Rapid binding to protofilament edge sites facilitates tip tracking of EB1 at growing microtubule plus-ends
Figures
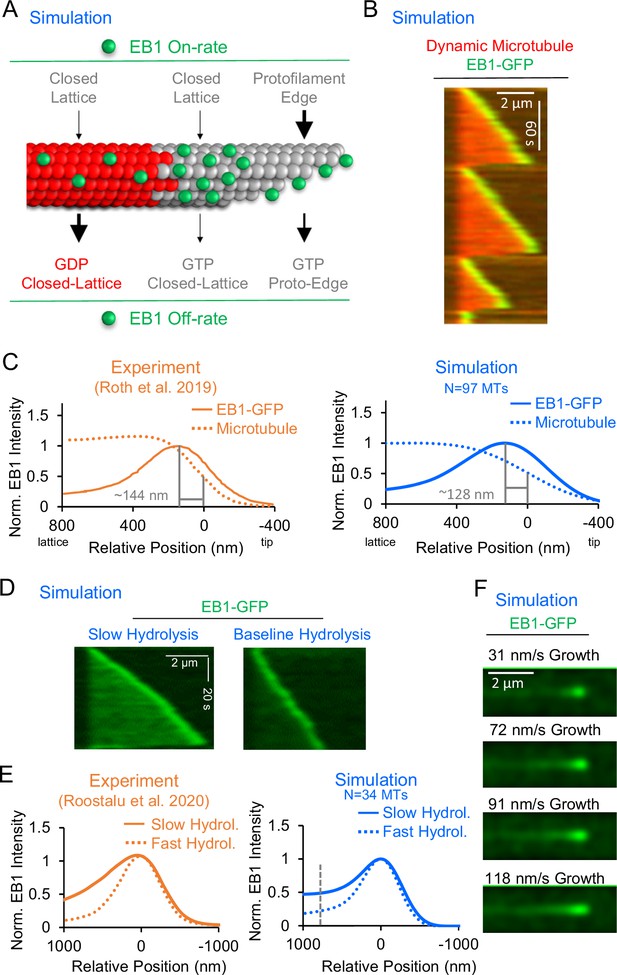
Development and validation of a stochastic simulation for EB1 tip tracking.
(A) Rules for a molecular-scale stochastic simulation that incorporates both tubulin subunit assembly and EB1 arrivals to and departures from the growing microtubule (See Methods). In the simulation, the EB1 protofilament-edge on-rate (top-right) is 50–100 fold higher than the EB1 closed-lattice on-rate (top-left and top-center). The EB1 off-rate is 6–12 fold faster for closed-lattice GDP-tubulin binding pockets (bottom-left) than for closed-lattice GTP-tubulin binding pockets (bottom-center). (B) Simulated EB1 tip tracking at growing microtubule ends. (C) Left: Line scans of EB1-GFP intensity (solid line), and microtubule intensity (dotted line) from experimentally reported data (Roth et al., 2019) (orange). Right: Line scans of EB1-GFP intensity (solid line), and microtubule intensity (dotted line) from the simulation (blue, see Methods). (D) Left: Simulated EB1 tip tracking with a slow GTP-tubulin hydrolysis rate (0.05 s–1) Right: Simulated EB1 tip tracking with the baseline GTP-tubulin hydrolysis rate (0.55 s–1). (E) Left: Experimental line scan quantification of EB3-GFP intensity along the length of the microtubule with two different hydrolysis rates (orange, Roostalu et al., 2020). Right: Simulated data line scan quantification of EB1-GFP intensity along the length of the microtubule with two different hydrolysis rates (blue). Slower hydrolysis leads to a ~ twofold increase in binding along the lattice at 768 nm distal of the peak EB1 position (dashed line). (F) Increasing the microtubule growth rate in the simulation increases the EB1 comet length, similar to reports in the literature (Bieling et al., 2007).
-
Figure 1—source data 1
Line scan data for EB1 intensity for Figure 1C, E (right).
- https://cdn.elifesciences.org/articles/91719/elife-91719-fig1-data1-v1.xlsx
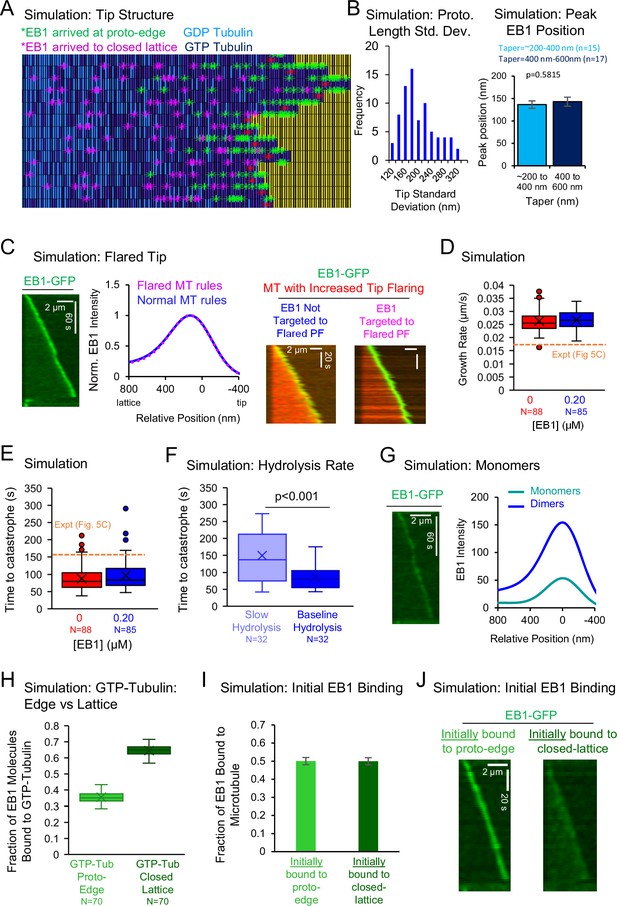
Additional simulation results.
