The co-receptor Tetraspanin12 directly captures Norrin to promote ligand-specific β-catenin signaling
Figures
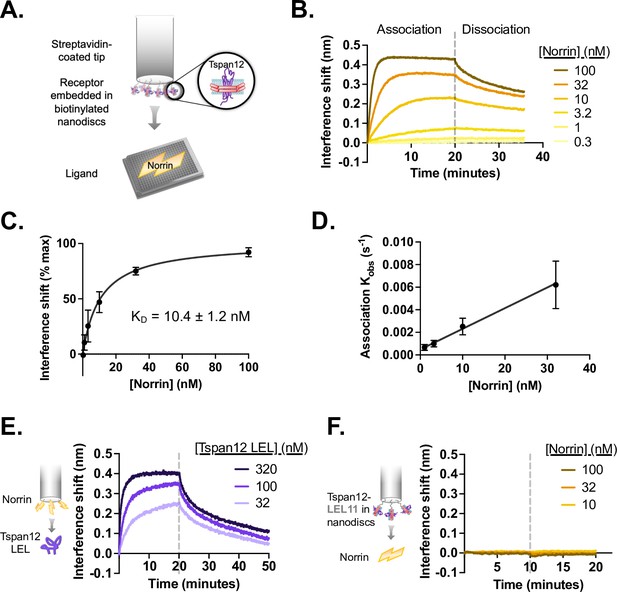
Tspan12 binds directly to Norrin with nanomolar affinity via the large extracellular loop (LEL).
(A) Schematic of biolayer interferometry (BLI) setup for Tspan12-Norrin binding: Tspan12 lacking the C-terminal tail (∆C), inserted into biotinylated nanodiscs, is immobilized on a streptavidin-coated biosensor, and Norrin association and dissociation are monitored in real time. (B) BLI traces of Norrin at indicated concentrations binding to and dissociating from Tspan12. (C) Steady-state binding curve fit to Norrin-Tspan12 binding (mean ± SD from three independent replicates at each concentration of Norrin) gives a KD of 10.4±1.2 nM (mean ± SEM). (D) Observed association rate constant (Kobs), determined from fitting BLI association traces (mean ± SD in three independent experiments), is linearly dependent on Norrin concentration with a slope Kon = 0.00019 ± 0.00003 nM –1 s–1 (mean ± SEM). When combined with the Koff = 0.0014 ± 0.00016 s–1 (mean ± SEM) determined from fitting the dissociation traces, we obtain a kinetic KD of 7.4±1.4 nM (mean ± SEM). (E) BLI traces of the soluble MBP-tagged Tspan12 LEL domain, at the indicated concentrations, associating to and dissociating from a biosensor loaded with MBP-tagged Norrin. Kinetic fitting gives an apparent affinity of 16±3 nM (mean ± SEM). (F) BLI traces of 10, 32, or 100 nM Norrin show no binding to a biosensor loaded with a nanodisc-embedded chimeric Tspan12 with the LEL replaced by that of Tspan11.
-
Figure 1—source data 1
Steady-state interference shift and Kobs values used to generate Figure 1C and D.
- https://cdn.elifesciences.org/articles/96743/elife-96743-fig1-data1-v1.xlsx
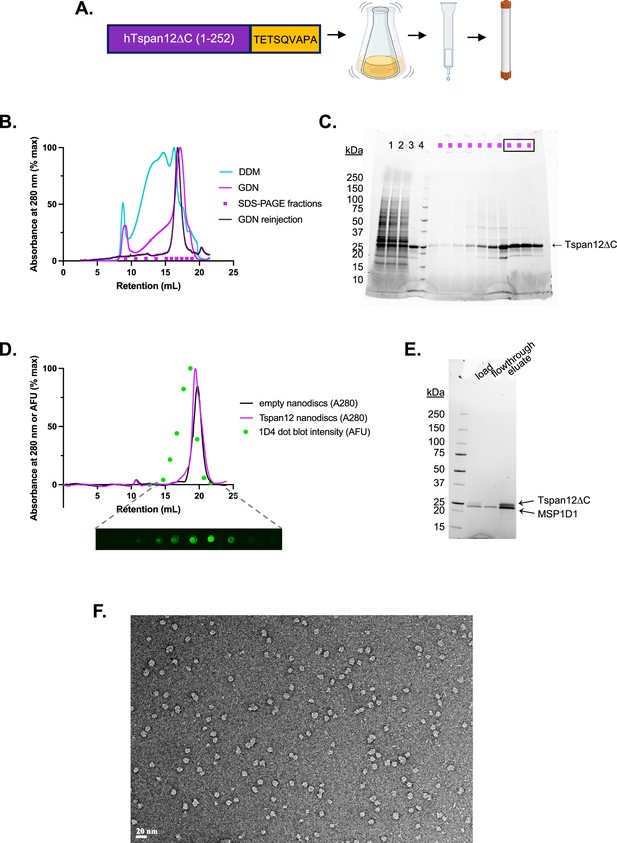
Tspan12 purification.
(A) Schematic of Tspan12 purification. Human Tspan12∆C (truncated at residue 252 after the fourth transmembrane domain) was tagged with the Rho1D4 tag TETSQVAPA and expressed in Sf9 cells by baculovirus. Detergent-solubilized Tspan12 was affinity purified on anti-Rho1D4 antibody resin followed by size exclusion chromatography. Panel A was created with BioRender.com. (B) Superose 6 10/300 size exclusion traces of Tspan12 purified in DDM (teal) or exchanged into glyco-diosgenin (GDN) during the affinity chromatography step (pink); the final concentrated product in GDN showed no signs of aggregation (purple). Indicated fractions of GDN-solubilized Tspan12 (pink trace) were run on (C) SDS-PAGE; boxed fractions were pooled and concentrated. (D) Size exclusion traces of empty nanodiscs (black) or Tspan12 reconstituted into excess MSP1D1 nanodiscs (pink). Tspan12 content of fractions was quantified by dot blot (anti-Rho1D4) and dot intensity was plotted accordingly (green). (E) Peak Tspan12-containing fractions from (D) were affinity purified on anti-Rho1D4 resin. Shown is an SDS-PAGE gel of the load, flowthrough, and eluate. (F) Uranyl acetate negative stain micrograph of final nanodisc-reconstituted Tspan12. Scale bar is 20 nm.
-
Figure 1—figure supplement 1—source data 1
Original files of gels in Figure 1—figure supplement 1.
- https://cdn.elifesciences.org/articles/96743/elife-96743-fig1-figsupp1-data1-v1.zip
-
Figure 1—figure supplement 1—source data 2
Labeled gels in Figure 1—figure supplement 1.
- https://cdn.elifesciences.org/articles/96743/elife-96743-fig1-figsupp1-data2-v1.zip
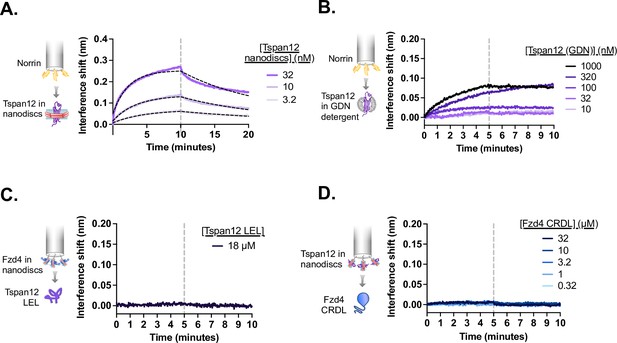
Tspan12 and Fzd4 each bind Norrin, but not one another, with high affinity through their extracellular domains.
