Truncated radial glia as a common precursor in the late corticogenesis of gyrencephalic mammals
Figures
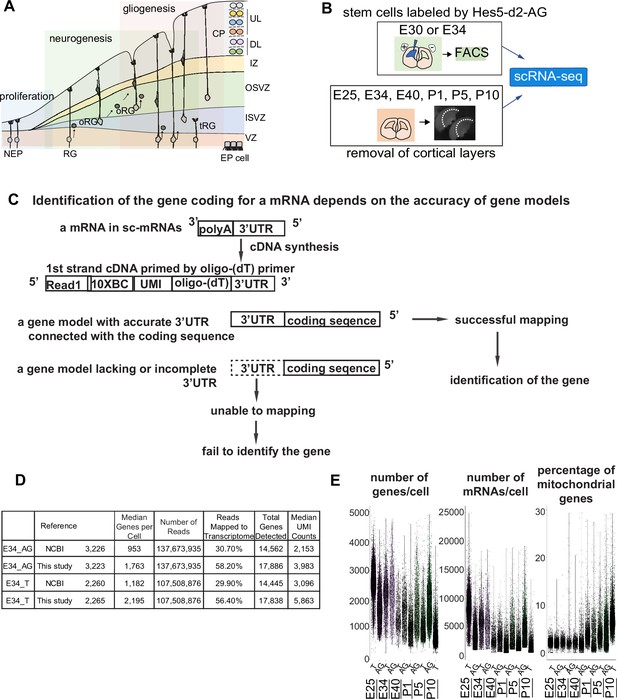
Improvement of the gene model for single-cell RNA-sequencing (scRNA-seq) of ferretes.
(A) Schematic representing cortical development and emergence of diverse neural progenitors in humans and ferrets. Progenitors sequentially generate DL- and subsequent UL-neurons, and finally glial cells, and form ependymal cells. Radial glia (RG) and outer RG (oRG) have been morphologically and positionally identified in both humans and ferrets while truncated RG (tRG) have been only reported in humans. VZ, ventricular zone; ISVZ, inner subventricular zone; OSVZ, outer subventricular zone; IZ, intermediate zone; DL, deep layer; UL, upper layer; CP, cortical plate; NEP cell, neuroepithelial cell; Mn, migrating neuron; and EP cell, ependymal cell. (B) Schematic representing the experimental design and time points used to build the transcriptome atlas of developing somatosensory cortex of ferrets. Single cells were isolated using 10x Chromium (see Materials and methods). (C) Linkage of the 3’-untranslated region (3’-UTR) sequence and the coding sequence of genes has been improved in this study. This linkage is necessary to assign the gene coding for an mRNA as far as 10x Genomics Chromium kit is used for scRNA-seq (see Materials and methods). (D) Table comparing quality control metrics of an alignment with either MusPutFur 1.0 (UCSC gene models) or MusPutFur 2.60 (this study) using E34 samples. The total number of genes detected and median genes per cell were higher with MusPutFur 2.60. (E) Violin plots showing the number of genes, mRNAs, and the percentage of mitochondrial genes per cell in each sample and time point.
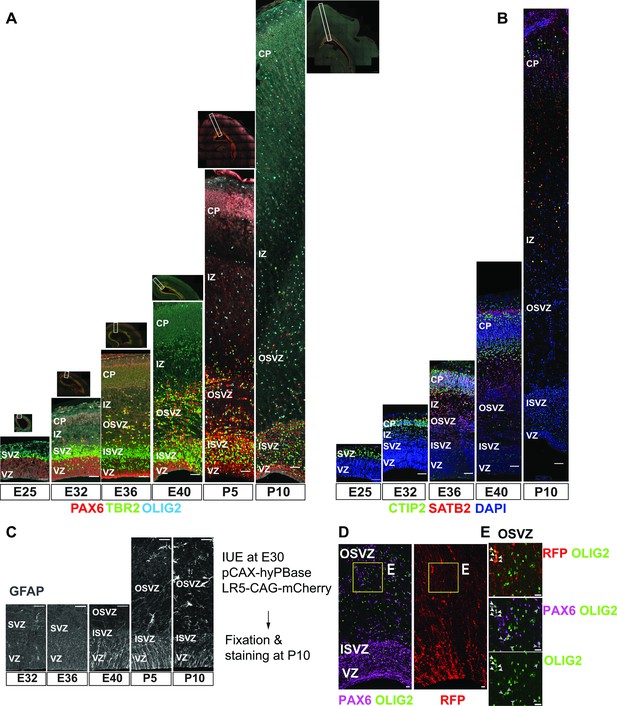
Temporal pattern of neurogenesis and gliogenesis in the cerebral cortex of ferrets.
(A) Coronal section of the dorsal cortices immunostained for radial glia (RG) (PAX6, red), IPC (TBR2, green), and OPC (OLIG2, cyan) from early to late neurogenesis at E25, E32, E36, E40, P5, and P10. Scale bars = 100 µm. The position of the image strip at each stage is shown in the corresponding dorsal hemisphere image above the strip. The approximative boundaries of dorsal cortex area used for single-cell RNA sequencing are highlighted with dotted line segments in the dorsal cortex hemisphere above each strip. (B) Immunostaining for CTIP2 (green) and SATB2 (red) at the same developmental stages as in (A), showing the onset of DL- (E25 or earlier) and UL-neurogenesis (E32–E34). Scale bars = 100 µm. (C) Immunostaining for GFAP, a marker showing gliogenic potential, in cortical germinal layers at E32, E36, E40, P5, and P10. Gliogenic progenitors emerge around E40, while mature astrocytes with a typical astrocytic morphology appear later at approximately P10 onward in the outside of the germinal layers (Reillo and Borrell, 2012). (D, E) mCherry labeling of the dorsal cortex at E30 showing that a population of OLIG2+ oligodendrocyte precursors are generated in the cerebral cortex by P10. (E) As reported for both mice and humans (Zheng et al., 2018; Rash et al., 2019; Huang et al., 2020; Kessaris et al., 2006). Arrowheads in the cropped images of the left panels (E area in D) indicate cells that co-express mCherry, PAX6, and OLIG2 in the outer sub-ventricular zone (OSVZ). Scale bars = 20 µm.
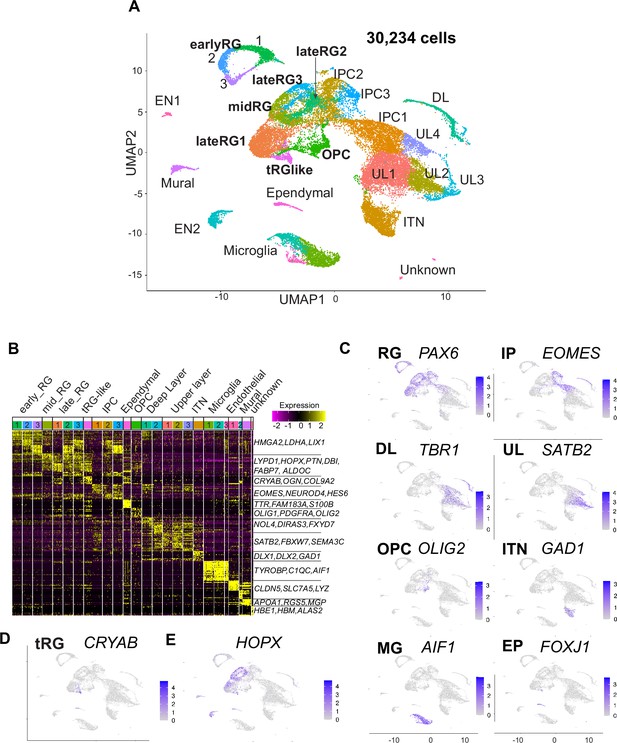
Single-cell RNA-sequencing reveals ferret transcriptome signatures and the cell types.