(A) Frame from animated simulated results in Video 2, with microtubule plus-end towards the right. Tubulin dimer hydrolysis state is indicated by color (GDP: light blue, GTP: dark blue). Green asterisks: EB1 molecules that originally bound to a protofilament-edge site. Purple asterisks: EB1 molecules that originally bound to a closed-lattice site. (B) Left: Tip standard deviation of simulated microtubules (Coombes et al., 2013; Demchouk et al., 2011). Simulated microtubules had a tip standard deviation of 191 ± 6 nm (Mean ± SEM). Right: Average EB1 peak position relative to microtubule tip, for averages of three simulation runs binned by microtubule taper size. (error bars, SEM; p=0.58, Mann Whitney U-test) (C) Left: A microtubule tip flaring approximation did not qualitatively alter EB1 tip tracking in the simulation. Center: The linescan of EB1 from the simulation was unaffected by whether the microtubule had tip flaring in the simulation. Right: Increased microtubule tip flaring was introduced into the simulation by reducing the lateral bond creation rate, while also increasing the tubulin on-rate to protofilaments that did not have lateral bonds with their neighbors. Blue: Simulation with increased protofilament flaring at the tip, but without EB1 targeting to flared protofilament edges. Magenta: Simulation with increased protofilament flaring at tip, and with EB1 targeting to flared protofilament edges. (D) Growth rates in the simulation were similar to experiments from Figure 5C (orange dashed line). (E) Time to catastrophe in the simulation was similar to experiments from Figure 5C (orange dashed line). (F) Decreasing the hydrolysis rate in the simulation increased the time to catastrophe (p<0.001, t-test). (G) To replicate EB1 monomer tip tracking, all off-rates were increased by fourfold (Song et al., 2020). Left: Qualitatively, higher off-rates led to a reduction in EB1 at the microtubule tip. Right: EB1 monomers (teal) in the simulation had a ~threefold reduction in the amount of EB1 at the growing microtubule end as dimers (blue). (H) Fraction of EB1 bound at GTP closed-lattice sites relative to GTP protofilament-edge sites. (I) Fraction of EB1 bound to the microtubule for EB1 molecules that initially bound to protofilament-edge sites (light green), and for EB1 molecules that initially bound to closed-lattice sites (dark green) (error bars, SEM). (J) Localization of EB1-GFP that bound originally to protofilament-edge sites (left) or to closed-lattice sites (right).
-
Figure 1—figure supplement 1—source data 1
Data for panels in Figure 1—figure supplement 1.
- https://cdn.elifesciences.org/articles/91719/elife-91719-fig1-figsupp1-data1-v1.xlsx
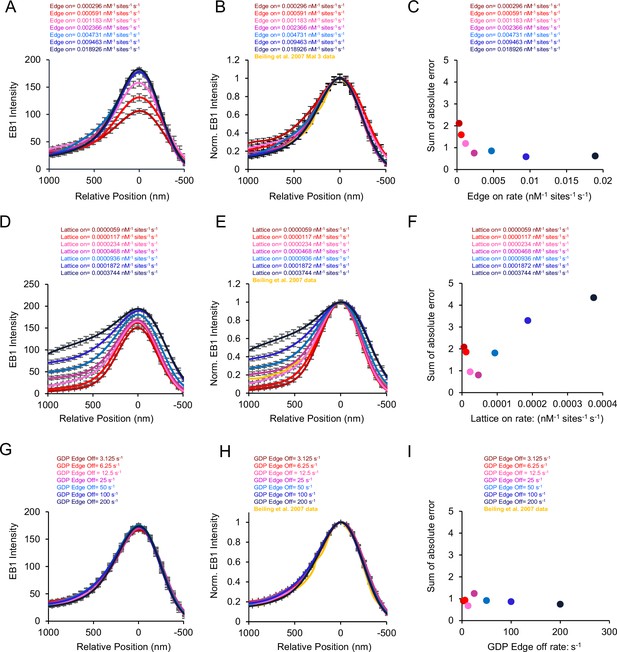
Parameter sensitivity testing for the EB1 tip tracking model I.
