Rapid translocation of NGR proteins driving polarization of PIN-activating D6 protein kinase during root gravitropism
Abstract
Root gravitropic bending represents a fundamental aspect of terrestrial plant physiology. Gravity is perceived by sedimentation of starch-rich plastids (statoliths) to the bottom of the central root cap cells. Following gravity perception, intercellular auxin transport is redirected downwards leading to an asymmetric auxin accumulation at the lower root side causing inhibition of cell expansion, ultimately resulting in downwards bending. How gravity-induced statoliths repositioning is translated into asymmetric auxin distribution remains unclear despite PIN auxin efflux carriers and the Negative Gravitropic Response of roots (NGR) proteins polarize along statolith sedimentation, thus providing a plausible mechanism for auxin flow redirection. In this study, using a functional NGR1-GFP construct, we visualized the NGR1 localization on the statolith surface and plasma membrane (PM) domains in close proximity to the statoliths, correlating with their movements. We determined that NGR1 binding to these PM domains is indispensable for NGR1 functionality and relies on cysteine acylation and adjacent polybasic regions as well as on lipid and sterol PM composition. Detailed timing of the early events following graviperception suggested that both NGR1 repolarization and initial auxin asymmetry precede the visible PIN3 polarization. This discrepancy motivated us to unveil a rapid, NGR-dependent translocation of PIN-activating AGCVIII kinase D6PK towards lower PMs of gravity-perceiving cells, thus providing an attractive model for rapid redirection of auxin fluxes following gravistimulation.
eLife assessment
This fundamental study addresses the earliest events that enable plant roots to reorient growth in response to gravity. Compelling molecular and cell biological data establish that plasma membrane localization of the LAZY or NEGATIVE GRAVITROPIC RESPONSE OF ROOTS (NGR) protein family is required for rapid and polar redirection of D6 protein kinase, an activator of the PIN3 auxin transporter. This work complements and extends recent publications on the NGR family in gravity sensing (PMID: 37741279 and PMID: 37561884). Collectively these papers advance our understanding of rapid plant gravity sensing and response.
https://doi.org/10.7554/eLife.91523.3.sa0Introduction
Plants are capable of sensing gravity and adjust their organs’ growth direction accordingly. This phenomenon is called gravitropism, with positive gravitropism referring to the root growing down towards the Earth center and negative gravitropism describing the upward growth of the shoot (Kawamoto and Morita, 2022; Kolesnikov et al., 2016; Takahashi et al., 2021). The model plant Arabidopsis thaliana senses gravity in central root cap (columella) cells and the shoot endodermal cells and, in special gravity-sensing cells, which contain starch-enriched plastids – amyloplasts (Kiss et al., 1997; Kiss et al., 1996; Kiss et al., 1989; Morita, 2010). Due to their higher density, amyloplasts thus act as statoliths and sediment within cells (Sack, 1991). Following amyloplast sedimentation, the PIN exporters for the plant hormone auxin (PIN3 and PIN7) polarize towards the bottom cell sides, thus creating a downwards polarized auxin flow, which correlates with the asymmetric auxin accumulation at the lower side of the organ mediating the shoot/root curvature (Friml et al., 2002; Kleine-Vehn et al., 2010; Rakusová et al., 2011; Band et al., 2012). While sedimenting amyloplasts clearly serve as a signal for asymmetric auxin distribution, the precise mechanism remains elusive (Han et al., 2021; Kiss et al., 1989).
Recently, the LAZY protein family has been identified as a crucial player in the process of auxin redistribution and normal tropic response for both roots and shoots following gravistimulation (Jiao et al., 2021; Li et al., 2007; Yoshihara et al., 2013; Yoshihara and Iino, 2007). LZY1, LZY2, and LZY3 function redundantly in shoot gravitropism; LZY2, LZY3, and LZY4 - also referred to as NGR1, NGR3, and NGR2 (NEGATIVE GRAVITROPIC RESPONSE OF ROOTS) - regulate the root gravitropism (Kawamoto and Morita, 2022). ngr1,2,3 triple mutant plants display an anti-gravitropic phenotype with the root growing slightly upwards (Ge and Chen, 2016). This phenotype is accompanied by an inverted PIN3 polarization, thus redirecting the auxin flow to the upper side of the gravistimulated root (Ge and Chen, 2019; Taniguchi et al., 2017). Additionally, it was recently demonstrated that following gravistimulation, NGR3 localizes asymmetrically to the plasma membrane (PM) in columella cells of lateral roots (Furutani et al., 2020). However, the structure and molecular function of NGR proteins, as well as specific domains that could allow for PM association and polarization are still unknown. Polarization of NGR3 further acts as a precursor of PIN3 relocalization (Furutani et al., 2020). Additionally, PIN transport activity is fine-tuned by phosphorylation mediated by AGCVIII serine/threonine kinases like D6 PROTEIN KINASE (D6PK) (Barbosa and Schwechheimer, 2014). Whether additional factors play a role in this signaling cascade and how the underlying molecular mechanism connecting gravity sensing to asymmetric auxin distribution may look like, remains to be investigated.
Here, we provide novel insights into mechanisms of gravity-induced NGR1 polarization and downstream processes leading to PIN-dependent auxin fluxes activation and ultimately, gravitropic bending. We show the crucial function of polybasic regions (PBRs) and acylation of NGR1 for its binding to the PM and amyloplasts in columella cells, which is essential for its function in gravitropic bending. Pharmacological approaches show that the membrane composition affects NGR1 PM binding and polarization. Finally, we show rapid translocation of PIN activator – D6 protein kinase as an event downstream of NGR signaling. These observations suggest a model where statolith sedimentation is accompanied by rapid NGR relocation, which mediates similar relocation of the D6PK activator of PIN transporters, providing means to rapidly redirect asymmetric auxin fluxes in response to gravity.
Results
NGR1 localizes to statoliths and their sedimentation is accompanied by NGR1 polarization at the lower PM
To observe NGR localization in the columella cells, we cloned NGR1-GFP under its native promoter (NGR1p::NGR1-GFP) and transformed it into the ngr1/2/3 triple mutants. This construct fully rescued the agravitropic bending phenotype of the ngr1/2/3 triple mutant (see further), proving functionality of the GFP-tagged protein. NGR1-GFP displayed a highly specific columella expression, which was most prominent at the PM and the statolith periphery. NGR1-GFP signal from the PM was not evenly distributed, but rather polarized to the lower side of the columella cells in the vicinity of the sedimented statoliths (Figure 1A).
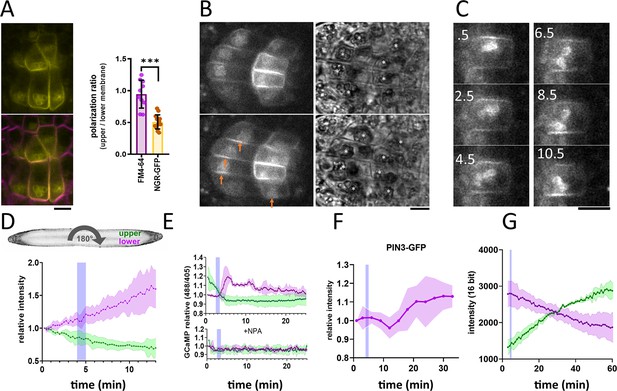
NGR1-GFP relocalization in the root columella.