(A) Uniform Manifold Approximation and Projection (UMAP) visualization showing cells colored by Seurat clusters and annotated by cell types (as shown in C, D). (B) Heatmap showing expression profiles of cluster marker genes based on log fold change values. Cells were grouped by Seurat clustering (transverse). Cell types were assigned according to the expression of marker and differentially expressed genes in each cluster. Early radial glia (RG) was defined by the expression of HMGA2, LDHA, and LIX1; and late RG, by PTN, ALDOC, and FABP7. OPC, oligodendrocyte precursor cell; ITN, interneuron; and EN, endothelial cells. Other cell type abbreviations are shown in A and the main text. Color bar matches the Seurat clusters in (A). The 10 most enriched representative genes in each cluster are shown, with typical marker genes noted in (C–E). (C) Normalized expression levels of representative marker genes of different cell types projected onto the UMAP plot in (A). MG, microglia. (D) Expression pattern of CRYAB, a marker for truncated RG (tRG), only described in humans and other primates. (E) The expression pattern of HOPX, a marker for outer RG (oRG) in humans and other primates in the UMAP plot.
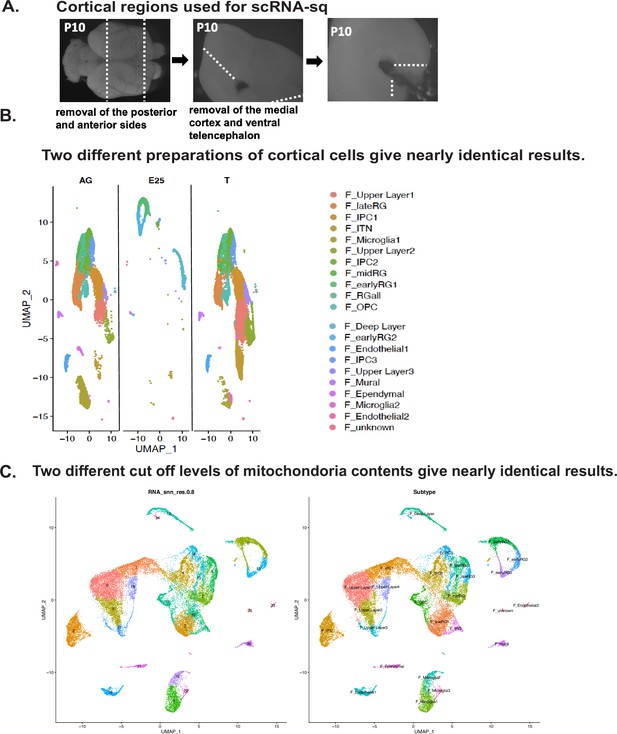
Quality and reproducibility of ferret single-cell transcriptome dataset.
(A) The brain region used for the cellular isolation for a single-cell RNA-sequencing (scRNA-seq) are shown. Dotted lines indicate the approximative boundaries where the cortical slices were cut. (B) Separate Uniform Manifold Approximation and Projection (UMAP) plots for each cell collection series that was prepared by different methods, after merged clustering. Left is the AzamiGreen (‘AG’) sample: FACS-based sorting of the neural stem cell fraction labeled with an AG driven by HES5 promoter (Ohtsuka et al., 2006). The ‘T’ samples are shown in the right: cells forming the ventricular zone (VZ), sub-VZ (SVZ), and intermediate zones (IZ) of cerebral cortices after discarding the cortical plate. E25 cells, which were mostly RG, were not collected by cell sorting and put between AG and T in UMAP plots. (C) Effects of cell filtration according to the mitochondrial content. We set the threshold to 10%, resulting in 28,686 cells in our dataset, and then performed the workflow from the normalization step with the same settings that had been applied to our original ferret dataset (Materials and methods). Left is clusters after the 10%-filtered subset on UMAP, and the original clusters in Figure 2A is right. Both conditions gave 26 clusters, and both major cell types and subtypes were conserved after filtering.
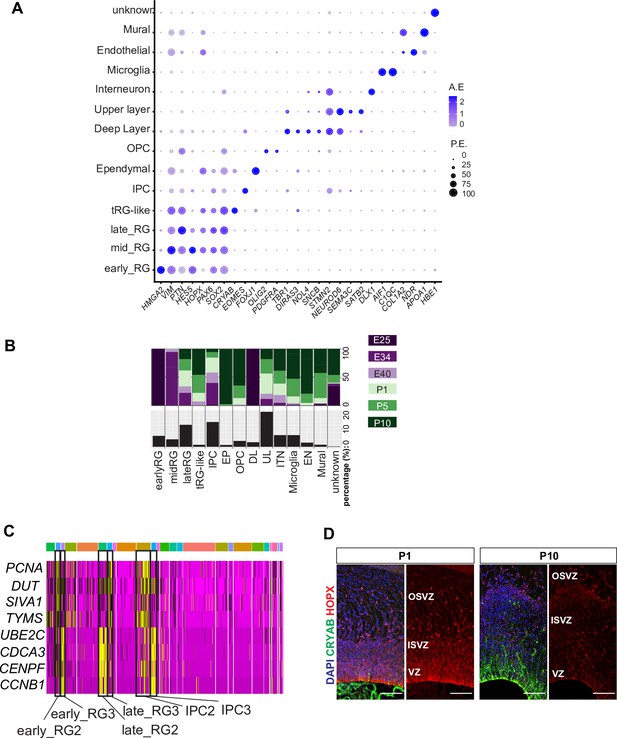
Cell types in developing ferret cerebral cortex.
(A) Dotplot showing average expression and percentage of expression of representative marker genes for individual cell types in the merged dataset. The largest cluster was composed of upper layer (UL) neurons, and their four clusters (Figure 2A) were combined as a single ‘UL’ group. P.E., percentage of expressing cells per cell type; A.E., average expression per cell type. (B) Percentage of sample collection stages in each cell type (top half) and percentage of each cell type in the merged dataset (bottom half). (C) Heatmap showing the expression level of cell cycle marker genes based on log fold change values. PCNA, DUT, SIVA1, and TYMS are markers of the S-phase; UBE2C, CDCA4, CENPF, and CCNB1 are markers of the G2M-phase. Three subclusters among early RG, late RG, and IPC clusters expressed different markers for cell cycle states. The color bar at the top indicates Seurat clusters matching those in Figure 2A. (D) Immunostaining for CRYAB+ truncated radial glia (tRG) (green) and HOPX+ RG (red) together with 4’,6-diamidino-2-phenylindole (DAPI) on P1 and P10 germinal layers.
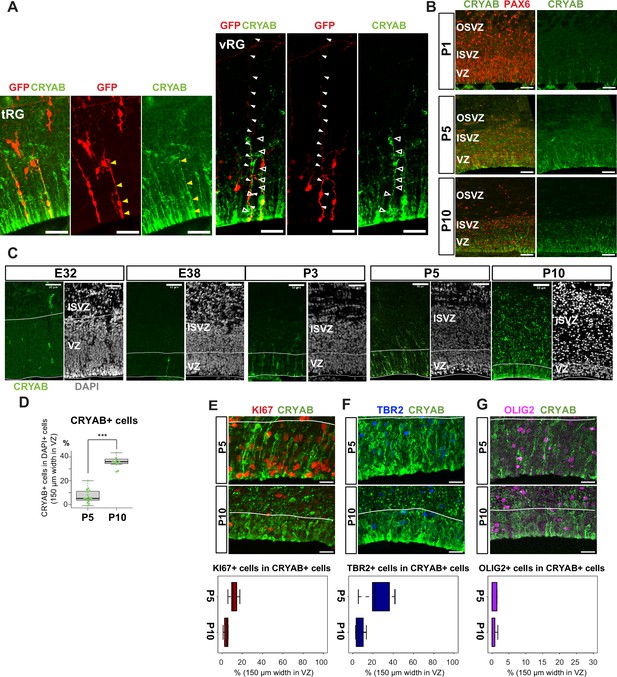
Truncated truncated radial glia (tRG) emerges during postnatal cortical development in ferrets.