(A) Biolayer interferometry (BLI) traces showing non-biotinylated Tspan12 nanodiscs in solution binding to biosensor-immobilized biotinylated MBP-Norrin (flipped setup compared to Figure 1A and B). Overlaid fits (black dashed line) give an apparent affinity of 34±11 nM. (B) BLI traces showing weak binding of indicated concentrations of Tspan12 in glyco-diosgenin (GDN) detergent to biosensor-immobilized biotinylated MBP-Norrin. (C) BLI trace showing no binding of MBP-tagged Tspan12 large extracellular loop (LEL) at 18 µM to biosensors loaded with nanodisc-embedded Fzd4. (D) BLI traces showing no binding of the soluble Fzd4 CRDL up to 32 µM to biosensors loaded with nanodisc-embedded Tspan12.
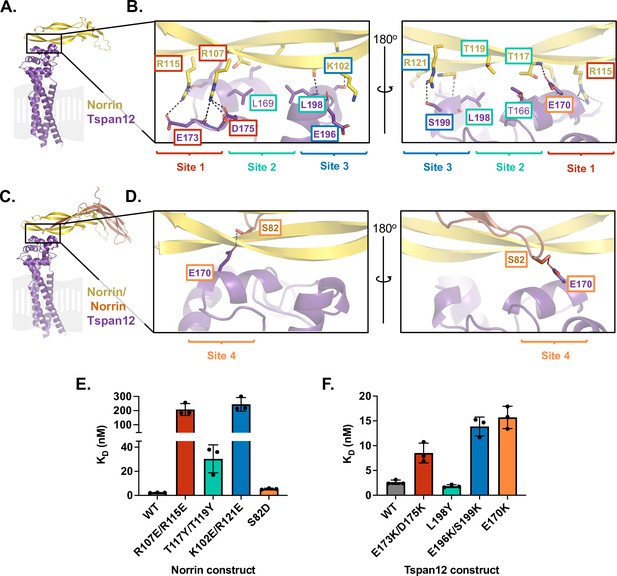
Mutational analysis of AlphaFold-predicted Tspan12-Norrin binding site.
(A) AlphaFold model of one Norrin protomer (yellow) bound to Tspan12 (purple), with the expected location of the plasma membrane shown in gray. (B) Zoomed view of the predicted Tspan12/Norrin binding site, front and rear view (flipped 180°). Predicted polar interactions are indicated with dark gray dashed lines. Within the binding interface, Site 1 (red), Site 2 (teal), and Site 3 (blue) are indicated. Bold residue labels indicate residues mutated for binding assays. (C) AlphaFold model of Tspan12 bound to Norrin dimer and (D) zoomed view of indicated area, showing the predicted polar interaction between residue S82 on the second Norrin protomer (orange) and residue E170 on Tspan12, termed Site 4 (orange). (E) Binding affinities (mean ± SD) for the indicated Norrin mutants binding to full-length wild-type (WT) Tspan12 and (F) WT Norrin binding to the indicated Tspan12 mutants, calculated from association and dissociation fits to biolayer interferometry (BLI) traces of 32 nM Norrin binding to Tspan12 in triplicate (see Figure 2—figure supplement 3A and B). Colors correspond to sites within the binding interface. Kinetic traces and kinetic constants are shown in Figure 2—figure supplement 3, and affinities and kinetic constants are reported in Supplementary file 1.
-
Figure 2—source data 1
Norrin-Tspan12 mutant affinities used to generate Figure 2E and F.
- https://cdn.elifesciences.org/articles/96743/elife-96743-fig2-data1-v1.xlsx
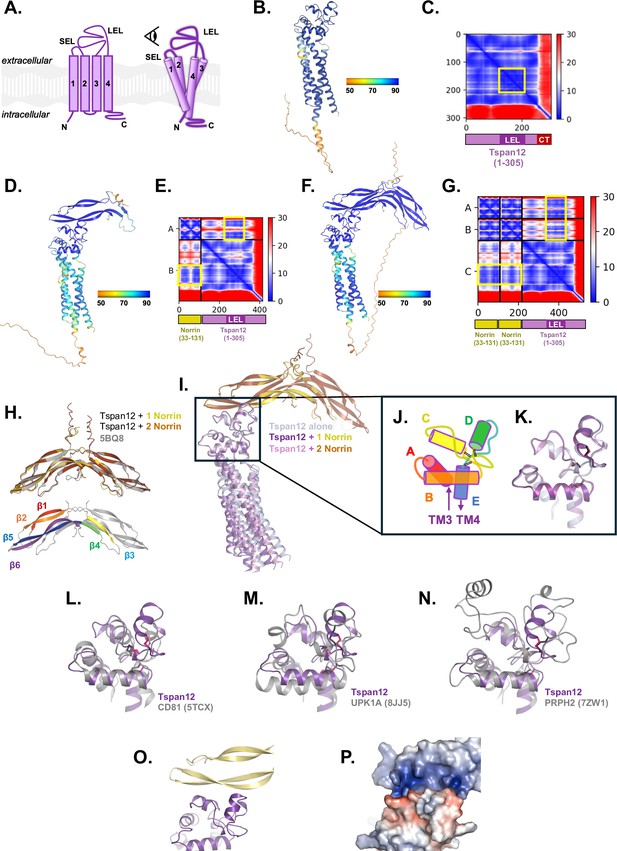
AlphaFold structural prediction of Tspan12 bound to Norrin.
(A) Cartoon of tetraspanin structure, comprised of transmembrane helices 1–4, a small extracellular loop (SEL, between helices 1 and 2) and a large extracellular loop (LEL, between helices 3 and 4). Eye icon indicates the viewing angle of AlphaFold models in the remainder of this figure (B, D, F, I–P) relative to this cartoon. (B) The structure of full-length human Tspan12 alone was predicted with AlphaFold. The best-scoring model is shown, colored by the per-residue predicted local distance difference test (pLDDT) confidence metric. (C) The predicted aligned error (PAE) for the model in B. The position of the LEL (darker purple) appears as a darker blue square in the heat map with low PAE (yellow box). The position of C-terminal residues (‘CT‘, red) is indicated; the heat map shows high PAE values for the C-terminus relative to the rest of the protein, indicating poor prediction of the relative positioning of the C-terminus. (D) The structure of full-length human Tspan12 together with one Norrin protomer (residues 25–133) was predicted with AlphaFold-Multimer and the best-scoring model is shown, colored by pLDDT. (E) The predicted aligned error for the model shown in D. The position of Norrin, Tspan12, and the LEL along the axes are indicated. Note low PAE between the LEL and parts of Norrin (yellow boxes). (F) The structure of full-length human Tspan12 together with two copies of Norrin (residues 25–133) was predicted with AlphaFold-Multimer and the best-scoring model is shown, colored by pLDDT. (G) The predicted aligned error for the model shown in F. The position of both copies of Norrin, Tspan12, and the LEL along the axes are indicated. Note low PAE between the LEL and parts of both Norrin protomers (yellow boxes). (H) Top: The structure of Norrin within the predicted structure of Tspan12+1 Norrin protomer (yellow), and within the predicted structure of Tspan12+2 Norrin protomers (orange), matches the crystal structure of Norrin (5BQ8 chains A and B; gray) with RMSDs of 0.383 and 0.413 Å respectively. Below: β strands 1, 2, 3, 4, 5, and 6 of one Norrin protomer, colored red, orange, yellow, green, blue, and purple, respectively; strands 5 and 6 are predicted to comprise the Tspan12 binding site. (I) The predicted model of Tspan12 alone (light blue) is similar to the predicted models with one (purple/yellow) or two (pink/orange) copies of Norrin, which each align with RMSDs of 1.247 and 1.057 Å, respectively, to the model of Tspan12 alone. Aligning only the LELs as shown gives RMSDs of 0.293 and 0.341 Å, respectively, and illustrates the slight variation in the predicted angle between the TMs and the LEL. The predicted position and orientation of Norrin relative to the LEL is unchanged between the one-protomer and two-protomer models. (J) Tetraspanin LELs are composed of helices A, B, C, D, and E. Helices C and D are the least conserved and are implicated in binding partner interactions (Susa et al., 2024). Black double lines represent disulfide bridges. Viewing angle is indicated by the eye in A. (K) Close-up of aligned LELs from I. (L) The predicted structure of the Tspan12 LEL (from the 1 Tspan12 : 1 Norrin protomer model; residues 123–215; purple) aligns to the experimentally determined structure of CD81 LEL (5TCX residues 123–198; gray), with an RMSD of 3.997 Å. CD81 is a C4 tetraspanin; disulfide bonds for Tspan12 and CD81 are shown in pink and black, respectively. (M) The predicted Tspan12 LEL (purple) aligns to the Uroplakin 1A LEL (8JJ5, residues 125–226; gray), a C6 tetraspanin, with an RMSD of 4.215 Å. (N) The predicted Tspan12 LEL (purple) aligns to the Peripherin-2 LEL (7ZW1, residues 126–258; gray), a C6 tetraspanin, with an RMSD of 2.528 Å. (O) The predicted interaction between Norrin and Tspan12 involves helices C and D of the LEL and residues on β5 and β6 of Norrin. (P) The predicted interaction between Norrin and Tspan12 colored by surface electrostatics (APBS) shows a highly polar interaction involving a basic patch on Norrin and an acidic patch on Tspan12.