(A) Colocalization of NGR1-GFP (yellow) with membrane stain FM4-64 (magenta). The statolith periphery is also decorated by the NGR1-GFP signal. NGR1-GFP is significantly polarized to the bottom area as quantified using upper/lower signal ratio (1=even distribution). (B) NGR1-GFP~30 s and 5 min after gravitropic bending. Orange arrows depict areas where the signal increased after contact with the statoliths. White stars in the transmission channel depict statoliths. (C) Time course of individual columella cell expressing NGR1-GFP. Numbers depict minutes from the 180° rotation. (D) Top: schematic depiction of the experiment design (valid for D-G). Bottom: Quantification of the NGR1-GFP signal at the lower and upper membranes. Similar setup was used for E. and F. Blue stripes in D-F represent intervals at which statoliths touched the bottom membrane. Average of five biological replicas. Shaded regions depict standard deviation (SD). (E) GCaMP signal after root gravitropic bending without (top) and with 20 µM NPA (bottom). The data represent three technical replicates, and three additional biological replicates were conducted, yielding similar results. Shaded regions depict SD.(F) PIN3 enrichment at the bottom of the central columella cells. The average is based on data from five biological replicas. Shaded regions depict SD.(G) DII Venus signal changes after root gravitropic bending. The average is calculated from three biological replicas. Shaded regions depict SD. Blue lines represent approximate time of statolith contact with the new bottom membrane. Bars = 10 µm.
-
Figure 1—source data 1
Data used for generating the graphs in the figure.
- https://cdn.elifesciences.org/articles/91523/elife-91523-fig1-data1-v1.xlsx
Constitutive overexpression of NGR1-GFP under 35 S promoter (35Sp::NGR1-GFP) confirmed that in multiple other cell types, NGR1-GFP localizes to the PM suggesting direct membrane binding mechanism. Additionally, NGR1 is associated with other plastid types such as chloroplasts in guard cells (Figure 1—figure supplement 1A). To separate the GFP signal from the chlorophyll, we used fluorescence lifetime imaging, which confirmed that NGR1-GFP localizes at the chloroplast periphery (Figure 1—figure supplement 1B). This implies a general plastid targeting mechanism of NGR. Notably, chloroplasts in the vicinity of the PM strongly correlated with NGR1 accumulating at the PM nearby, similar to the scenario in columella (Figure 1—figure supplement 1A and C).
This shows that NGR1-GFP localizes to the plastids and PM in their vicinity providing a mechanism for how gravity-induced statolith sedimentation can polarize NGR1-GFP to the bottom PM.
Timing of events during the gravitropic response
Next, we tracked the timing of events during gravitropic bending. For that, we used a vertical stage microscope with a rotation stage allowing us to perform gravistimulation while imaging and minimize the time required for rotating the sample. For the gravistimulation, roots, initially located vertically, were rotated 90° to the horizontal position.Further gravistimulation was performed by flipping the root 180° (with the root tip from pointing left to pointing right, or vice versa). 180° rotations were performed during the imaging thus the precise timing could be determined from the individual frame timestamps. Following gravitropic stimulus, NGR1 at the PM relocalized dynamically with the falling statoliths (Figure 1B). First, the signal at the top membrane decreased and then reached the bottom PM together with statoliths, around 5 min after the stimulation (Figure 1C and D). If the root was rotated 180° from the vertical position (from root tip pointing down to pointing up), the time required for the statolith sedimentation has increased due to the cell’s elongated shape and the presence of vacuole. In such a scenario, it took more than 15 min for the statoliths to fall. Again, the increase of the NGR1 signal at the bottom side correlated with the statolith occurrence, implying that statolith vicinity is the region where NGR1 signal gets enriched (Figure 1—figure supplement 1D). When monitored for an extended period of time, NGR1 signal was always decorating the PM proximal to the statolith (Video 1).
NGR-GFP protein localizes in the vicinity of statoliths during the course of root gravitropic bending.
To put these early gravitropic events in the context of the auxin gradient formation, we tracked PIN3 localization dynamics, intracellular calcium transients, and auxin reporter DII-Venus (Brunoud et al., 2012). Calcium transients are one of the fastest known auxin response events (Monshausen et al., 2011) and we monitored them using plants stably transformed with GCaMP3 fluorescent sensor (Zariwala et al., 2012). While the signal increase at the bottom side of the root was visible within a minute after statoliths touched the bottom membrane, its decrease at the top PM started immediately after rotation. This implies that the first signaling event in the root gravitropic bending is the statolith removal from the top membrane, rather than its arrival at the bottom (Figure 1E). While subsequent changes in the DII-Venus degradation were consistent with the calcium-based timing, the first visible PIN3 polarization to the bottom columella cell sides was detectable only after 15 minutes (Figure 1F and G). Nonetheless, already the earliest auxin asymmetry is likely generated by PIN-mediated polar auxin transport as it was completely blocked by the treatment with N-1-naphthylphthalamic acid (NPA) (Figure 1E), a PIN inhibitor (Abas et al., 2021).
To summarize, statolith sedimentation is accompanied by rapid NGR1 relocation to the PM subdomain proximal to the NGR1-containing statoliths. The first event in gravitropic response is the NGR1 leaving the top membrane accompanied by decrease in the auxin signaling at the upper root side, followed by NGR1 arrival at the bottom membrane and increase in auxin signaling at the bottom side. This initial auxin asymmetry is mediated by PIN-dependent auxin transport, despite visible polarization of PIN3 can be detected only later.
NGR1 polarization does not require BFA-sensitive trafficking
To gain more insight into the dynamics of the NGR1 localization to the PM and amyloplasts, we tested different inhibitors to alter membrane composition and interfere with endocytic trafficking. Recently, it has been shown that RCC1-like domain (RLD) proteins directly interact with NGRs and play a crucial role in polar auxin transport during gravity signaling (Furutani et al., 2020). As RLD trafficking is known to be sensitive to the ARF-GEF inhibitor Brefeldin A (BFA) (Furutani et al., 2020; Wang et al., 2022), we examined if the drug also affects the localization and polarization of NGR1. Five-day-old NGR1p::NGR1-GFP seedlings were incubated with 50 µM BFA together with the protein synthesis inhibitor, cycloheximide (CHX, 50 µM) and the endocytic tracer FM4-64 (2 µM) as described previously (Geldner et al., 2001). Interestingly, while FM4-64-stained membranes accumulated into so-called BFA compartments, NGR1-GFP did not and still showed association with the PM and amyloplasts (Figure 2A–C). Furthermore, the polarization of NGR1 following gravity stimulation was not disrupted in the presence of BFA. Similar effects were observed in overexpressed NGR1 as it also did not show co-localization with BFA aggregates (Figure 2—figure supplement 1A–C).
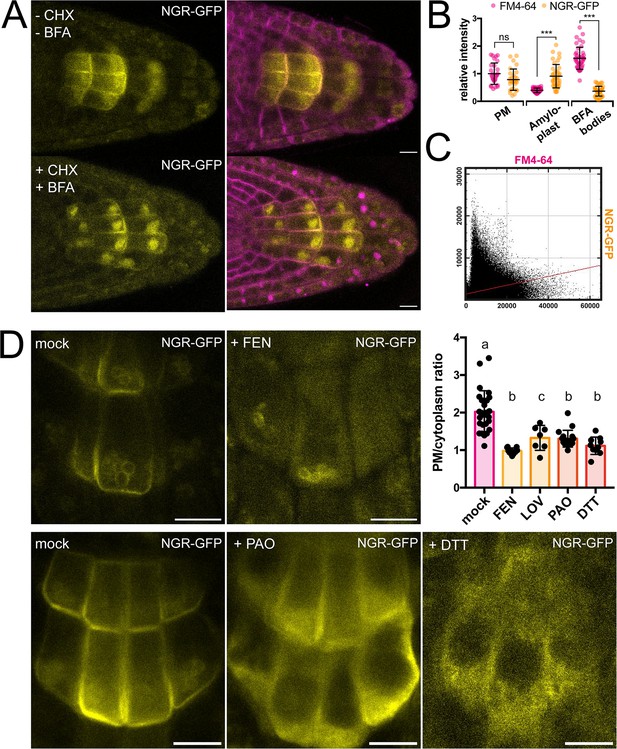
Role of trafficking and PM composition in NGR1 localization.