(A) Representative images showing the cellular features of tRG and aRG in ventricular zone (VZ) at P0 and P5, respectively, stained for GFP (red) and CRYAB (green). RG cells were sparsely labeled with a GFP-expressing plasmid at E30 via in utero electroporation (IUE) for P0 samples, and at P3 for P5 samples. MAX projection was performed on a 30 µm vibratome section with 5 µm or 2.5 µm interval for each z-image at P0 or P5, respectively. Scale bar, 50 µm. (B) Expression patterns of CRYAB (green) and PAX6 (red) in ferret germinal zones during postnatal development (P1, P5, and P10) (cryosection thickness = 12 µm). (C) Developmental profile of CRYAB expression in ferret cortices. Immunostaining for CRYAB (green) and 4’,6-diamidino-2-phenylindole (DAPI) (gray) on cryosections at E32, E38, P3, P5, and P10 (cryosection thickness = 12 µm). (D) Quantification of CRYAB+ cells among all nuclei (DAPI) in VZ strips through immunostaining, indicating a great increase in CRYAB-expressing cells (strip width = 150 µm; n=3 for P5; n=2 for P10; Wilcoxon rank-sum test, p-value=6.574e-08). (E–G) Expression of other markers in CRYAB+ cells. Representative images taken with a 100× objective are shown with MAX projection. The border of VZ is shown with a white line. Below each double staining image, quantification of KI67-, TBR2-, or OLIG2-expressing cells among the CRYAB+ cell population in the VZ is shown (n=2 for P5; n=2 for P10 for each staining except n=3 for TBR2 staining). Box and whisker plots indicate ranges (lines) and upper and lower quartiles (box) with the median. Scale bars, 20 µm.
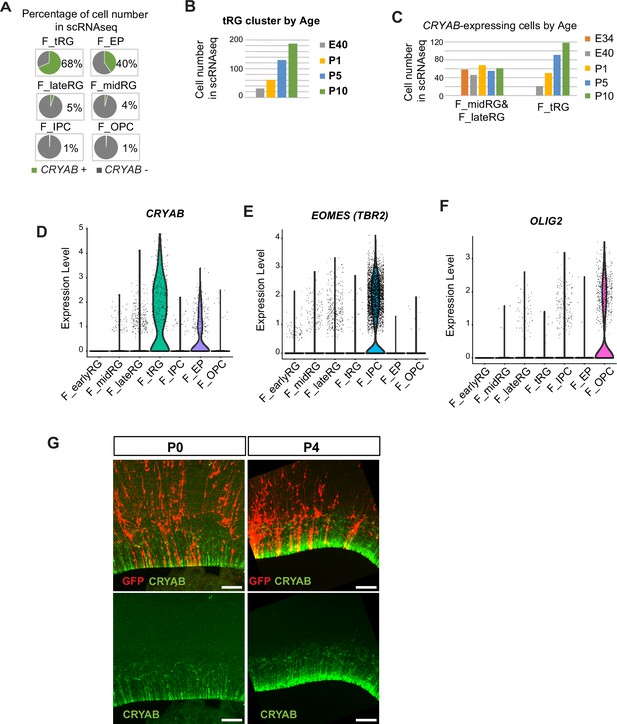
Development of ferret truncated radial glia (tRG) during postnatal cortical development.
(A) Pie charts showing the percentage of CRYAB expression in the ferret clusters of the merged transcriptome dataset of all stages (Figure 2). Cells were counted as positive if unique molecular identifier counts for CRYAB were >1 using Seurat ‘WhichCells’ function (slot = ‘counts’). (B) Number of cells in the tRG clustered by age annotated in the ferret transcriptome dataset (Figure 2). (C) Number of CRYAB-expressing cells in RG clusters of the transcriptome dataset (Figure 2). CRYAB expression was undetectable at E25. CRYAB-expressing cells in the midRG and lateRG subtypes did not change in all stages (cell numbers: 58, 46, 68, 55, and 61 at E34, E40, P1, P5, and P10, respectively), while the number of CRYAB+ tRG cells increased after birth (cell numbers: 0, 21, 50, 91, and 118 at E34, E40, P1, P5, and P10, respectively). (D–F) Violin plots indicating the normalized expression of CRYAB (D), TBR2 or EOMES (E), and OLIG2 (F) in the ferret clusters shown in Figure 2. (G) Cellular features of a tRG cell in cortex labeled with GFP at embryonic stages via in utero electroporation (IUE). Vibratome sections at P0 and P4 (thickness = 200 µm) were immunostained.
Rotated 3D-reconstructed image of the transparent slice used for taking the images in Figure 3A.
Green mainly indicates radial glia and their progeny neurons, which had been electroporated with enhanced green fluorescent protein (EGFP) expression vector. CRYAB immunostaining was shown in red. CRYAB+ truncated radial glia (tRG) extend a short radial process, some of which had a breb at the top of a radial process. Thickness is approximately 50 μm.
Rotated 3D-reconstructed image of the slice, from which the image of Figure 3—figure supplement 1G was taken.
Colors indicate the same as Figure 3—video 1.
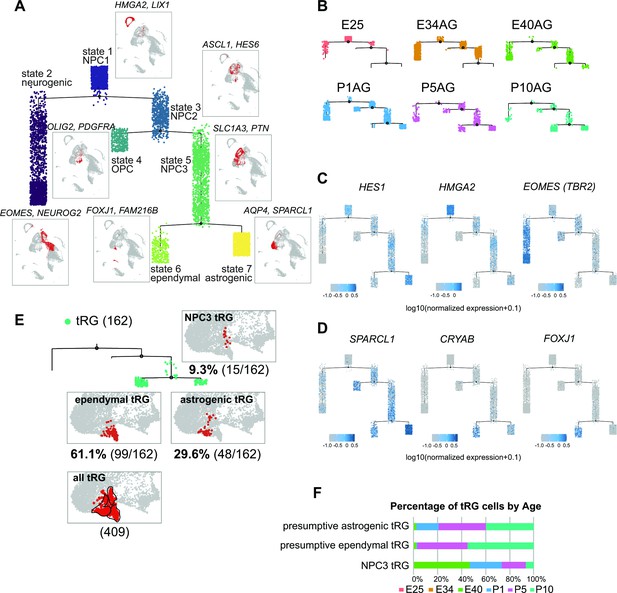
Temporal fates of radial glia (RG) cells predicted by pseudo-time trajectory analysis.
(A) Pseudo-time trajectory tree of progenitor cells in the ferret developing cortex (assembled with Monocle v2). Cellular distribution at each state is the same as in the Uniform Manifold Approximation and Projection (UMAP) plot shown in Figure 1C. Cell types representing each state and their gene markers are shown next to each state (see below). (B) Trajectory trees split by collection stages (AzamiGreen [AG] samples are shown). (C) Distribution of cells expressing marker genes for stem cell states (HES1 and HMGA2) and the neurogenic state (EOMES) along trajectories. Color densities indicate the log-normalized unique molecular identifier count for each gene. (D) Normalized expression of three genes: SPARCL1, CRYAB, and FOXJ1 in the state 6 and state 7 cells in the pseudo-time trajectory tree (A). The combination of expression levels of the four marker genes discriminates state 6 (ependymal) and state 7 (astrogenic). See the text for details. (E) Distribution of truncated RG (tRG) cells along the tree and tRG-focused UMAP visualization. The tRG cells (n=162) were found on the three states, NPC3 (9.3%; 15 cells), ependymal tRG (61.1%; 99 cells), and astrogenic tRG (29.6%; 48 cells). (F) Composition of the three types of tRG in (D) by the collection stage (AG and T samples are combined). No tRG cells were collected from E25 and E34.
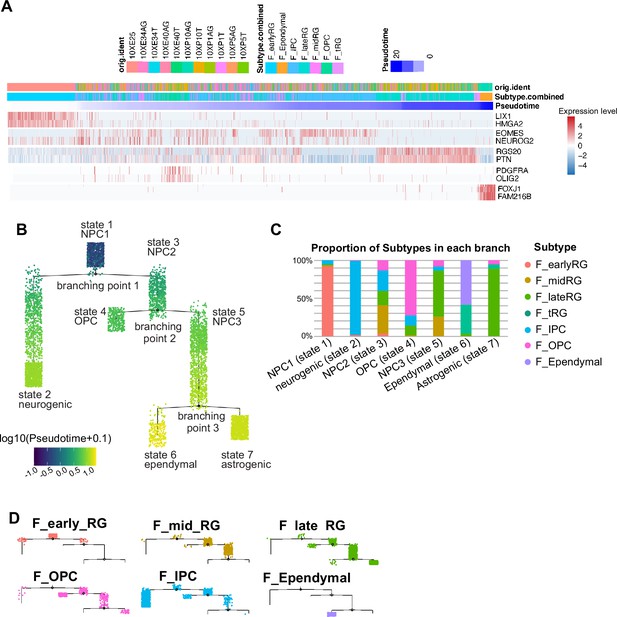
Temporal fates of radial glia (RG) cells identified by pseudo-time trajectory analysis.
