Autophagosome development and chloroplast segmentation occur synchronously for piecemeal degradation of chloroplasts
Figures
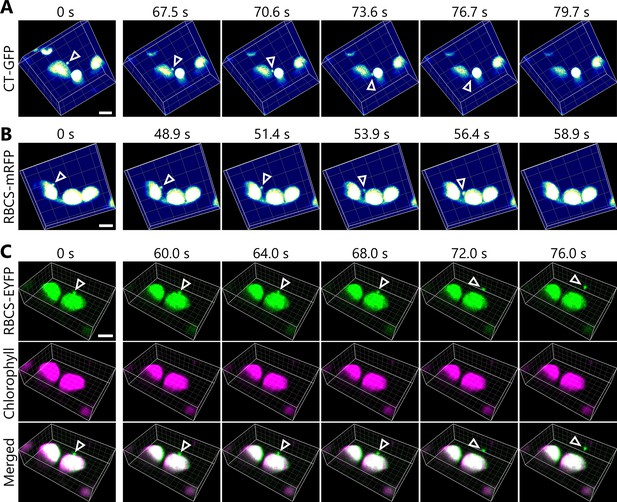
Chloroplast buds are released in sugar-starved leaves.
Time-lapse observations of three-dimensional (3D) reconstructed chloroplast morphology in Arabidopsis mesophyll cells accumulating chloroplast stroma–targeted fluorescent markers. A leaf from a plant accumulating chloroplast stroma–targeted GFP (CT-GFP) (A), RBCS-mRFP (B), or RBCS-EYFP (C) was incubated in sugar-free solution in darkness for 5 hr from dawn (A), 21 hr (B), or 24 hr from the period in light (C) and then observed through a two-photon excitation microscope equipped with a confocal spinning-disk unit. Second rosette leaves from 20- to 22-day-old plants were used. Images in (A–C) are still frames from Videos 1–3, respectively. Time scales above the images indicate the elapsed time from the start of the respective videos. Arrowheads indicate chloroplast budding structures. Scale bars, 5 µm. In (C), green, RBCS-EYFP; magenta, chlorophyll fluorescence. In the merged images, the overlapping regions of RBCS-EYFP and chlorophyll signals appear white.
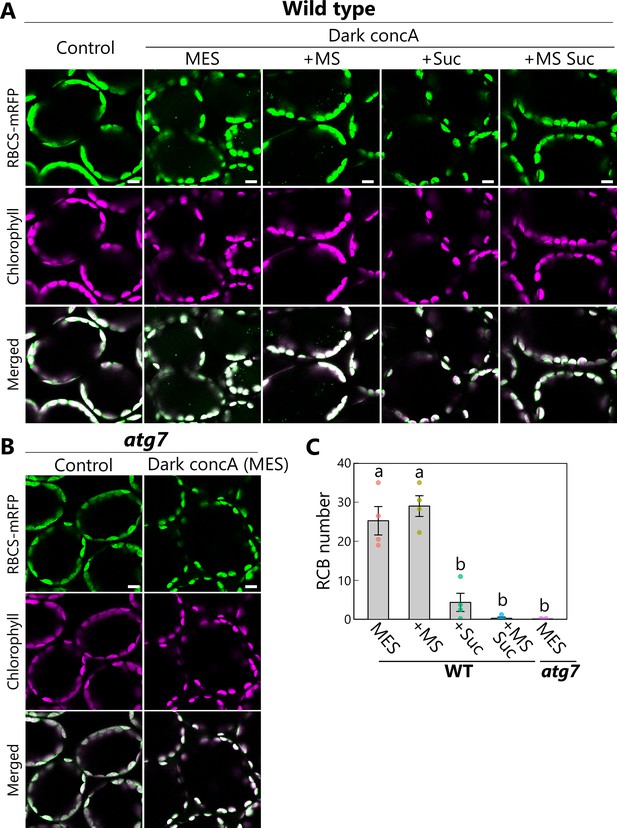
Accumulation of chloroplast stroma components in the vacuole via autophagy.
Confocal images of mesophyll cells from wild-type (A) or atg7 (B) plants accumulating the chloroplast stroma marker RBCS-mRFP. Second rosette leaves of 21-day-old plants were excised and incubated in 10 mM MES-NaOH containing 1 µM concanamycin A (concA) in darkness for 1 day. Sucrose (Suc) or Murashige and Skoog (MS) salts were added as an energy or nutrient source, respectively. Second rosette leaves from nontreated plants are shown as control. Green, RBCS-mRFP; magenta, chlorophyll fluorescence. Scale bars, 10 µm. The small puncta containing RBCS-mRFP without chlorophyll signal appear green and are Rubisco-containing bodies (RCBs) in the vacuole. (C) Number of accumulated RCBs from the observations described in (A) and (B). Different lowercase letters denote significant differences based on Tukey’s test (p < 0.05). Values are means ± SE (n = 4). Dots represent individual data points.
-
Figure 1—figure supplement 1—source data 1
Source data for the graph in Figure 1—figure supplement 1.
- https://cdn.elifesciences.org/articles/93232/elife-93232-fig1-figsupp1-data1-v1.zip
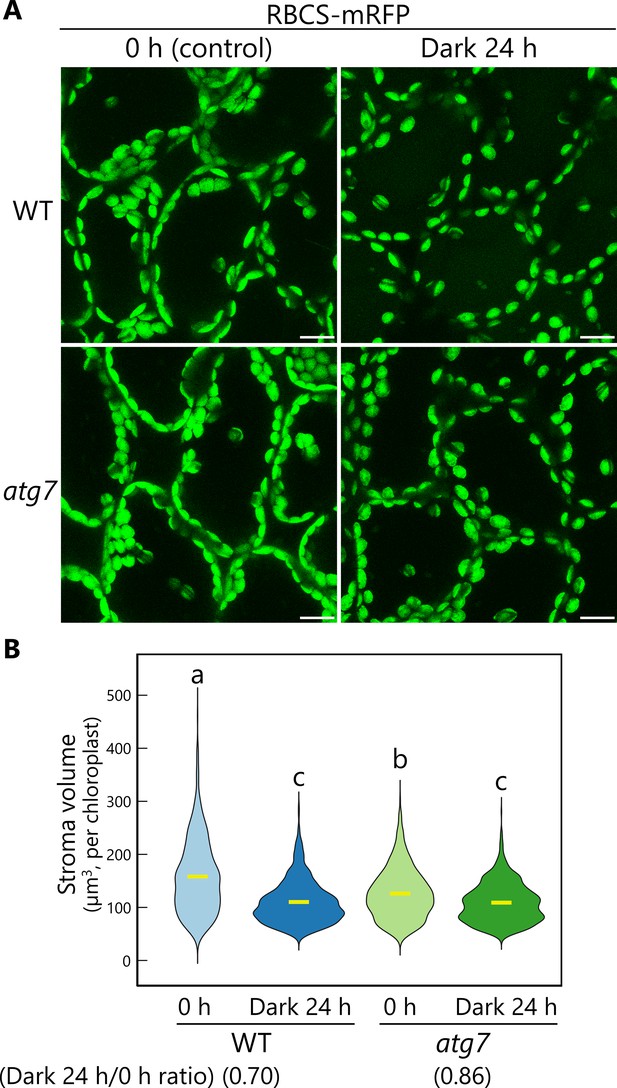
The release of chloroplast buds by autophagy contributes to the decline in chloroplast stroma volume.
(A) Orthogonal projections produced from z-stack images (30 µm in depth) of mesophyll cells from wild-type (WT) or atg7 leaves accumulating the chloroplast stroma marker RBCS-mRFP. Second rosette leaves of 21-day-old plants were excised at dawn and incubated in 10 mM MES-NaOH in the dark for 24 hr. Mesophyll cells were observed before (0 hr) and after (Dark 24 hr) the treatment. Green, RBCS-mRFP. Scale bars, 10 µm. (B) Violin plot showing the volume of chloroplast stroma labeled by RBCS-mRFP, measured from the observations described in (A). Different lowercase letters denote significant differences based on Tukey’s test (p < 0.05). Yellow bars indicate mean values. Five individual plants were observed, and the volumes of 564 (WT, 0 hr), 554 (WT, dark 24 hr), 597 (atg7, 0 hr), and 725 (atg7, dark 24 hr) chloroplasts were scored.
-
Figure 1—figure supplement 2—source data 1
Source data for the graph in Figure 1—figure supplement 2.
- https://cdn.elifesciences.org/articles/93232/elife-93232-fig1-figsupp2-data1-v1.zip
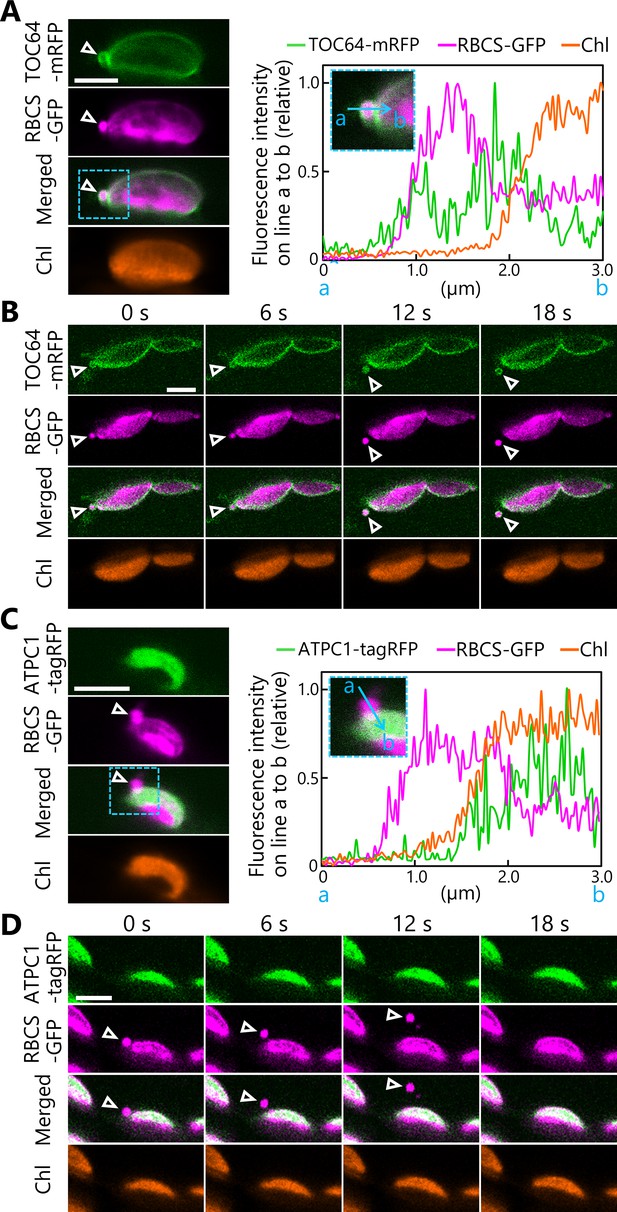
Chloroplast buds containing stroma and envelope components are released from the chloroplasts.