(A) Representative images of NGR1p::NGR1-GFP roots treated with 50 µM CHX, 50 µM BFA, and 2 µM FM4-64; mock: DMSO; NGR1-GFP in yellow, FM4-64 in magenta. Three biological replicas were made with similar results. Error bars represent SD. (B) Fluorescence intensity of FM4-64 and NGR1-GFP at the PM (n=29), amyloplasts (n=39) and BFA bodies (n=42) normalized to the average fluorescence intensity of FM4-64 at the PM. Data is pooled from columella cells of the representative experiment shown in A. n equals measured points. Statistical measurements were done with one-way ANOVA; *** equals adj. p-value of <0.0001. (C) Cytofluorogram of colocalization of FM4-64 and NGR1-GFP from the A using Pearson’s coefficient. (D) NGR1p::NGR1-GFP roots treated with FEN, PAO, and DTT. PM/cytoplasm ratio was calculated for mock (n=26), FEN (n=11), LOV (n=7), PAO (n=18), and DTT (n=10) treated seedlings. n equals the number of cells evaluated. Using one-way ANOVA, b is significantly different from a with an adj. p-value of <0.0001, c is significantly different from a with an adj. p-value of 0.006. Bars = 10 µm.
-
Figure 2—source data 1
Data used for generating the graphs in the figure.
- https://cdn.elifesciences.org/articles/91523/elife-91523-fig2-data1-v1.xlsx
This shows that NGR1 localization and gravity-induced polarization does not undergo BFA-sensitive endocytic recycling mediated by the ARF-GEF GNOM as it has been shown for other proteins involved in gravity response like RLDs and PINs (Geldner et al., 2001; Naramoto et al., 2014; Wang et al., 2022).
NGR1 PM association requires specific membrane composition
NGR1 displays PM localization although transmembrane domains are not predicted in its structure and it does not undergo BFA-sensitive trafficking. This implies the existence of posttranslational modifications such as S-acylation or N-myristoylation to associate with PM. For initial insight we used GPS Lipid prediction tool (Xie et al., 2016), which navigated us towards S-acylation. To test for the presence of S-acylation sites, we utilized the reducing properties of Dithiothreitol (DTT), which interferes with the formation of the thioester bond thus effectively preventing S-acylation (Sabol et al., 2017). Following previously described conditions, NGR1-GFP showed an increase in cytoplasmic signal and reduction of PM association (Figure 2D). Similar effect of DTT was observed when expressing NGR1 under the 35 S promoter, suggesting that there is indeed an acylation site (Figure 2—figure supplement 1D–E). Interestingly, NGR1 still localizes to amyloplasts, indicating a different way of plastid association compared to the PM.
Next, we tested whether apart from the NGR1 S-acylation, the membrane composition itself might be essential for the NGR1 PM association. Previously, it has been shown that PBRs of proteins can target them to anionic phospholipids like PtdIns(4)P (PI4P) (Hammond et al., 2012; McLaughlin and Murray, 2005). We tested whether the depletion of PI4P from the PM will affect the NGR1 PM association. NGR1p::NGR1-GFP seedlings were incubated for 30 min with 30 µM phenylarsine oxide (PAO), a PtdIns(4)-kinase inhibitor (Hammond et al., 2012; Vermeer et al., 2009). The NGR1-GFP cytosolic signal was significantly increased, indicating that the interplay between PBRs and phospholipids is important for NGR1 PM but not amyloplast association (Figure 2D).
Next, we wanted to determine whether sterols might influence the PM association of NGR1. NGR1-GFP seedlings were grown on fenpropimorph (FEN), an inhibitor of sterol biosynthesis that decreases the number of sterols and can trigger their conversion into cyclopropyl sterols (Frescatada-Rosa et al., 2014). NGR1-GFP showed an increase in cytoplasmic signal and membrane localization was largely lost (Figure 2D). Comparable effects were also observed after treatment with another sterol synthesis inhibitor lovastatin (LOV) (Figure 2D; Figure 2—figure supplement 1F). However, similarly to DTT and PAO, amyloplast localization could not be disturbed.
These observations show that NGR1 PM but not necessarily plastid localization depends likely on acylation, phospholipid, and sterol membrane composition.
NGR1 PM localization is synergistically mediated by PBRs and a palmitoylation site
To further study NGR1 membrane localization, we examined the amino acid sequence of the NGR1 to understand which sites of the protein mediate its association with the membrane. We noticed 2 PBRs K113K114R115K116 (PBR1) and K186K187K188R189 (PBR2) – these strands of positively charged amino acids may mediate the interaction of NGR1 with negatively charged phospholipids. Also, we noticed a conserved Cys206 residue – a potential S-palmitoylation (PALM) site according to the GPS-Lipid post-translational modification prediction tool (Xie et al., 2016).
To test the role of these sites in the NGR1 membrane localization we performed site-directed mutagenesis based on the NGR1p::NGR1-GFP construct. We introduced mutations K114E&R115E (-PBR1), and K187E&K188E (-PBR2) to the PBRs of the NGR1 to neutralize their positive charge, and mutated the conserved cysteine C206A (-PALM) to abolish the potential palmitoylation site (Figure 3A). NGR1-PALM-GFP, NGR1-PBR2-GFP, and NGR1-PBR1&2-GFP under the native NGR1 promoter were transformed into the ngr1/2/3 background. These mutant versions of the NGR1 restored the anti-gravitropic root phenotype of the ngr1/2/3 triple mutant similar to the NGR1-GFP version without mutations (Figure 3—figure supplement 1A). The NGR1-PALM version resembled the root bending of the NGR1WT-GFP version, and NGR1-PBR2 and NGR1-PBR1&2 versions demonstrated a slight delay in the main root angle restoration after reorientation in the vertical scanner setup (Figure 3—figure supplement 1B). Then we performed confocal imaging of the root columella cells to check the localization of the NGR1-PALM-GFP, NGR1-PBR2-GFP, and NGR1-PBR1&2-GFP proteins (Figure 3—figure supplement 1C). To compare the different mutant versions, we quantified the PM/cytoplasm signal ratio. The PM/cytoplasm ratio was slightly decreased for NGR1-PALM-GFP, NGR1-PBR2-GFP, and NGR1-PBR1&2-GFP mutants compared to NGR1WT-GFP (Figure 3—figure supplement 1D).
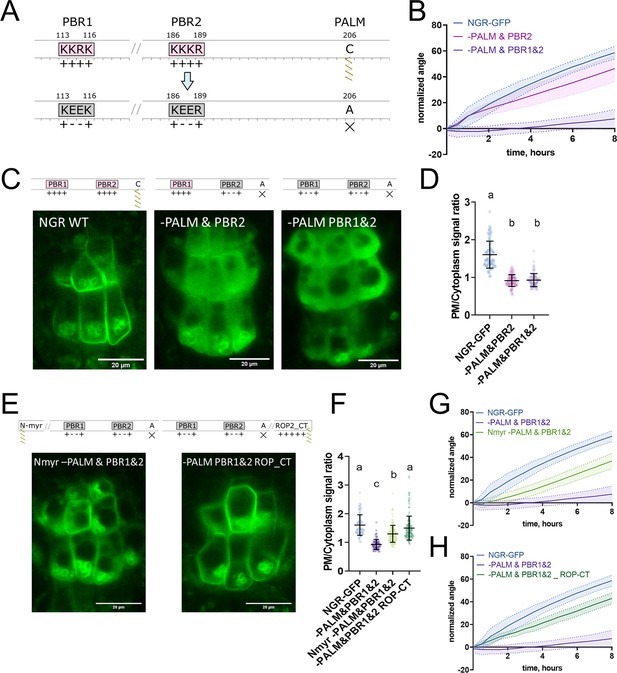
Polybasic regions and a palmitoylation site mediate NGR1 membrane localization.
