Mechanism of barotaxis in marine zooplankton
Figures
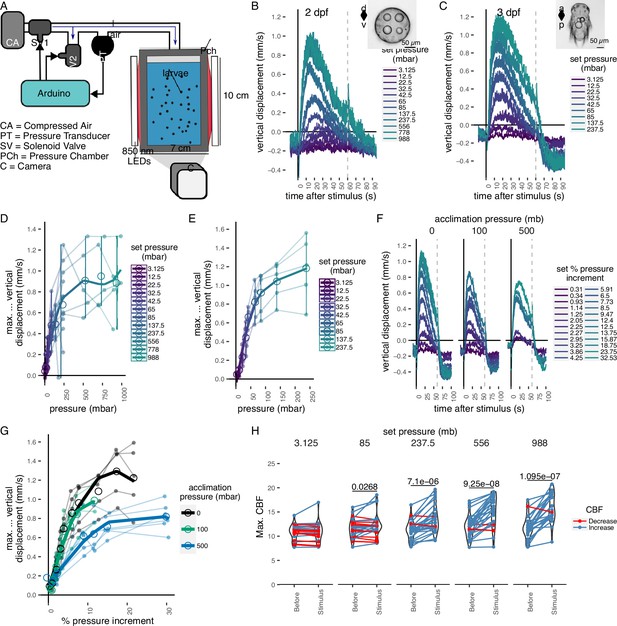
Pressure response in Platynereis larvae.
(A) Schematic of the behavioural setup used to stimulate larvae with controlled pressure levels. Vertical displacement of (B) 2-day-old and (C) 3-day-old larvae (insets) as a function of time relative to different step increases in pressure. Dashed line at 60 s indicates the end of stimulation. Each data point is the average of 2–12 (B) or 4–5 (C) batches of larvae. Maximum increase in relative vertical displacement of (D) 2-day-old and (E) 3-day-old larvae for each pressure level tested. Small filled circles represent individual data points, data points from the same batch are joined by lines. Larger open circles indicate the mean across all batches. (F) Vertical displacement of 3-day-old larvae acclimated for ca. 10 min to either ambient pressure (0 mb, left), 100 mb (centre), or 500 mb (right) prior to the experiment. Lines are coloured by set fractional increments in pressure. (G) Maximum increase in relative vertical displacement of 3-day-old larvae acclimated to 0, 100, or 500 mb. The data are fitted with a saturation curve. 5 (0, 100 mb) or 6 (500 mb) batches tested in F–G. (H) Maximum ciliary beat frequency (CBF) that single larvae reached in the 30 s prior (category Before), or during the first 30 s (Stimulus) of the indicated increase in pressure. N = 18–22 larvae. Data points for the same larva are joined by lines. One-tailed paired t-test with Bonferroni correction testing for an increase in CBF; p-values <0.05 are shown. Error bars in B, C, and F show the standard error of the mean. Data in D, E, and G were fitted with a third (D, E) or a second (G) order polynomial function. Figure 1—source data 1 (A, D), Figure 1—source data 2 (C, E), Figure 1—source data 3 (F, G), Figure 1—source data 4 (H).
-
Figure 1—source data 1
Swimming metrics of mixed batches of 2-day-old wildtype larvae subjected to randomized step increases in pressure.
- https://cdn.elifesciences.org/articles/94306/elife-94306-fig1-data1-v1.csv
-
Figure 1—source data 2
Swimming metrics of mixed batches of 3-day-old wildtype larvae subjected to randomized step increases in pressure.
- https://cdn.elifesciences.org/articles/94306/elife-94306-fig1-data2-v1.csv
-
Figure 1—source data 3
Swimming metrics of mixed batches of 3-day-old wildtype larvae subjected to randomized step increases in pressure after acclimatization to higher than ambient pressures for 10 min.
- https://cdn.elifesciences.org/articles/94306/elife-94306-fig1-data3-v1.csv
-
Figure 1—source data 4
Ciliary-band dynamics of individual 2-day-old wildtype or c-opsin-1 mutant larvae subjected to randomized step increases in pressure.
- https://cdn.elifesciences.org/articles/94306/elife-94306-fig1-data4-v1.csv
-
Figure 1—source data 5
Swimming metrics of 1-day-old-larvae subjected to randomized step increases in pressure.
- https://cdn.elifesciences.org/articles/94306/elife-94306-fig1-data5-v1.csv
-
Figure 1—source data 6
Swimming metrics of 3-day-old-larvae subjected to randomized step increases in pressure using a water column of different heights.
- https://cdn.elifesciences.org/articles/94306/elife-94306-fig1-data6-v1.csv
-
Figure 1—source data 7
Swimming metrics of 3-day-old-larvae subjected to randomized linear increases in pressure.
- https://cdn.elifesciences.org/articles/94306/elife-94306-fig1-data7-v1.csv
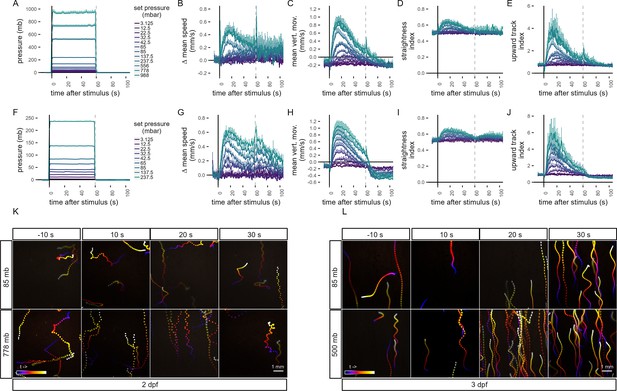
Quantification of swimming behaviour in Platynereis larvae in response to pressure.