Time-lapse observations of Arabidopsis mesophyll cells accumulating the chloroplast stroma marker along with an envelope marker or a thylakoid membrane marker. Leaves accumulating stromal RBCS-GFP along with outer envelope–bound TOC64-mRFP (A, B) or with thylakoid membrane–bound ATPC1-tagRFP (C, D) were incubated in sugar-free solution in darkness for 5–9 hr from dawn and then observed. Second rosette leaves from 21- to 22-day-old plants were used. Images in (B) or (D) are still frames from Videos 4 and 6, respectively. Time scales above the images indicate the elapsed time from the start of the respective videos. Arrowheads indicate chloroplast budding structures. Scale bars, 5 µm. Green, TOC64-mRFP or ATPC1-tagRFP; magenta, RBCS-GFP; orange, chlorophyll (Chl) fluorescence. The graphs in (A, C) show fluorescence intensities along the blue lines (a to b) in the magnified images of the area indicated by dashed blue boxes. The intensities are shown relative to the maximum intensity for each fluorescence channel, set to 1.
-
Figure 2—source data 1
Source data for the graphs in Figure 2.
- https://cdn.elifesciences.org/articles/93232/elife-93232-fig2-data1-v1.xlsx
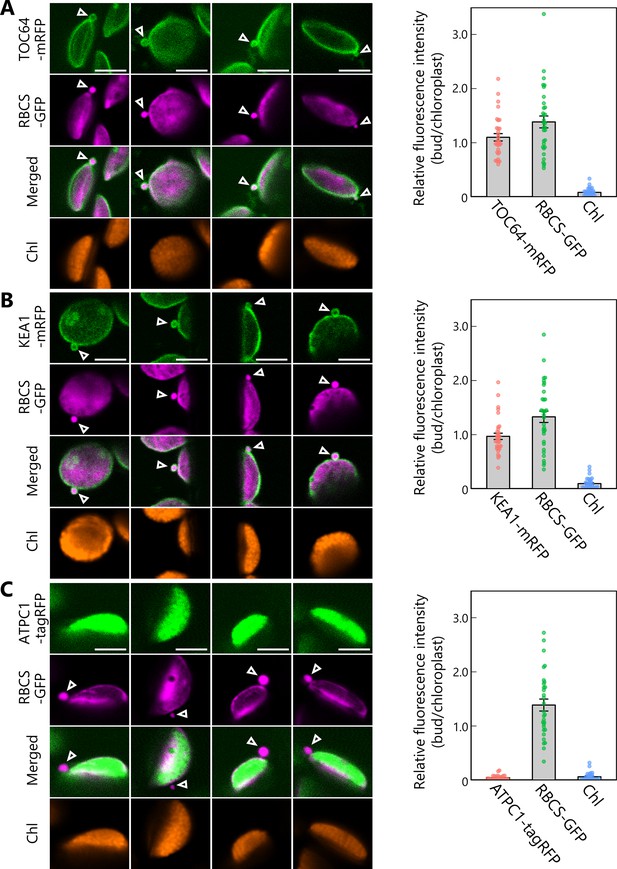
Chloroplast buds contain stroma and envelope proteins.
Second rosette leaves accumulating stromal RBCS-GFP along with outer envelope membrane–bound TOC64-mRFP (A), inner envelope membrane–bound KEA1-mRFP (B), or thylakoid membrane–bound ATPC1-tagRFP (C) were incubated in sugar-free solution in the dark for 5–9 hr from dawn, and chloroplast budding structures were observed. Second rosette leaves from 21- to 22-day-old plants were used. Arrowheads indicate chloroplast budding structures. Four representative images of chloroplast buds are shown for each plant line. Scale bars, 5 µm. Green, TOC64-mRFP, KEA1-mRFP, or ATPC1-tagRFP; magenta, RBCS-GFP; orange, chlorophyll (Chl) fluorescence. The graphs show the relative ratio of GFP, RFP, or chlorophyll fluorescence intensity per unit area within chloroplast budding structures vs. their neighboring chloroplasts (bud/chloroplast). The intensities from 33 (A), 32 (B), or 30 (C) chloroplast buds from eight individual plants were measured. Values are means ± SE (n = 30–33). Dots represent individual data points in each graph.
-
Figure 2—figure supplement 1—source data 1
Source data for the graphs in Figure 2—figure supplement 1.
- https://cdn.elifesciences.org/articles/93232/elife-93232-fig2-figsupp1-data1-v1.zip
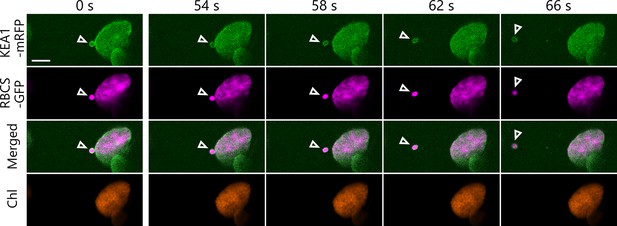
A released chloroplast bud contains an inner envelope marker protein.
Time-lapse observations of Arabidopsis mesophyll cells accumulating the chloroplast stroma marker RBCS-GFP along with inner envelope membrane–bound KEA1-mRFP. The second rosette leaf from a 22-day-old plant was incubated in sugar-free solution in the dark for 6 hr from dawn and observed. The images are still frames from Video 5. Time scales above the images indicate the elapsed time from the start of the video. Arrowheads indicate chloroplast budding structures. Scale bars, 5 µm. Green, KEA1-mRFP; magenta, RBCS-GFP; orange, chlorophyll (Chl) fluorescence.
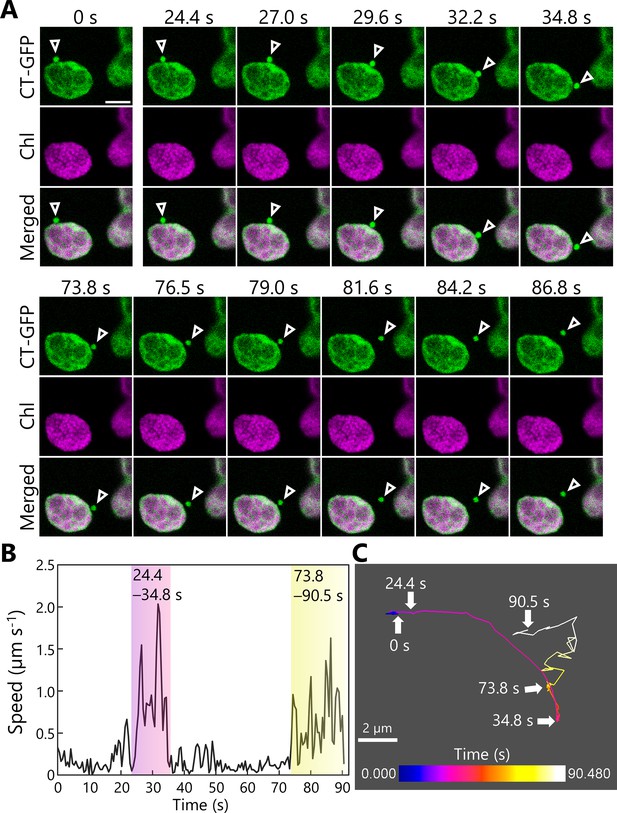
Tracking the transport of a Rubisco-containing body (RCB).
A leaf accumulating chloroplast stroma–targeted GFP (CT-GFP) was incubated in sugar-free solution in darkness for 6 hr from dawn, and the transport of an RCB was tracked. The second rosette leaf from a 21-day-old plant was used. (A) Confocal images during the periods when the RCB moved quickly (24.4–34.8 and 73.8–90.5 s). Arrowheads indicate an RCB. The images are still frames from Video 7. Time scales above the images indicate the elapsed time from the start of the video. Green, CT-GFP; magenta, chlorophyll (Chl) fluorescence. Scale bar, 5 µm. (B) Calculated speed of the tracked RCB in (A). (C) The track of the RCB. The color of the track line changes over time, as indicated by the color bar. Scale bar, 2 µm.
-
Figure 3—source data 1
Source data for the graph in Figure 3.
- https://cdn.elifesciences.org/articles/93232/elife-93232-fig3-data1-v1.xlsx
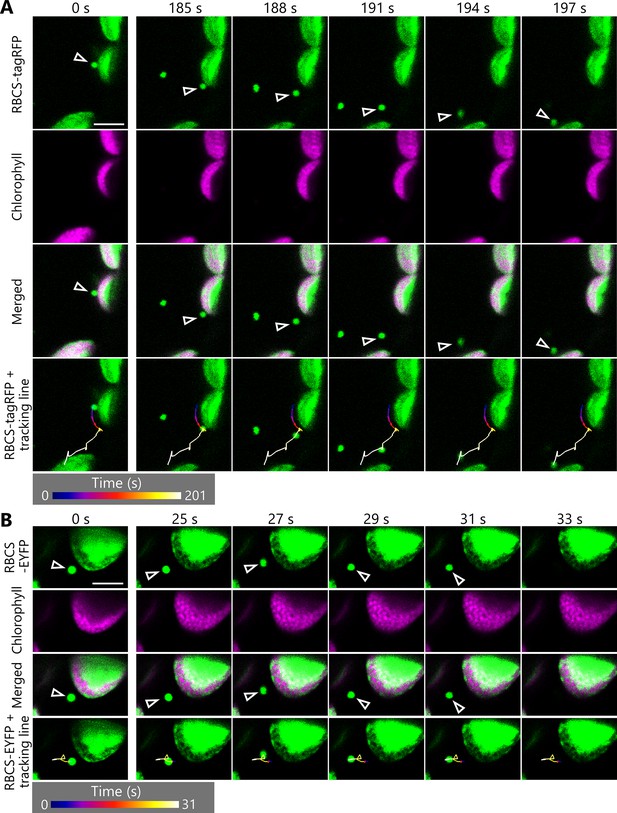
Rubisco-containing bodies (RCBs) appear to begin random movement during their tracking.