(A) Ferret cells used for pseudo-time trajectory analysis were lined up along the stretched pseudo-time axis. Expression levels of markers for typical cell types are also shown along the pseudo-time axis: LIX1 and HMGA2 for early RG, EOMES and NEUROG2 for IP, RGS20 and PTN for late RG, PDGFR and OLIG2 for glial cells, and FOXJ1 and FAM216B for ependymal cells. (B) Branching trees labeled by pseudo-time score. Color scale indicates log10 (pseudo-time + 0.1) values. Each state is named after the major cell type. (C) The composition of each state is shown by clusters defined in the Uniform Manifold Approximation and Projection (UMAP) plot (Figure 2A). Percentages for clusters were calculated from all cells in each state. (D) Branching trees split by UMAP clusters (Figure 2).
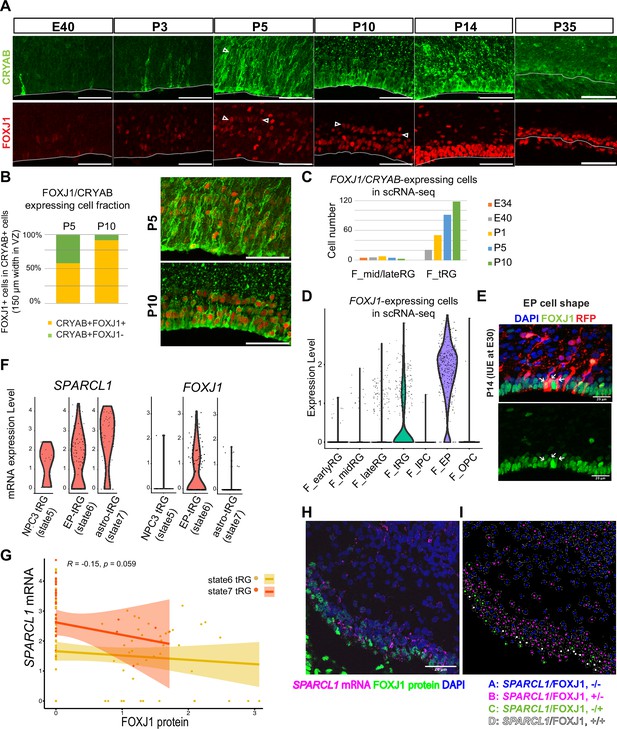
Truncated radial glia (tRG) adopt both ependymal cells and astrogenic fates in ferrets.
(A) Immunostaining of 12 µm cortical cryosections with CRYAB (green) and FOXJ1 (red), focusing on the ventricular zone (VZ) at E40, P3, P5, P10, P14, and P35. Scale bar, 50 µm. CRYAB and FOXJ1 double-positive nuclear rows are shown with arrowheads at P5 and P10 when this nuclear alignment is visible. (B) Percentage of FOXJ1 expression in CRYAB-expressing cells (n=3 for P5; n=2 for P10; Wilcoxon rank-sum test p-value=1.749e-05). Images with merged channels in A are shown with the same color codes, antibodies, and scale bars as A. (C) Number of cells expressing both CRYAB and FOXJ1 in the mid and late RG, or tRG clusters shown in Figure 2A (with unique molecular identifier counts higher than 0.25). Colored bars indicate the stages of sample collection. CRYAB- and FOXJ1-expressing cells increased over time only in the tRG cluster. (D) Normalized expression levels of FOXJ1 in each cell in the indicated ferret clusters in Figure 2A. (E) Cortical origin and shape of FOXJ1-expressing ependymal cells indicated with white arrows. Staining for FOXJ1 at P14 after labeling cortical progenitors with an mCherry-expressing vector via in utero electroporation (IUE) at E30. The maximum projection images with 1 µm z-step size are shown. Cryosection thickness = 12 µm; scale bar = 20 µm. (F) Normalized expression of SPARCL1 and FOXJ1 in each cell in ferret tRG clusters separated by the pseudo-time trajectory analysis (see Supplementary file 3). (G) Relationship between SPARCL1 and FOXJ1 transcripts in individual cells within each tRG cluster. Within the 15 cells that were classified in state 5 tRG, only one expressed FOXJ1 mRNA. Pearson relationship analysis was performed using cells from states 6 and 7 (R=0.19, p-value = 0.018). (H) Immunostaining of a P10 cortical tissue for FOXJ1 protein and SPARCL1 mRNA. (I) Cells within the VZ in (H) were clustered into four classes by FOXJ1 protein± and SPARCL1 mRNA±. Cluster A: SPARCL1/FOXJ1-/-, B: SPARCL1/FOXJ1+/-, C: SPARCL1/FOXJ1-/+, D: SPARCL1 /FOXJ1+/+ (Supplementary file 4). See Materials and methods for clustering. Scale bar = 20 µm.
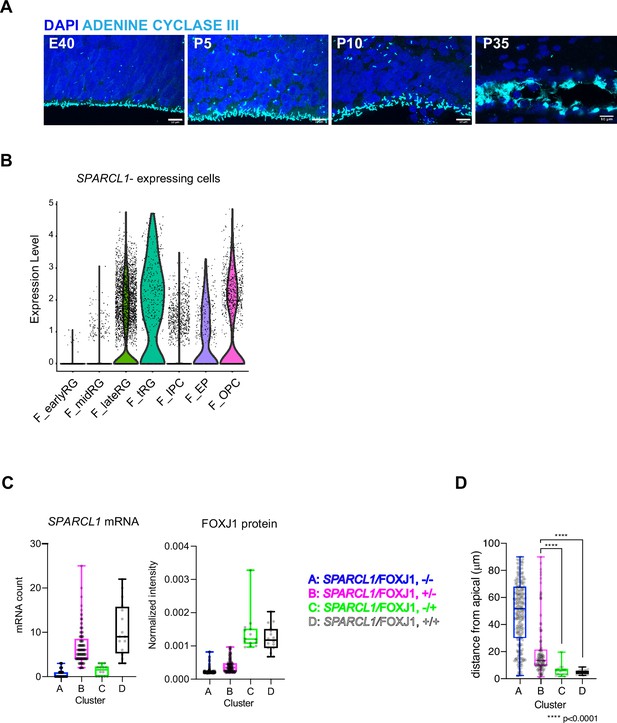
Ferret truncated radial glia (tRG) adopt both ependymal and astrogenic fates in ferrets.
(A) Onset of ciliogenesis in the developing cortex of ferrets, shown via staining for ADENINE CYCLASE III (cyan) along the ventricular zone (VZ) surface at E40, P5, P10, and P35. Scale bars = 10 µm. (B) Normalized expression of SPARCL1/cell in the major cell types shown in Figure 2A. (C) Plots showing SPARCL1 mRNA count (left) and normalized FOXJ1 protein levels (right) in each cell clustered into individual classes (A–D) within the VZ shown in Figure 5H (Supplementary file 4). The box and whisker plots indicate the range (lines) and the upper and lower quartiles with the median (box). (D) Distances of each cell (its center of gravity) from the apical surface in the individual clusters (A–D), as indicated by the box and whisker plots in (C). Cluster C and D cells are significantly located on the apical surface compared to cluster B cells. Data normality was analyzed by Anderson-Darling test and statistical significance was calculated by Kruskal-Wallis test with Dunn’s multiple comparisons test.
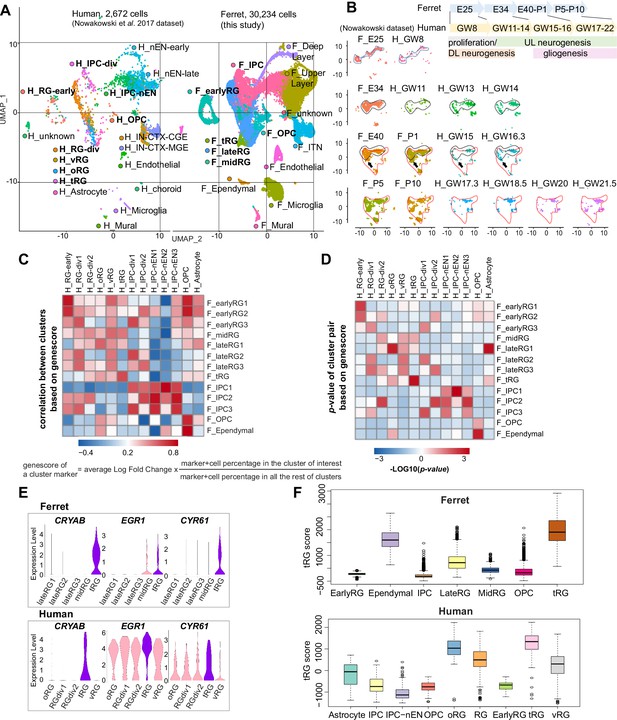
Comparison of molecular identity of radial glia (RG) subtypes between ferret and human.