Metrics of the pressure response in 2-day-old larvae (A–E) and 3-day-old larvae (F–J). Dashed line at 60 s in each plot indicates the end of stimulus. (A, F) Range of step increases in pressure applied to larvae. The step increases were applied in randomized order to each batch of larvae. (B, G) Change in mean speed relative to mean speed before stimulus. (C, H) Mean vertical movement. (D, I) Straightness index. The closer the value is to 1, the less tortuous the track is. (E, J) Upward track index. Defined as the number of upward tracks divided by the number of downward tracks. Colour-coded trajectories of 2-day-old (K, Video 2) and 3-day-old (L, Video 3) larvae prior and during a step increase in pressure. Trajectories to two different pressure levels are shown, a moderate (top row), and a high (bottom row) step increase. Each projection is 10 s long starting 10 s before stimulus (−10 s, left-most column) and ending in the 30 s after stimulus (30 s, right-most column). Each data point is the average of 2–12 (A–E) or 4–5 (F–J) batches. Error bars in A-J show the standard error of the mean. Colour code in B–E as in A and in G–J as in F. Figure 1—source data 1 (A–E), Figure 1—source data 2 (F–J).
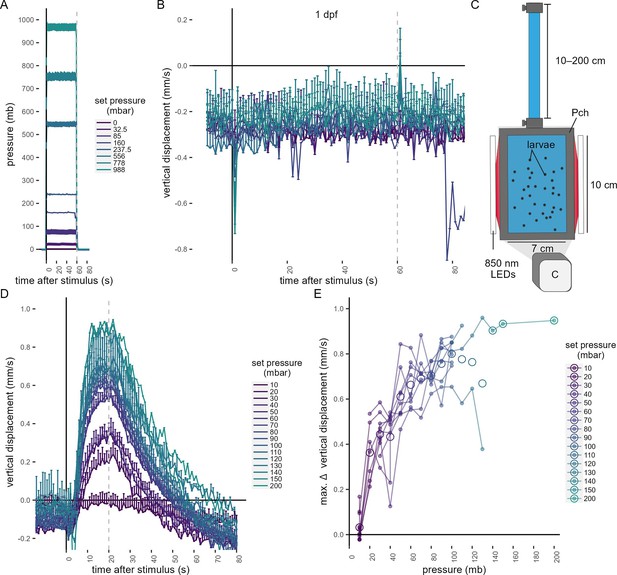
Swimming behaviour in 1-day-old-larvae to changes in pressure and in 3-day-old-larvae to the application of a hydrostatic pressure stimulus by a column of water.
(A, B) One-day-old Platynereis larvae do not respond to small increases in pressure. (A) Range of step increases in pressure applied to 1-day-old larvae. (B) Average vertical displacement of 1-day-old larvae relative to stimulus onset. Each data point is the average of 1–4 batches. (C–E) Three-day-old Platynereis larvae respond to small changes in hydrostatic pressure applied with a column of water. (C) Schematic of the behavioural setup used to stimulate larvae with a column of water. The height of the column was varied at random between 10 and 200 cm, in 10 cm increments. 1 mb ≈ 1 cm. (D) Vertical displacement as a function of pressure onset. Vertical dashed line at 20 s indicates end of stimulus. (E) Maximum increase in vertical displacement as a function of pressure. Each data point in D and E is the average of up to 12 batches. Error bars in A , B, and D show the standard error of the mean. Data in B colour-coded as in A. Figure 1—source data 5 (A, B), Figure 1—source data 6 (D, E).
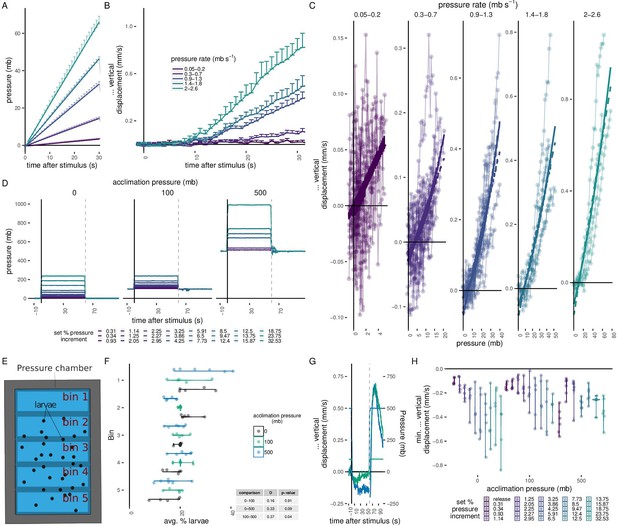
Quantification of swimming behaviour in 3-day-old larvae subjected to linear increases in pressure and to long-lasting periods of increased pressure.
(A–C) Response of 3-day-old larvae to a linear increase in pressure (N = 4–14 batches). (A) Average linear increase at five different rates (colour code in B) and the fitted curves for the time interval shown. (B) Mean change in vertical displacement at the five different rates of pressure increase shown in A as a function of time. (C) Maximum change in vertical displacement for each rate of pressure increase. Data fitted with either a linear (dashed line) or a second-degree polynomial function (solid line). (D) Average pressure changes applied for data shown in Figure 1F, G. (E, F) Average particle distribution (as a proxy of larval distribution) across the chamber (divided in 5 bins, as schematized in E) prior to pressure stimulus for each batch tested. (G) Mean change in vertical displacement upon release of pressure from 100 and 500 mb after testing the step increases in pressure shown in D. The mean pressure change applied is overlaid on the same plot. (H) Maximum decrease in relative vertical displacement after stimulus start for each set increment in pressure across the three acclimation conditions. The ‘release’ dataset consists of the trials shown in G. Dashed line at 60 s in D and G indicates the point of pressure release and increase, respectively. 5 (0, 100 mb) or 6 (500 mb) batches tested in D–H. Error bars in A, B, D and G show the standard error of the mean. Figure 1—source data 7 (A–C), Figure 1—source data 3 (D, F–H).