A leaf accumulating RBCS-tagRFP (A) or RBCS-EYFP (B) was incubated in sugar-free solution in the dark for 7–8 hr from dawn, and the transport of an RCB was tracked. Second rosette leaves from 22-day-old plants were used. Arrowheads indicate a tracked RCB. The images are still frames from Videos 8 and 9, respectively. The images show the time when RCBs appeared to begin random movement (185–197 s in A or 25–33 s in B). Time scales above the images indicate the elapsed time from the start of the respective videos. Green, RBCS-tagRFP or RBCS-EYFP; magenta, chlorophyll fluorescence. Scale bars, 5 µm. The color of the track line changes over time, as indicated by the color bars below the images.
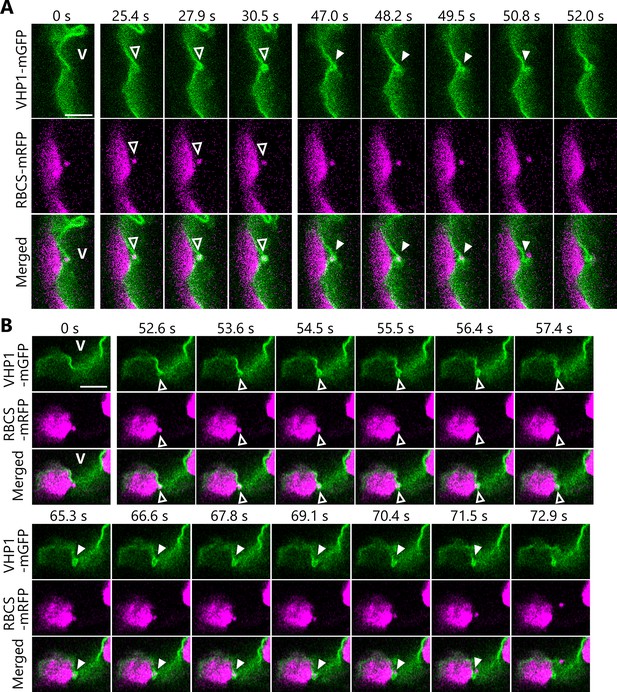
Dynamics of the vacuolar membrane during the incorporation of Rubisco-containing bodies (RCBs).
Leaves accumulating the chloroplast stroma marker RBCS-mRFP along with the vacuolar membrane marker VHP1-mGFP were incubated in sugar-free solution in darkness for 6–8 hr from dawn, and the behavior of cytosolic RCBs was monitored. Second rosette leaves from 21- or 23-day-old plants were used. The images when the vacuolar membrane engulfs an RCB (25.4–30.5 s in A and 52.6–57.4 s in B) and when an RCB is incorporated into the vacuolar lumen (47.0–52.0 s in A and 65.3–72.9 s in B) are shown. The images in (A) and (B) are still frames from Videos 10 and 11, respectively. Time scales above the images indicate the elapsed time from the start of the respective videos. Open arrowheads indicate an RCB engulfed by the vacuolar membrane. Closed arrowheads indicate the open site of the vacuolar membrane for the release of an RCB into vacuolar lumen. V indicates the region of the vacuolar lumen. Green, VHP1-mGFP; magenta, RBCS-mRFP. Scale bars, 5 µm.
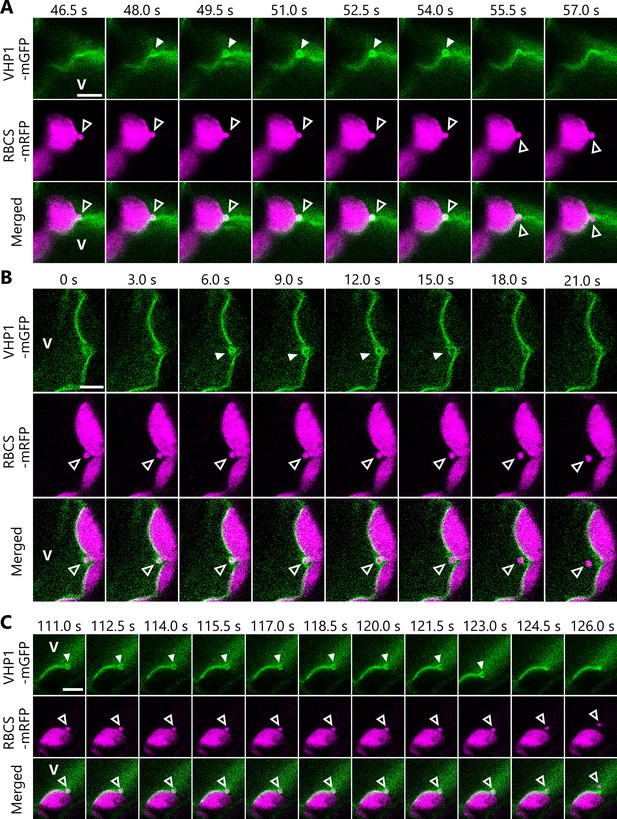
Additional observations of the incorporation of Rubisco-containing bodies (RCBs) into the vacuolar lumen.
Additional time-lapse data of the experiments described in Figure 4. The second rosette leaves from 21- (A) or 24-day-old (B, C) plants were used. The images in (A), (B) and (C) are still frames from Videos 12–14, respectively. Time scales above the images indicate the elapsed time from the start of the respective videos. Open arrowheads indicate an RCB engulfed by the vacuolar membrane. Closed arrowheads indicate the vacuolar membrane structure that engulfs an RCB. V indicates the region of the vacuolar lumen. Green, VHP1-mGFP; magenta, RBCS-mRFP. Scale bars, 5 µm.
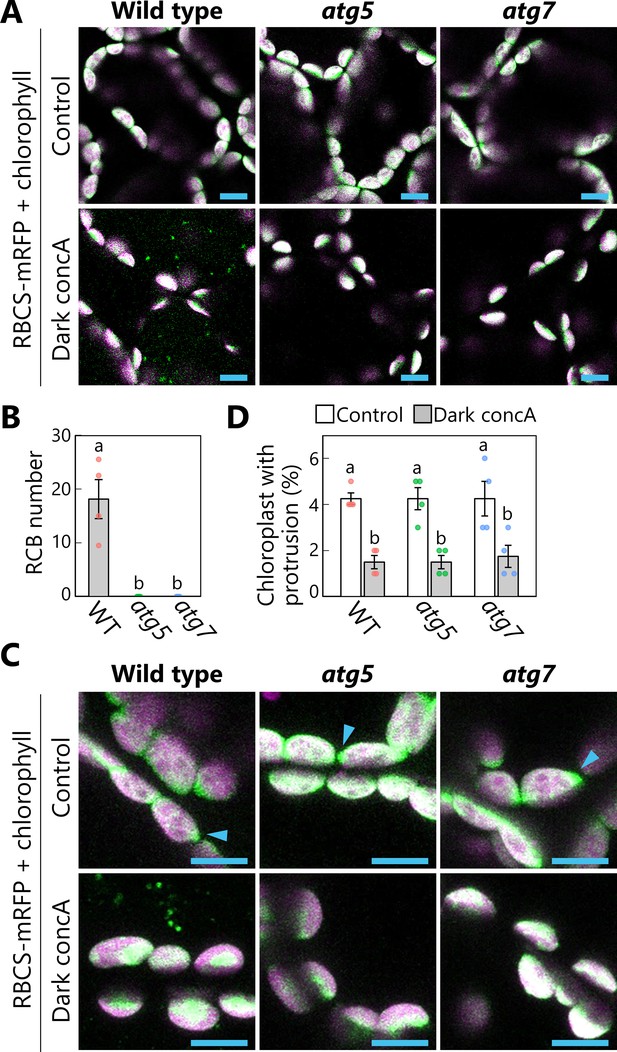
Autophagy deficiency does not increase the number of chloroplast protrusions during a 1-day dark treatment.
Leaves from wild-type (WT), atg5, or atg7 plants accumulating the chloroplast stroma marker RBCS-mRFP were incubated in sugar-free solution containing 1 µM concanamycin A (concA) for 1 day in darkness. Second rosette leaves from 21-day-old plants were used. Two-dimensional (2D) images of mesophyll cells were acquired (A), and the number of accumulated Rubisco-containing bodies (RCBs) in the vacuoles was scored (B). Small puncta containing RBCS-mRFP without the chlorophyll signal appear green and are RCBs in the vacuole. Leaves from untreated plants are shown as control. The appearance of chloroplast protrusions was observed from orthogonal projections created from z-stack images (15 µm in depth; C), and the proportion of chloroplasts forming protrusion structures out of 50 chloroplasts was scored (D). Scale bars, 10 µm. Green, RBCS-mRFP; magenta, chlorophyll fluorescence. Only the merged channels are shown. The overlapping regions of RBCS-mRFP and chlorophyll signals appear white. Arrowheads indicate the structures that were counted as a chloroplast protrusion in (D). Different lowercase letters denote significant differences based on Tukey’s test (p < 0.05). Values are means ± SE (n = 4). Dots represent individual data points in each graph.
-
Figure 5—source data 1
Source data for the graphs in Figure 5.
- https://cdn.elifesciences.org/articles/93232/elife-93232-fig5-data1-v1.zip
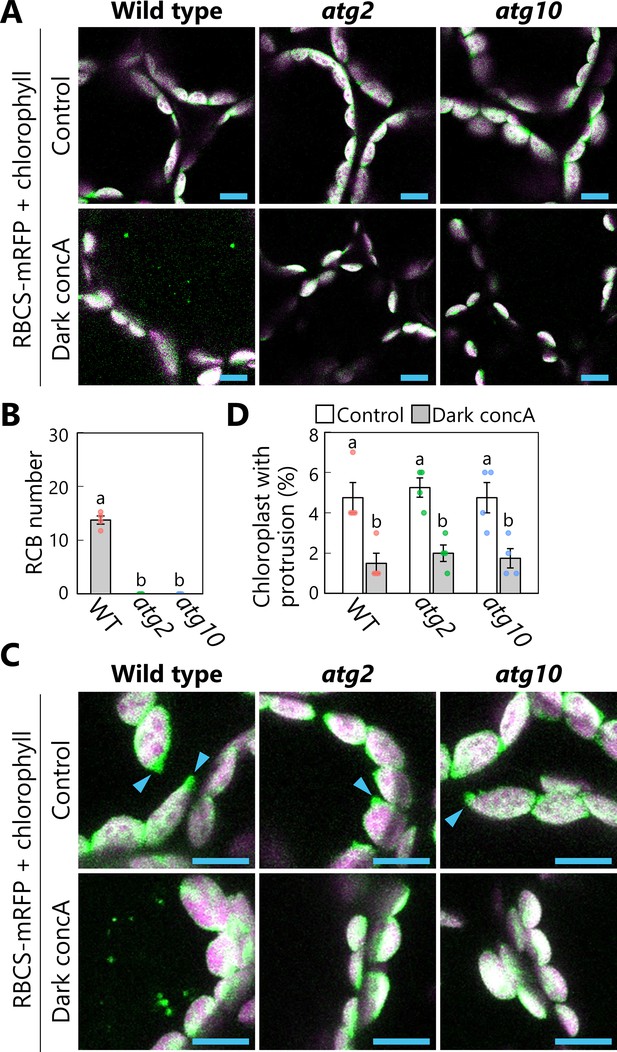
Chloroplast protrusions do not increase in atg2 or atg10 mutant leaves during a 1-day dark treatment.