(A) Uniform Manifold Approximation and Projection (UMAP) visualization of integrated human (n=2672; left) and ferret (n=30,234; right) single-cell datasets colored according to the different clusters. The names of clusters from human and ferret cells begin with ‘H’ and ‘F’, respectively. (B) Homologous temporal pattern of transcriptomic characters of progenitors and their progenies between ferrets and humans. Corresponding neurogenic stages between ferrets and humans can be assigned by cell distribution at homologous positions in UMAP plots. Gliogenic RG cells (a subtype of the ‘late_RG’ group and OPC in Figure 2A) were first distinguished transcriptionally at ferret E40 and at human GW14, as pointed out by arrows. (C, D) Correlation coefficient (C) and significance (D) between indicated clusters of ferrets and humans, calculated by marker gene scores. See the text and Materials and methods for details. (E) Normalized expression levels of the indicated genes in humans and ferrets from each progenitor cluster. See the text and Materials and methods for details. (F) Truncated RG (tRG) scores of the indicated clusters are presented as box and whisker plots for humans and ferrets. As for the definition of cluster scores, see the text and Material and methods. These represent the range without outliers (lines) and upper and lower quartiles with the median (box). Outliers are represented as points outside the box. Outliers are 1.5-fold larger or smaller than interquartile range from the third or first quartile, respectively.
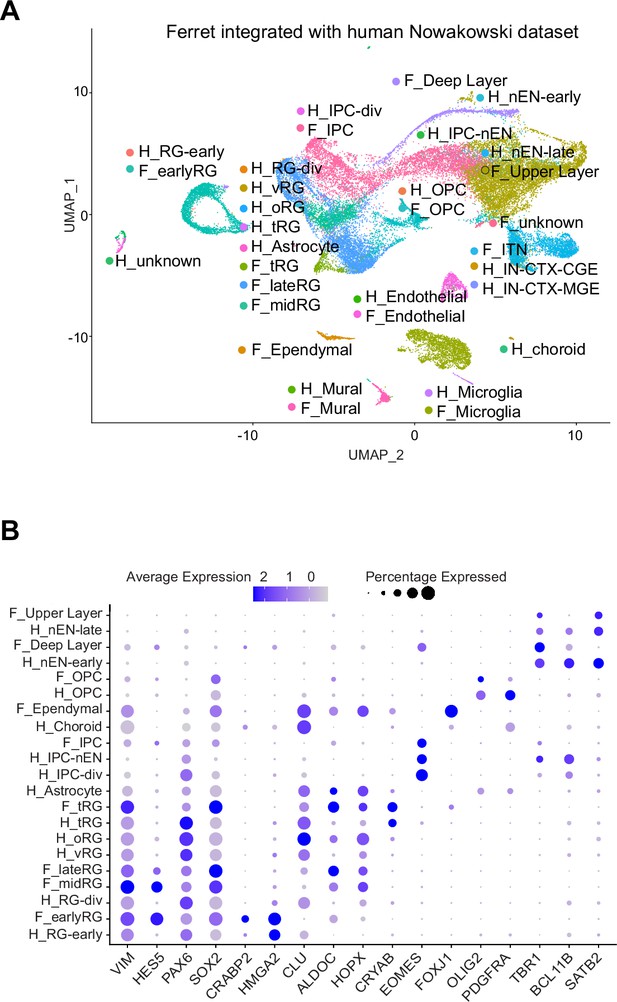
Comparison of molecular identity of radial glia (RG) subtypes between ferret and human.
(A) Uniform Manifold Approximation and Projection (UMAP) visualization of integrated ferret (n=30,234) and human (n=2672) single-cell datasets colored according to the different clusters. The names of clusters from human and ferret cells begin with ‘H’ and ‘F’, respectively. (B) Expression patterns of the indicated genes in each cluster. Circle color indicates the expression level of each gene. Circles size indicates the percentage of cells expressing the gene in the indicated cluster.
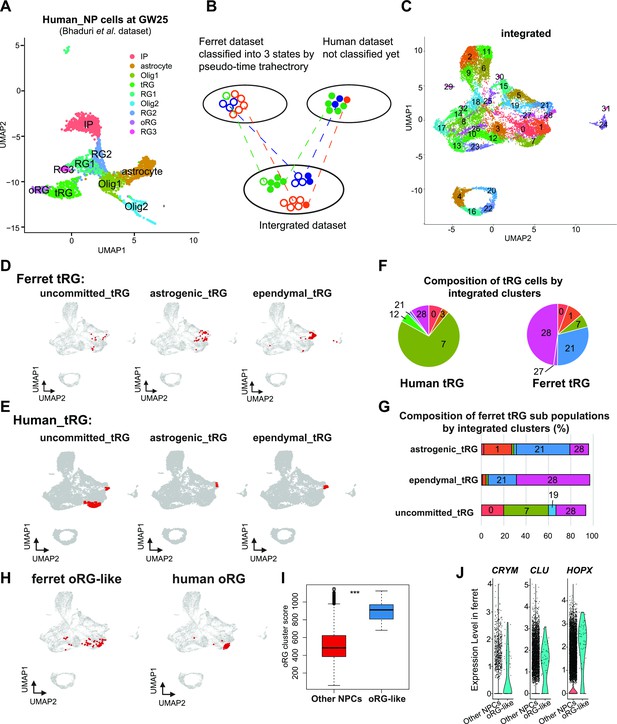
Identification of truncated radial glia (tRG) subtypes in humans and outer RG (oRG) in ferrets.
(A) Uniform Manifold Approximation and Projection (UMAP) visualization of human brain cells at GW25 (and removing neurons and other cell types). Cells are colored by cell type and identified by marker genes (data from Bhaduri et al., 2021). (B) Schematic of the integration strategy of human and ferret subtypes. We merged the two datasets by pairing mutual nearest neighbor cells (MNN) following canonical correlation analysis (CCA) across species (Stuart et al., 2019). (C) UMAP visualization of integrated ferret and human datasets colored and numbered by different clusters. (D, E) Identification of three tRG subtypes in ferrets (D) and humans (E). The red dots highlight the indicated tRG subtypes named after pseudo-time trajectory analysis in Figure 4: presumptively ‘uncommitted’, ‘astrogenic’, and ‘ependymal’. (F) Distribution of humans (left) and ferrets (right) tRG in the integrated dataset. Human and ferret tRG were identified from the separated dataset. The numbering corresponds to cluster numbers in the integrated clustering (C) of human and ferret datasets. (G) Distribution of ferret tRG subtypes (identified by the pseudo-time analysis) in the integrated dataset. A major cell population of each of the three ferret tRG subtypes (classified by the pseudo-time analysis), more or less, belongs to a single cluster in ferret-human integrated clustering; astrogenic tRG corresponds to cluster 21; ependymal tRG to cluster 20; uncommitted tRG to cluster 7. (H) Identification of oRG-like cells in ferret (left) and oRG cells in human (right), by the same way that we have done for tRG. All cells highlighted as red dots. (I) oRG scores for oRG-like cells and other neural progenitor cells (NPCs) in ferrets. The result indicates that oRG-like cells share more transcriptomic features than other NPCs, whereas ordinary clustering among ferret NPCs failed to distinguish oRG-like cells from the rest. Box and whisker plots indicate ranges (lines) and upper and lower quartiles with the median (box). (J) The expression levels of CRYM, CLU, and HOPX in oRG-like cells and other NPCs in ferrets.