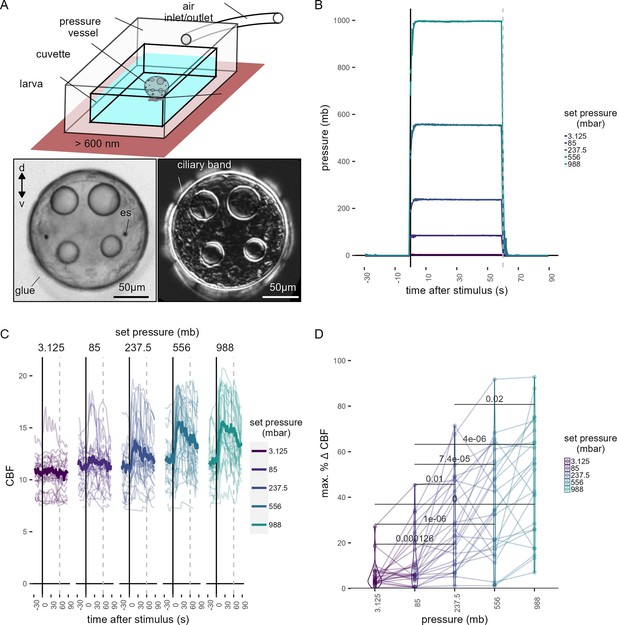
Ciliary dynamics assay.
(A, top) Schematic of the preparation used to quantify ciliary beat frequency (CBF). (A, bottom left) Snapshot of a glued 2-day-old larva in the pressure setup. (A, bottom right) Standard deviation projection of time-lapse recording to highlight ciliary band activity. (B) Average pressure levels measured during the experiments corresponding to data shown in Figure 1H. The step increases were applied in randomized order to each larva. Error bars show the standard error of the mean. (C) Maximum percentage change in CBF during the first 30 s of the stimulus period for each of the step increases in pressure tested. One-tailed unpaired t-test with Bonferroni correction. p-values <0.05 are shown. Data points from the same larva are joined by lines. N = 18–22 larvae. (D) Individual (thin traces) and mean (thick traces) CBF as a function of pressure level. Dashed line indicates end of pressure stimulus. Figure 1—source data 4 (B–D).
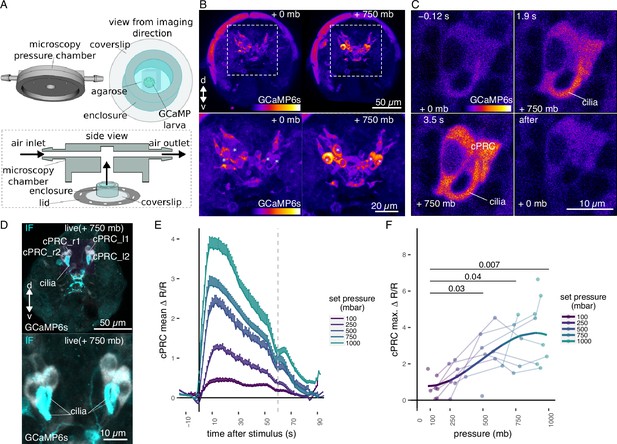
Ca2+ imaging of Platynereis larvae during pressure increases.
(A) Ca2+ imaging preparation to analyse neuronal activation upon pressure stimulation. Left: side view of the three-dimensional (3D) model of the microscopy pressure chamber. Right: larvae embedded in agarose are placed on a round coverslip. An enclosure around the embedded larva serves to keep it under water. Bottom: the enclosed larva on the coverslip is inserted in the central hole of the chamber. A screwable lid secures the coverslip to the chamber and prevents air leaks. Pressure is increased with compressed air entering from one of the inlets. (B, top) Maximum intensity projection of a Z-stack acquired before (left) or during (right) the pressure stimulus of a 2-day-old larva expressing GCaMP6s. (B, bottom) Enlarged views of the corresponding regions highlighted with dashed squares in the top panels. Asterisks mark the position of cell nuclei of the four cells activated by pressure. (C) Still images of a ciliary photoreceptor cell (cPRC) acquired at different time points relative to increase in pressure (t = 0, Video 5). The time points are indicated on the upper left of each panel. Pressure level is also indicated. (D) Maximum intensity projection of a GCaMP6s Z-stack during raised pressure (white channel) and of a Z-stack of the same larva after immunofluorescence (IF) with NIT-GC2, a marker for cPRC cilia (Jokura et al., 2023), and for serotonin (cyan channel). Anterior view in B–D. (E) Mean ∆R/R in cPRC_l1 across different step increases in pressure as a function of time of stimulation. Dashed line at 60 s marks the end of stimulus. N = 8 larvae. Error bars show the standard error of the mean. (F) Max. ∆R/R in cPRC_l1 as a function of pressure level. Data points from the same larva are joined by lines. Regression line fitting the data is also shown. One-tailed unpaired t-test with Bonferroni correction testing for an increase in Max. ∆R/R with pressure. p-values <0.05 are shown. Figure 2—source data 1 (E, F).