The experiments described in Figure 5 were performed on leaves from wild-type (WT), atg2, or atg10 plants accumulating the chloroplast stroma marker RBCS-mRFP. Second rosette leaves from 21-day-old plants were incubated in sugar-free solution containing 1 µM concanamycin A (concA) for 1 day in darkness. Two-dimensional (2D) images of mesophyll cells were acquired (A), and the number of accumulated Rubisco-containing bodie (RCBs) in the vacuoles was scored (B). Small puncta containing RBCS-mRFP without the chlorophyll signal appear green and are RCBs in the vacuole. Leaves from untreated plants are shown as control. The appearance of chloroplast protrusions was observed from orthogonal projections created from z-stack images (15 µm in depth; C), and the proportion of chloroplasts forming protrusion structures out of 50 chloroplasts was scored (D). Scale bars, 10 µm. Green, RBCS-mRFP; magenta, chlorophyll fluorescence. Only the merged channels are shown. The overlapping regions of RBCS-mRFP and chlorophyll signals appear white. Arrowheads indicate the structures that were counted as a chloroplast protrusion in (D). Different lowercase letters denote significant differences based on Tukey’s test (p < 0.05). Values are means ± SE (n = 4). Dots represent individual data points in each graph.
-
Figure 5—figure supplement 1—source data 1
Source data for the graphs in Figure 5—figure supplement 1.
- https://cdn.elifesciences.org/articles/93232/elife-93232-fig5-figsupp1-data1-v1.zip
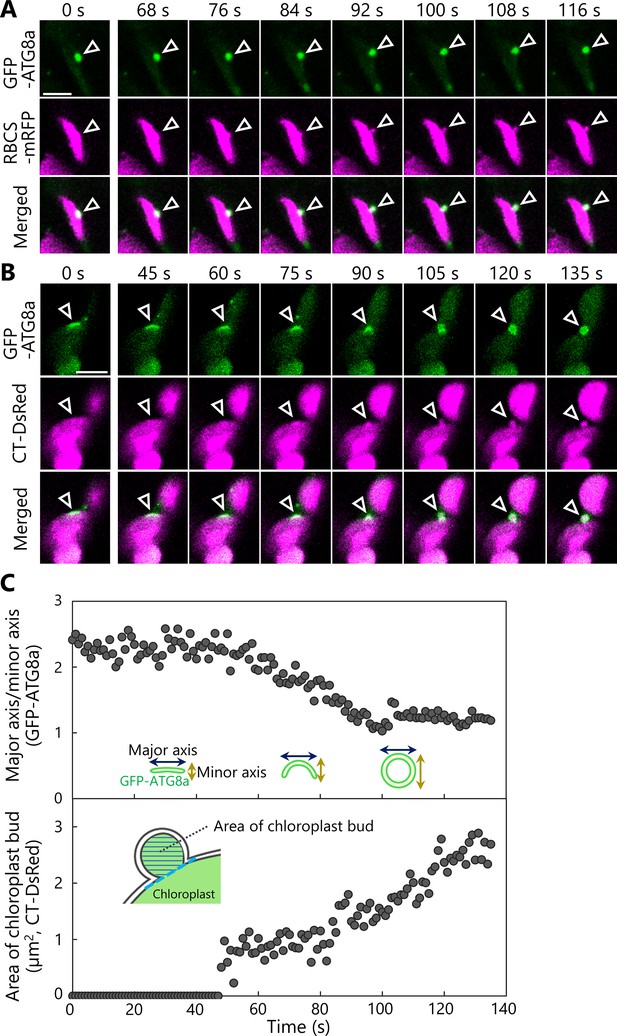
The formation of a chloroplast bud and the maturation of the chloroplast-associated isolation membrane occur concomitantly.
Leaves accumulating the chloroplast stroma marker, RBCS-mRFP (A) or CT-DsRed (B), and the isolation membrane marker GFP-ATG8a were incubated in sugar-free solution in darkness for 6 hr from dawn (A) or 21 hr from the period in light (B) and then observed. Second rosette leaves from 23-day-old plants were used. Arrowheads indicate the position of the chloroplast-associated isolation membrane. Images in (A) and (B) are still frames from Videos 15 and 16, respectively. Time scales above the images indicate the elapsed time from the start of the respective videos. Green, GFP-ATG8a; magenta, RBCS-mRFP or CT-DsRed. Scale bars, 5 µm. (C) Time-dependent changes in the ratio of the major axis to the minor axis in the GFP-ATG8a-labeled isolation membrane (top) or in the area of the chloroplast budding structure (bottom) as measured from the images in (B).
-
Figure 6—source data 1
Source data for the graphs in Figure 6.
- https://cdn.elifesciences.org/articles/93232/elife-93232-fig6-data1-v1.xlsx
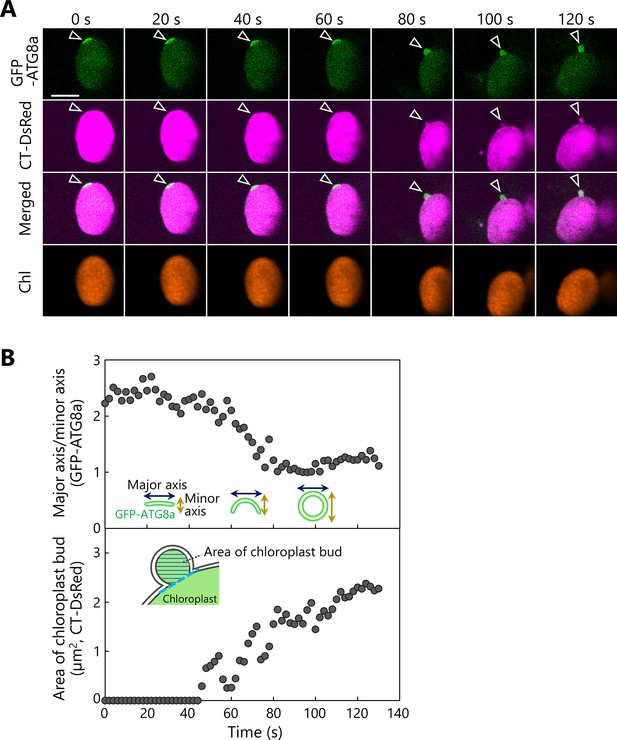
Another observation of the budding of the isolation membrane–associated site in a chloroplast.
(A) A leaf accumulating the chloroplast stroma marker CT-DsRed and the isolation membrane marker GFP-ATG8a was incubated in sugar-free solution in darkness for 6 hr from dawn and then observed. A second rosette leaf from a 22-day-old plant was used. Arrowheads indicate the position of the chloroplast-associated isolation membrane. The images are still frames from Video 17. Time scales above the images indicate the elapsed time from the start of the video. Green, GFP-ATG8a; magenta, CT-DsRed. Scale bars, 5 µm. (B) Time-dependent changes in the ratio of the major axis to the minor axis in the GFP-ATG8a-labeled isolation membrane (top) or in the area of the chloroplast budding structure (bottom), as measured from the images in (A).
-
Figure 6—figure supplement 1—source data 1
Source data for the graphs in Figure 6—figure supplement 1.
- https://cdn.elifesciences.org/articles/93232/elife-93232-fig6-figsupp1-data1-v1.xlsx
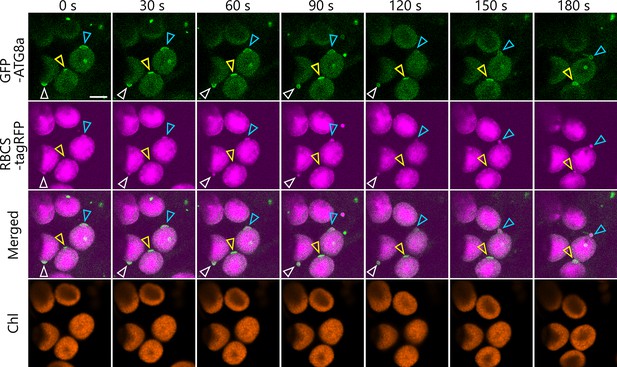
Chloroplast buds surrounded by the isolation membrane appear in multiple chloroplasts.
A leaf accumulating the chloroplast stroma marker RBCS-tagRFP and the isolation membrane marker GFP-ATG8a was incubated in sugar-free solution in darkness for 5 hr from dawn and then observed. A second rosette leaf from a 21-day-old plant was used. White, yellow, and blue arrowheads indicate the isolation membrane–associated site in different chloroplasts, respectively. The images are still frames from Video 18. Time scales above the images indicate the elapsed time from the start of the video. Green, GFP-ATG8a; magenta, RBCS-tagRFP; orange, chlorophyll (Chl) fluorescence. Scale bars, 5 µm.
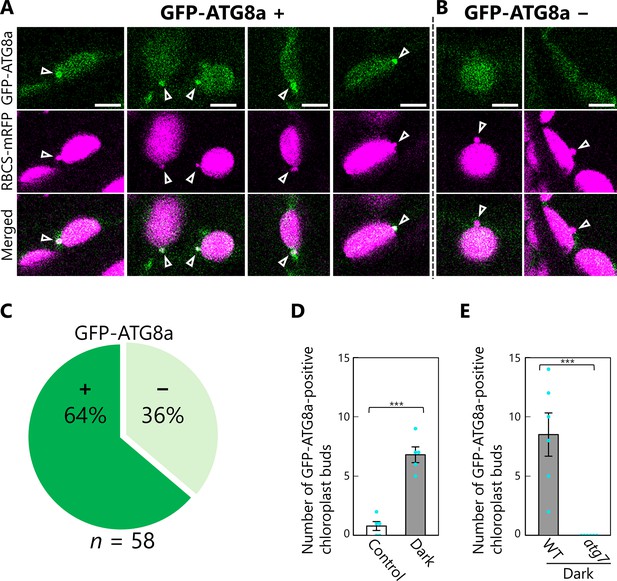
Proportion of chloroplast buds engulfed by GFP-ATG8a-labeled isolation membranes.