(A) Scheme of mutagenesis. K114E&R115E (-PBR1), K187E&K188E (-PBR2), and C206A (-PALM) were introduced to disrupt NGR1 polybasic regions and palmitoylation site. Different combinations of mutations were tested. (B) Main root angle restoration upon reorientation in the vertical scanner setup of the ngr1/2/3 triple mutant complemented with NGR1p::NGR1-PALM&PBR2-GFP and NGR1p::NGR1-PALM&PBR1&2-GFP in comparison to NGR1p::NGR1WT-GFP, Col-0 and ngr1/2/3 triple mutant. (C) Representative confocal microscopy images of the root columella cells of NGR1p::NGR1-GFP, NGR1p::NGR1-PALM&PBR2-GFP, and NGR1p::NGR1-PALM&PBR1&2-GFP. (D) PM/cytoplasm signal ratio for NGR1p::NGR1-GFP, NGR1p::NGR1-PALM&PBR2-GFP, and NGR1p::NGR1-PALM&PBR1&2-GFP variants. Mutation in the polybasic region and palmitoylation site leads to the dissociation of NGR1 protein from the PM. For statistical analysis one-way ANOVA test was used, ‘a’ is significantly different from ‘b’ with a p-value <0.0001. The difference between the PM/Cytoplasm signal ratio of NGR1p::NGR1-PALM&PBR2-GFP and NGR1p::NGR1-PALM&PBR1&2-GFP is not statistically significant. (E) Representative pictures of the root columella cells of NGR1Nmyr -PALM&PBR1&2-GFP and NGR1-PALM&PBR1&2 ROP-CT-GFP. Membrane localization of NGR1-PALM&PBR1&2-GFP is restored upon the addition of LZY1 N-terminal myristoylation site (left) or C-terminal region of ROP2 bearing polybasic region and geranyl-geranylation site (right). (F) PM/cytoplasm ratio quantified for the versions with another acylation site. Different letters designate the statistically significant difference with p<0.0001 in a one-way ANOVA test. For each genotype >35 cells were quantified. (G) Rescued main root angle restoration upon reorientation in the vertical scanner setup of the NGR1p::NGR1Nmyr -PALM&PBR1&2-GFP in ngr1/2/3 line, after the addition of LZY1 N-myristoylation site to the NGR1 version with mutated polybasic regions and palmitoylation site. (H) Rescued gravitropic response of the NGR1p::NGR1-PALM&PBR1&2 ROP-CT-GFP in ngr1/2/3, with the addition of the C-terminus of the ROP2 after the GFP tag. For all the mutant variants more than five separate transgenic lines were observed with similar results. 8–15 roots were quantified for each gravitropic bending experiment. Shaded regions depict SD.
-
Figure 3—source data 1
Data used for generating the graphs in the figure.
- https://cdn.elifesciences.org/articles/91523/elife-91523-fig3-data1-v1.xlsx
As the mutations only in the palmitoylation site or only in the PBRs of the NGR1 did not lead to the significant disruption of the plant gravitropic response and the dissociation of the protein from the membrane, we combined these mutations to check their collective effect. We generated NGR1-PALM&PBR2-GFP with a mutation in the PALM site and the PBR2, and NGR1-PALM&PBR1&2 with a mutation in both PBR1 and PBR2 and PALM site. NGR1p::NGR1-PALM&PBR2-GFP and NGR1p::NGR1-PALM&PBR1&2-GFP could not fully complement ngr1/2/3 triple mutant. The main root of the 5 d.o. seedlings demonstrated inclination from the vertical angle (Figure 3—figure supplement 1E). In contrast to NGR1-GFP complemented ngr1/2/3 plants, complementation with NGR1-PALM&PBR2 displayed partially compromised restoration of the main root angle upon reorientation, while the NGR1-PALM&PBR1&2 root bending was completely disrupted (Figure 3B). With confocal microscopy we observed the dissociation of the NGR1-PALM&PBR2 and NGR1-PALM&PBR1&2 from the PM (Figure 3C). PM/cytoplasm ratio was significantly decreased for both NGR1-PALM&PBR2 and NGR1-PALM&PBR1&2 compared to non-mutated NGR1-GFP (Figure 3D). Taken together, the combination of mutations in PBRs and palmitoylation site resulted in the decrease of NGR1-GFP PM binding and loss of the protein functionality.
If the selected PBRs and palmitoylation site represent solely the mean of the PM anchoring, then bringing the protein back to the PM by different targeting signal should restore its functionality. To test this hypothesis, we introduced another membrane-association site to the mutant versions of NGR1-GFP. It was shown that the N-terminus of LZY1 mediates its membrane localization potentially via a predicted N-myristoylation site (Yoshihara and Spalding, 2020). This site is not conserved in the NGR1. Remarkably, LZY1 does not possess the palmitoylated Cys residue conserved in NGR1 (Figure 3—figure supplement 2A). We changed the N-terminus of all the NGR1 mutant versions carrying the mutation C206A to introduce the LZY1 N-terminal myristoylation site. The obtained NGR1Nmyr -PALM-GFP, NGR1Nmyr -PALM&PBR2-GFP, NGR1Nmyr -PALM&PBR1&2-GFP versions under NGR1 native promoter were transformed to the ngr1/2/3.
As an alternative, we used a well-characterized membrane-association sequence from the C-terminus of ROP2 (ROP-CT) carrying a PBR and geranyl-geranylation site (Figure 3—figure supplement 2B; Lin et al., 1996; Yalovsky et al., 2008). Similarly, as for the N-myristoylation site, we added the ROP-CT on the C-terminus after the GFP tag to all the variants with the mutated PALM site. The resulting NGR1p::NGR1-PALM ROP-CT-GFP, NGR1p::NGR1-PALM&PBR2 ROP-CT-GFP, NGR1p::NGR1-PALM&PBR1&2 ROP-CT-GFP were transformed into ngr1/2/3 background. PM localization and gravitropic response of the versions with another acylation site were restored gradually (Figure 3—figure supplement 2C–H).
We examined the localization of the GFP signal in the columella of both restored lines (NGR1p::NGR1Nmyr -PALM&PBR1&2-GFP and NGR1p::NGR1-PALM&PBR1&2 ROP-CT-GFP). NGR1Nmyr -PALM&PBR1&2 membrane association was partially restored, and NGR1-PALM&PBR1&2 ROP-CT membrane association was fully restored (Figure 3E and F). In accordance with PM localization, the gravitropic response of those plants was also restored (Figure 3G and H).
Thus NGR1 PM localization is mediated synergistically by PBRs and palmitoylation site, and the NGR1 PM association is crucial for NGR1 functioning in root gravitropic response.
D6PK relocalizes in columella following gravistimulation in NGR1-dependent manner
NGR1 protein relocalization occurs simultaneously with statolith sedimentation and is rapidly followed by PIN-dependent asymmetric auxin accumulation at the lower root side. These events, however, precede a visible PIN3 polarization towards the bottom columella cell sides (Figure 1D–G). Alternatively, we hypothesized that asymmetric PIN activation at this position may precede the PIN polarization there and mediate the early auxin asymmetry. To test this, we analyzed the localization of D6PK, a well-established PIN3 activating kinase (Willige et al., 2013). We monitored D6PK under its native promoter with N-terminal YFP and mCHERRY (mCH) tags. This revealed a highly dynamic polarized D6PK localization following gravistimulation. Following 180° rotation, YFP-D6PK and mCH-D6PK displayed a very clear translocation to the new bottom side of the columella cells (Figure 4A–C). To resolve whether this polarization depends on NGR proteins, we transformed mCHERRY-D6PK (mCH-D6PK) to both ngr1/2/3 triple mutants and Col-0 wild type plants. Notably, following gravistimulation, D6PK polarization was absent in ngr1/2/3 triple mutants (Figure 4C–D, Video 2). Surprisingly, other cell types such as meristematic cells or epidermal cells still displayed undisturbed basal (WT-like) D6PK polarity in ngr1/2/3 triple mutant suggesting a role of NGRs in D6PK targeting specifically during gravity response (Figure 4C). To compare D6PK translocation with other events during gravity response, we generated a movie, where polarization of D6PK is compared with the calcium transients, PIN3 mobility, and DII Venus signal (Video 3).
