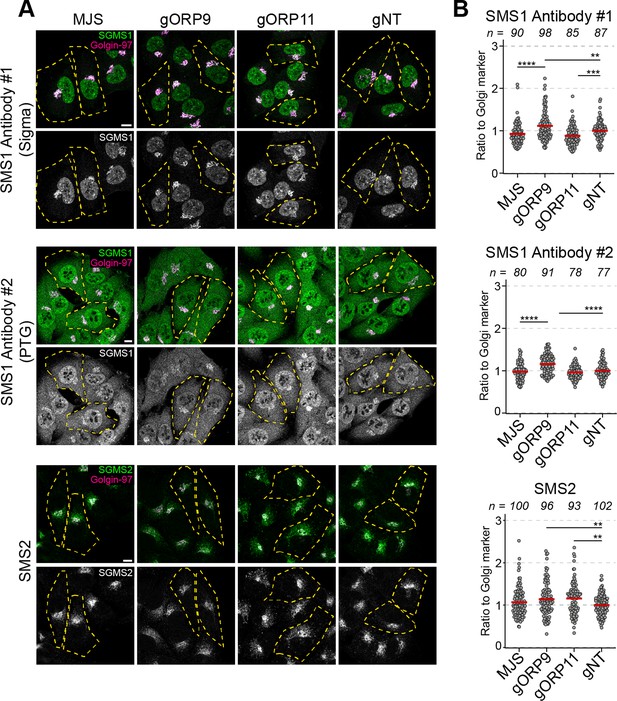
Golgi localization of sphingomyelin synthases is not reduced in ORP9 and ORP11 knockout cells.
(A) Immunofluorescence images showing localization of sphingomyelin synthases in ORP9 and ORP11 knockout cells. (B) Quantification of images corresponding to panel A. Note that the localization of SMS1 or SMS2 is not reduced in the knockout cells. An increased localization for SMS1 in the Golgi apparatus in ORP9 knockout cells is detected by two antibodies. Red lines correspond to mean from three experiments. n is the number of analyzed cells.
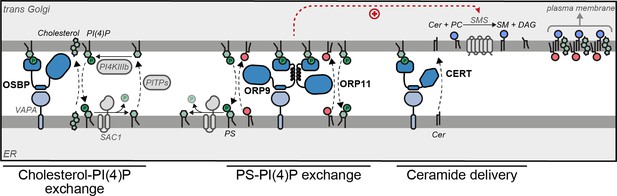
Model of ORP9-ORP11 mediated promotion of sphingomyelin synthesis at the ER-trans-Golgi contact site.
ORP9 and ORP11 require dimerization with each other to localize at ER-trans-Golgi membrane contact sites, where they exchange phosphatidylserine (PS) for phosphatidylinositol-4-phosphate (PI(4)P). Consequently, loss of either protein causes PS and PI(4)P imbalances in the Golgi apparatus. Since only ORP9 contains a FFAT motif, its loss leads to a more pronounced effect on PS, PI(4)P, and sphingolipid levels. The same contact site accommodates other LTPs. Multiple phosphatidylinositol transfer proteins (PITPs) traffic phosphatidylinositol to the Golgi for their phosphorylation. Each PITP at the ER-Golgi contact site serves a different function—one of these proteins, Nir2, also contains a FFAT motif (Peretti et al., 2008; Peretti et al., 2019). OSBP and CERT are responsible for the anterograde trafficking of cholesterol and ceramide, the latter is used for sphingomyelin production. Cholesterol, sphingomyelin, and PS are trafficked to the plasma membrane by vesicular means to be asymmetrically distributed between the leaflets of the plasma membrane.
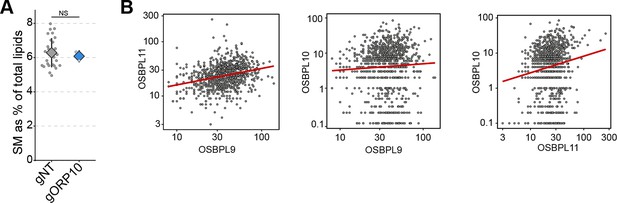
ORP10 knockouts do not display sphingolipid imbalances.
(A) Lipidomics analysis of ORP10 knockouts do not reveal sphingolipid imbalances that are observed in ORP9 or ORP11 knockouts. Diamonds and error bars denote mean and standard deviations, respectively. (B) Expression analysis from Cancer Cell Line Encyclopedia shows that ORP9, ORP10, and ORP11 are not differentially expressed, but show a positive correlation.
Additional files
-
Supplementary file 1
Overview of lipid transfer proteins (LTPs) targeted in this library and the list of short guide RNA sequences used in the knockout library to target LTPs.
- https://cdn.elifesciences.org/articles/91345/elife-91345-supp1-v1.xlsx
-
Supplementary file 2
Dataset file for the lipidomics analysis of lipid transfer protein (LTP) knockout cell lines.
- https://cdn.elifesciences.org/articles/91345/elife-91345-supp2-v1.xlsx
-
MDAR checklist
- https://cdn.elifesciences.org/articles/91345/elife-91345-mdarchecklist1-v1.pdf