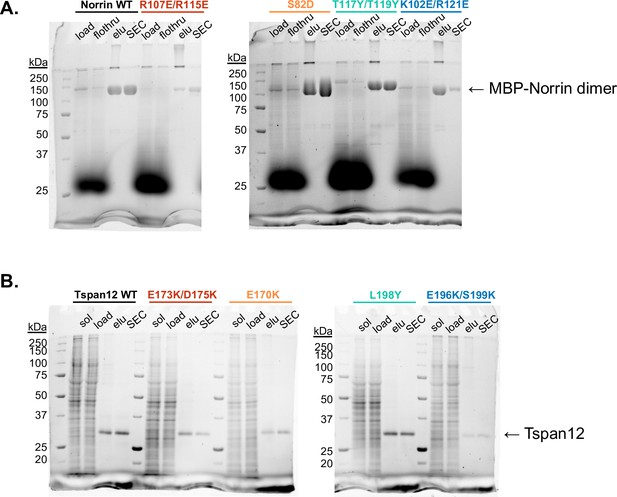
Purification of Norrin and Tspan12 mutants.
(A) Stain-free non-reducing SDS-PAGE gels of Sf9 supernatant media (‘load’), amylose resin flowthrough (‘flothru’), amylose eluate (‘elu’), and pooled fractions after size exclusion (‘SEC’) for wild-type (WT) and indicated mutant MBP-Norrin. (B) Stain-free SDS-PAGE gels of DDM-solubilized Expi293 membranes (‘sol’), supernatant post-ultracentrifugation (‘load’), 1D4 antibody resin eluate (‘elu’), and pooled fractions after size exclusion (‘SEC’) for full-length WT and indicated mutant Tspan12.
-
Figure 2—figure supplement 2—source data 1
Original files of gels in Figure 2—figure supplement 2.
- https://cdn.elifesciences.org/articles/96743/elife-96743-fig2-figsupp2-data1-v1.zip
-
Figure 2—figure supplement 2—source data 2
Labeled gels in Figure 2—figure supplement 2.
- https://cdn.elifesciences.org/articles/96743/elife-96743-fig2-figsupp2-data2-v1.zip
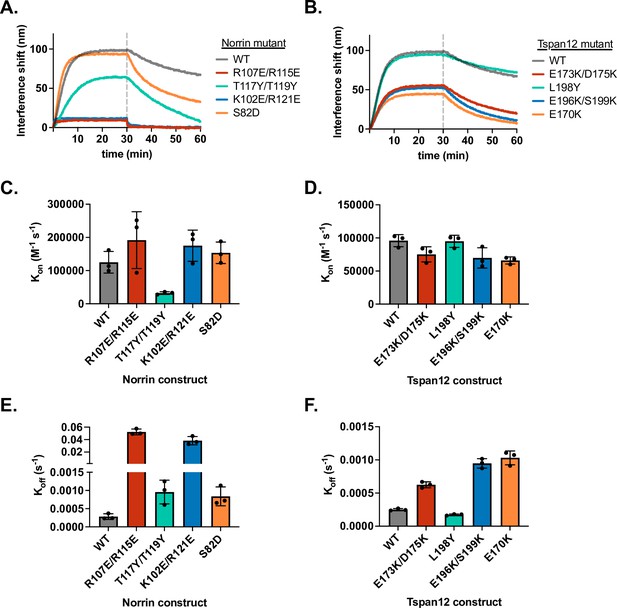
Binding kinetics of Norrin and Tspan12 mutants.
(A) Representative biolayer interferometry (BLI) association and dissociation traces of 32 nM wild-type (WT) or mutant Norrin binding to immobilized WT Tspan12, and for (B) 32 nM WT Norrin binding to immobilized WT or mutant Tspan12. Colors correspond to sites within the binding interface defined in Figure 2. (C and D) Rate constant Kon (mean ± SD) calculated from association and dissociation fits to traces shown in A and B, respectively; each mutant was measured in triplicate. (E and F) Rate constant Koff (mean ± SD) calculated from dissociation fits to traces shown in A and B, respectively; each mutant was measured in triplicate. Across the board, differences in KD for mutants compared to WT (Figure 2E and F) were driven largely by differences in Koff. Kinetic constants reported in Supplementary file 1.
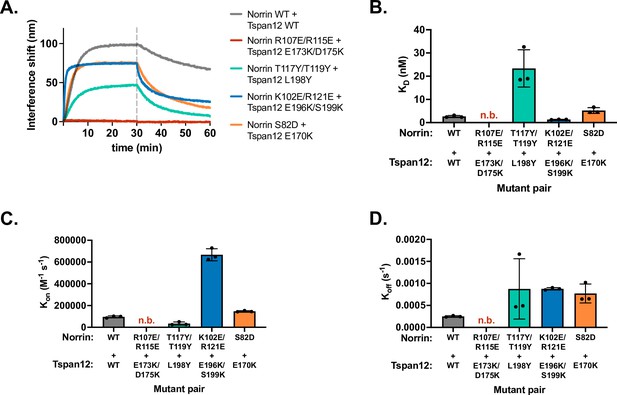
Binding affinity and kinetics of mutant Norrin binding to mutant Tspan12.
(A) Representative biolayer interferometry (BLI) association and dissociation traces showing 32 nM mutant Norrin binding to immobilized mutant Tspan12 relative to the WT/WT binding (gray) for mutants at Site 1 (red), Site 2 (teal), Site 3 (blue), and Site 4 (orange). For each site, mutated residues on Norrin and Tspan12 are predicted to interact according to the AlphaFold structure; in the case of charge-swapped mutations (i.e., Sites 1, 3, and 4), we hypothesized that the mutations to the two proteins may be compensatory. (B) Binding affinities calculated from kinetic fits to traces in A for the indicated mutant pairs. The charge-swapped mutants at Site 1, Norrin R107E/R115E and Tspan12 E173K/D175K, do not bind appreciably at 32 nM Norrin. At Site 3, charge-swapped Norrin K102E/R121E and Tspan12 196K/S199K binding is rescued to WT/WT levels compared to the much weaker binding affinities of either mutant for its WT counterpart: i.e., the mutations are compensatory. At Site 4, the Norrin S82D mutation partially rescues the deleterious effects of Tspan12 E170K. Data represent mean ± SD from three replicates. Binding affinities and kinetic constants reported in Supplementary file 1. (C) Kon and (D) Koff, calculated from each individual trace shown in A, measured in triplicate. Plots represent three replicates ± SD, and kinetic constants are reported in Supplementary file 1. WT, wild-type.