Leaves accumulating the chloroplast stroma marker RBCS-mRFP and the isolation membrane marker GFP-ATG8a were incubated in sugar-free solution in the dark for 5–9 hr from dawn, and chloroplast budding structures were observed. Second rosette leaves from 22- to 23-day-old plants were used. Four representative images of chloroplasts buds that are surrounded by GFP-ATG8a signals (A) or two representative images of chloroplast buds that are not labeled by GFP-ATG8a signals (B) are shown. Arrowheads indicate chloroplast budding structures. Green, GFP-ATG8a; magenta, RBCS-mRFP. Scale bars, 5 µm. (C) The proportions of chloroplast buds that are labeled by GFP-ATG8a signals (+) or not (−) were calculated from observations of 58 chloroplast buds from 8 individual plants. (D) The number of GFP-ATG8a-positive chloroplast buds, as shown in (A), was counted in the fixed regions from leaves incubated in the dark for 5–9 hr from dawn (Dark) or from untreated leaves of plants grown under normal conditions (Control). Five individual plants were observed. (E) Leaves from wild-type (WT) or atg7 plants accumulating RBCS-mRFP and GFP-ATG8a were incubated in the dark for 5–9 hr from dawn, and the number of GFP-ATG8a-positive chloroplast buds was counted. Six individual plants were observed. Values are means ± SE (n = 5–6). Asterisks denote significant differences based on t-test (***p < 0.001). Dots represent individual data points in each graph.
-
Figure 6—figure supplement 3—source data 1
Source data for the graphs in Figure 6—figure supplement 3.
- https://cdn.elifesciences.org/articles/93232/elife-93232-fig6-figsupp3-data1-v1.xlsx
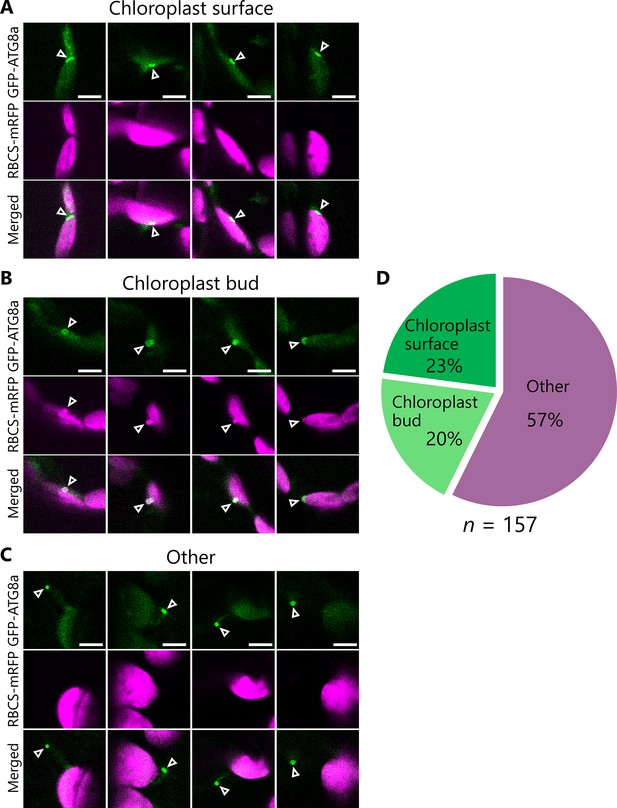
Proportion of GFP-ATG8a-labeled structures that are associated with chloroplasts.
Leaves accumulating the chloroplast stroma marker RBCS-mRFP and the isolation membrane marker GFP-ATG8a were incubated in sugar-free solution in the dark for 5–9 hr from dawn, and GFP-ATG8a-labeled structures in mesophyll cells were observed. Second rosette leaves from 22-day-old plants were used. GFP-ATG8a-positive structures were classified into three types: flattened structures associated with the chloroplast surface (A), spherical or curved structures associated with chloroplast budding structures (B), and other structures (C). Four representative images of each type are shown. Green, GFP-ATG8a; magenta, RBCS-mRFP. Scale bars, 5 µm. (D) The proportions of the three types of GFP-ATG8a-labeled structures were calculated from observations of 157 GFP-ATG8a-labeled structures from 13 individual plants.
-
Figure 6—figure supplement 4—source data 1
Source data for the graph in Figure 6—figure supplement 4.
- https://cdn.elifesciences.org/articles/93232/elife-93232-fig6-figsupp4-data1-v1.xlsx
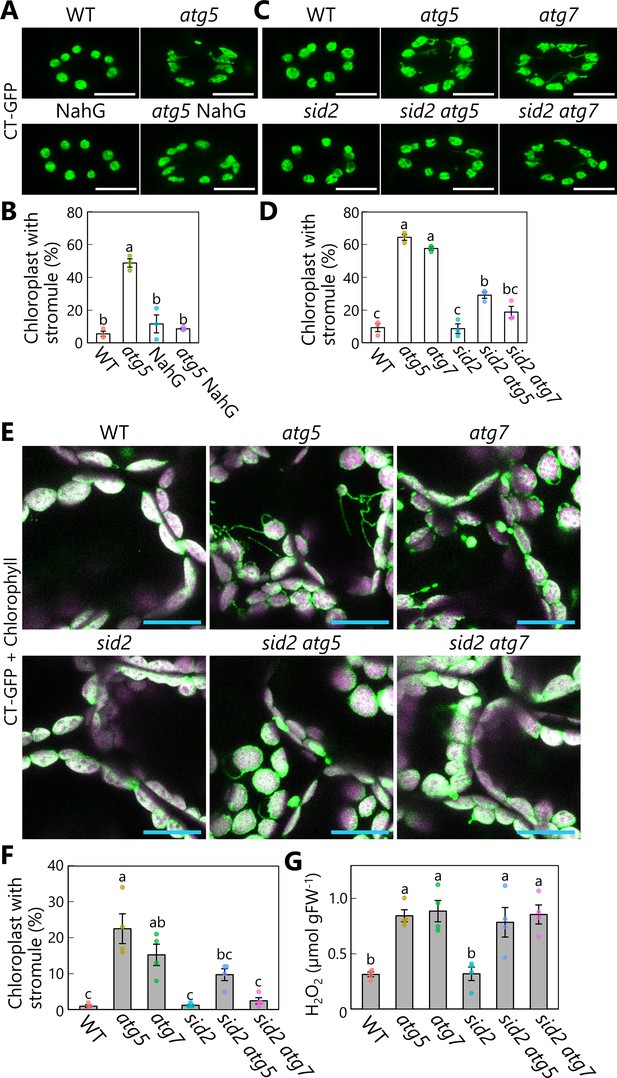
Diminished salicylic acid signal suppresses stromule formation in autophagy-deficient mutants.
(A) Orthogonal projections produced from z-stack images (10 μm in depth) of guard cells from wild-type (WT), atg5, NahG, and atg5 NahG leaves accumulating chloroplast stroma−targeted GFP (CT-GFP). Second rosette leaves from 13-day-old plants were used. Scale bars, 10 µm. (B) Percentage of chloroplasts forming stromules in 10 stomata, from the observations described in (A). (C) Orthogonal projections produced from z-stack images (10 μm in depth) of guard cells from WT, atg5, atg7, sid2, sid2 atg5, and sid2 atg7 leaves accumulating CT-GFP. Scale bars, 10 µm. (D) Percentage of chloroplasts forming stromules in 10 stomata, from the observations described in (C). (E) Orthogonal projections produced from z-stack images (15 µm in depth) of mesophyll cells from WT, atg5, atg7, sid2, sid2 atg5, and sid2 atg7 leaves accumulating CT-GFP. Third rosette leaves from 36-day-old plants were observed. Green, CT-GFP; magenta, chlorophyll fluorescence. Only the merged channels are shown. Scale bars, 20 µm. (F) Percentage of chloroplasts forming stromules out of 50 chloroplasts in mesophyll cells, from the observations described in (E). (G) Hydrogen peroxide (H2O2) content in third rosette leaves from 36-day-old WT, atg5, atg7, sid2, sid2 atg5, and sid2 atg7 plants. Different lowercase letters denote significant differences based on Tukey’s test (p < 0.05). Values are means ± SE (n = 3 in B and D or 4 in F and G). Dots represent individual data points in each graph.
-
Figure 7—source data 1
Source data for the graphs in Figure 7.
- https://cdn.elifesciences.org/articles/93232/elife-93232-fig7-data1-v1.zip
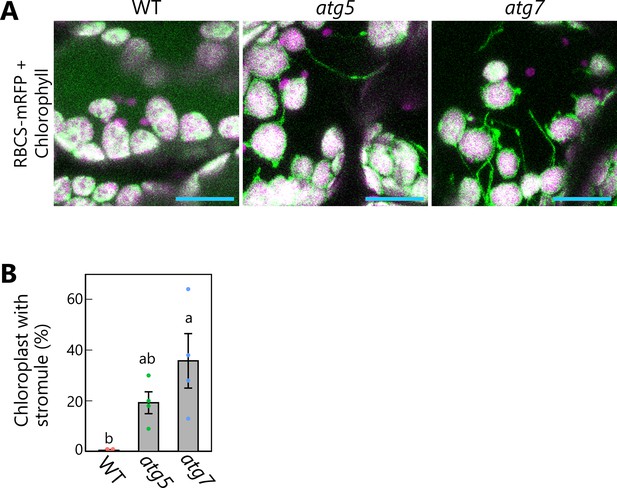
Autophagy deficiency activates stromule formation from mesophyll chloroplasts accumulating RBCS-mRFP in senescing leaves.
(A) Orthogonal projections produced from z-stack images (15 µm in depth) of mesophyll cells from leaves of wild-type (WT), atg5, and atg7 plants accumulating RBCS-mRFP. Third rosette leaves from 36-day-old plants were observed. Green, RBCS-mRFP; magenta, chlorophyll fluorescence. Only the merged channels are shown. Spherical bodies only exhibiting chlorophyll signal represent a portion of chloroplasts in pavement cells. Scale bars, 20 µm. (B) Percentage of chloroplasts forming stromules out of 50 chloroplasts in mesophyll cells, from the observations described in (A). Different lowercase letters denote significant differences based on Tukey’s test (p < 0.05). Values are means ± SE (n = 4). Dots represent individual data points in the graph.
-
Figure 7—figure supplement 1—source data 1
Source data for the graph in Figure 7—figure supplement 1.