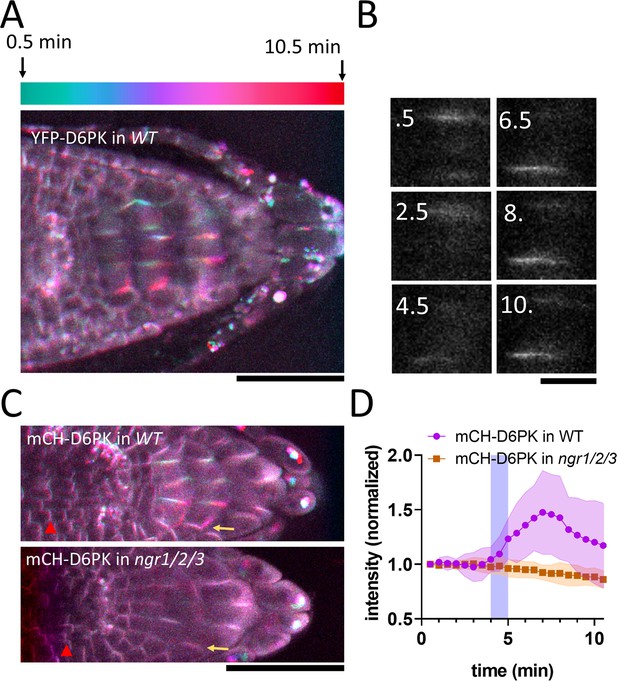
NGR-dependent D6 protein kinase translocation.
(A) Temporal color-coded image of YFP-D6PK translocation induced by 180° rotation. (B) Single gravistimulated (180°) columella cell undergoing YFP-D6PK translocation. White numbers indicate time after rotation (min). (C) mCH-D6PK 10 min after gravistimulation in WT and ngr1/2/3 triple mutant, same temporal color code as in A. Yellow arrow depicts the new bottom membrane. Red arrowhead depicts meristematic cells, which displayed basal D6PK polarity in both genotypes. (D) Quantification of D6PK signal at the columella cell bottom PM upon 180° rotation. Blue strip depicts approximate time of statolith contact with the bottom PM. Bars = 50 µm (A,C) 10 µm (B). Ten cells out of three roots were quantified. Experiment was replicated three times with similar results. Shaded regions depict SD.
-
Figure 4—source data 1
Data used for generating the graphs in the figure.
- https://cdn.elifesciences.org/articles/91523/elife-91523-fig4-data1-v1.xlsx
mCHERRY-D6PK in WT and ngr1/2/3 mutant upon gravitropic stimulus.
Summary of the timing of gravitropic bending for YFP-D6PK, PIN3, calcium transients, and DII-Venus responses during a series of 180° flips.
Taken together, we identified D6PK, a PIN activating kinase to be translocated towards the bottom columella cell sides in an NGR-dependent manner. This provides a plausible mechanism for rapidly redirecting auxin fluxes prior to PIN relocation during root gravitropic response.
Discussion
In this study, we provide several new crucial insights into the mechanism through which plant roots adapt their growth in response to gravity: (i) we mapped the early events after gravistimulation with unprecedented time resolution challenging the current gravitropic models; (ii) we observed real-time relocalization of the key gravitropic regulator, NGR1 on and alongside the descending statoliths and (iii) mapped the conditions for NGR1 crucial association with the PM; and, finally, (iv) we detected rapid, NGR1-dependent relocation of D6PK auxin transport activator. This altogether suggests a new mechanism for rapid redirection of auxin fluxes following gravistimulation.
Early events during root gravitropic response
The temporal correlation between NGR1 relocation and auxin asymmetry dynamics was tested by monitoring auxin-dependent calcium transients. Interestingly, while an auxin-induced calcium wave can be observed following statolith descent to the bottom side of the gravity-sensing cells, preceding this, a decline in calcium transients occurs at the upper root side. These observations suggest that the first signaling event in root gravitropic bending may not be statolith contact with the cell bottom, but rather the departure of statoliths from the upper PM, closely followed by NGR1 and D6PK translocation to the bottom cell sides. Only significantly later, similar polarization of PIN auxin exporters can be confidently observed. This may be reflected by actual onset of the root bending, as there is growing evidence, that the actual root bending begins much sooner than previously reported, in fact, some degree of bending was observed already within the first 5 min following gravistimulation (Dubey et al., 2023; Serre et al., 2023).
Insights into NGR membrane association and gravity-induced polarization
NGR-GFP relocation to the new bottom membrane happens rapidly and is not affected when ARF GEF-mediated endomembrane trafficking is inhibited. Although the precise mechanism of NGR1 dynamic relocation following gravitropic stimulus still remains elusive, this study brings some new insights. The NGR1 membrane anchoring mechanism is dependent on cysteine acylation and adjacent polybasic stretches, which interact with charged lipids at the membrane surface. Such a mechanism is typical for a plethora of proteins (Hemsley and Grierson, 2008). Since we could restore the protein functionality by adding two different membrane anchors on the NGR1 N and C terminus, we conclude that the mechanism of membrane targeting is rather universal. Point mutated NGR1 proteins which lost their membrane binding ability still retained the statolith binding, implying an independent targeting mechanism. Therefore, we can conclude that statolith-bound NGR1 is not sufficient for the NGR1 function in gravitropism. On the other hand, NGR amyloplast association is apparently an essential aspect of the NGR mobility. While writing this manuscript, two concurrent articles have addressed the mechanism of NGR starch grain association and its translocation to the plasma membrane. These studies reveal that the mobility of the NGR (LAZY4) protein depends on the N-terminal transit peptides. The association of LAZY4 with the translocons in the outer plastid membrane is modulated by mitogen-activated protein kinases, facilitating its dynamic transfer to the plasma membrane (Nishimura et al., 2023; Chen et al., 2023) with a specific lipid composition.
Our pharmacological experiments indicate that both sterols and charged lipids play a role in the NGR membrane binding. This resembles other highly polarized structures such as root hairs, where sterols and phosphatidylinositol 4,5-bisphosphate are vital for the polarity (Champeyroux et al., 2020; Ovecka et al., 2010). Furthermore, PIN2 polarity was defective in sterol synthesis mutants, implying the role of sterols in planar polarity as well (Men et al., 2008). Whether there is mobile sterol-rich domain moving alongside the statolith or the membrane composition is uniform remains to be elucidated.
Gravity-induced D6PK kinase polarization as mechanism for rapid auxin fluxes redirection
PIN auxin transporters were shown to polarize following gravistimulation to the bottom of gravity-sensing cells (Friml et al., 2002; Furutani et al., 2020; Kleine-Vehn et al., 2010) providing a mechanism for redirection of auxin fluxes downwards. Although PIN polarization has been shown to be reliant on NGR proteins (Ge and Chen, 2019), the timing of early gravitropic events resolved here suggests that it occurs too late to account for the initial auxin asymmetry. Either, we are unable to detect early, less-pronounced PIN asymmetry or there is an additional mechanism preceding the auxin flux redirection via PIN relocation.