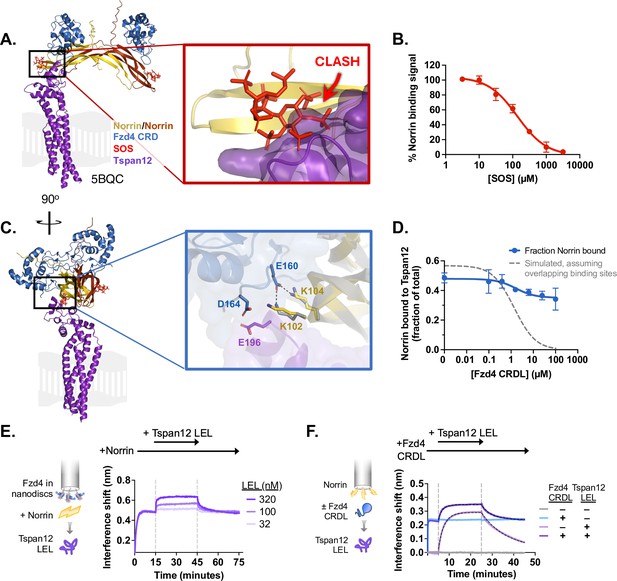
Norrin-Tspan12 binding is competitive with heparan sulfate proteoglycans but compatible with Fzd4 binding.
(A) The AlphaFold-predicted structure of Tspan12 (purple) bound to a Norrin dimer (yellow/orange), aligned to the crystal structure (5BQC) of a Norrin dimer bound to the Fzd4 CRD (blue) and sucrose octasulfate (SOS) (red). A zoomed view of the indicated region shows an overlap in the predicted binding site of Tspan12 with that of SOS, suggesting that Tspan12 and SOS cannot bind simultaneously to Norrin. (B) Displacement of Norrin (32 nM) from immobilized Tspan12 by increasing concentrations of SOS, as measured by biolayer interferometry (BLI) (see Figure 3—figure supplement 1A). The equilibrium binding signal is plotted as a percent of signal in the absence of SOS (mean ± SD of three independent experiments), yielding a Ki of 34±4 µM. (C) Side view of structures in A. A zoomed view of the indicated region shows that Tspan12 is predicted to occupy a site on Norrin adjacent to, but not overlapping with, the Fzd4 binding site; adjacent residues are shown. Norrin from the Tspan12-bound AlphaFold model (yellow) and the Fzd4 CRD-bound crystal structure (5BQC, gray) are overlaid. (D) Fzd4 CRDL does not fully compete with Tspan12-Norrin binding, as shown by equilibrium binding of 32 nM Norrin to Tspan12 immobilized on paramagnetic particles in the presence of increasing concentrations of purified Fzd4 CRDL. Bound Norrin and Norrin in the supernatant were both quantified by western blot (anti-Rho1D4; see Figure 3—figure supplement 1B) and used to calculate bound Norrin as a percentage of total Norrin. The expected competition curve, assuming fully competitive binding sites, was simulated (gray dashed line) given starting concentrations of 50 nM Tspan12 and 32 nM Norrin, and binding affinities of 10.4 nM for Tspan12-Norrin and 200 nM for Fzd4 CRDL-Norrin. However, the data better fit a model in which CRDL binding to Norrin shifts Norrin affinity for Tspan12 (blue line). Data represent mean ± SD of three replicates. (E) BLI traces of a ternary Fzd4-Norrin-Tspan12 large extracellular loop (LEL) complex. Biosensors loaded with nanodisc-embedded Fzd4 were first saturated with 100 nM Norrin, then bound to 32, 100, or 320 nM Tspan12 LEL. (F) BLI traces of ternary complex formation. Biosensors loaded with maltose binding protein (MBP)-tagged Norrin were pre-incubated in buffer or saturated with Fzd4 CRDL (5 µM), then bound to 100 nM MBP-tagged Tspan12 LEL (±5 µM CRDL). Tspan12 LEL did bind to Norrin in the presence of the Fzd4 CRDL (dark purple; apparent KD = 27 ± 2.8 nM), albeit more weakly than it bound to Norrin alone (light purple; apparent KD = 16 ± 1.8 nM; see also Figure 1E). Binding affinities were obtained from kinetic fits (black dotted line) to association and dissociation traces of MBP-LEL (100 nM) from three independent experiments.
-
Figure 3—source data 1
Interference shift and band quantification values used to generate Figure 3B and D.
- https://cdn.elifesciences.org/articles/96743/elife-96743-fig3-data1-v1.xlsx
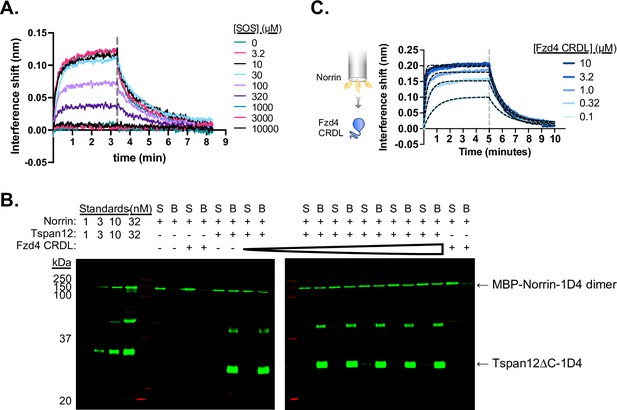
Tspan12 Norrin binding can be competed with sucrose octasulfate (SOS) but not the Fzd4 CRDL domain.
(A) Representative biolayer interferometry (BLI) traces of 32 nM Norrin binding to biosensor-immobilized Tspan12 in the presence of increasing concentrations of SOS. (B) Representative western blot of Norrin in the supernatant (S) or remaining bound (B) to Tspan12 or empty nanodiscs immobilized on paramagnetic particles in the presence of 0, 0.1, 0.4, 1.6, 6, 25, or 100 µM of purified Fzd4 CRD-linker domain, with both Norrin and Tspan12 detected by anti-Rho1D4 antibody. Norrin is detected within the dynamic range, as shown by concentration standards loaded at left. Tspan12 forms SDS-induced dimers, which can be detected at about 50 kDa. (C) BLI traces showing binding of the Fzd4 CRDL to biosensor-immobilized MBP-Norrin, which reaches saturation at 3.2 µM CRDL. Equilibrium binding values obtained from the overlaid fits (black dashed lines) give a steady-state affinity of 122±38 nM (mean ± SEM), in agreement with previously reported affinity measurements (Bang et al., 2018).
-
Figure 3—figure supplement 1—source data 1
Original files of western blots in Figure 3—figure supplement 1.
- https://cdn.elifesciences.org/articles/96743/elife-96743-fig3-figsupp1-data1-v1.zip
-
Figure 3—figure supplement 1—source data 2
Labeled western blots in Figure 3—figure supplement 1.
- https://cdn.elifesciences.org/articles/96743/elife-96743-fig3-figsupp1-data2-v1.zip
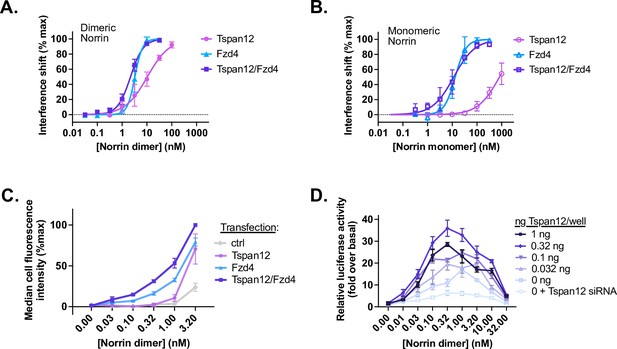
Tspan12 enhances Norrin-Fzd4 binding, cell-surface binding, and Norrin-stimulated β-catenin signaling at low Norrin concentrations.