(A) Line scans of simulated EB1 tip tracking with varying protofilament-edge on-rates. (B) Normalized line scans of simulated EB1 tip tracking with varying protofilament-edge on-rates, as compared to literature data (yellow) (Bieling et al., 2007). (C) Sum of absolute error between the simulation and the literature data for each protofilament-edge on-rate. (D) Line scans of simulated EB1 tip tracking with varying closed-lattice on-rates. (E) Normalized line scans of simulated EB1 tip tracking with varying closed-lattice on-rates, as compared to literature data (yellow) (Bieling et al., 2007). (F) Sum of absolute error between the simulation and the literature data for each closed-lattice on-rate. (G) Line scans of simulated EB1 tip tracking with varying GDP protofilament-edge off-rates. (H) Normalized line scans of simulated EB1 tip tracking with varying GDP protofilament-edge off-rates, as compared to literature data (yellow) (Bieling et al., 2007). (I) Sum of absolute error between the simulation and the literature data for each GDP protofilament-edge off-rate.
-
Figure 1—figure supplement 2—source data 1
Data for panels in Figure 1—figure supplement 2.
- https://cdn.elifesciences.org/articles/91719/elife-91719-fig1-figsupp2-data1-v1.xlsx
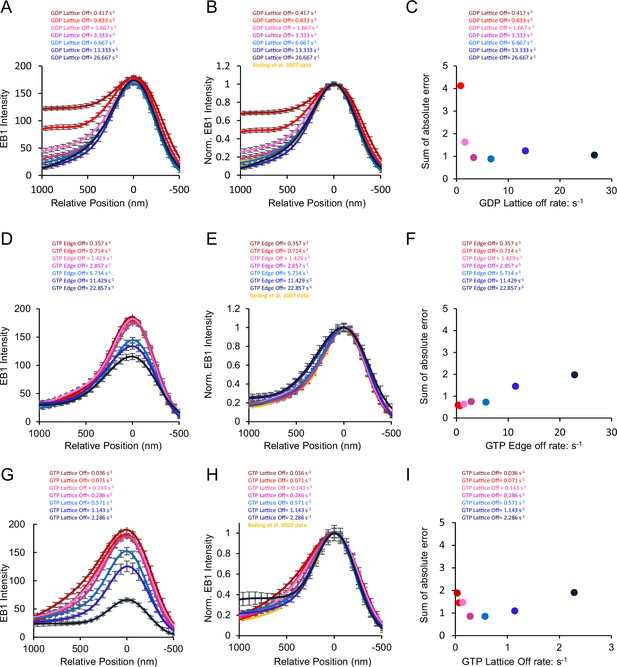
Parameter sensitivity testing for the EB1 tip tracking model II.
(A) Line scans of simulated EB1 tip tracking with varying GDP closed-lattice off-rates. (B) Normalized line scans of simulated EB1 tip tracking with varying GDP closed-lattice off-rates, as compared to literature data (yellow) (Bieling et al., 2007). (C) Sum of absolute error between the simulation and the literature data for each GDP closed-lattice off-rate. (D) Line scans of simulated EB1 tip tracking with varying GTP protofilament-edge off-rates. (E) Normalized line scans of simulated EB1 tip tracking with varying GTP protofilament-edge off-rates, as compared to literature data (yellow) (Bieling et al., 2007). (F) Sum of absolute error between the simulation and the literature data for each GTP protofilament-edge off-rate. (G) Line scans of simulated EB1 tip tracking with varying GTP closed-lattice off-rates. (H) Normalized line scans of simulated EB1 tip tracking with varying GTP closed-lattice off-rates, as compared to literature data (yellow) (Bieling et al., 2007). (I) Sum of absolute error between the simulation and the literature data for each GTP closed-lattice off-rate.
-
Figure 1—figure supplement 3—source data 1
Data for Figure 1—figure supplement 3.
- https://cdn.elifesciences.org/articles/91719/elife-91719-fig1-figsupp3-data1-v1.xlsx
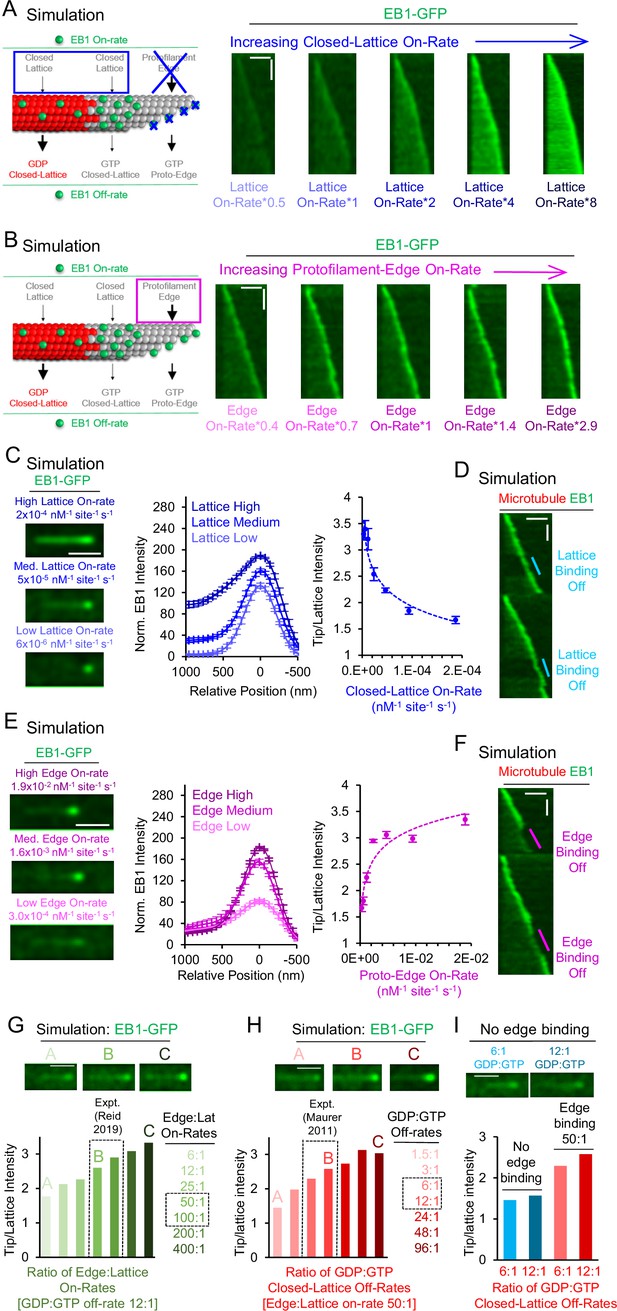
Simulations predict that protofilament-edge binding facilitates robust EB1 tip tracking.