- https://cdn.elifesciences.org/articles/93232/elife-93232-fig7-figsupp1-data1-v1.zip
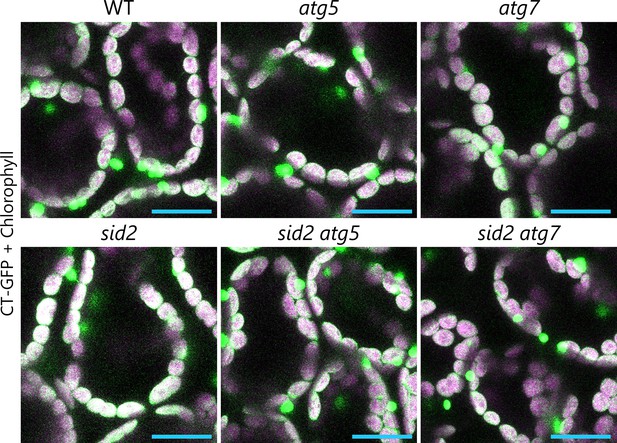
Autophagy deficiency does not activate stromule formation from mesophyll chloroplasts in young leaves.
Orthogonal projections produced from z-stack images (15 µm in depth) of mesophyll cells from wild-type (WT), atg5, atg7, sid2, sid2 atg5, and sid2 atg7 leaves accumulating CT-GFP. Third rosette leaves from 20-day-old plants were observed. Green, CT-GFP; magenta, chlorophyll fluorescence. Only the merged channels are shown. Scale bars, 20 µm.
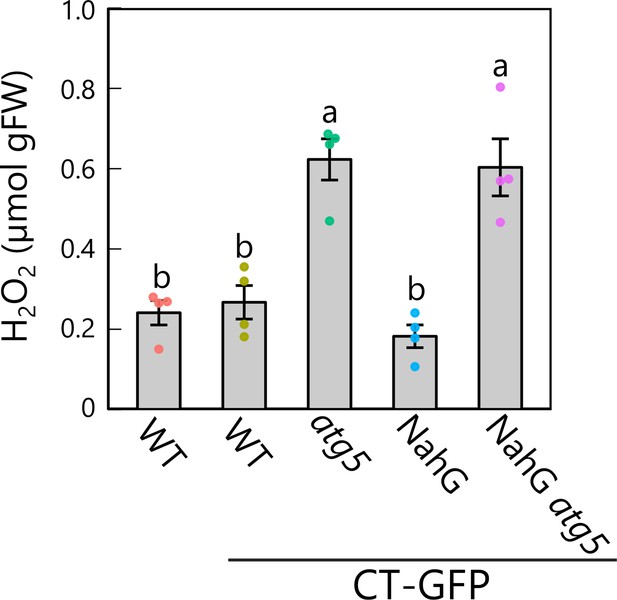
NahG expression does not counteract the increase in hydrogen peroxide (H2O2) content produced by the atg5 mutation.
H2O2 contents in third rosette leaves from 36-day-old plants. Wild-type (WT) plants without any transgenic construct and CT-GFP-expressing plants in the WT, atg5, NahG, and atg5 NahG backgrounds were used. Different lowercase letters denote significant differences based on Tukey’s test (p < 0.05). Values are means ± SE (n = 4). Dots represent individual data points.
-
Figure 7—figure supplement 3—source data 1
Source data for the graph in Figure 7—figure supplement 3.
- https://cdn.elifesciences.org/articles/93232/elife-93232-fig7-figsupp3-data1-v1.zip
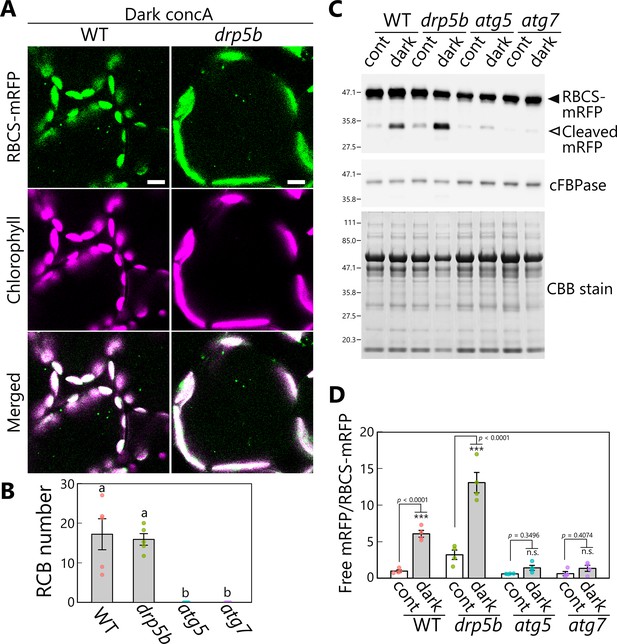
DRP5B is dispensable for chloroplast autophagy in sugar-starved leaves.
(A) Confocal images of mesophyll cells from wild-type (WT) and drp5b leaves accumulating the stroma marker RBCS-mRFP. Second rosette leaves from 21-day-old plants were incubated in sugar-free solution containing 1 µM concanamycin A (concA) in darkness for 1 day. Green, RBCS-mRFP; magenta, chlorophyll fluorescence. Scale bars, 10 µm. (B) Number of accumulated Rubisco-containing bodies (RCBs) in WT, drp5b, atg5, and atg7 leaves, counted from the observations of leaves incubated in the dark with concA described in (A). Different lowercase letters denote significant differences based on Tukey’s test (p < 0.05). Values are means ± SE (n = 4–5). (C) Biochemical detection of autophagy flux for chloroplast stroma based on a free RFP assay. RFP and cFBPase (loading control) were detected by immunoblotting of soluble protein extracts from leaves of WT, drp5b, atg5, and atg7 plants accumulating RBCS-mRFP. Protein extracts from either untreated control leaves (cont) or leaves after 2 days of incubation in darkness (dark) were used. Total protein was detected by Coomassie Brilliant Blue (CBB) staining as a loading control. The filled arrowhead indicates RBCS-mRFP fusion, and the open arrowhead indicates free mRFP derived from the cleavage of RBCS-mRFP. (D) Quantification of the free mRFP/RBCS-mRFP ratio shown relative to that of untreated WT plants, which was set to 1. Asterisks denote significant differences based on t-test (***p < 0.001; n.s., not significant). Values are means ± SE (n = 4). Dots represent individual data points in each graph.
-
Figure 8—source data 1
Original files for western blot analysis displayed in Figure 8C.
- https://cdn.elifesciences.org/articles/93232/elife-93232-fig8-data1-v1.zip
-
Figure 8—source data 2
PDF file containing original western blots for Figure 8C, indicating the relevant bands, genotypes, and conditions.
- https://cdn.elifesciences.org/articles/93232/elife-93232-fig8-data2-v1.zip
-
Figure 8—source data 3
Source data for the graphs in Figure 8.
- https://cdn.elifesciences.org/articles/93232/elife-93232-fig8-data3-v1.zip
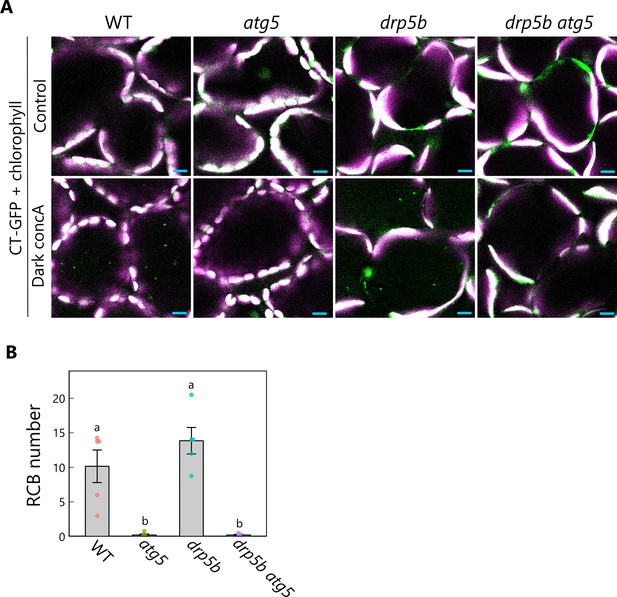
Production of Rubisco-containing bodies (RCBs) in drp5b mutants is ATG5 dependent.
(A) Confocal images of mesophyll cells from wild-type (WT), atg5, drp5b, and drp5b atg5 leaves accumulating the stroma marker CT-GFP. Second rosette leaves from 21-day-old plants were incubated in sugar-free solution containing 1 µM concanamycin A (concA) in darkness for 1 day. Second rosette leaves from untreated plants were used as control. Green, CT-GFP; magenta, chlorophyll fluorescence. Only the merged channels are shown. Small puncta containing CT-GFP without chlorophyll signal appear as green and are RCBs in the vacuole. Scale bars, 10 µm. (B) Number of accumulated RCBs, counted from the observations of leaves incubated in the dark with concA described in (A). Different lowercase letters denote significant differences based on Tukey’s test (p < 0.05). Values are means ± SE (n = 5). Dots represent individual data points.
-
Figure 8—figure supplement 1—source data 1
Source data for the graph in Figure 8—figure supplement 1.
- https://cdn.elifesciences.org/articles/93232/elife-93232-fig8-figsupp1-data1-v1.zip
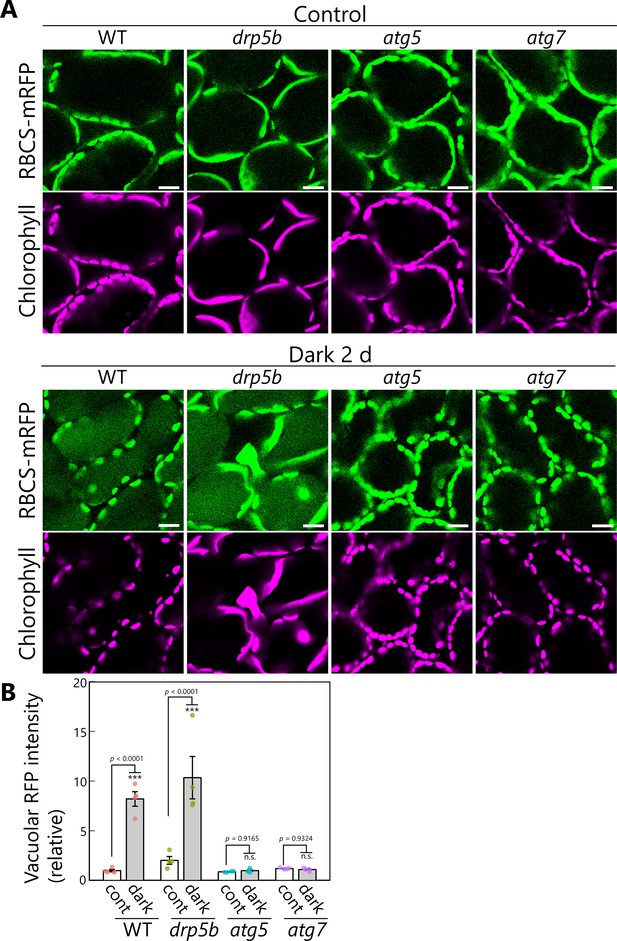
Vacuolar accumulation of stromal marker proteins in sugar-starved leaves.