-
Figure 2—source data 1
Raw intensity values of GCaMP6s (GC) and tdTomato (Tom) channels in cPRCs of 2-day-old larvae subjected to randomized step increases in pressure.
dF and dR metrics calculated from raw values are also included.
- https://cdn.elifesciences.org/articles/94306/elife-94306-fig2-data1-v1.csv
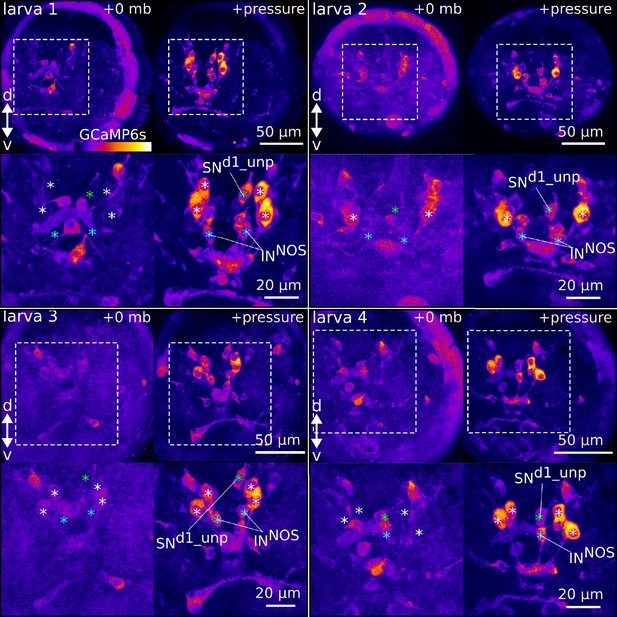
Ca2+ imaging during pressure increase revealed cells activated during pressure.
Maximum intensity projections of 2-day-old larvae expressing GCaMP6s. Four larvae are shown. (Top panels for each larva) Projections acquired before (+0 mb) or during (+pressure) presentation of the stimulus. (Bottom panels for each larva) Close-up views of the regions highlighted with dashed squares in the top panels. White asteriks mark the position of cell nuclei of the four sensory cells activated by pressure (ciliary photoreceptor cells, cPRCs). Blue asterisk marks the position of the assymetric sensory cell SNd1_unp. Green asterisks mark the position of the putative NOS-expressing interneurons (INNOS). Pressure >500 mb in all samples shown.
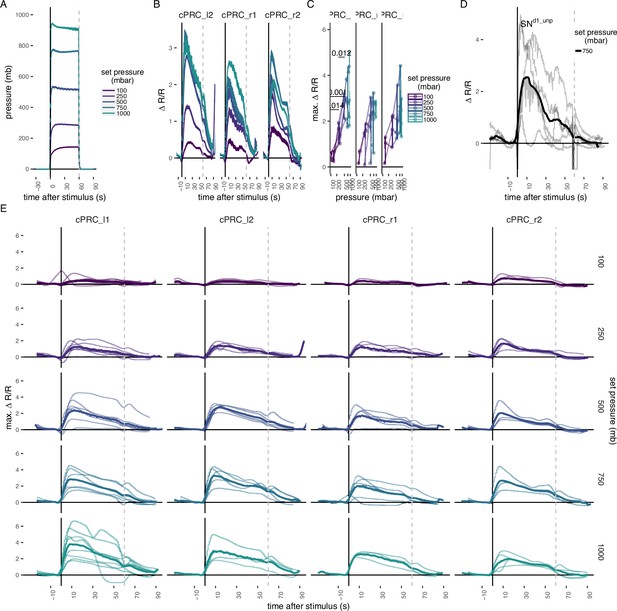
Ca2+ imaging in ciliary photoreceptor cells (cPRCs) and in SNd1_unp.
(A) Step pressure increases applied to 2-day-old larvae in the Ca2+ imaging pressure setup (see Figure 2A). The step increases were applied to each larva in a randomized order. (B) Mean ∆R/R in cPRC_r1, cPRC_l2, and cPRC_r2 as a function of time relative to pressure increase (t = 0). (C) Max. ∆R/R in in cPRC_r1, cPRC_l2, and cPRC_r2 as a function of pressure level. One-tailed unpaired t-test with Bonferroni correction testing for an increase in Max. ∆R/R with pressure. p-values <0.05 are shown. (D) ∆R/R in SNd1_unp upon 750 mb pressure increase. Thick line is the mean of individual measurements (thinner lines). (E) Individual ∆R/R measurements (thin lines) in the four cPRCs across different steps of pressure increase as a function of time of stimulation. Thicker line is the mean ∆R/R for each pressure level and cell. Dashed lines at 60 s in B–D mark the end of stimulus. N = 2–4 (cPRC_r1), 4–5 (cPRC_l2), 4 (cPRC_r2), 4 (SNd1_unp) cells in B–D. Error bars in A and B show the standard error of the mean. Figure 2—source data 1 (A–E).