(A) Left: Simulations were performed in which the EB1 protofilament-edge on-rate was set to zero, and the closed-lattice on-rate was gradually increased. Right: Simulated kymographs in which the EB1 protofilament-edge on-rate was set to zero, and the on-rate at closed-lattice sites was gradually increased (scale bars: 2 µm and 10 s). (B) Left: Simulations were performed in which the closed-lattice on-rate remained constant at its baseline (non-zero) value, and the protofilament-edge on-rate was gradually increased. Right: Simulated kymographs in which the closed-lattice on-rate remained constant at its baseline (non-zero) value, and the protofilament-edge on-rate was gradually increased (scale bars: 2 µm and 10 s). (C) Left: Simulated images of EB1-GFP tip tracking over a range of closed-lattice on-rates (scale bar: 1 µm). Center: Line scans from simulated images of EB1-GFP intensity for a range of closed-lattice on-rates (error bars, SEM). Right: Tip:Lattice EB1-GFP intensity ratio vs closed-lattice on-rates in the simulation (error bars, SEM). The tip:Lattice EB1-GFP intensity ratio decreases with increasing closed-lattice on-rates. (D) Simulated kymograph in which the closed-lattice on-rate is set to zero partway through the simulation, and later returned to its baseline value (scale bars: 2 μm and 20 s). (E) Left: Simulated images of EB1-GFP tip tracking over a range of protofilament-edge on-rates (scale bar: 1 µm). Center: Line scans from simulated images of EB1-GFP intensity for a range of protofilament-edge on-rates (error bars, SEM). Right: Tip:Lattice EB1-GFP intensity ratio vs protofilament-edge on-rates in the simulation (error bars, SEM). Localization to the microtubule tip increases with increasing protofilament-edge on-rates. (F) Simulated kymograph in which the protofilament-edge on-rate is set to zero partway through the simulation and later returned to its baseline value (scale bars: 2 μm and 20 s). (G) Top: Representative images of EB1-GFP tip tracking for increasing Protofilament-edge:Closed-lattice on-rate ratios. Bottom: Tip:Lattice EB1-GFP intensity ratio for increasing Protofilament-edge:Closed-lattice on-rate ratios (GDP:GTP off-rate ratio is constant and set to 12:1). The experimentally measured Protofilament-edge:Closed-lattice on-rate ratio is 50–100 (Reid et al., 2019) (gray dashed boxes). (H) Top: Representative images of EB1-GFP tip tracking for increasing GDP:GTP closed-lattice off-rate ratios. Bottom: Tip:Lattice EB1-GFP intensity ratio for increasing GDP:GTP closed-lattice off-rate ratios (Protofilament-edge:Closed-lattice on-rate ratio is constant and set to 50:1). The experimentally measured GDP:GTP closed-lattice off-rate ratio is 6–12 (Maurer et al., 2011) (gray dashed boxes). (I) Top: Representative images of EB1-GFP tip tracking without protofilament-edge binding. Bottom: Tip:Lattice EB1-GFP intensity ratio for experimentally measured GDP:GTP closed-lattice off-rate ratios, with (red) or without (blue) EB1 binding at protofilament-edge sites.
-
Figure 2—source data 1
Data for Figure 2C, E, G, H, and I.