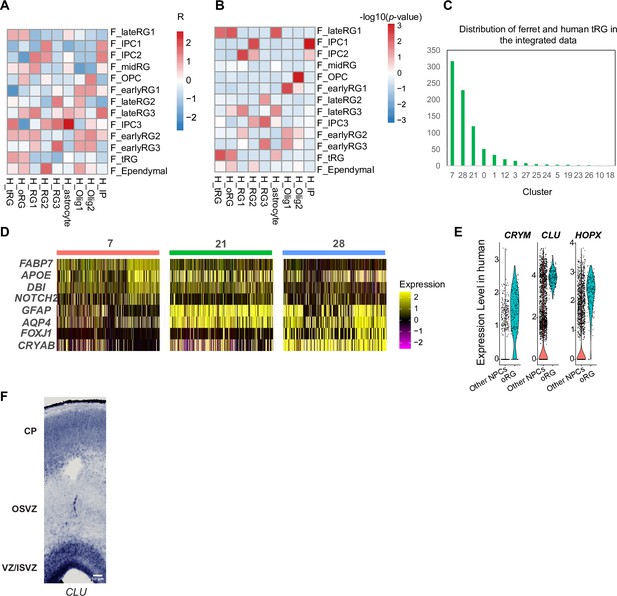
Comparison of the truncated radial glia (tRG) and outer RG (oRG) subtypes between ferrets and humans.
(A, B) Matrices of correlations between two arbitrary RG subtypes from humans and ferrets by calculating marker gene scores as described for Figure 5. (A) Correlations and (B) p-values between pairs in human and ferret clusters are shown. The early RG in ferrets showed the highest similarity with ‘OLIG1’ in human data. This might be because of two reasons: (1) cells only at GW25 without early RG were used for comparison and (2) cells in ‘OLIG1’ highly expressed genes related with cell proliferation, as the early RG also did (Supplementary file 6). We did not observe any cell types in human data corresponding to the ferret ‘mid RG’ with the same reason described above. (C) Distribution of human and ferret tRGs in the integrated dataset. The number in the X-axis indicates the cluster number identified by the Uniform Manifold Approximation and Projection (UMAP) in Figure 7C. (D) Expression levels of the subtype maker genes in the three tRG-enriched clusters (7, 21, and 28). Marker enrichment is consistent with the presumptive identification of tRG subtypes according to pseudo-time trajectory analysis. (E) Expression patterns of CRYM, CLU, and HOPX in oRG cells and other neural progenitor cells (NPCs) in humans. (F) In situ hybridization of CLU at the ferret cortex showed its expression in all ventricular zone (VZ), inner sub-VZ (ISVZ), and outer sub-VZ (OSVZ) at P5. Scale bar is 50 µm.
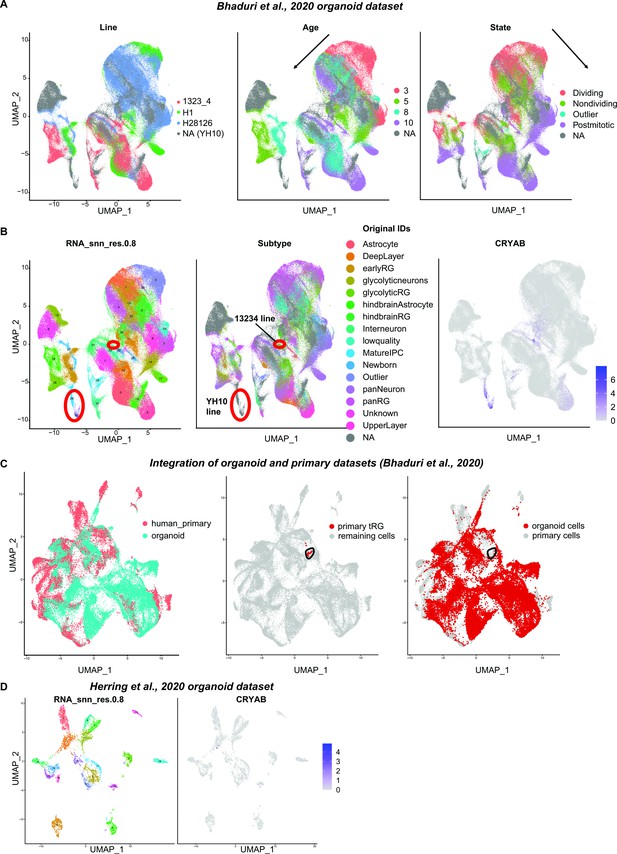
The absence of a truncated radial glia (tRG) cluster in human organoid datasets.
(A) Uniform Manifold Approximation and Projection (UMAP) visualization of human organoid-driven cells colored by the original annotations for the cell line (left), age (middle), or state (right). Human organoid dataset from Bhaduri et al., 2020, was used. Bhaduri dataset contained organoids generated from four different lines, which showed a variability in terms of cell distribution on UMAP while overall temporal and differentiation axes were recapitulated. (B) UMAP visualization of cells by clusters (left), original cluster annotations (middle), or by the normalized expression level of CRYAB (right). Red circles on UMAP highlight the group of cells expressing CRYAB. CRYAB expression was restricted to two out of four cell lines. CRYAB was expressed in clusters 26 and 30 enriched in the YH10 line, and cluster 29 enriched in the 13234 cell line. (C) UMAP visualization of integrated primary and organoid datasets from Bhaduri et al., 2020. Cells are colored by the originated dataset (left), primary tRG cluster (middle), or organoid dataset (right). Black circles were used to indicate the overlap of the region where human primary tRG was found. Human tRG did not overlap with organoid cells. We found that CRYAB-expressing organoid clusters 26 and 30 overlapped with ‘oRG/astrocyte’ clusters of primary tissues. (D) UMAP visualization of cells derived from Herring et al., 2022, human organoid dataset, colored by clusters (left) or by the normalized expression level of CRYAB (right).
Tables
Reagent type (species) or resource | Designation | Source or reference | Identifiers | Additional information |
---|---|---|---|---|
Strain, strain background (Mustela putorus furo) | Ferret | Marshall BioResources | N/A | |
Antibody | Anti-GFP (Chicken polyclonal) | Aves Labs | Cat#GFP-1020; RRID: AB_10000240 | IHC (1:500) |
Antibody | Anti-Alpha B Crystallin (Mouse monoclonal; clone 1B6.1–3G4) | Abcam | Cat#ab13496; RRID: AB_300400 | IHC (1:500) |
Antibody | Anti-FoxJ1 (Rabbit monoclonal; clone EPR21874) | Abcam | Cat#ab235445; RRID: N/A | IHC (1:500) |
Antibody | Anti-Olig2 (Goat polyclonal) | R&D Systems | Cat#AF2418; RRID: AB_2157554 | IHC (1:500) |
Antibody | Anti-EOMES (Rat monoclonal; clone Dan11mag, eFluor 660, eBioscience) | Thermo Fisher Scientific | Cat#50-4875-82; RRID: AB_2574227 | IHC (1:500) |
Antibody | Anti-Pax6 (Rabbit polyclonal) | Covance | PRB-278P; RRID: AB_291612 | IHC (1:500) |
Antibody | Anti-GFAP (mouse) | Sigma-Aldrich | Cat# G3893, RRID: AB_477010 | IHC (1:500) |
Antibody | Anti-HOPX (Rabbit polyclonal; clone FL-73) | Santa Cruz Biotechnology | Cat#sc-30216; RRID: AB_2120833 | IHC (1:500) |
Antibody | Anti-RFP (Rat monoclonal; clone 5F8) | ChromoTek | Cat#5f8-100; RRID: AB_2336064 | IHC (1:500) |
Antibody | Anti-A cyclase III (Goat polyclonal; clone N-14) | Santa Cruz Biotechnology | Cat#sc-32113; RRID: AB_2223118 | IHC (1:250) |
Antibody | Anti-Ctip2 (Rat monoclonal; clone 25B6) | Abcam | Cat#ab18465; RRID: AB_2064130 | IHC (1:500) |
Antibody | Anti-SATB2 (Mouse monoclonal; clone SATBA4B10) | Abcam | Cat#ab51502; RRID: AB_882455 | IHC (1:500) |
Antibody | Ki67 (Rat monoclonal; clone SolA15, eFluor 660, eBioscience) | Thermo Fisher Scientific | Cat#50-5698-82; RRID: AB_257423 | IHC (1:500) |
Antibody | Anti-Mouse IgG AF488 (Donkey) | Jackson ImmunoResearch Labs | Cat#715-545-151; RRID: AB_2341099 | IHC (1:500) |
Antibody | Donkey anti-Mouse IgG Cy3 | Jackson ImmunoResearch Labs | Cat#715-165-151; RRID: AB_2315777 | IHC (1:500) |
Antibody | Anti-Mouse IgG 647 (Donkey) | Jackson ImmunoResearch Labs | Cat:715-605-151; RRID: AB_2340863 | IHC (1:500) |
Antibody | Anti-Rat IgG 647 (Donkey) | Jackson ImmunoResearch Labs | Cat#712-605-153; RRID: AB_2340694 | IHC (1:500) |
Antibody | Anti-Rat IgG Cy3 (Donkey) | Jackson ImmunoResearch Labs | Cat#712-165-153; RRID: AB_2340667 | IHC (1:500) |
Antibody | Anti-Rabbit IgG Cy3 (Donkey) | Jackson ImmunoResearch Labs | Cat#711-165-152; RRID: AB_2307443 | IHC (1:500) |
Antibody | Anti-Rabbit IgG AF647 (Donkey) | Jackson ImmunoResearch Labs | Cat#711-605-152; RRID: AB_2492288 | IHC (1:500) |
Antibody | Anti-Goat IgG AF488 (Donkey) | Jackson ImmunoResearch Labs | Cat#705-545-003; RRID: AB_2340428 | IHC (1:500) |
Antibody | Anti-Goat IgG AF647 (Donkey) | Jackson ImmunoResearch Labs | Cat#705-605-147; RRID: AB_2340437 | IHC (1:500) |
Antibody | Anti-Chicken IgG Alexa Fluor 488 (Donkey polyclonal) | Jackson ImmunoResearch Labs | Cat#703-545-155; RRID: AB_2340375 | IHC (1:500) |