(A) Confocal images of mesophyll cells from wild-type (WT), drp5b, atg5, and atg7 leaves accumulating the stroma marker RBCS-mRFP. Second rosette leaves from 21-day-old plants were incubated in sugar-free solution in darkness for 2 days. Second rosette leaves from untreated plants are shown as control. Green, RBCS-mRFP; magenta, chlorophyll fluorescence. Scale bars, 20 µm. (B) RFP intensity in the vacuolar lumen, as measured from the observations described in (A) and shown relative to that of untreated WT plants, which was set to 1. Asterisks denote significant differences based on t-test (***p < 0.001; n.s., not significant). Values are means ± SE (n = 4). Dots represent individual data points.
-
Figure 8—figure supplement 2—source data 1
Source data for the graph in Figure 8—figure supplement 2.
- https://cdn.elifesciences.org/articles/93232/elife-93232-fig8-figsupp2-data1-v1.zip
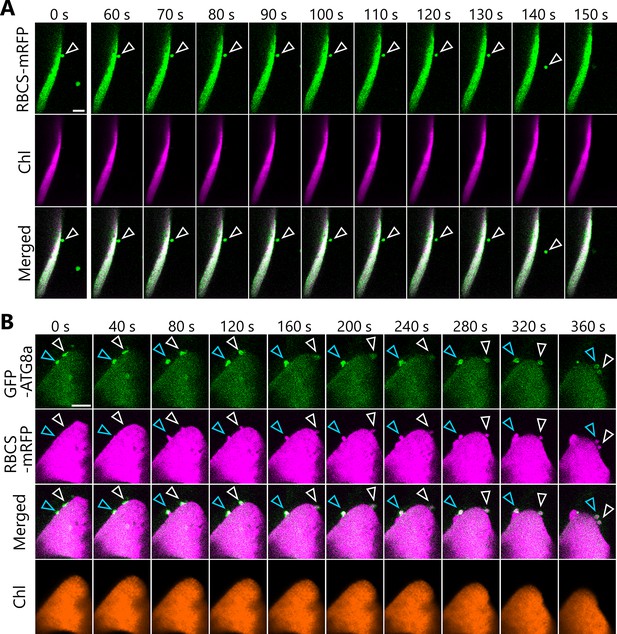
Formation and segmentation of chloroplast buds in leaves of the drp5b mutant.
(A) A leaf from the drp5b mutant accumulating the stroma marker RBCS-mRFP was incubated in sugar-free solution in darkness for 5 hr from dawn and then observed. Arrowheads indicate a chloroplast bud. Green, RBCS-mRFP; magenta, chlorophyll fluorescence (Chl). (B) A leaf from the drp5b mutant accumulating the stroma marker RBCS-mRFP and the isolation membrane marker GFP-ATG8a were incubated in sugar-free solution in darkness for 6 hr from dawn and then observed. Second rosette leaves from 21- to 22-day-old plants were used. Arrowheads indicate the position of the chloroplast-associated isolation membrane. White and blue arrowheads indicate different isolation membrane−associated sites in a chloroplast. Images in (A) and (B) are still frames from Videos 19 and 20, respectively. Time scales above the images indicate the elapsed time from the start of the respective videos. Green, GFP-ATG8a; magenta, RBCS-mRFP; orange, chlorophyll (Chl) fluorescence. Scale bars, 5 µm.

Mesophyll cells in a leaf of atg7 accumulating stromal CT-GFP, reconstructed from the data shown in the previous version of Figure 7–figure supplement 1.
(A) Individual channel images (CT-GFP and chlorophyll) from the merged orthogonal projection image shown in the previous version of Figure 7–figure supplement 1. The right panel shows the enhanced chlorophyll signal to clearly visualize the chloroplasts in epidermal cells. Green, CTGFP; magenta, chlorophyll fluorescence. Scale bar, 20 µm. (B) 3D structure of the merged image shown in (A). (C) Images of the cross section indicated by the blue dotted line (a to b) in B. Arrowheads indicate the edges of chloroplasts in epidermal cells.
Videos
Release of a chloroplast bud as visualized by chloroplast stroma–targeted GFP.
The second rosette leaf from a 21-day-old plant accumulating the chloroplast stroma–targeted GFP (CT-GFP) was incubated in sugar-free solution in darkness for time-lapse imaging with a two-photon excitation microscope equipped with a confocal spinning-disk unit. Arrowhead indicates a chloroplast budding structure. Three-dimensional (3D) reconstructed images (8 µm in depth) acquired about every 3 s are displayed at 10 frames/s. Scale bar, 5 µm. This video was used to generate Figure 1A.
Release of a chloroplast bud as visualized by RBCS-mRFP.
The second rosette leaf from a 22-day-old plant accumulating the chloroplast stroma marker RBCS-mRFP was incubated in sugar-free solution in darkness for time-lapse imaging with a two-photon excitation microscope equipped with a confocal spinning-disk unit. Arrowhead indicates a chloroplast budding structure. Three-dimensional (3D) reconstructed images (2 µm in depth) acquired about every 1.2 s are displayed at 10 frames/s. Scale bar, 5 µm. This video was used to generate Figure 1B.
Tracking of the stroma marker and chlorophyll fluorescence during the release of a chloroplast bud.
The second rosette leaf from a 20-day-old plant accumulating the chloroplast stroma marker RBCS-EYFP was incubated in sugar-free solution in darkness for time-lapse imaging with a two-photon excitation microscope equipped with a confocal spinning-disk unit. Arrowhead indicates a chloroplast budding structure. Three-dimensional (3D) reconstructed images (8 µm in depth) acquired every 4 s are displayed at 10 frames/s. Scale bar, 5 µm. Green, RBCS-EYFP; magenta, chlorophyll fluorescence. Only the video of the merged channels is shown. The overlapping regions of RBCS-EYFP and chlorophyll signals appear white. This video was used to generate Figure 1C.
A released chloroplast bud contains the outer envelope marker TOC64-mRFP.
The second rosette leaf from a 21-day-old plant accumulating the chloroplast stroma marker RBCS-GFP and the outer envelope marker TOC64-mRFP was incubated in sugar-free solution in darkness for time-lapse imaging. Arrowheads indicate chloroplast budding structures. Images acquired every 2 s are displayed at 10 frames/s. Scale bar, 5 µm. Green, TOC64-mRFP; magenta, RBCS-GFP. The video contains TOC64-mRFP and the merged channels. This video was used to generate Figure 2B.
A released chloroplast bud contains the inner envelope marker KEA1-mRFP.
The second rosette leaf from a 22-day-old plant accumulating the chloroplast stroma marker RBCS-GFP and the inner envelope marker KEA1-mRFP was incubated in sugar-free solution in the dark for time-lapse imaging. Arrowheads indicate chloroplast budding structures. Images acquired every 2 s are displayed at 10 frames/s. Scale bar, 5 µm. Green, KEA1-mRFP; magenta, RBCS-GFP. The video contains KEA1-mRFP and the merged channels. This video was used to generate Figure 2—figure supplement 2.
A released chloroplast bud does not contain the thylakoid membrane marker ATPC1-tagRFP.
The second rosette leaf from a 21-day-old plant accumulating the chloroplast stroma marker RBCS-GFP and the thylakoid membrane marker ATPC1-tagRFP was incubated in sugar-free solution in darkness for time-lapse imaging. Arrowheads indicate chloroplast budding structures. Images acquired every 2 s are displayed at 10 frames/s. Scale bar, 5 µm. Green, ATPC1-tagRFP; magenta, RBCS-GFP. The video contains ATPC1-tagRFP and the merged channels. This video was used to generate Figure 2D.
Tracking of a Rubisco-containing body (RCB) marked by CT-GFP.
The second rosette leaf from a 21-day-old plant accumulating chloroplast stroma–targeted GFP (CT-GFP) was incubated in sugar-free solution in darkness for time-lapse monitoring of an RCB marked by CT-GFP. Arrowhead indicates an RCB. Images acquired every 0.52 s are displayed at 20 frames/s. Scale bar, 5 µm. Green, CT-GFP; magenta, chlorophyll fluorescence. Only the video of the merged channels is shown. This video was used to generate Figure 3A.
Tracking of a Rubisco-containing body (RCB) marked by RBCS-tagRFP.
The second rosette leaf from a 22-day-old plant accumulating stromal RBCS-tagRFP was incubated in sugar-free solution in the dark for time-lapse monitoring of an RCB marked by RBCS-tagRFP. Arrowhead indicates an RCB. Images acquired every 1 s are displayed at 20 frames/s. Scale bar, 5 µm. Green, RBCS-tagRFP; magenta, chlorophyll fluorescence. Only the video of the merged channels is shown. This video was used to generate Figure 3—figure supplement 1A.
Tracking of a Rubisco-containing body (RCB) marked by RBCS-EYFP.
The second rosette leaf from a 22-day-old plant accumulating stromal RBCS-EYFP was incubated in sugar-free solution in the dark for time-lapse monitoring of an RCB marked by RBCS-EYFP. Arrowhead indicates an RCB. Images acquired every 1 s are displayed at 10 frames/s. Scale bar, 5 µm. Green, RBCS-EYFP; magenta, chlorophyll fluorescence. Only the video of the merged channels is shown. This video was used to generate Figure 3—figure supplement 1B.
Incorporation of a Rubisco-containing body (RCB) into the vacuolar lumen.
The second rosette leaf from a 21-day-old plant accumulating the chloroplast stroma marker RBCS-mRFP along with the vacuolar membrane marker VHP1-mGFP was incubated in sugar-free solution in darkness for time-lapse imaging. Arrowhead indicates an RCB incorporated into the vacuolar lumen. Images acquired every 1.27 s are displayed at 10 frames/s. Scale bar, 5 µm. Green, VHP1-mGFP; magenta, RBCS-mRFP. Only the video of the merged channels is shown. This video was used to generate Figure 4A.
Video 2 of the vacuolar incorporation of a Rubisco-containing body (RCB).