- https://cdn.elifesciences.org/articles/91719/elife-91719-fig2-data1-v1.xlsx
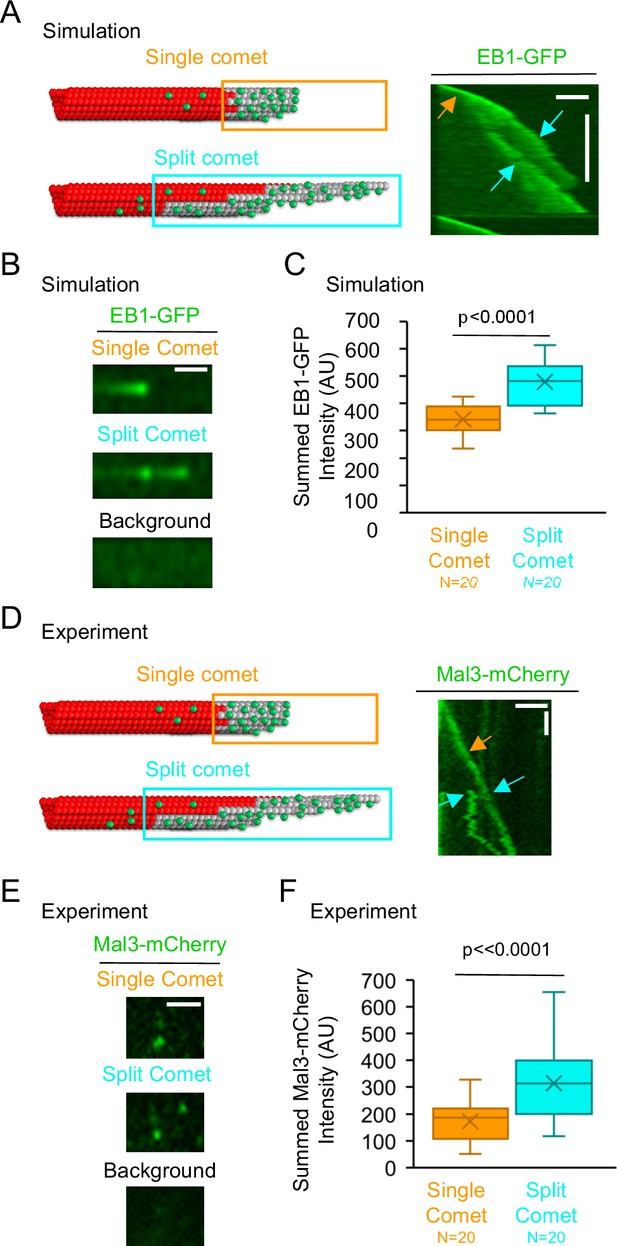
Summed Mal3 comet intensity is increased in split comets relative to single comets.
(A) Left: Schematic of a single comet (top), and a split comet (bottom). Right: Simulated kymograph with a single comet (orange arrow), and split comet (cyan arrows), within one common microtubule growth event (scale bars: 2 μm and 60 s). (B) The summed comet intensity can be measured for a single simulated comet (top) and, later on, the same simulated microtubule growth event, for a split simulated comet (middle). The background (bottom) was subtracted from both the single comet and split comet summed intensity measurements. (C) Simulated split comets have a higher summed EB1 intensity at the microtubule tip than their single comet counterparts on the same growth event (p<0.0001, paired t-test,). (D) Left: Schematic of a single comet (top), and a split comet (bottom). Right: Typical experimental kymograph with a single comet (orange arrow), and split comet (cyan arrows), within one common microtubule growth event (scale bars: 2 μm and 60 s). (E) The summed comet intensity can be measured for a single comet (top) and, later on, the same microtubule growth event, for a split comet (middle). The background (bottom) was subtracted from both the single comet and split comet summed intensity measurements. (F) Split comets had a higher summed Mal3-mCherry intensity at the microtubule tip than their single comet counterparts from the same growth event (p<0.0001, paired t-test).
-
Figure 3—source data 1
Data for Figure 3C, F.
- https://cdn.elifesciences.org/articles/91719/elife-91719-fig3-data1-v1.xlsx
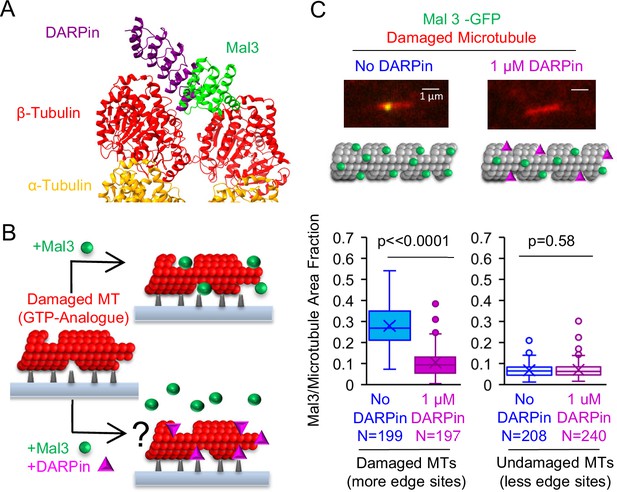
Synthetic Designed Ankyrin Repeat Protein (DARPin) peptide blocks EB binding to protofilament-edge sites.
(A) Structure of DARPin and Mal3, together with α and β tubulin at a microtubule tip was created in ChimeraX (Pettersen et al., 2021) using the crystal structures 4drx (DARPin) and 4abo (Mal3). (B) Left: To test whether DARPin blocks EB binding to protofilament-edge sites, we first generated stabilized GTP-analogue (GMPCPP) microtubules that were damaged with CaCl2 treatment, thus creating protofilament-edge sites along the length of the microtubule. Right: The damaged microtubules were incubated with Mal3-GFP in the presence (bottom) or absence (top) of 1 μM DARPin. Suppression of Mal3-GFP binding to the damaged microtubule in the presence of DARPin suggests that DARPin blocks Mal3 from binding to protofilament-edge sites (right, bottom). (C) Top: Representative images of Mal3-GFP binding to damaged microtubules, in the absence (blue) or presence (magenta) of 1 μM DARPin. Middle: Cartoons depicting the relative binding of Mal3 and DARPin in each experiment. Bottom-Left: Quantification of the fraction of microtubule area bound by Mal3-GFP for damaged microtubules in the absence or presence of DARPin (p<0.0001, t-test; sample size indicates number of images). Bottom-Right: Quantification of the fraction of microtubule area bound by Mal3-GFP for undamaged microtubules in the absence or presence of DARPin (p=0.58, t-test; sample size indicates number of images).