Our discovery of similar gravity-induced polarization of D6PK AGCVIII kinases, which is contingent on NGR activity, bridges this critical knowledge gap and suggests that, prior to relocation of PIN transporters, AGCVIII-mediated PIN activation at the lower cell sides rapidly redirects auxin fluxes following gravity perception. Notably, PIN3 phosphorylation is important for gravity response (Grones et al., 2018) and higher order D6PK mutants display mild gravitropic bending phenotypes (Zourelidou et al., 2009), which are, however, weaker than ngr1/2/3 mutants. This observation might be attributed to the multiplicity of the AGC kinase family and/or an additional mechanism governing the differential accumulation of PIN proteins, following D6PK polarization, thus contributing to the regulation of auxin flux.
Similarly to NGRs, D6PK membrane localization depends on PBRs and sterol-rich environments (Barbosa et al., 2016; Stanislas et al., 2015). However, in contrast to NGR proteins, D6PK localization is dependent on BFA-sensitive trafficking regulator GNOM (Barbosa et al., 2016; Barbosa et al., 2014) and thus is likely delivered to the PM by vesicle trafficking. RLD proteins, implicated in the regulation of the GNOM-dependent PIN trafficking pathway, are directly recruited by NGR homolog NGR3 (LZY3; Furutani et al., 2020). Notably, RLD proteins contain the BRX domain, which is responsible for the NGR interaction (Furutani et al., 2020). In the different cellular context, BRX protein was shown to be an inhibitor of AGC kinase (PAX) induced PIN3 activation and an interactor of multiple D6PK proteins (Marhava et al., 2018). This implies that BRX containing proteins may act as negative feedback on PIN phosphorylation after gravistimulation. Indeed, our observations indicate that the D6PK signal at the new bottom membrane reaches its maximum approximately 7–8 min after gravistimulation and subsequently diminishes, hinting at the existence of a negative feedback mechanism. The involvement of RLDs in this process remains to be investigated.
Taken together, an updated model of early events in root gravity-sensing cells emerges: (i) Statoliths sediment and bring associated NGR proteins to the lower PM; by a mechanism requiring electrostatic interaction-based association of NGR with the PM; (ii) dependent on these events, the D6PK translocates almost concomitantly also to the bottom cell sides, likely by GNOM-dependent trafficking mechanism; (iii) D6PK activates PINs at the lower side, auxin transport get redirected downwards reflected by a decrease of auxin response at the upper side followed by auxin response increase at the lower root side; (iv) D6PK asymmetry gradually diminishes, possibly by action of BRX domain-containing proteins and the whole PIN-dependent auxin transport machinery gradually relocates to the lower cell side for more sustained redirection of auxin fluxes. There are still many mechanistic links to be confirmed and clarified in this model but it is consistent with all observations on the sequence of events following gravistimulation and with all additional data from other developmental contexts.
Materials and methods
Plant cultivation
Request a detailed protocolAll Arabidopsis mutants and transgenic lines which were used in this project are in the Columbia-0 (Col-0) background. NGR1p::NGR1-GFP, 35Sp::NGR1-GFP, and D6PKp::mCH-D6PK were generated as part of this research project. D6PKp::YFP-D6PK has been described previously (Zourelidou et al., 2009). Overnight seed sterilization was executed with chlorine gas. Seeds were sown on solid agarose media – half-strength Murashige and Skoog medium supplemented with 1% sucrose (AM+) and 0.8% phyto agar (pH = 5.9) – stratified at 4 °C for 2 days and subsequently grown vertically at 21 °C with 16 hr light/8 hr dark cycles.
Cloning strategy
Request a detailed protocolNGR1 promoter was amplified from genomic DNA using the primer pairs NGR1p-B4-FP and NGR1p-B1r-RP, and was cloned into pDONR-P4P1r. NGR1-GFP fusion fragment was obtained by overlapping PCR using the prime pairs NGR1g-B1-FP and NGR1g-L-RP to amplify the NGR1 protein-coding region with genomic DNA as the template and the primer pairs eGFP-L-FP and eGFP-B2-RP to amplify the eGFP coding sequence, with a PKPA protein linker inserted between NGR1 and GFP, and the resulting fragment was cloned into pDONR221. The resulting pENTRY clones were recombined into pH7m24GW to get the final expression construct NGR1p::NGR1-GFP. The pENTR clone NGR1-GFP in pDONR221 was recombined with pH2GW7 to get the construct 35Sp::NGR1-GFP.
To obtain desired point mutations in PBRs and acylation sites, Mutagenesis of NGR1p::NGR1-GFP was performed using Gibson assembly (NEB HiFi DNA assembly E2621L), overlapping compatible cohesive ends were modified. For ROP C-terminus, an oligo-bridge Gibson assembly was performed with an oligonucleotide containing the entire ROP2 hypervariable region. Plants were transformed with floral dip method (Zhang et al., 2006).
NGR1 transformants were selected on plates containing 30 µg/ml hygromycin. NGR1p::NGR1-GFP plants with genotyped with primers gNGRmut-NGR1prom-fwd and gNGRmut-T35S-rev, and the middle part of the NGR1 was sequenced to confirm the presence of the mutations.
D6PKp::mCH-D6PK was generated using the Greengate cloning strategy. Greengate entry blocks were generated with the use of the Gibson assembly (pGGA-D6PK promotor) and restriction-ligation assembly (pGGC-D6PK). Two KB fragment upstream of D6PK start codon was used and the BsaI site was removed using point mutated compatible cohesive ends for the Gibson assembly between fragments A and B. Other building blocks used were: pGGB-mCHERRY-linker (pGGB001), pGGD-Dummy (pGGD002), pGGE UBQ10 terminator (pGGE009), pGGF-d-AlanineR (pGGF003), destination vector (pGGZ001 modified to contain bacterial kanamycin resistance; Lampropoulos et al., 2013), plasmids were obtained from Addgene or were kindly provided by Dr. Andrea Bleckmann. pD6PK::mCH-D6PK transformants bearing DAO-selection cassette were selected on the 3 mM D-Alanine.
Microscopy
Request a detailed protocolFor imaging, vertically mounted Zeiss LSM800 was used with air objectives Plan Apochromat 20 x/0.8 M27 and Plan Apochromat 10 x/0.45 M27 (Figure 1G, Video 3). Air objective Plan Apochromat 20 x/0.8 M27 and water objective Plan-Apochromat 40 x/1.2 M27 were used in Figure 2, Figure 1—figure supplement 1, Figure 2—figure supplement 1, Figure 3, Figure 3—figure supplements 1 and 2, Figure 4, Video 2 and Video 3. Plan apochromat 60x1.4 Oil objective was used for the airyscan imaging (Figure 1—figure supplement 1C). For fluorescence lifetime imaging (Figure 1—figure supplement 1B), Leica Stellaris 5 with Plan-Apochromat 40 x/1.2 M27 was used. GCaMP signal was imaged using GFP emission (500–540 nm) using 405 nm and 488 nm excitation lasers. Signal obtained by 405 nm excitation was used as a ratiometric reference. For the gravitropic experiments, brightfield images were always taken together with the fluorescent probes to determine the statolith contact with basal PM. This was determined as a time at which statolith movement relative to the cell surface stopped.