(A) Steady-state binding curves of monomeric Tspan12∆C, monomeric Fzd4, or heterodimeric Tspan12∆C/Fzd4∆C receptors in biotinylated nanodiscs binding to dimeric or (B) monomeric (C93A/C95A/C131A) Norrin by biolayer interferometry (BLI). Steady-state binding signal is plotted as a percent of Bmax for three independent replicates (mean ± SD). Affinities and kinetic constants are reported in Supplementary file 1. (C) Indicated concentrations of Norrin-1D4 dimer binding to Expi293 cells transfected with Fzd4, Tspan12, or both Fzd4 and Tspan12, detected with fluorescently labeled Rho1D4 antibody and quantified by flow cytometry. Mean ± SD of three independent experiments are plotted. Co-transfection of Tspan12 increased Norrin recruitment to Fzd4-transfected cells at 0.1, 0.32, 1, and 3.2 nM Norrin (two-tailed t-test p-values of 0.00026, 0.00079, 0.0049, and 0.0018, respectively). (D) β-Catenin pathway activation resulting from increasing concentrations of Norrin was assessed in Fzd1/2/4/5/7/8-knockout HEK293T cells transfected with Tspan12 siRNA or increasing amounts of Tspan12 plasmid, along with Fzd4 and TopFlash luciferase reporter plasmids. Data are plotted as mean ± SD from triplicate wells are representative of three independent experiments.
-
Figure 4—source data 1
Interference shift, cell fluorescence, and luciferase activity values used to generate Figure 4A–D.
- https://cdn.elifesciences.org/articles/96743/elife-96743-fig4-data1-v1.xlsx
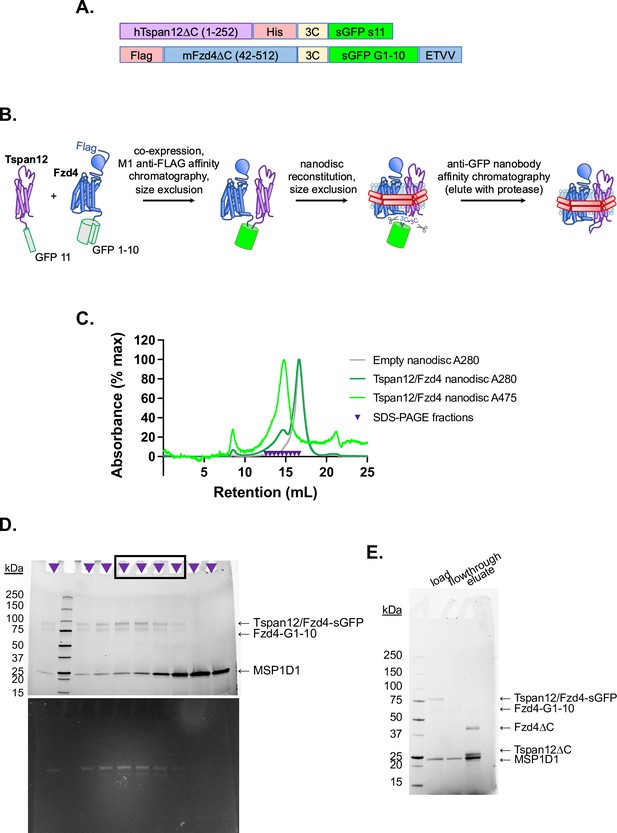
Purification of Fzd4/Tspan12 dimer and insertion into nanodiscs.
(A) Construct design of Tspan12 and Fzd4 C-terminally tagged with split GFP fragments s11 and s1–10, respectively, both downstream of a 3C protease recognition sequence. Fzd4 is additionally tagged with an N-terminal FLAG tag and Tspan12 is additionally tagged with a C-terminal 6xHis tag. The Fzd4 C-terminal PDZ ligand (ETVV) is appended after split GFP to improve expression and surface localization (Bruguera et al., 2022). (B) Schematic of Tspan12/Fzd4 heterodimer expression and purification. The constructs in A were co-expressed in Sf9 cells, solubilized in n-dodecyl-β-D-maltopyranoside (DDM), and co-purified on M1 anti-FLAG affinity resin followed by size exclusion chromatography. The dimer was reconstituted into nanodiscs and further purified by size exclusion followed by capture on anti-GFP nanobody resin, from which it was eluted by 3C protease. (C) Size exclusion traces of empty nanodiscs or Tspan12/Fzd4 dimer reconstituted into excess nanodiscs, with absorbance detected at 280 and 475 nm (GFP absorption peak), on a Superose 6 Increase column. Indicated fractions were pooled for (D) SDS-PAGE, imaged using StainFree imaging (above) or GFP fluorescence (below); intact GFP is SDS-resistant. Fractions on the right side of the peak (boxed) were pooled in order to exclude any potential separately-reconstituted dimers (i.e., two nanodiscs with one receptor each, linked together by the GFP moiety). (E) Pooled fractions were purified by GFP nanobody resin and eluted with 3C protease. The load, flowthrough, and eluate are shown.
-
Figure 4—figure supplement 1—source data 1
Original files of gels in Figure 4—figure supplement 1.
- https://cdn.elifesciences.org/articles/96743/elife-96743-fig4-figsupp1-data1-v1.zip
-
Figure 4—figure supplement 1—source data 2
Labeled gels in Figure 4—figure supplement 1.
- https://cdn.elifesciences.org/articles/96743/elife-96743-fig4-figsupp1-data2-v1.zip
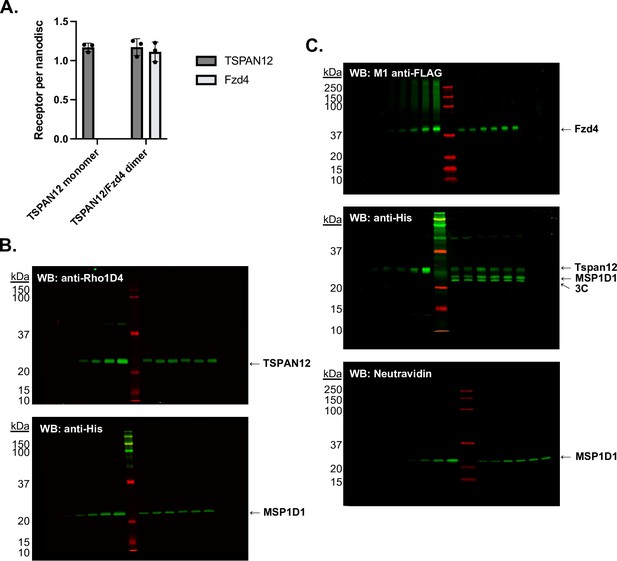
Stoichiometry of receptors in nanodiscs was determined by quantitative western blot.
(A) Receptors per nanodisc in monomeric Tspan12 (mean ± SEM 1.17±0.05 Tspan12 per two MSP1D1) and heterodimeric Tspan12/Fzd4 nanodiscs (1.17±0.11 Tspan12 and 1.11±0.12 Fzd4 per two MSP1D1) as calculated from three independent samples each, with each component measured three times each by quantitative western blot. (B) Representative anti-Rho1D4 (top) and anti-His (bottom) western blots to quantify Tspan12-1D4 and His-MSP1D1, respectively, in monomeric Tspan12 reconstitutions. A known dilution series of purified protein was loaded in left lanes to generate a standard curve in the linear range of detection, against which dilutions of three nanodisc reconstitutions, loaded in duplicate in right lanes, were compared. (C) Representative anti-FLAG (top), anti-His (middle), and Neutravidin-800 (bottom) western blots to quantify FLAG-Fzd4, Tspan12-His, and biotinylated MSP1D1, respectively, in Tspan12/Fzd4 heterodimer preparations.
-
Figure 4—figure supplement 2—source data 1
Original files of western blots in Figure 4—figure supplement 2.
- https://cdn.elifesciences.org/articles/96743/elife-96743-fig4-figsupp2-data1-v1.zip
-
Figure 4—figure supplement 2—source data 2
Labeled western blots in Figure 4—figure supplement 2.