-
Figure 4—source data 1
Data for Figure 4C.
- https://cdn.elifesciences.org/articles/91719/elife-91719-fig4-data1-v1.xlsx
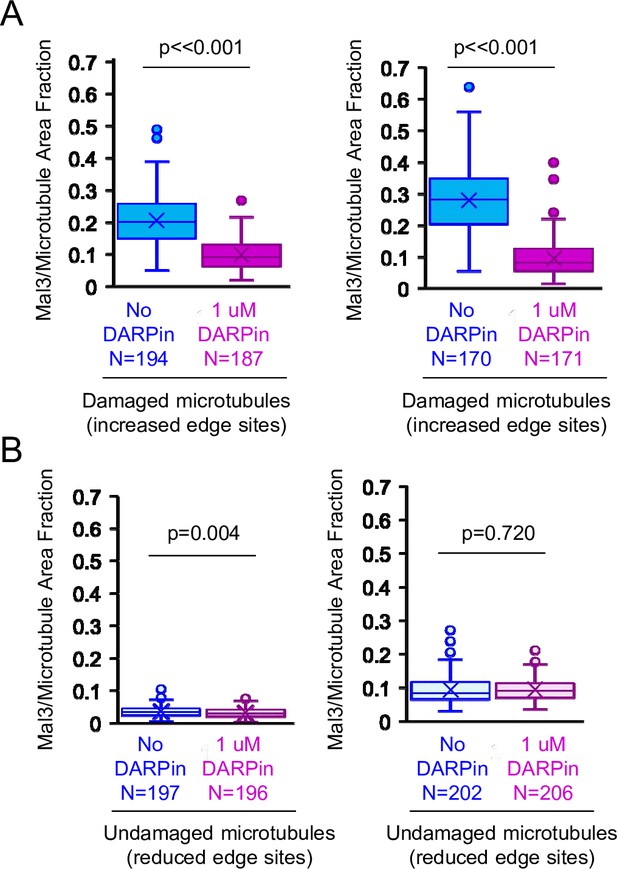
Additional experimental data for binding of Mal3-GFP to stabilized GMPCPP microtubules.
(A) Quantification of the fraction of microtubule area bound by Mal3-GFP for damaged microtubules in the absence or presence of 1 μM DARPin (second and third replicates; t-tests). (B) Quantification of the fraction of microtubule area bound by Mal3-GFP for undamaged microtubules in the absence or presence of 1 μM Designed Ankyrin Repeat Protein (DARPin) (second and third replicates; t-tests).
-
Figure 4—figure supplement 1—source data 1
Data for Figure 4—figure supplement 1.
- https://cdn.elifesciences.org/articles/91719/elife-91719-fig4-figsupp1-data1-v1.xlsx
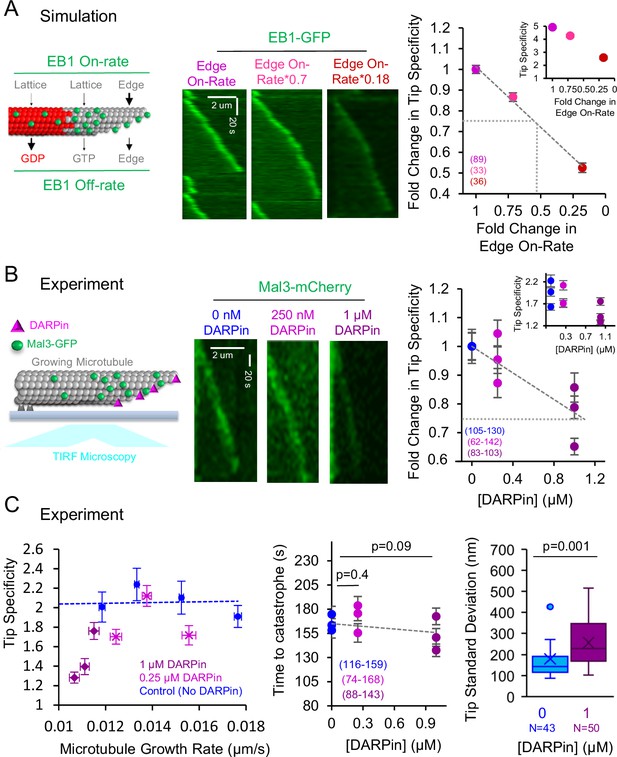
In cell-free experiments, Designed Ankyrin Repeat Protein (DARPin) disrupts tip tracking by blocking EB1 access to protofilament-edge sites.