Recombinant DNA reagent | phmAG1-S1 (humanized monomeric Azami Green 1) | MBL | Cat#AM-V0034 | |
Recombinant DNA reagent | Plasmid: pLR5-Hes5-d2-Azamigreen | This paper | N/A | |
Recombinant DNA reagent | Plasmid: pPB-LR5-mCherry | This paper | N/A | |
Recombinant DNA reagent | Plasmid: pCAX-hyPBase | Fujita et al., 2020 | N/A | |
Recombinant DNA reagent | Plasmid: pCAG-Cre | Fujita et al., 2020 | N/A | |
Recombinant DNA reagent | Plasmid: pBP-LR5-floxstop-EGFP | Fujita et al., 2020 | N/A | |
Recombinant DNA reagent | Plasmid: pCR-BluntII-TOPO-Clu-probe | This paper | N/A | |
Chemical compound, drug | Dulbecco’s Modified Eagle Medium (DMEM) | Nacalai Tesque | Cat#08458-45 | |
Chemical compound, drug | Dulbecco’s Modified Eagle Medium (DMEM) F12+GlutaMax | Gibco | Cat#10565-018 | |
Chemical compound, drug | BSA Fraction V (7.5%) | Gibco | Cat#15260-037 | |
Chemical compound, drug | EDTA (0.5M) | Invitrogen | Cat#AM9260G | |
Chemical compound, drug | Papain | Funakoshi | Cat#WOR-1231-78 | |
Chemical compound, drug | Hank’s Balanced Salt Solution, HBSS (-) | Nacalai Tesque | Cat#17460-15 | |
Chemical compound, drug | Fast Green | Wako Pure Chemical Industries | Cat#2353-45-9 | |
Chemical compound, drug | Human FGF-basic | Peprotech | Cat#100-18B-10UG | |
Chemical compound, drug | B27 Supplement (50x) | Gibco | Cat#12587-010 | |
Chemical compound, drug | Penicillin/Streptomycin | Nacalai Tesque | Cat#09367-34 | |
Chemical compound, drug | HistoVT one | Nacalai Tesque | Cat#06380-05 | |
Chemical compound, drug | TissueTek O.C.T. compound | Sakura | Cat#4583 | |
Chemical compound, drug | Triton X-100 | Bio-Rad Laboratories | Cat#1610407 | |
Chemical compound, drug | Tet System Approved FBS US-sourced | Clontech | Cat#631105 | |
Chemical compound, drug | Donkey serum | Sigma | Cat#S30 | |
Chemical compound, drug | DAPI | Nacalai Tesque | Cat#1034-56 | |
Chemical compound, drug | Mountant (PermaFluor) | Thermo Fisher Scientific | TA-006-FM | |
Chemical compound, drug | UltraPure Low Melting Point agarose | Thermo Fisher Scientific | Cat#16520050 | |
Chemical compound, drug | Aqueous Mounting Medium PermaFluor | Thermo Fisher Scientific | Cat#TA-030-FM | |
Chemical compound, drug | DIG RNA labeling Mix, 10x | Roche | Cat#11277073910 | |
Chemical compound, drug | Anti-DIG-AP Fab fragments | Roche | Cat#11093274910 | |
Chemical compound, drug | 20x SSC (pH 4.5) | Invitrogen | Cat#AM9763 | |
Chemical compound, drug | Formamide | Wako | Cat#11-0740-5 | |
Chemical compound, drug | Formaldehyde solution | Sigma | Cat#11-0720-5 | |
Chemical compound, drug | Brewer’s yeast tRNA | Roche | Cat#10109525001 | |
Chemical compound, drug | Acetylated BSA | Nacalai Tesque | Cat#01278-44 | |
Chemical compound, drug | Heparin | Sigma | Cat#9041-08-1 | |
Chemical compound, drug | NBT/BCIP Stock solution | Roche | Cat#11681451001 | |
Chemical compound, drug | Proteinase K | Roche | Cat#03115887001 | |
Chemical compound, drug | Proteinase K | QIAGEN | Cat#158920 | |
Chemical compound, drug | RNase A | QIAGEN | Cat#158924 | |
Chemical compound, drug | Agarase | Life Technologies | Cat#EO0461 | |
Chemical compound, drug | T7 RNA polymerase | Roche | Car#10881767001 | |
Sequence-based reagent | CLU_F | This paper | PCR primers | GAATGACACCAAGGATTCAGAAACGAAGCT |
Sequence-based reagent | CLU_R | This paper | PCR primers | ATGGAATTCACAGAAGAAGACAACCAGGAC |
Commercial assay or kit | Chromium Single Cell 3′ Library & Gel Bead Kit v2 | 10x Genomics | Cat#120267 | |
Commercial assay or kit | Chromium i7 Multiplex Kit Kit | 10x Genomics | Cat#120262 | |
Commercial assay or kit | Chromium Single Cell A Chip | 10x Genomics | Cat#1000009 | |
Commercial assay or kit | Chromium Controller & Accessory Kit | 10x Genomics | Cat#120223 | |
Commercial assay or kit | Chromium Genome Reagent Kit v1 Chemistry | 10x Genomics | Cat#PN-120216 | |
Commercial assay or kit | HiSeq PE Rapid Cluster Kit v2 | Illumina | Cat#PE-402-4002 | |
Commercial assay or kit | TruSeq PE Cluster Kit v3-cBot-HS | Illumina | Cat#PE-401-3001 | |
Commercial assay or kit | Qubit RNA HS Assay Kit | Thermo Fisher Scientific | Cat#Q32852 | |
Commercial assay or kit | RNA 6000 Nano kit | Agilent Technologies | Cat#5067-1511 | |
Commercial assay or kit | RNA 6000 Nano kit | Agilent Technologies | Cat#5067-1511 | |
Commercial assay or kit | TruSeq Stranded mRNA Sample Prep Kit | Illumina | Cat#20020594 | |
Commercial assay or kit | TruSeq single-index adaptor kit | Illumina | Cat#20015960 | |
Commercial assay or kit | KAPA Real-Time Library Amplification Kit | Roche | Cat#KK2611 | |
Commercial assay or kit | PrimeScript II 1st strand cDNA Synthesis Kit | Takara | Cat#6210A | |
Commercial assay or kit | PrimeScript 1st strand cDNA Synthesis Kit | Takara | Cat#6110A | |
Commercial assay or kit | RNeasy mini kit | QIAGEN | Cat#74106 | |
Commercial assay or kit | CHEF Mammalian Genomic DNA Plug Kit | Bio-Rad Laboratories | Cat#1703591 | |
Commercial assay or kit | Zero Blunt TOPO PCR Cloning Kit | Thermo Fisher Scientific | Cat#450245 | |
Software, algorithm | R (v.3.6.3 2020-02-29) | R project for Statistical Computing | https://www.r-project.org/; RRID: SCR_001905 | |
Software, algorithm | R version 4.1.2 | R project for Statistical Computing | https://www.r-project.org/; RRID: SCR_001905 | |
Software, algorithm | Seurat v3 | Stuart et al., 2019 | RRID: SCR_016341; https://satijalab.org/seurat/get_started.html | |
Software, algorithm | Monocle2 | Qiu et al., 2017; Trapnell et al., 2014 | RRID: SCR_016339; http://cole-trapnell-lab.github.io/monocle-release/docs/ | |
Software, algorithm | Enrichr | Chen et al., 2013; Kuleshov et al., 2016 | http://amp.pharm.mssm.edu/Enrichr/; RRID: SCR_001575 | |
Software, algorithm | Cell Ranger v2 | 10x Genomics | RRID: SCR_017344; https://support.10xgenomics.com/single-cell-gene-expression/software/pipelines/latest/what-is-cell-ranger | |
Software, algorithm | ggplot2 | Wickham, 2016 | https://ggplot2.tidyverse.org | |
Software, algorithm | pheatmap | N/A | https://rdrr.io/cran/pheatmap/ | |
Software, algorithm | bcl2fastq ver. 1.8.4 | Illumina | RRID:SCR_015058; https://support.illumina.com/sequencing/sequencing_software/bcl2fastq-conversion-software.html | |