Plant treatments
Request a detailed protocolThe following inhibitors were dissolved in DMSO: Brefeldin A (Sigma B7651); Cycloheximide (Sigma C1988); PAO (Sigma P3075); FEN (Sigma 36772); LOV (Sigma PHR1285); NPA (DUCHEFA N0926). BFA treatment was performed as described previously (Geldner et al., 2001). In brief, seedlings were submerged in liquid AM + medium and pre-treated with 50 µM CHX for 30 minutes. Subsequently, they were incubated with 50 µM BFA and 50 µM CHX for 75 minutes. Then, 2 µM FM4-64 (Invitrogen T13320) was added and co-incubated with 50 µM BFA and 50 µM CHX for 15 minutes. Treatment with PAO was executed as described previously Barbosa et al., 2016; seedlings were incubated in 30 µM PAO-containing liquid AM + medium for 20 min, followed by the addition of 2 µM FM4-64 and another 10-min incubation. For quantification purposes, 5-day-old seedlings grown on AM + plates with 1 µM LOV and 250 µg/ml FEN were utilized (Stanislas et al., 2015). Representative images were captured of 5-day-old seedlings that were initially grown on AM + and subsequently transferred to 1 µM LOV and 50 µg/ml FEN for a duration of 2 hr. To treat with DTT, seedlings were incubated in liquid AM + medium supplemented with 50 mM DTT (Sigma; dissolved in H2O) for 3 hr, followed by a 10-min incubation with 2 µM FM4-64 (Sabol et al., 2017). Unless otherwise stated, treated seedlings were mounted in liquid AM + medium for live imaging. As a control for used inhibitors, solvent control treatments were performed in parallel.
Data analysis
Request a detailed protocolTo quantify signals in the individual cells, time series were stabilized by Correct 3D drift Fiji plugin. All image data was obtained in 16-bit format. ROI were selected and measured using Microsoft Excel and GraphPad Prism. Data was normalized so that the first value = 1 (Figures 1A, D, F, 4D) or by using reference channel (Figures 1E and 2B).
In order to analyze subcellular colocalization we utilized the tool JACoP (Just Another Co-localization Plugin), that allows for a correlation of pixels of dual-channel images based on Pearson’s coefficient (Bolte and Cordelières, 2006).
Gravitropic bending experiments were performed in the vertically oriented scanner setup. 4 d.o. seedlings were transferred to a new solid agarose media plate and aligned so that the roots were straightened. Images obtained with 30 min intervals were registered with the Fiji StackReg plugin (Schindelin et al., 2012). Roots were manually tracked and angle increments were calculated using Microsoft Excel.
For the PM/cytoplasm ratio quantification, the background of the confocal images was subtracted; with the multi-point selection tool 15–50 points on the PM, and 15–50 points in the cytoplasm were selected, and the intensity of the GFP signal was measured.
The location of the PM membrane was confirmed with the FM4-64 staining. The PM region was selected on the bottom side of the cells, as NGR1 is localized polarly. The cytoplasmic region was selected close to the selected membrane region and did not include the region with amyloplasts and the vacuole. For individual cells the averaged PM signal was divided by the averaged cytoplasmic signal. The PM/cytoplasm ratio for the lines is quantified as the average of the PM/cytoplasm ratios for individual cells of this line.
If not stated otherwise, all experiments were performed in triplicate. Three or more independent transgenic lines were always used with similar results. All error bars and shaded areas around average represent SD.
List of primers used in the study
Request a detailed protocolPrimer name | Primer sequence |
---|---|
Transgenic line preparation | |
NGR1p-B4-FP | GGGGACAACTTTGTATAGAAAAGTTGGAAGAGGAGAAGGTGGGAGAGC |
NGR1p-B1r-RP | GGGGACTGCTTTTTTGTACAAACTTGTGTTTCTTTTTTTCTGACAATTGACTG |
NGR1p-S-F1 | ACTAAACAACCCCTTTTGAAACC |
NGR1p-S-F2 | CAAGATTGAAAAACTATTTGCCCT |
NGR1g-B1-FP | GGGGACAAGTTTGTACAAAAAAGCAGGCTCCATGAAGTTCTTCGGGTGGATG |
NGR1g-L-RP | CACCATAGCAGGCTTAGGTATCTCGAGAACTATGACTGTAATCA |
eGFP-L-FP | GAGATACCTAAGCCTGCTATGGTGAGCAAGGGCGAGGAG |
eGFP-B2-RP | GGGGACCACTTTGTACAAGAAAGCTGGGTGCTACTTGTACAGCTCGTCCATGCC |
pGGC_D6PK _for | AGAAGTGAAGCTTGGTCTCAGGCTCCATGATGGCTTCAAAAACTCC |
pGGC_D6PK_rev | AGGGCGAGAATTCGGTCTCACTGATCAGAAGAAATCAAACTCAAGATA |
pGGA_D6PKpromA_for | GTGAAGCTTGGTCTCAACCTCTGTTGAACCATTTCTAAAAAAAC |
pGGA_D6PKpromA_rev | GAGAGAGAGTCCCAATAAATCGTTACCTG |
pGGA_D6PKpromB_for | ATTTATTGGGACTCTCTCTCTCTCTCTCTC |
pGGA_D6PKpromB_rev | CGAGAATTCGGTCTCATGTTTAACACAGAGCAATCTTAAAC |
pGGA_backbone_for | AACATGAGACCGAATTCTC |
pGGA_backbone_rev | AGGTTGAGACCAAGCTTC |
Genotyping | |
ngr1-F199G07-GT-FP | GCGCAGACAAAAATCTTCTTG |
ngr1-F199G07-GT-RP | TTGGTGGACTCGTTTGCTTAC |
FLAG-LB4 | CGTGTGCCAGGTGCCCACGGAATAGT |
ngr2-SAIL723H11-GT-FP | TTTGGTTTTATGGACCCAACC |
ngr2-SAIL723H11-GT-RP | AAGAGCTTTCTTCCTCCGATG |
SAIL-LB3 | TAGCATCTGAATTTCATAACCAATCTCGATACAC |
ngr3-GK479C08-GT-FP | GCAAACAGATTTTCTTCACCAC |
ngr3-GK479C08-GT-RP | GCACAAGTGGCTTCAAAACTC |
GABI-O8409 | ATATTGACCATCATACTCATTGC |
gNGRmut-NGR1prom-fwd | GTAGTCAAAGTTTGGAACTTGAACACC |
gNGRmut-T35S-rev | CTGGGAACTACTCACACATTATTCTGG |
Mutagenesis | |
mutatePBR2-fwd | TAAGAATAACAAGGAAGAAAGAGACATAAGCAAGAACTCTG |
mutatePBR2-rev | TCTTTCTTCCTTGTTATTCTTACTCTCTATTGATATCTC |
PBR1&2-fragment1-bb-fwd | TAAGAATAACAAGGAAGAAAGAGACATAAGCAAGAACTCTG |
PBR1&2-fragment1-bb-rev | TCACATCAGACTTTTCTTCCTTAGTTCTTGACAAGAGCTTC |
PBR1&2-fragment2-insert-fwd | AAGGAAGAAAAGTCTGATGTGAATAGAGAGC |
PBR1&2-fragment2-insert-rev | TCTTTCTTCCTTGTTATTCTTACTCTCTATTGATATCTC |
mutatePALM_C206A-fwd | CAAGAAGATTTTTGTCGCTGCAGATGGG |
mutatePALM_C206A-rev | CAGCGACAAAAATCTTCTTGAAAAGATATGAGACAGAG |
add-Nmyr-fwd | CCACAAGTTCAGGGGGGATCATAACAGAACAAGCACTTCC |
add-Nmyr-rev | CCTGAACTTGTGGTGCATCCACCCCCAGAACTTCATGGAGCC |
ROP2-CT-oligo-bridge | GCTATAAAAGTGGTGCTTCAGCCACCAAAGCAGAAGAAGAAGAAAAAGAATAAGAACCGTTGCGCGTTCTTGTGA |
NGR-ROP2_CT-after-GFP-fwd | ACCGTTGCGCGTTCTTGTGACACCCAGCTTTCTTGTAC |
NGR-ROP2_CT-after-GFP-rev | TGAAGCACCACTTTTATAGCCTTGTACAGCTCGTCCATG |
All the transgenic lines generated in this study can be accessed by contacting the corresponding author.
Data availability
All data generated in this study are included as a manuscript supporting files.