- https://cdn.elifesciences.org/articles/96743/elife-96743-fig4-figsupp2-data2-v1.zip
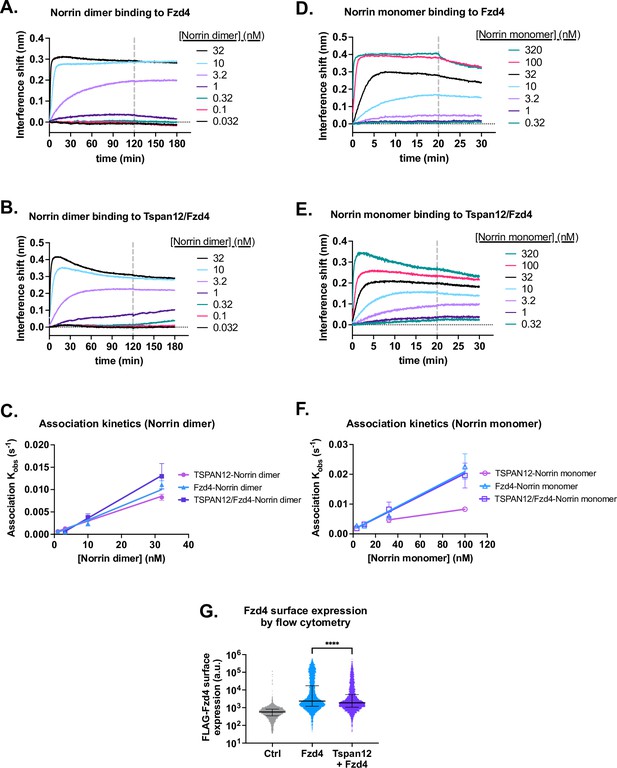
Tspan12 enhances Norrin recruitment.
(A) Representative biolayer interferometry (BLI) association and dissociation traces of dimeric Norrin binding to Fzd4 monomer or (B) Tspan12/Fzd4 heterodimer in nanodiscs. (C) Observed association rate constant Kobs of Norrin dimer binding to Tspan12, Fzd4, or Tspan12/Fzd4 heterodimer in nanodiscs, plotted against Norrin concentration. Linear fits were used to obtain association rate constants reported in Supplementary file 1. Data represent mean ± SD for three independent replicates. (D) Representative BLI association and dissociation traces of monomeric Norrin (C93A/ C95A/C131A) binding to Fzd4 monomer or (E) Tspan12/Fzd4 heterodimer in nanodiscs. (F) Observed association rate constant Kobs (mean ± SD) of Norrin monomer binding to Tspan12, Fzd4, or Tspan12/Fzd4 heterodimer in nanodiscs, plotted against Norrin monomer concentration. (G) Fzd4 surface expression on Expi293 cells transfected with empty vector, FLAG-Fzd4, or FLAG-Fzd4+Tspan12, which were then stained with M1 anti-FLAG antibody conjugated to Alexa Fluor 647. Cell fluorescence is measured by flow cytometry and plotted along with the median and interquartile range. Co-expression of Tspan12 modestly but significantly decreases surface expression of Fzd4 (Mann-Whitney test, p-value<0.0001 in each of three independent experiments).
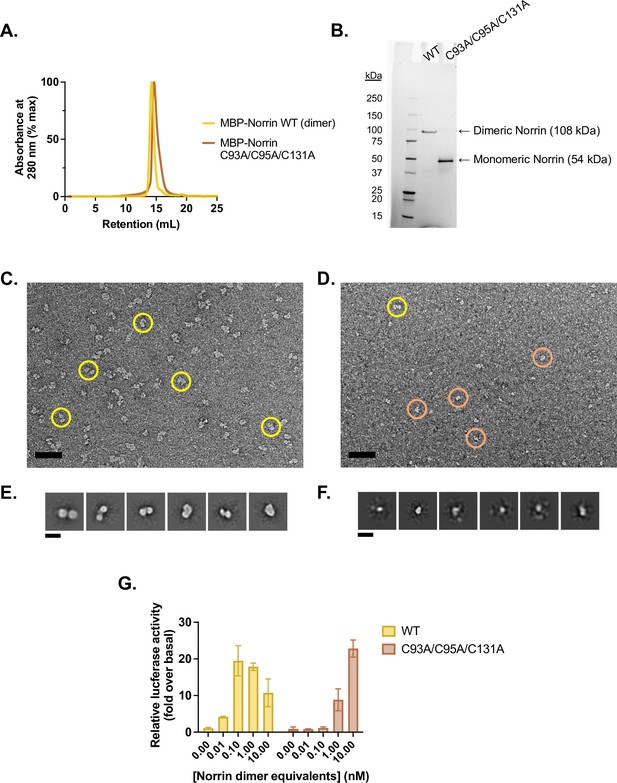
Purification and validation of monomeric Norrin.
(A) Analytical size exclusion traces of wild-type (WT) (dimeric) MBP-Norrin (yellow) and MBP-Norrin rendered monomeric (brown) via mutations C93A/C95A/C131A to eliminate the intermolecular disulfides. Purified protein was injected at 25 µM on a Superdex 200 Increase 10/300 column, resulting on an on-column concentration in excess of 2.5 µM assuming a 10-fold on-column dilution factor. (B) Non-reducing SDS-PAGE gel of WT and C93A/C95A/C131A MBP-Norrin. (C) Uranyl acetate negative stain micrograph of WT MBP-Norrin, prepared at 100 nM. Scale bar is 50 nm. Representative picked particles indicated in yellow. (D) Uranyl acetate negative stain micrograph of MBP-Norrin C93A/C95A/C131A, prepared at 100 nM. Scale bar is 50 nm. Representative picked particles indicated with circles. Yellow-circled particle appears to be large enough to potentially be a dimer; brown circles show some smaller species, which dominate. (E) 2D class averages of picked particles from C show two lobes, consistent with two copies of MBP-Norrin (54 kDa each). (F) 2D class averages of picked particles from D show small, single particles that are hard to align; they are about half the size of particles in E, consistent with one copy of MBP-Norrin. This suggests that MBP-Norrin C93A/C95A/C131A is monomeric at 100 nM. (G) β-Catenin transcriptional activity in response to 0.01–10 nM purified WT (dimeric) or 0.02–20 nM C93A/C95A/C131A (monomeric) Norrin, in Fzd1/2/4/5/7/8-knockout HEK293T cells transfected with Fzd4 and TopFlash luciferase reporter plasmids. Data are plotted as mean ± SD from n=3 replicate wells.
-
Figure 4—figure supplement 4—source data 1
Original file of gel in Figure 4—figure supplement 4.
- https://cdn.elifesciences.org/articles/96743/elife-96743-fig4-figsupp4-data1-v1.zip
-
Figure 4—figure supplement 4—source data 2
Labeled gel in Figure 4—figure supplement 4.
- https://cdn.elifesciences.org/articles/96743/elife-96743-fig4-figsupp4-data2-v1.zip
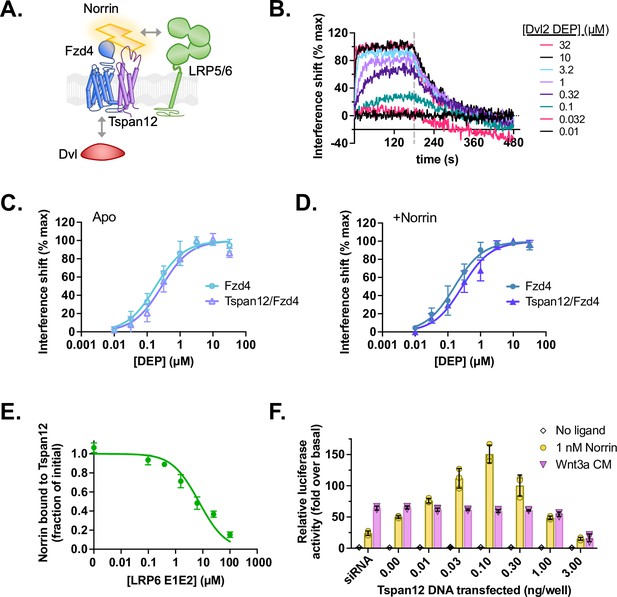
Tspan12 does not directly enhance formation of a Norrin-LRP5/6-Fzd4-Dvl signaling complex.