(A) Left: Cartoon depicting simulation rules for EB1 binding and unbinding to growing microtubules. Center: Simulated kymographs of EB1-GFP at growing microtubule tips, with decreasing protofilament-edge on-rates. Right: Simulation prediction for the fold change in the EB1 Tip Specificity as a function of the fold-change in the protofilament-edge on-rate (error bars: SEM; p<0.001, Kruskall Wallis). Gray dotted lines correspond to a twofold decrease in protofilament-edge on-rate, which leads to a ~25% decrease in predicted Tip Specificity. Inset: Absolute EB1 tip Specificity as a function of the fold-change in the protofilament-edge on-rate. (B) Left: Cartoon depicting experimental setup for dynamic microtubules with Mal3-mCherry, in the presence of DARPin, and visualized using TIRF microscopy. Center: Experimental kymographs of Mal3-mCherry tip tracking along dynamic microtubules in the presence of increasing DARPin concentrations. Right: Experimental results for the fold change in the Mal3-GFP Tip Specificity as a function of DARPin concentration (error bars: SEM; p<0.001, Kruskall Wallis). Gray dotted line represents a~25% decrease in Mal3-GFP Tip Specificity, which corresponds to a simulation prediction of a ~two-fold decrease in protofilament-edge on-rate. Inset: Absolute Mal3-GFP Tip Specificity as a function of DARPin concentration. (C) Left: The decrease in Mal3-GFP Tip Specificity in DARPin is not due to a decrease in microtubule growth rate (p<0.001, Kruskall Wallis comparing DARPin data to control data at a ~0.012 μm/s growth rate,). Center: The time to catastrophe is not altered by DARPin, suggesting that the GTP-cap size remains similar in the presence and absence of DARPin (Tukey’s post-hoc analysis after an ANOVA). Right: Tip standard deviation in the presence and absence of DARPin (p=0.001, t-test,).
-
Figure 5—source data 1
Data for Figure 5.
- https://cdn.elifesciences.org/articles/91719/elife-91719-fig5-data1-v1.xlsx
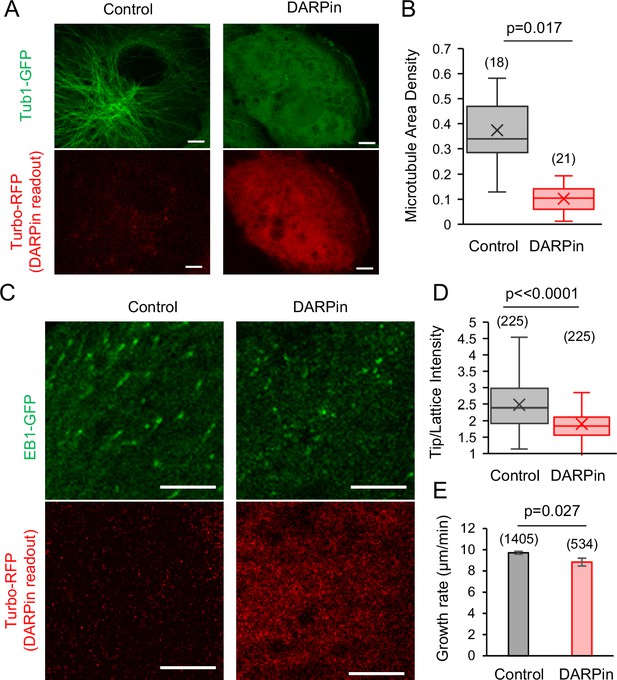
EB1 tip tracking is suppressed in cells that expressed Designed Ankyrin Repeat Protein (DARPin).
(A) Left: Tubulin-GFP (top) and Turbo-RFP expression (bottom) in LLC-Pk1 cells that were not transiently transfected (scale bar: 5 μm). Right: Tubulin-GFP (top) and Turbo-RFP expression (bottom) in LLC-Pk1 cells that were transiently transfected (scale bar: 5 μm). (B) Microtubule density is lower in cells transfected with DARPin (p=0.017, Mann-Whitney U Test). (C) Left: EB1-GFP (top) and Turbo-RFP expression (bottom) in LLC-Pk1 cells that were not transiently transfected (scale bar: 5 μm). Right: EB1-GFP (top) and Turbo-RFP expression (bottom) in LLC-Pk1 cells that were transiently transfected (scale bar: 5 μm). (D) EB1-GFP Tip:Lattice intensity ratio is lower in cells transfected with DARPin as compared to control cells (p<0.0001, t-test). (E) Microtubule growth rate is similar in cells transfected with DARPin as compared to control cells (p=0.027, t-test).
-
Figure 6—source data 1
Data for Figure 6B, D, and E.
- https://cdn.elifesciences.org/articles/91719/elife-91719-fig6-data1-v1.xlsx
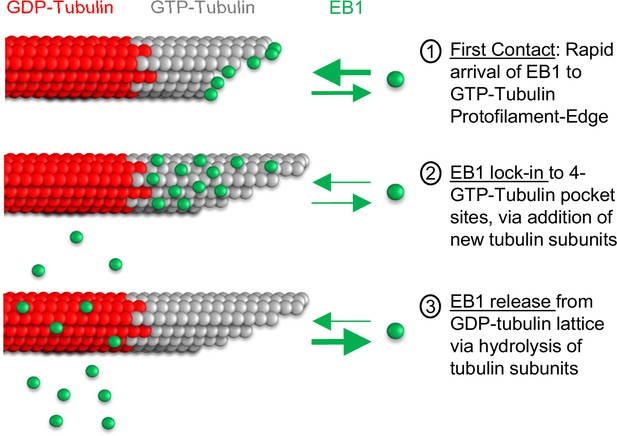
Model for EB1 tip tracking.