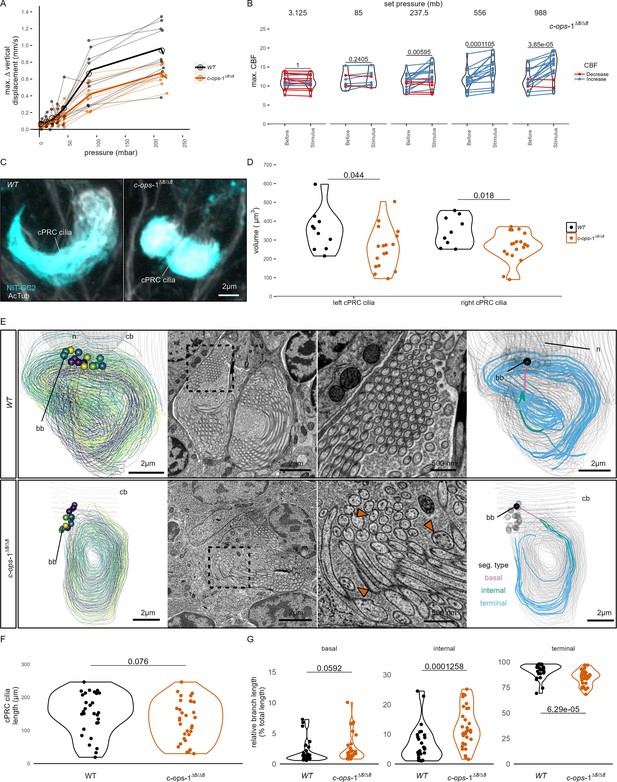
c-ops-1∆8/∆8 larvae show a weaker response to pressure and have structural defects in ciliary photoreceptor cell (cPRC) cilia.
(A) Maximum change in vertical displacement of wild-type (WT) and c-ops-1∆8/∆8 3-day-old larvae for each pressure step-change tested. Data points from the same batch are joined by lines. Larger open circles indicate the mean value. Thicker solid lines show the regression model predictions. N = 7–9 (c-ops-1∆8/∆8), N = 8–10 (WT) batches. (B) Max. ciliary beat frequency (CBF) that individual c-ops-1∆8/∆8 larvae reached in the 30 s prior (Before), or during the first 30 s of the indicated increase in pressure (Stimulus). Data points for the same larva are joined by lines. One-tailed paired t-test with Bonferroni correction testing for an increase in CBF; all p-values are shown. N = 10–17 larvae. (C, D) cPRC ciliary volume measured for each pair of cPRC cilia on the left and right body sides in 2-day-old WT and c-ops-1∆8/∆8 larvae. Volumes were measured using the signal of the NIT-GC2 antibody (α-NIT-GC2). (C) Maximum intensity projections of IF stainings used for quantifying cPRC cilary volume. (D) cPRC ciliary volume distribution sorted by genotype and body side. One-tailed unpaired t-test with Bonferroni correction testing for a decrease in ciliary volume in mutant larvae. p-values <0.05 are shown. N = 9–10 (WT), 17–19 (c-ops-1∆8/∆8) larvae. (E) Morphology of cPRC cilia in 3-day-old WT (top row) and c-ops-1∆8/∆8 (bottom row) larvae reconstructed by serial-section electron microscopy (ssEM). Reconstructions of cPRC cilia are shown in the left-most panels. A representative micrograph of the ssEM data used for the reconstructions is shown in the adjacent panels. Dashed squares in these images mark the regions shown in the enlarged views to the right. Orange arrowheads point to cilia with more than one microtubule dublets. In the right-most panels, the branches of single cPRC cilia are coloured by its position relative to the basal body (bb): basal, internal, or terminal branches. The remaining cPRC cilia are coloured in grey. cb: cell body; n: nucleus. (F) Length of cPRC cilia of WT (N = 29 cilia) and c-ops-1∆8/∆8 (N = 32 cilia) larvae measured from ssEM volume reconstructions. Unpaired Wilcoxon test for larger branches in the WT larva: p = 0.076. (G) Length distribution of basal, internal and terminal branches for each genotype shown as a percentage of the total ciliary arbor length. One-tailed unpaired Wilcoxon test with Bonferroni correction for larger basal, internal, and terminal branches in the c-ops-1∆8/∆8 mutant: p = 0.0592, p = 1.26E-4, p = 6.29E-5, respectively. Figure 3—source data 1 (A), Figure 1—source data 4 (B), Figure 3—source data 2 (D).
-
Figure 3—source data 1
Swimming metrics of individual batches of 3-day-old wildtype or c-opsin-1 mutant larvae subjected to randomized step increases in pressure.
- https://cdn.elifesciences.org/articles/94306/elife-94306-fig3-data1-v1.csv
-
Figure 3—source data 2
Volume of ciliated structure of individual cPRCs of wildtype or c-opsin-1 mutant 2-day-old larvae measured by quantifying the NIT-GC2 antibody signal.
- https://cdn.elifesciences.org/articles/94306/elife-94306-fig3-data2-v1.csv
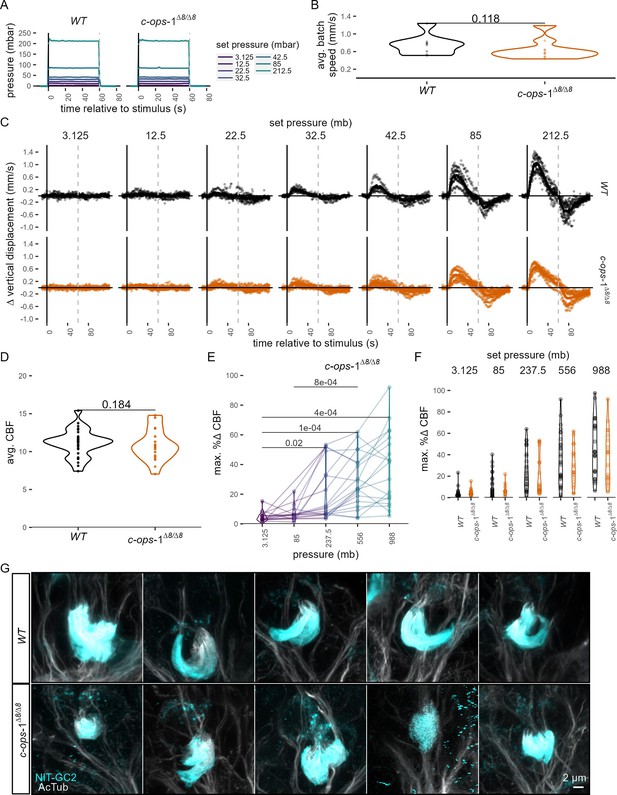
Response of 3-day-old c-ops-1∆8/∆8 larvae to pressure.