Software, algorithm | Real Time Analysis ver. 1.18.64 | Illumina | RRID:SCR_014332; http://support.illumina.com/sequencing/sequencing_software/real-time_analysis_rta.html | |
Software, algorithm | Supernova ver. 2.0.0 | 10x Genomics | https://support.10xgenomics.com/de-novo-assembly/software/overview/latest/welcome | |
Software, algorithm | RepeatMasker ver. 4.0.7 | Smit et al., 2013 | RRID:SCR_012954; http://repeatmasker.org/ | |
Software, algorithm | Repbase ver. 23.01 | N/A | RRID:SCR_021169; https://www.girinst.org/repbase/ | |
Software, algorithm | BRAKER ver. 2.0.5 | Hoff et al., 2019 | RRID:SCR_018964; https://github.com/Gaius-Augustus/BRAKER; Stanke et al., 2023 | |
Software, algorithm | GeneMark-ET ver. 4.33 | Lomsadze et al., 2014 | http://topaz.gatech.edu/GeneMark/ | |
Software, algorithm | AUGUSTUS ver. 3.3 | Stanke et al., 2008 | RRID:SCR_008417; http://bioinf.uni-greifswald.de/augustus/ | |
Software, algorithm | STAR | Dobin et al., 2013 | https://github.com/alexdobin/STAR (Dobin, 2023) | |
Software, algorithm | HISAT2 ver. 2.1.0 | Kim et al., 2019 | RRID:SCR_015530; http://ccb.jhu.edu/software/hisat2/index.shtml | |
Software, algorithm | StringTie ver. 1.3.4d | Kovaka et al., 2019 | RRID:SCR_016323; https://ccb.jhu.edu/software/stringtie/ | |
Software, algorithm | GMAP ver. 2017-11-15 | Wu and Watanabe, 2005 | RRID:SCR_008992 | |
Software, algorithm | gVolante ver. 1.2.1 | Nishimura et al., 2017 | https://gvolante.riken.jp | |
Software, algorithm | Volocity 3D Image Analysis Software | Perkin Elmer | RRID: SCR_002668 | |
Software, algorithm | MetaMorph | Molecular Devices | https://www.moleculardevices.com/products/cellular-imaging-systems/acquisition-and-analysis-software/metamorph-microscopy#gref | |
Software, algorithm | Adobe Illustrator | Adobe | RRID: SCR_010279; http://www.adobe.com/products/illustrator.html | |
Software, algorithm | ImageJ (Fiji) | Schindelin et al., 2012 | RRID: SCR_002285; http://fiji.sc | |
Other | CUBIC solution 2 | Susaki et al., 2015 | N/A | |
Other | SH800 Cell Sorter | SONY | https://www.sonybiotechnology.com | |
Other | Countess Cell Counter | Thermo Fisher Scientific | https://www.thermofisher.com | |
Other | Countess II Cell Counter | Thermo Fisher Scientific | https://www.thermofisher.com | |
Other | Cryostat CM3050 S | Leica | https://www.leicabiosystems.com | |
Other | Liner Slicer vibratome | Dosaka EM | http://www.dosaka-em.jp | |
Other | FV1000 confocal microscope | Olympus | https://www.olympus-lifescience.com | |
Other | scRNA-seq data from developing human cerebral cortex | Nowakowski et al., 2017 | https://cells.ucsc.edu/?ds=cortex-dev | |
Other | scRNA-seq data from GW25 human cerebral cortex | Bhaduri et al., 2021 | https://kriegsteinlab.ucsf.edu/datasets/arealization | |
Other | scRNA-seq data from human organoids | Herring et al., 2022 | http://development.psychencode.org/ | |
Other | scRNA-seq data from human primary brain tissue and organoids | Bhaduri et al., 2020 | https://organoidreportcard.cells.ucsc.edu |
Additional files
-
Supplementary file 1
Quality control, metadata, and cluster information, related to Figure 1 and Figure 2.
(A) Summary of statistics for each sequencing library aligned to our custom genome. (B) Summary of statistics for representative libraries aligned to MusPutFur 1.0 (NCBI) and our custom genome. (C) Metadata for each cell. (D) Cluster markers identified using ‘FindAllMarkers’ Seurat function and filtered by min.pct=0.25 and logfc.threshold=0.25. (E) Ratios of clusters in each sample.
- https://cdn.elifesciences.org/articles/91406/elife-91406-supp1-v1.xlsx
-
Supplementary file 2
Pseudo-time trajectory analysis related to Figure 4.
(A) Pseudo-time values for all cells treated in monocle v2. (B) Differentially expressed genes between cells at different pseudo-time branches, obtained by ‘FindMarkers’ Seurat function.
- https://cdn.elifesciences.org/articles/91406/elife-91406-supp2-v1.xlsx
-
Supplementary file 3
Expression of SPARCL1 and FOXJ1 in ferret truncated radial glia (tRG) cells, related to Figure 5.
Cells are sorted by decreasing expression level of SPARCL1 or FOXJ1 in ‘sorting by Sparcl1’ or ‘sorting by Foxj1’ sheets, respectively. Cells are colored and sorted by their pseudo-time state in Figure 4 in ‘state composition Foxj1& cryab’.
- https://cdn.elifesciences.org/articles/91406/elife-91406-supp3-v1.xlsx
-
Supplementary file 4
Distance of P10 cells with SPARCL1 mRNAs±, FOXJ1 protein± from the apical surface, related to Figure 5.
- https://cdn.elifesciences.org/articles/91406/elife-91406-supp4-v1.xlsx
-
Supplementary file 5
Human-ferret integration, metadata, cluster markers, related to Figure 6.
(A) Metadata for each cell in the integrated dataset. Ferret data was integrated with Nowakowski et al., 2017 (Science) dataset. (B) Cluster markers used for calculating genescore in Figure 6C–D, obtained from Nowakowski et al., 2017. (C) Correlation between ferret and human cluster markers as shown in Figure 6C–D. (D) Markers shared between ferret and human truncated radial glia (tRG). (E) tRG score for ferret and human cells as shown in Figure 6F. Markers in D were used for calculating tRG score in Figure 3F.
- https://cdn.elifesciences.org/articles/91406/elife-91406-supp5-v1.xlsx
-
Supplementary file 6
Cluster markers in the integrated dataset, related to Figure 7.
(A) Markers identified using the ‘FindAllMarkers’ Seurat function. (B) Differentially expressed genes identified using the ‘FindMarkers’ Seurat function, between cluster 28 and cluster 7 of the integrated data. (C) Differentially expressed genes identified using the ‘FindMarkers’ Seurat function, between cluster 21 and cluster 7 of the integrated data. (D) Markers of human outer radial glia (oRG). (E) Markers of ferret oRG.
- https://cdn.elifesciences.org/articles/91406/elife-91406-supp6-v1.xlsx
-
Supplementary file 7
Extraction of total RNA from different tissues, used for the gene model reconstruction, related to Figure 1.
- https://cdn.elifesciences.org/articles/91406/elife-91406-supp7-v1.xlsx
-
MDAR checklist
- https://cdn.elifesciences.org/articles/91406/elife-91406-mdarchecklist1-v1.docx