Step 1 (top): EB1 binds rapidly to protofilament-edge sites, with a slower on-rate to 4-tubulin closed lattice binding sites. Step 2 (middle): Incorporation of new tubulin subunits ‘locks in’ EB1 bound at protofilament-edge sites as the binding pocket transitions from a protofilament-edge site to a 4-tubulin closed-lattice site. Step 3 (bottom): As the GTP-tubulin hydrolyzes to GDP-tubulin, the EB1 dissociates from the GDP closed-lattice binding site.
Videos
Simulated EB1 tip tracking.
EB1-GFP in green, Microtubule in red. 2 µm scale bar.
Animated simulation output for a growth event of one microtubule.
Green asterisks: EB1 that originally bound to a protofilament-edge site. Purple asterisks: EB1 that originally bound to a closed-lattice site. Red crosses: location of most distal lateral bond for each protofilament. Protofilament #13 shares a lateral bond with protofilament #1. Dark blue rectangles: GTP-tubulin. Light blue rectangles: GDP-tubulin. A seed of 25 dimers was maintained as GTP-tubulin to represent a GMPCPP seed. The Video is updated every 1000 steps within the simulation.
Simulated EB1 tip tracking for different hydrolysis rates.
EB1-GFP in green, microtubule in red. 2 µm scale bar. Slower hydrolysis rate on left (0.05 s–1), baseline hydrolysis rate on right (0.55 s–1).
Simulated EB1 tip tracking over a range of EB1 closed-lattice on rates (1.2 x 10–5 – 1.9 x 10–4 nM–1 sites –1 s–1).
EB1 protofilament-edge binding on-rate was set to 0. Then, the remaining EB1 on and off rates were kept constant except for the closed-lattice on-rate, which increases from left to right for each subset of the panel. EB1-GFP in green, microtubule in red. 2 µm scale bars.
Simulated EB1 tip tracking over a range of protofilament-edge on-rates (5.9 x 10–4 – 4.7 x 10–3 nM–1 sites–1 s–1).
All EB1 on-and off-rates were kept constant except for the protofilament-edge on-rate, which increases from left to right for each subset of the panel. EB1-GFP in green, microtubule in red. 2 µm scale bars.
Simulated EB1 tip tracking, with EB1 closed-lattice binding dynamically set to 0 during the run.
Simulation is run with all baseline parameter values (Supplementary file 2). At times 57 s and 209 s, EB1 closed-lattice on-rate is set to zero. Then, at times 87 s and 239 s, the EB1 closed-lattice on-rate is reset to its baseline value. EB1-GFP in green, microtubule in red. 2 µm scale bar.
Simulated EB1 tip tracking, with EB1 protofilament-edge binding dynamically set to 0 during the run.
Simulation is run with all baseline parameter values (Supplementary file 2). At times 56 s and 203 s, EB1 protofilament-edge on-rate is set to zero. Then, at times 85 s and 233 s, the EB1 protofilament-edge on-rate is reset to its baseline value. EB1-GFP in green, microtubule in red. 2 µm scale bar.
Mal3 and Designed Ankyrin Repeat Protein (DARPin) overlap along an exposed tubulin plus-end.
Created in ChimeraX using the structures 4DRX (DARPin) and 4ABO (Mal3).
Experimental EB1-GFP tip tracking in live LLC-PK1 cells without Designed Ankyrin Repeat Protein (DARPin) transfection.
5 µm scale bar.
Experimental EB1-GFP tip tracking in live LLC-PK1 cells with Designed Ankyrin Repeat Protein (DARPin) transfection.
5 µm scale bar.
Additional files
-
Supplementary file 1
Summary table with simulation parameters for the microtubule assembly portion of the simulation.
Parameters determine the on and off rates of tubulin subunits from the microtubule tip, as well as parameters that control the hydrolysis rate of GTP-tubulin subunits within the lattice.
- https://cdn.elifesciences.org/articles/91719/elife-91719-supp1-v1.docx
-
Supplementary file 2
Summary table with simulation parameters for single-molecule EB1 dynamics.
Parameters determine the on and off rates of EB1 molecules from the microtubule tip and lattice.
- https://cdn.elifesciences.org/articles/91719/elife-91719-supp2-v1.docx
-
Supplementary file 3
Summary table with model parameter comparisons between the EB1 on and off rates from the microtubule tip and lattice for the current study model, as compared to a model developed by Maurer et al., 2014.
In addition, tubulin ‘maturation rates’ are compared, which define the EB1 binding zones in the Maurer et al model.
- https://cdn.elifesciences.org/articles/91719/elife-91719-supp3-v1.docx
-
MDAR checklist
- https://cdn.elifesciences.org/articles/91719/elife-91719-mdarchecklist1-v1.pdf