(A) Mean pressure increase steps applied to 3-day-old wild-type (WT) or c-ops-1∆8/∆8 larvae. (B) Average swimming speed per batch of WT or c-ops-1∆8/∆8 larvae in the 10 s prior to pressure increase. One-tailed unpaired Wilcoxon test for a decrease in swimming speed in mutant larvae: p = 0.188. (C) Change in mean vertical displacement as a function of time of stimulation for each genotype and step increase in pressure. Individual data points are also plotted. Dashed lines at 60 s mark the end of stimulus. N = 7–9 (c-ops-1∆8/∆8) batches, N = 8–10 (WT) batches in A–C. (D) Mean ciliary beat frequency (CBF) for 2-day-old WT or c-ops-1∆8/∆8 larvae in the 30 s prior to stimulus onset. N = 29 (WT), 19 (c-ops-1∆8/∆8) larvae. One-tailed unpaired t-test testing for a decrease in CBF in mutant larvae: p = 0.786. (E) Maximum % change in CBF (% ∆CBF) upon pressure increase in 2-day-old c-ops-1∆8/∆8 larvae. One-tailed unpaired t-test with Bonferroni correction. p-values <0.05 are shown. N = 10–17 larvae. (F) Maximum percentage change in CBF during the first 30 s of the stimulus period in WT (N = 18–22 larvae) and in c-ops-1∆8/∆8 (N = 10–17 larvae) 2-day-old larvae. One-tailed unpaired t-test with Bonferroni correction (G) Max. intensity projections of ciliary photoreceptor cell (cPRC) cilia of 2-day-old WT and c-ops-1∆8/∆8 larvae used for quantifiying cPRC ciliary volume (see Figure 3D). Figure 3—source data 1 (A–C), Figure 1—source data 4 (D–F).
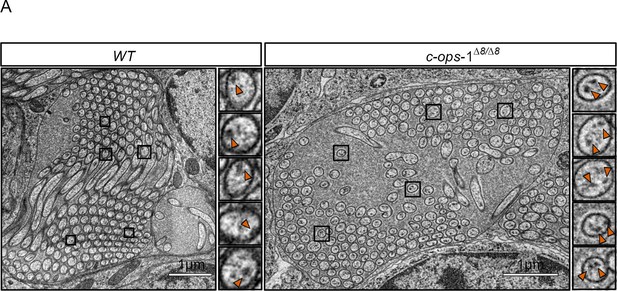
Morphology of ciliary photoreceptor cell (cPRC) cilia in 3-day-old c-ops-1∆8/∆8 larvae.
(A) Ultrastucture of cPRC cilia in a wild type and a c-ops-1∆8/∆8 mutant larva. Rectangles highlight branches with two pairs of microtubule doublets shown in enlarged views on the right.
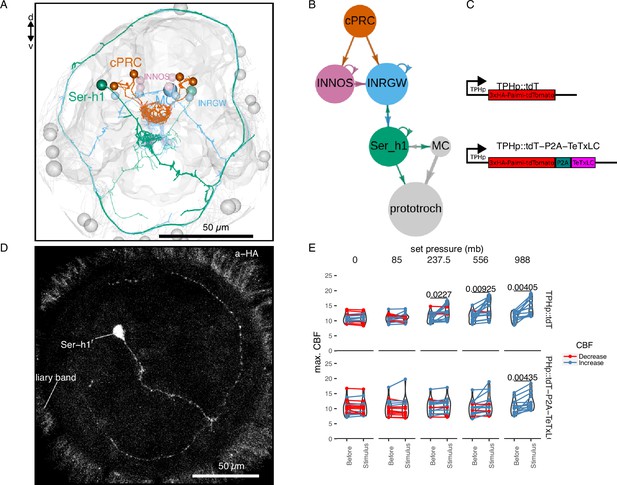
TeTxLC expression in Ser-h1 neurons inhibits ciliary beat frequency (CBF) increase during pressure.
Volume EM reconstruction (A) of the cells in the ciliary photoreceptor cell (cPRC) synaptic circuit (B). cPRCs synpase in the middle of the brain on both INNOS and INRGWa neurons. INRGWa cells synapse on Ser-h1 neurons, which directly innervate the ciliary band and also target the MC cell. Only the cells in the shortest path to the prototroch are included. (C) Schematic of the gene constructs used to test the role of Ser-h1 in the pressure response. The TPHp::tdT construct drives expression of the reporter protein Palmi-tdTomato in Ser-h1 and other serotonergic neurons. It was used as a control. The TPHp::tdT-P2A-TeTxLC construct expresses Palmi-tdTomato and the synaptic blocker TeTxLC as a fusion that gets post-translationally self-cleaved by the P2A peptide. (D) Maximum intensity projections of a larva injected with the TPHp::tdT-P2A-TeTxLC construct and stained with α-HA. Ser-h1 was labelled in this animal. Anterior view. (E) Max. CBF that single larvae reached in the 30 s prior (Before), or during the first 30 s of the indicated increase in pressure (Stimulus). Data points for the same larva are joined by lines. Larvae were injected either with the control plasmid TPHp::tdT (top row, N = 14–16 larvae), or with the TPHp::tdT-P2A-TeTxLC plasmid (bottom row, N = 13–16 larvae). One-tailed paired t-test with Bonferroni correction testing for an increase in CBF; p-values <0.05 are shown. Figure 4—source data 1 (E).