(A) Hypothesis: Tspan12 could enhance Norrin signaling by enhancing interactions within the Norrin-LRP5/6-Fzd4-Dvl complex, including Fzd-Dvl binding and Norrin-LRP binding. (B) Representative biolayer interferometry (BLI) traces of the Dvl2 DEP domain associating to and dissociating from Fzd4 in nanodiscs containing 75:20:5 POPC:Ccholesterol:PIP2. (C) Equilibrium binding of the Dvl2 DEP domain to Fzd4 monomer or Tspan12/Fzd4 heterodimer in nanodiscs; affinities ± SEM are 183±24 and 279±46 nM, respectively. (D) Equilibrium binding of the Dvl2 DEP domain to Fzd4 monomer or Tspan12/Fzd4 heterodimer nanodiscs, each pre-saturated with 10 nM Norrin. Binding affinities are 161±21 and 274±39 nM (mean ± SEM), respectively, determined from three independent replicates. Affinities and kinetic constants are reported in Supplementary file 1. (E) The LRP6 E1E2 domain fully competes with Tspan12-Norrin binding, as shown by decreased equilibrium binding of 32 nM Norrin to Tspan12 immobilized on paramagnetic particles in the presence of increasing concentrations of purified LRP6 E1E2 domain. Norrin was quantified by western blot (anti-Rho1D4; see Figure 5—figure supplement 1) and plotted as a percent of bound Norrin in the absence of LRP6 E1E2. The curve was fit to a competitive binding model using known binding affinities of 10.4 nM for Tspan12-Norrin and starting concentrations of 50 nM Tspan12 and 32 nM Norrin; the best fit reported a Norrin-LRP6 E1E2 binding affinity of 1.06 µM (95% CI 0.747–1.51 µM). Data represent mean ± SD of three replicates. (F) β-Catenin transcriptional activity in response to no ligand, 1 nM recombinant Norrin, or Wnt3a conditioned media (Wnt3a CM) in Fzd1/2/4/5/7/8-knockout HEK293T cells transfected with Tspan12 siRNA or indicated amount of Tspan12_pTT5 plasmid, along with Fzd4 and TopFlash luciferase reporter plasmids. Data are plotted as mean ± SD from n=3 replicate wells.
-
Figure 5—source data 1
Interference shift, band quantification, and luciferase activity values used to generate Figure 5C–F.
- https://cdn.elifesciences.org/articles/96743/elife-96743-fig5-data1-v1.xlsx
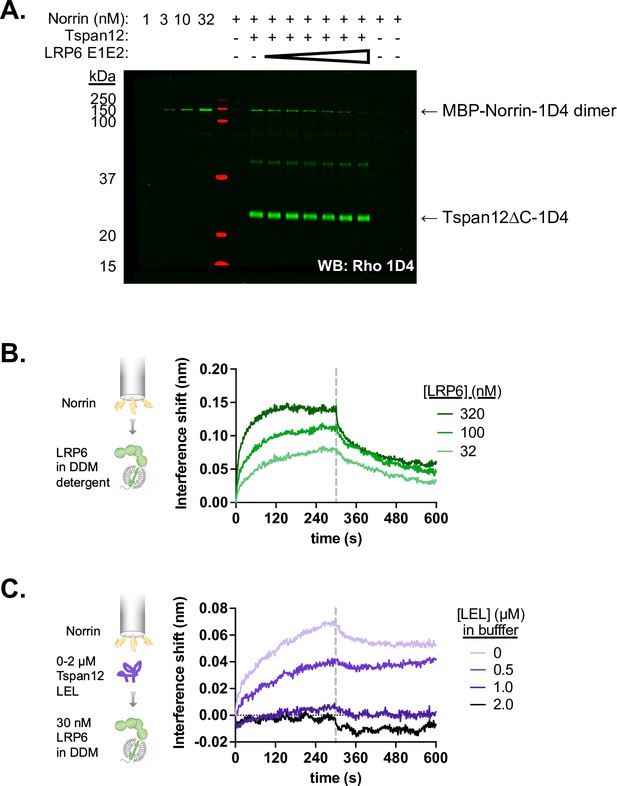
Tspan12 and LRP6 E1E2 compete for Norrin binding.
(A) Representative western blot of Norrin bound to Tspan12 or empty nanodiscs immobilized on paramagnetic particles in the presence of 0, 0.1, 0.4, 1.6, 6, 25, or 100 µM of purified LRP6 E1E2 domain, with both Norrin and Tspan12 detected by anti-Rho1D4 antibody. Norrin is detected within the dynamic range, as shown by concentration standards loaded to the left of the ladder. (B) Association and dissociation biolayer interferometry traces of purified LRP6 (residues 20–1439, including the transmembrane domain but with a truncated C-terminus, in n-dodecyl-β-D-maltopyranoside [DDM] detergent, at 32, 100, or 320 nM) binding to MBP-Norrin-loaded biosensors. (C) Biolayer interferometry traces of 30 nM LRP6 associating to and dissociating from MBP-Norrin-loaded biosensors, pre-equilibrated with increasing concentrations of MBP-fused Tspan12 large extracellular loop (LEL).
-
Figure 5—figure supplement 1—source data 1
Original file of western blot in Figure 5—figure supplement 1.
- https://cdn.elifesciences.org/articles/96743/elife-96743-fig5-figsupp1-data1-v1.zip
-
Figure 5—figure supplement 1—source data 2
Labeled western blot in Figure 5—figure supplement 1.
- https://cdn.elifesciences.org/articles/96743/elife-96743-fig5-figsupp1-data2-v1.zip
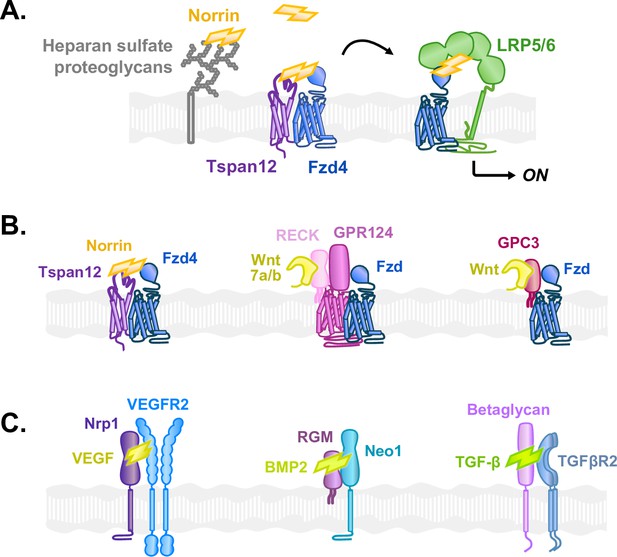
Diverse co-receptors facilitate growth factor signaling by capturing and delivering ligands to their target receptors.
(A) Model: Norrin is captured by Tspan12 or heparan sulfate proteoglycans (HSPGs) and is handed off to Fzd4 for association with LRP5/6 and subsequent signaling. Norrin binding to cell-surface Fzd4 is enhanced when HSPGs concentrate Norrin at the cell surface. In contrast, Tspan12 directly and specifically delivers Norrin to co-localized Fzd4. (B) In the β-catenin signaling pathway, Left: Tspan12 captures Norrin and co-localizes with Fzd4, delivering Norrin to Fzd4. Middle: Likewise, RECK binds Wnt7a/b and co-localizes with Fzd via GPR124, delivering Wnt7a/b to Fzd. Right: Glypican-3 (GPC3) also binds both Fzd and Wnt to deliver Wnt to Fzd and enhance signaling. (C) Structurally diverse co-receptors play a similar role to Tspan12 in various pathways activated by cystine knot growth factors. Left: Neuropilin-1 (Nrp1) captures vascular endothelial growth factor A (VEGF) and co-localizes with the VEGF receptor 2 (VEGFR2) to specifically deliver VEGF to VEGFR2. Middle: The repulsive guidance molecule (RGM) binds bone morphogenic protein 2 (BMP2) as well as neogenin-1 (Neo1) to facilitate BMP signaling. Right: Betaglycan captures transforming growth factor β1 (TGF-β) and presents it to TGF-β receptor type 2 (TGFβR2).