References
-
Dynamic control of auxin transport-dependent growth by AGCVIII protein kinasesCurrent Opinion in Plant Biology 22:108–115.https://doi.org/10.1016/j.pbi.2014.09.010
-
A guided tour into subcellular colocalization analysis in light microscopyJournal of Microscopy 224:213–232.https://doi.org/10.1111/j.1365-2818.2006.01706.x
-
Signaling phospholipids in plant development: small couriers determining cell fateCurrent Opinion in Plant Biology 57:61–71.https://doi.org/10.1016/j.pbi.2020.05.007
-
Negative gravitropism in plant rootsNature Plants 2:16155.https://doi.org/10.1038/nplants.2016.155
-
Negative gravitropic response of roots directs auxin flow to control root gravitropismPlant, Cell & Environment 42:2372–2383.https://doi.org/10.1111/pce.13559
-
PIN-mediated polar auxin transport regulations in plant tropic responsesThe New Phytologist 232:510–522.https://doi.org/10.1111/nph.17617
-
Multiple roles for protein palmitoylation in plantsTrends in Plant Science 13:295–302.https://doi.org/10.1016/j.tplants.2008.04.006
-
LAZY gene family in plant gravitropismFrontiers in Plant Science 11:606241.https://doi.org/10.3389/fpls.2020.606241
-
Gravity sensing and responses in the coordination of the shoot gravitropic setpoint angleThe New Phytologist 236:1637–1654.https://doi.org/10.1111/nph.18474
-
Amyloplasts are necessary for full gravitropic sensitivity in roots of Arabidopsis thalianaPlanta 177:198–206.
-
Gravitropism in roots of intermediate-starch mutants of ArabidopsisPhysiologia Plantarum 97:237–244.https://doi.org/10.1034/j.1399-3054.1996.970205.x
-
Reduced gravitropism in hypocotyls of starch-deficient mutants of ArabidopsisPlant & Cell Physiology 38:518–525.https://doi.org/10.1093/oxfordjournals.pcp.a029199
-
Directional gravity sensing in gravitropismAnnual Review of Plant Biology 61:705–720.https://doi.org/10.1146/annurev.arplant.043008.092042
-
RIN4 recruits the exocyst subunit EXO70B1 to the plasma membraneJournal of Experimental Botany 68:3253–3265.https://doi.org/10.1093/jxb/erx007
-
Plant gravity sensingInternational Review of Cytology 127:193–252.https://doi.org/10.1016/s0074-7696(08)60695-6
-
Fiji: an open-source platform for biological-image analysisNature Methods 9:676–682.https://doi.org/10.1038/nmeth.2019
-
Switching the Direction of Stem Gravitropism by Altering Two Amino Acids in AtLAZY1Plant Physiology 182:1039–1051.https://doi.org/10.1104/pp.19.01144
-
A Cre-dependent GCaMP3 reporter mouse for neuronal imaging in vivoThe Journal of Neuroscience 32:3131–3141.https://doi.org/10.1523/JNEUROSCI.4469-11.2012
Article and author information
Author details
Funding
Horizon 2020 Framework Programme (No 742985)
- Jiří Friml
FEMtech Internships for Female Students (Federal Ministry Republic of Austria for Climate Action, Environment, Energy, Mobility, Innovation and Technology)
- Julia Schmid
Austrian Science Fund (I3630-775 B25)
- Jiří Friml
FemTECH fellowship
- Julia Schmid
The funders had no role in study design, data collection and interpretation, or the decision to submit the work for publication.
Acknowledgements
The research leading to these results has received funding from the European Research Council (ERC) under the European Union’s Horizon 2020 research and innovation programme grant agreement No 742985 and Austrian Science Fund (FWF): I3630-775 B25 to J.F. This research was also supported by the Lab Support Facility (LSF) and the Imaging and Optics Facility (IOF) of IST Austria, namely Tereza Bělinová for her help with the imaging. JS was supported by FemTECH fellowship.
Version history
- Sent for peer review: August 15, 2023
- Preprint posted: September 1, 2023 (view preprint)
- Preprint posted: November 23, 2023 (view preprint)
- Preprint posted: February 22, 2024 (view preprint)
- Version of Record published: March 5, 2024 (version 1)
Cite all versions
You can cite all versions using the DOI https://doi.org/10.7554/eLife.91523. This DOI represents all versions, and will always resolve to the latest one.
Copyright
© 2023, Kulich, Schmid, Teplova et al.
This article is distributed under the terms of the Creative Commons Attribution License, which permits unrestricted use and redistribution provided that the original author and source are credited.
Metrics
-
- 963
- views
-
- 121
- downloads
-
- 0
- citations
Views, downloads and citations are aggregated across all versions of this paper published by eLife.
Download links
Downloads (link to download the article as PDF)
Open citations (links to open the citations from this article in various online reference manager services)
Cite this article (links to download the citations from this article in formats compatible with various reference manager tools)
Further reading
-
- Plant Biology
Plant pathogens secrete proteins, known as effectors, that function in the apoplast or inside plant cells to promote virulence. Effector recognition by cell-surface or cytosolic receptors results in the activation of defence pathways and plant immunity. Despite their importance, our general understanding of fungal effector function and recognition by immunity receptors remains poor. One complication often associated with effectors is their high sequence diversity and lack of identifiable sequence motifs precluding prediction of structure or function. In recent years, several studies have demonstrated that fungal effectors can be grouped into structural classes, despite significant sequence variation and existence across taxonomic groups. Using protein X-ray crystallography, we identify a new structural class of effectors hidden within the secreted in xylem (SIX) effectors from Fusarium oxysporum f. sp. lycopersici (Fol). The recognised effectors Avr1 (SIX4) and Avr3 (SIX1) represent the founding members of the Fol dual-domain (FOLD) effector class, with members containing two distinct domains. Using AlphaFold2, we predicted the full SIX effector repertoire of Fol and show that SIX6 and SIX13 are also FOLD effectors, which we validated experimentally for SIX6. Based on structural prediction and comparisons, we show that FOLD effectors are present within three divisions of fungi and are expanded in pathogens and symbionts. Further structural comparisons demonstrate that Fol secretes effectors that adopt a limited number of structural folds during infection of tomato. This analysis also revealed a structural relationship between transcriptionally co-regulated effector pairs. We make use of the Avr1 structure to understand its recognition by the I receptor, which leads to disease resistance in tomato. This study represents an important advance in our understanding of Fol-tomato, and by extension plant–fungal interactions, which will assist in the development of novel control and engineering strategies to combat plant pathogens.
-
- Ecology
- Plant Biology
Volatiles emitted by herbivore-attacked plants (senders) can enhance defenses in neighboring plants (receivers), however, the temporal dynamics of this phenomenon remain poorly studied. Using a custom-built, high-throughput proton transfer reaction time-of-flight mass spectrometry (PTR-ToF-MS) system, we explored temporal patterns of volatile transfer and responses between herbivore-attacked and undamaged maize plants. We found that continuous exposure to natural blends of herbivore-induced volatiles results in clocked temporal response patterns in neighboring plants, characterized by an induced terpene burst at the onset of the second day of exposure. This delayed burst is not explained by terpene accumulation during the night, but coincides with delayed jasmonate accumulation in receiver plants. The delayed burst occurs independent of day:night light transitions and cannot be fully explained by sender volatile dynamics. Instead, it is the result of a stress memory from volatile exposure during the first day and secondary exposure to bioactive volatiles on the second day. Our study reveals that prolonged exposure to natural blends of stress-induced volatiles results in a response that integrates priming and direct induction into a distinct and predictable temporal response pattern. This provides an answer to the long-standing question of whether stress volatiles predominantly induce or prime plant defenses in neighboring plants, by revealing that they can do both in sequence.