-
Figure 4—source data 1
Ciliary band dynamics of individual 2-day-old wildtype larvae injected with pLB316 or with pLB253 plasmid constructs and subjected to randomized step increases in pressure.
- https://cdn.elifesciences.org/articles/94306/elife-94306-fig4-data1-v1.csv
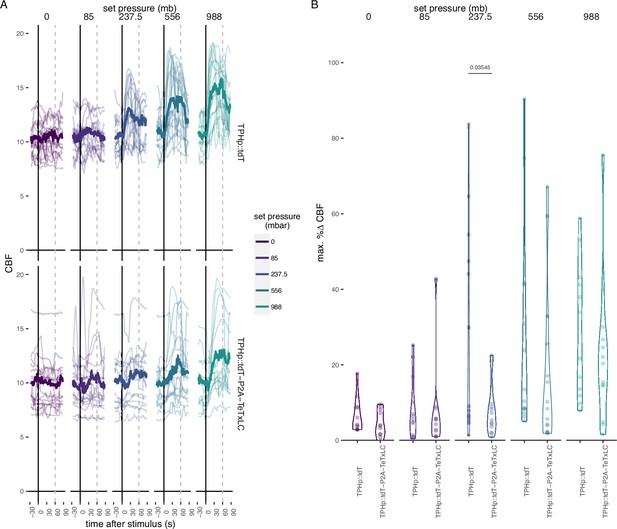
Effect of TeTxLC-mediated inhibition of serotonergic ciliomotor neurons on ciliary beat frequency (CBF) during pressure stimulation.
(A) Maximum percentage change in CBF during the first 30 s of the stimulus period in 2-day-old larvae injected with the control plasmid TPHp::tdT (N = 14–16 larvae), or with the TPHp::tdT-P2A-TeTxLC plasmid (N = 13–16 larvae). One-tailed unpaired t-test with Bonferroni correction. (B) Individual (thin traces) and mean (thick traces) CBF as a function of pressure level for larvae injected with the indicated plasmid construct. Dashed line indicates end of pressure stimulus. Figure 4—source data 1 (A, B).
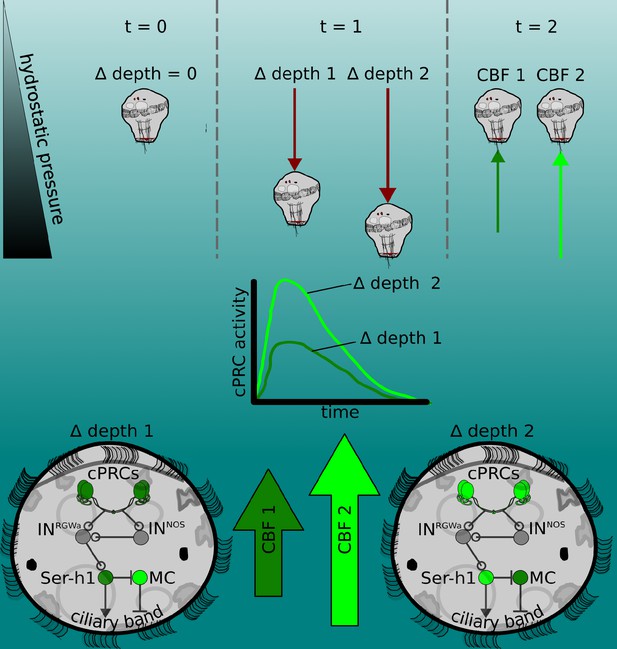
The pressure response as a depth-retention mechanism mediated by the ciliary photoreceptor-cell circuit.
(Top) Platynereis larvae maintain their position in the water column by controlling the beating dynamics of the ciliary band (t = 0, no net change in depth, ∆ depth = 0). A sufficiently rapid increase in depth (at t = 1) caused by intrinsic or extrinsic factors would lead to an increase in hydrostatic pressure. The change in depth relative to the previous state (∆ depth 1 or ∆ depth 2) will be perceived by the larva, which will try to counteract this change by increasing ciliary beat frequency (CBF) of the prototroch, leading to upward swimming (at t = 2) until the pressure returns to the level previously experienced. A smaller change in pressure (∆ depth 1) will lead to a smaller increase in CBF (CBF 1, light green arrow) than a larger change (CBF 2). (Middle) Changes in pressure are sensed by the ciliary photoreceptor cells (cPRCs). These sensory cells are activated in a graded manner according to the change in pressure. (Bottom) cPRCs signal via a postsynaptic circuit including the INRGWa and INNOS interneurons to the Ser-h1 neurons. Activation of these serotonergic neurons is required to increase ciliary beating directly on the prototroch cells and indirectly by inhibiting the MC neuron (CBF 1 or CBF2) proportional to the change in pressure.
Videos
Response of 2-day-old Platynereis larvae to a pressure increase of 1 bar.
Larvae were placed in a pressure vessel (100 mm height) and recorded in effective darkness (with 850 nm light) with a camera placed in front of the vessel (see Figure 1A). Larvae swim towards the top of the chamber as soon as pressure is increased. Blue traces (10 s long) delineate swimming trajectories.
Zoomed-in view of 2-day-old larvae as they respond to an increase in pressure of 85 mb (left panel) or 500 mb.
Blue traces (each 4 s long) delineate swimming trajectories. The two videos are played at the same speed.