Additional time-lapse data for the experiment described in Figure 4 and Video 10. The second rosette leaf from a 23-day-old plant was used. Arrowhead indicates an RCB incorporated into the vacuolar lumen. Images acquired every 0.317 s are displayed at 25 frames/s. Scale bar, 5 µm. Green, VHP1-mGFP; magenta, RBCS-mRFP. Only the video of the merged channels is shown. This video was used to generate Figure 4B.
Video 3 of the vacuolar incorporation of a Rubisco-containing body (RCB).
Additional time-lapse data for the experiment described in Figure 4 and Video 10. Arrowhead indicates an RCB incorporated into the vacuolar lumen. Images acquired every 1.5 s are displayed at 10 frames/s. Scale bar, 5 µm. Green, VHP1-mGFP; magenta, RBCS-mRFP. Only the video of the merged channels is shown. This video was used to generate Figure 4—figure supplement 1A.
Video 4 of the vacuolar incorporation of a Rubisco-containing body (RCB).
Additional time-lapse data for the experiment described in Figure 4 and Video 10. Arrowhead indicates an RCB incorporated into the vacuolar lumen. Images acquired every 1.5 s are displayed at 10 frames/s. Scale bar, 5 µm. Green, VHP1-mGFP; magenta, RBCS-mRFP. Only the video of the merged channels is shown. This video was used to generate Figure 4—figure supplement 1B.
Video 5 of the vacuolar incorporation of a Rubisco-containing body (RCB).
Additional time-lapse data for the experiment described in Figure 4 and Video 10. Arrowhead indicates an RCB incorporated into the vacuolar lumen. Images acquired every 1.5 s are displayed at 10 frames/s. Scale bar, 5 µm. Green, VHP1-mGFP; magenta, RBCS-mRFP. Only the video of the merged channels is shown. This video was used to generate Figure 4—figure supplement 1C.
Budding of the isolation membrane–associated site within a chloroplast.
The second rosette leaf from a 23-day-old plant accumulating the chloroplast stroma marker RBCS-mRFP and the isolation membrane marker GFP-ATG8a was incubated in sugar-free solution in darkness for time-lapse imaging. Arrowheads indicate the portion of the chloroplast-associated isolation membrane. Images acquired every 4 s are displayed at 10 frames/s. Scale bar, 5 µm. Green, GFP-ATG8a; magenta, RBCS-mRFP. The video contains RBCS-mRFP and the merged channels. This video was used to generate Figure 6A.
Autophagosome development and chloroplast segmentation occur concomitantly.
The second rosette leaf from a 23-day-old plant accumulating the chloroplast stroma–targeted DsRed (CT-DsRed) and the isolation membrane marker GFP-ATG8a was incubated in sugar-free solution in darkness for time-lapse imaging. Arrowhead indicates the portion of the chloroplast-associated isolation membrane. Images acquired every 1 s are displayed at 20 frames/s. Scale bar, 5 µm. Green, GFP-ATG8a; magenta, CT-DsRed. Only the video of the merged channels is shown. This video was used to generate Figure 6B.
Another time-lapse assay showing the concomitant progression of autophagosome development and chloroplast segmentation.
The second rosette leaf from a 22-day-old plant accumulating the chloroplast stroma–targeted DsRed (CT-DsRed) and the isolation membrane marker GFP-ATG8a was incubated in sugar-free solution in darkness for time-lapse imaging. Arrowhead indicates the portion of the chloroplast-associated isolation membrane. Images acquired every 2 s are displayed at 10 frames/s. Scale bar, 5 µm. Green, GFP-ATG8a; magenta, CT-DsRed. Only the video of the merged channels is shown. This video was used to generate Figure 6—figure supplement 1.
Autophagy-related chloroplast segmentation occurs in sequence.
The second rosette leaf from a 21-day-old plant accumulating the chloroplast stroma marker RBCS-tagRFP and the isolation membrane marker GFP-ATG8a was incubated in sugar-free solution in darkness for time-lapse imaging. White, yellow, and blue arrowheads indicate the isolation membrane−associated sites in different chloroplasts. Images acquired every 2 s are displayed at 10 frames/s. Scale bar, 5 µm. Green, GFP-ATG8a; magenta, RBCS-tagRFP. Only the video of the merged channels is shown. This video was used to generate Figure 6—figure supplement 2.
Release of a chloroplast bud in a sugar-starved leaf of the drp5b mutant.
The second rosette leaf from a 21-day-old drp5b plant accumulating the chloroplast stroma marker RBCS-mRFP was incubated in sugar-free solution in darkness for time-lapse imaging. Arrowhead indicates a chloroplast bud. Images acquired every 1 s are displayed at 20 frames/s. Scale bar, 5 µm. Green, RBCS-mRFP; magenta, chlorophyll fluorescence. Only the video of the merged channels is shown. This video was used to generate Figure 9A.
An enlarged chloroplast caused by the drp5b mutation forms Rubisco-containing bodies (RCBs) along the isolation membrane−associated sites.
The second rosette leaf from a 22-day-old drp5b plant accumulating the chloroplast stroma marker RBCS-mRFP and the isolation membrane marker GFP-ATG8a was incubated in sugar-free solution in darkness for time-lapse imaging. White or blue arrowheads indicate different isolation membrane−associated sites in a chloroplast. Images acquired every 2 s are displayed at 20 frames/s. Scale bar, 5 µm. Green, GFP-ATG8a; magenta, RBCS-mRFP. Only the video of the merged channels is shown. This video was used to generate Figure 9B.
Tables
Reagent type (species) or resource | Designation | Source or reference | Identifiers | Additional information |
---|---|---|---|---|
Gene (Arabidopsis thaliana) | RBCS2B | TAIR | AT5G38420 | |
Gene (A. thaliana) | VHP1 | TAIR | AT1G15690 | |
Gene (A. thaliana) | TOC64-III | TAIR | AT3G17970 | |
Gene (A. thaliana) | ATG8a | TAIR | AT4G21980 | |
Gene (A. thaliana) | ATPC1 | TAIR | AT4G04640 | |
Gene (A. thaliana) | KEA1 | TAIR | AT1G01790 | |
Genetic reagent (A. thaliana) | atg5-1 | ABRC | SAIL_129_B07 | |
Genetic reagent (A. thaliana) | atg7-2 | ABRC | GABI_655B06 | |
Genetic reagent (A. thaliana) | atg2-1 | ABRC | SALK_076727 | |
Genetic reagent (A. thaliana) | atg10-1 | ABRC | SALK_084434 | |
Genetic reagent (A. thaliana) | drp5b (arc5-2) | ABRC | SAIL_71_D11 | |
Genetic reagent (A. thaliana) | sid2-2 | ABRC | CS16438 | |
Genetic reagent (A. thaliana) | NahG atg5-1 | 10.1105/tpc.109.068635 | ||
Genetic reagent (A. thaliana) | sid2-2 atg5-1 | 10.1105/tpc.109.068635 | ||
Genetic reagent (A. thaliana) | Pro35S:CT-GFP | 10.1126/science.276.5321.2039 | ||
Genetic reagent (A. thaliana) | Pro35S:CT-DsRed | 10.1093/pcp/pcab084 | ||
Genetic reagent (A. thaliana) | ProRBCS:RBCS-GFP | 10.1104/pp.108.122770 | ||
Genetic reagent (A. thaliana) | ProVHP1:VHP1-mGFP | 10.1105/tpc.114.127571 | ||
Genetic reagent (A. thaliana) | ProUBQ:GFP-ATG8a | 10.1093/pcp/pcaa162 | ||
Genetic reagent (A. thaliana) | ProTOC64:TOC64-mRFP | 10.26434/chemrxiv-2023-kx6gp | ||
Genetic reagent (A. thaliana) | ProRBCS:RBCS-EYFP | This paper | See ‘Plant materials’ in Materials and methods | |
Genetic reagent (A. thaliana) | ProRBCS:RBCS-mRFP | This paper | See ‘Plant materials’ in Materials and methods | |
Genetic reagent (A. thaliana) | ProRBCS:RBCS-tagRFP | This paper | See ‘Plant materials’ in Materials and methods | |
Genetic reagent (A. thaliana) | ProATPC1:ATPC1-tagRFP | This paper | See ‘Plant materials’ in Materials and methods | |
Genetic reagent (A. thaliana) | ProKEA1:KEA1-mRFP | This paper | See ‘Plant materials’ in Materials and methods | |
Antibody | Anti-RFP (Mouse, monoclonal) | MBL | M204-3 | (1:2000) |
Antibody | Anti-cFBPase (Rabbit, polyclonal) | Agrisera | AS04043 | (1:5000) |
Recombinant DNA reagent | ProRBCS:RBCS-EYFP | This paper | See ‘Plant materials’ in Materials and methods | |
Recombinant DNA reagent | ProRBCS:RBCS-mRFP | This paper | See ‘Plant materials’ in Materials and methods | |
Recombinant DNA reagent | ProRBCS:RBCS-tagRFP | This paper | See ‘Plant materials’ in Materials and methods | |
Recombinant DNA reagent | ProATPC1:ATPC1-tagRFP | This paper | See ‘Plant materials’ in Materials and methods | |
Recombinant DNA reagent | ProKEA1:KEA1-mRFP | This paper | See ‘Plant materials’ in Materials and methods | |
Sequence-based reagent | ATPC1_F | This paper | PCR primers (cloning) | CACCCATGGAGAGGGCTCGTACCTTAC |
Sequence-based reagent | ATPC1_R | This paper | PCR primers (cloning) | AACCTGTGCATTAGCTCCAG |
Sequence-based reagent | KEA1_F | This paper | PCR primers (cloning) | AGGAACCAATTCAGTCGACTCATGATCATAACAAGTCTC |
Sequence-based reagent | KEA1_R | This paper | PCR primers (cloning) | AAAGCTGGGTCTAGATATCCGATTACGACTGTGCCTCCTTC |
Commercial assay or kit | Amplex Red Hydrogen Peroxide/Peroxidase Assay Kit | Invitrogen | A22188 | |
Chemical compound, drug | Concanamycin A | Santa Cruz | sc-202111 | |
Software, algorithm | ZEN | Carl Zeiss | RRID:SCR_013672 | Image processing and quantification (microscopy) |
Software, algorithm | NIS-Elements C | Nikon | RRID:SCR_020318 | Image processing and quantification (microscopy) |
Software, algorithm | LAS X | Leica | RRID:SCR_013673 | Image processing and quantification (microscopy) |
Software, algorithm | Imaris | Bitplane | RRID:SCR_007370 | Image processing and quantification (microscopy) |
Software, algorithm | Image lab | Bio-Rad | RRID:SCR_014210 | Image processing and quantification (western blot) |
Software, algorithm | JMP14 | SAS | RRID:SCR_022199 | Statistics |