Tables
Reagent type (species) or resource | Designation | Source or reference | Identifiers | Additional information |
---|---|---|---|---|
Recombinant DNA reagent | untagged Tspan12 | DNASU | Clone HsCD00045716 | Subcloned into pTT5 vector |
Recombinant DNA reagent | Tspan12∆C (human) | This paper | pVL1393 vector; residues 1–252; Rho-1D4-tagged | |
Recombinant DNA reagent | Tspan12 (full-length, human) | This paper | RRID:Addgene_216381 | pTT5 vector; Rho-1D4 tagged; deposited at Addgene (#216381) |
Recombinant DNA reagent | Tspan12-LEL11 (human) | PMID:28658627 | Rho-1D4-tagged and subcloned into pVL1393 vector | |
Recombinant DNA reagent | Tspan12LEL (human) | This paper | RRID:Addgene_216383 | pAcGP67a vector; residues 118–218; MBP-tagged; Deposited at Addgene (#216383) |
Recombinant DNA reagent | Fzd4 (mouse) | PMID:35998232 | RRID:Addgene_216378 | pFastBac1 vector; residues 42–537; FLAG-tagged; Deposited at Addgene (#216378) |
Recombinant DNA reagent | HA-FLAG-Fzd4∆C-3C-GFP1-10OPT-ETVV (mouse) | PMID:35074428 | RRID:Addgene_216380 | pVL1393 vector; residues 42–513; Deposited at Addgene (#216380) |
Recombinant DNA reagent | Tspan12∆C-His-3C-GFP11 (human) | This paper | RRID:Addgene_216382 | pVL1393 vector; residues 1–252; Deposited at Addgene (#216382) |
Recombinant DNA reagent | LRP6 (human) | PMID:35074428 | pEZT-BM vector; residues 20–1439; FLAG-tagged | |
Recombinant DNA reagent | LRP6 E1E2 (human) | PMID:22000856 | pAcGP67a vector; 10xHis-tagged | |
Recombinant DNA reagent | MESD (human) | PMID:35074428 | pEZT-BM vector | |
Recombinant DNA reagent | Norrin (human) | PMID:35074428 | RRID:Addgene_216384 | pAcGP67a vector; residues 33–133; MBP- and Rho-1D4 tags; Deposited at Addgene (#216384) |
Recombinant DNA reagent | Monomeric Norrin | This paper | RRID:Addgene_216386 | pAcGP67a vector; residues 33–133; MBP- and Rho-1D4-tagged; C93A/C95A/C131A; Deposited at Addgene (#216386) |
Recombinant DNA reagent | Fzd4 CRDL (mouse) | PMID:30104375 | RRID:Addgene_216379 | pAcGP67a vector; residues 42–203; 6xHis-tagged; Deposited at Addgene (#216379) |
Recombinant DNA reagent | DEP (DEP domain from mouse Dvl2) | PMID:35998232 | RRID:Addgene_216386 | pCDF vector; residues 416–510; MBP-tagged; Deposited at Addgene (#216386) |
Recombinant DNA reagent | GFP nanobody | PMID:20945358 | pET26(+) vector; with pelB signal sequence and 6xHis tag | |
Recombinant DNA reagent | MSP1D1 | Addgene | RRID:Addgene_20061 | Sligar lab |
Recombinant DNA reagent | MSP1E3D1 | Addgene | RRID:Addgene_20066 | Sligar lab |
Commercial assay or kit | EZ-link NHS-PEG4-Biotin | Thermo | Cat. #: A39259 | |
Other | 16:0–18:1 phosphatidyl choline (POPC) | Avanti Polar Lipids | Cat. #: 850457 | For nanodisc reconstitution (see Materials and methods section) |
Other | 16:0–18:1 phosphatidylglycerol (POPG) | Avanti Polar Lipids | Cat. #: 840457 | For nanodisc reconstitution (see Materials and methods section) |
Other | 18:0–20:4 brain phosphatidylinositol-4,5-bisphosphate (PIP2) | Avanti Polar Lipids | Cat. #: 840046 | For nanodisc reconstitution (see Materials and methods section) |
Antibody | Rho anti-1D4 (mouse monoclonal) | Santa Cruz Biotechnologies | Cat. #: sc-57432; RRID:AB_785511 | (0.5 µg/mL) |
Antibody | THE anti-His (mouse monoclonal) | GenScript | Cat. #: A00186; RRID:AB_914704 | (0.33 µg/mL) |
Antibody | M1 anti-FLAG (mouse monoclonal) | Sigma | Cat. #: F3040; RRID:AB_439712 | (0.5 µg/mL) |
Antibody | IRDye 800 goat anti-mouse secondary | LI-COR | Cat. #: 926–32210; RRID:AB_621842 | (1:15,000) |
Peptide, recombinant protein | Dylight Neutravidin-800 | Invitrogen | Cat. #: 22853 | (1:10,000) |
Other | CNBr-activated sepharose | Cytiva | Cat. #: 17043001 | To generate GFP nanobody resin (see Materials and methods section) |
Other | Streptavidin paramagnetic particles | Promega | Cat. #: Z5481 | For bead binding competition experiments (see Materials and methods section) |
Strain, strain background (Escherichia coli) | BL21(DE3)-RIL | Agilent | Cat. #: 230245 | |
Cell line (Spodoptera frugiperda) | Sf9 cells | Expression Systems | Cat. #: 94-001F; RRID:CVCL_0549 | |
Cell line (human) | Expi293 cells | Thermo | Cat. #: A14527; RRID:CVCL_D615 | |
Cell line (human) | Freestyle293 cells | Thermo | Cat. #: R79007; RRID:CVCL_D603 | |
Cell line (human) | HEK293T cells (Fzd1/2/4/5/7/8 KO) | PMID:28733458 | Boutros lab | |
Sequence-based reagent | Tspan12 siRNA duplex #1 | Sigma | Cat. #: VC30002 | 5’-GCUUAUCUUUGCCUUCUCCTT-3’ and 5’-GGAGAAGGCAAAGAUAAGCTT-3’ |
Sequence-based reagent | Tspan12 siRNA duplex #2 | Sigma | Cat. #: VC30002 | 5’-AUGAGGGACUACCUAAAUATT-3’ and 5’-UAUUUAGGUAGUCCCUCAUTT-3’ |
Sequence-based reagent | Control siRNA | Sigma | Cat. #: SIC001 | |
Recombinant DNA reagent | Super8xTOPFLASH | Addgene | RRID:Addgene_12456 | Moon lab |
Commercial assay or kit | Dual-Light reporter assay system | Applied Biosystems | Cat. #: T1005 | |
Software, algorithm | Prism 9 | GraphPad Software, LLC | RRID:SCR_002798 | |
Software, algorithm | AlphaFold2 | PMID:34265844 | RRID:SCR_025454 | |
Software, algorithm | AlphaFold Multimer v2 | DOI: 10.1101/2021.10.04.463034 | ||
Software, algorithm | ColabFold | PMID:35637307 | RRID:SCR_025453 | |
Software, algorithm | ChimeraX 1.6 | PMID:28710774 | RRID:SCR_015872 | |
Software, algorithm | cryoSPARC v4 | Structura Biotechnology | RRID:SCR_016501 |
Additional files
-
Supplementary file 1
Table of kinetic constants and steady-state affinities quantified by biolayer interferometry.
- https://cdn.elifesciences.org/articles/96743/elife-96743-supp1-v1.docx
-
MDAR checklist
- https://cdn.elifesciences.org/articles/96743/elife-96743-mdarchecklist1-v1.docx