Zoomed-in view of 3-day-old larvae as they respond to an increase in pressure of 32 mb (left panel) or 778 mb.
Blue traces (each 4 s long) delineate swimming trajectories. The two videos are played at the same speed.
Representative recording of a 2-day-old larva tethered to a glass cuvette and imaged from the anterior side to quantify the effect of pressure on the ciliary dynamics.
Note how ciliary beating speeds up soon after the step change in pressure (998 mb).
Time-lapse confocal microscopy recording showing the change in GCaMP6s fluorescence (fire colour scale) in a ciliary photoreceptor cell (cPRC) in response to pressure increase (750 mb).
The increase in GCaMP6s signal is noticeable both in the cell body (cb) and in the cilia. Some frames are shifted in XY due to sample movement.
360° view of electron microscopy reconstruction of sensory cilia from one pair of ciliary photoreceptor cells in a wild-type 3-day-old larva.
The branches of each cilium are coloured according to their position along the cilium: basal (pink), intermediate (green), or light blue (terminal). Basal bodies are shown as black spheres. The cell and nucleus of one of the cells are outlined (previously reconstructed in Verasztó et al., 2018).
360° view of electron microscopy reconstruction of sensory cilia from one pair of ciliary photoreceptor cells in a c-ops-1∆8/∆8 3-day-old larva.
The branches of each cilium are coloured according to their position along the cilium: basal (pink), intermediate (green), or light blue (terminal). Basal bodies are shown as black spheres. The part of the cell body imaged in the volume is outlined.
Tables
Reagent type (species) or resource | Designation | Source or reference | Identifiers | Additional information |
---|---|---|---|---|
Strain, strain background (Platynereis dumerilii) | Wild-type | Marine Invertebrate Culture Unit, University of Exeter | NCBITaxon:6359 | |
Strain, strain background (P. dumerilii) | c-opsin1Δ8/Δ8 knockout | Verasztó et al., 2018 | NCBITaxon:6359 | Knockout generated by TALEN-induced gene editing |
Transfected construct (mRNA) | GCaMP6s mRNA | Randel et al., 2014 | 1 mg/ml | |
Transfected construct (mRNA) | 3xHA-Palmi-tdTomato mRNA | This paper | <0.2 ng/µl | |
Antibody | Monoclonal Anti-Tubulin, Acetylated antibody produced in mouse | Sigma-Aldrich | Cat# T6793, RRID:AB_477585 | (1:250) |
Antibody | Polyclonal NIT-GC2 antibody, produced in rabbit | Jokura et al., 2023 | aNIT-GC2 | 5 mg/ml |
Antibody | anti-5-HT, produced in rabit | ImmunoStar | Cat# 20080, RRID:AB_572263 | 2 mg/ml |
Antibody | Monoclonal Anti-HA antibody produced in mouse | Cell Signaling Technology | HA-Tag (6E2), Cat# 2367, RRID:AB_10691311 | (1:250) |
Antibody | Monoclonal Anti-HA antibody produced in rabbit | Cell Signaling Technology | HA-Tag (C29F4) Cat #3724, RRID:AB_1549585 | (1:250) |
Antibody | Goat anti-Rabbit IgG (H+L) Cross-Adsorbed Secondary Antibody, Alexa Fluor 488 produced in rabbit | Thermo Fisher Scientific | Cat# A-11008, RRID:AB_143165 | (1:250) |
Antibody | F(ab’)2-Goat anti-Mouse IgG (H+L) Cross-Adsorbed Secondary Antibody, Alexa Fluor 546 | Thermo Fisher Scientific | Cat# A-11018, RRID:AB_2534085 | (1:250) |
Commercial assay or kit | mMESSAGEmMACHINE T7ULTRA Transcription Kit | Ambion, Thermo Fisher Scientific | Cat# AM1345 | |
Recombinant DNA reagent | pUC57-TPHp3xHA-Palmi-tdTomato-P2A-TeTxLC (plasmid) | This paper | pLB316 | Injected at 250 ng/µl in water |
Recombinant DNA reagent | pUC57-TPHp3xHA-Palmi-tdTomato (plasmid) | Verasztó et al., 2017 | pLB253 | Injected at 250 ng/µl in water |
Recombinant DNA reagent | pUC57-T7-RPP2-3xHA-Palmi-tdTomato (plasmid) | This paper | pLB260 | Injected at 250 ng/µl in water |
Recombinant DNA reagent | pUC57-T7-RPP2-GCaMP6s (plasmid) | Randel et al., 2014 | pLB112 | Injected at 250 ng/µl in water |
Recombinant DNA reagent | pGEMTEZ-TeTxLC | Yu et al., 2004 | Addgene plasmid # 32640, RRID:Addgene_32640 | Richard Axel & Joseph Gogos & C. Ron Yu |
Chemical compound, drug | Ficoll PM70 | Sigma-Aldrich | Cat# F2878 | −20% |
Chemical compound, drug | Formaldehyde Aqueous Solution (Paraformaldehyde Aqueous Solution) EM Grade | Electron Microscopy Sciences | Cat# 15710 | −4% |
Chemical compound, drug | Low-melting agarose | Hampton Research | LM AgaroseTM, Cat# HR8-092 | (2.5–3%) |
Software, algorithm | Fiji | NIH | RRID:SCR_002285 | |
Software, algorithm | CATMAID | Saalfeld et al., 2009 | RRID:SCR_006278 | |
Other | Wormglu | GluStitch Inc |
Swimming metrics.
Metric | Formula |
---|---|
Average vertical displacement | |
Vertical movement | |
Average speed | |
Average straightness vertical path | |
Straightness index |