Energetic demands regulate sleep-wake rhythm circuit development
Figures
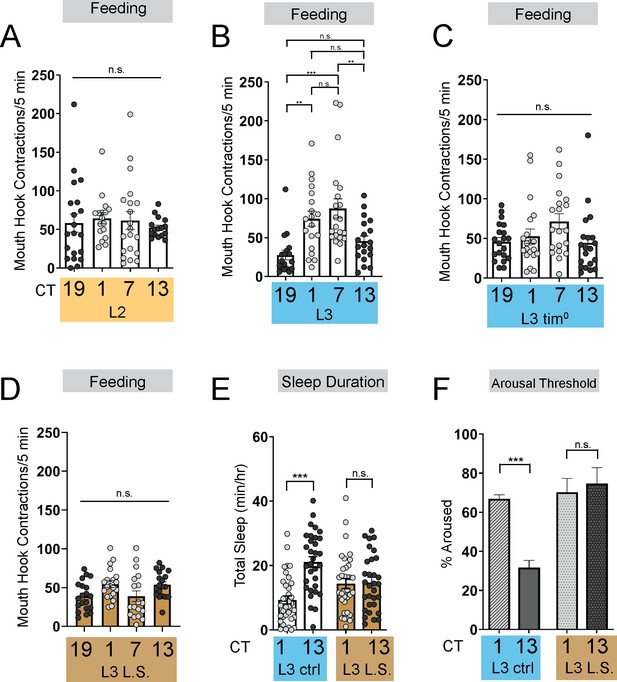
Energetic drive limits sleep rhythm development.
(A–D) Feeding rate (# of mouth hook contractions per 5 min) of L2 controls (A) L3 raised on regular (ctrl) food (B) L3 clock mutants (C) and L3 raised on low sugar (L.S.) food (D) across the day. (E) Sleep duration at CT1 and CT13 in L3 raised on regular (ctrl) and L.S. food. (F) Arousal threshold at CT1 and CT13 in L3 raised on regular (ctrl) and L.S. food. A-D, n=18–20 larvae; E, n=29–34 larvae; (F) n=100–172 sleep episodes, 18 larvae per genotype. One-way ANOVAs followed by Sidak’s multiple comparisons tests (A–D) Two-way ANOVAs followed by Sidak’s multiple comparison test (E–F). For this and all other figures unless otherwise specified, data are presented as mean ± SEM; n.s., not significant, *p<0.05, **p<0.01, ***p<0.001. Source data in Figure 1—source data 1.
-
Figure 1—source data 1
Raw values for sleep and feeding in genetic and nutritional manipulations.
- https://cdn.elifesciences.org/articles/97256/elife-97256-fig1-data1-v1.xlsx
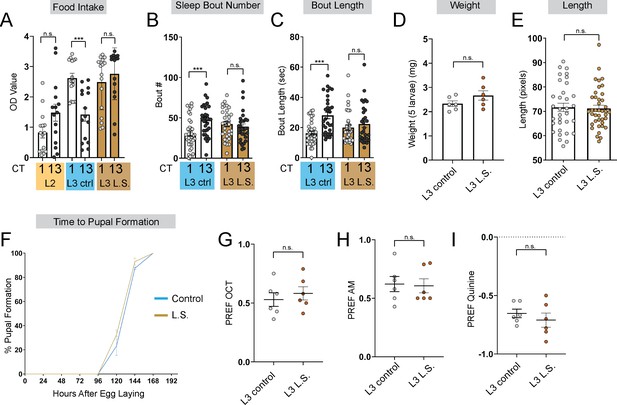
Larvae reared on low sugar diet develop normally.
(A) Feeding amount of L2 controls, L3 raised on regular (ctrl) food, and L3 raised on low sugar (L.S.) food at CT1 and CT13. (B, C) Sleep bout number (B) and bout length (C) at CT1 and CT13 in L3 raised on regular (ctrl) and L.S. food. (D) Total body weight of early L3 (in groups of 5) raised on ctrl or L.S. food. (E) Total body length of early L3 raised on ctrl or L.S. food. (F) Developmental analysis of time to pupal formation of animals raised on ctrl or L.S. food. (G–I) Naïve OCT, AM, and quinine preference in L3 raised on ctrl and L.S. food. (B-C) n=29–34 larvae; (D) n=30 larvae per food condition; (E) n=33–40 larvae; (F) n=100–170 larvae; (G-I) n=6 PREFs (180 larvae) per genotype. Two-way ANOVAs followed by Sidak’s multiple comparison test (B–C) Unpaired two-tailed Student’s t-tests (D–E) and (G–I). Source data in Figure 1—figure supplement 1—source data 1.
-
Figure 1—figure supplement 1—source data 1
Measures of all sleep and developmental parameters on L.S. food.
- https://cdn.elifesciences.org/articles/97256/elife-97256-fig1-figsupp1-data1-v1.xlsx
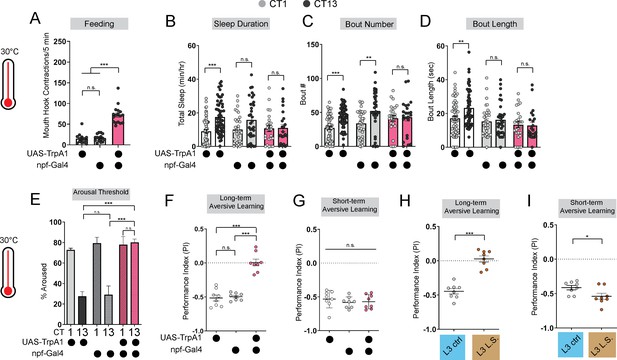
Immature feeding strategies limit LTM.
(A) Feeding rate of L3 expressing npf-Gal4 >UAS-TrpA1 and genetic controls at 30 °C at CT13. (B–D) Sleep duration (B), bout number (C), and bout length (D) of L3 expressing npf-Gal4 >UAS-TrpA1 and genetic controls at 30 °C. (E–G) Arousal threshold (E), long-term aversive memory performance (F), and short-term aversive memory performance (G) in L3 expressing npf-Gal4 >UAS-TrpA1 and genetic controls at 30 °C. (H,I) Short-and long-term term aversive memory performance in L3 raised on ctrl and L.S. food. A, n=18–20 larvae; B-D, n=24–61 larvae; E, n=125–160 sleep episodes, 18 larvae per genotype; F-I, n=8 PIs (240 larvae) per genotype. One-way ANOVAs followed by Sidak’s multiple comparisons tests (A) and (E–G) Two-way ANOVAs followed by Sidak’s multiple comparison test (B–D) unpaired two-tailed Student’s t-test (H and I). Source data in Figure 2—source data 1.
-
Figure 2—source data 1
Sleep and LTM values for npf manipulations at 30c.
- https://cdn.elifesciences.org/articles/97256/elife-97256-fig2-data1-v1.xlsx
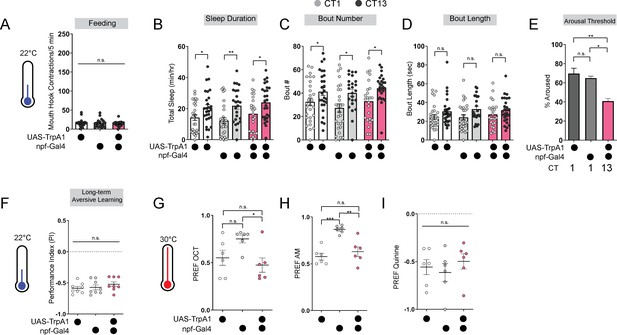
Baseline odor preferences, feeding, and sleep are not affected by npf-Gal4 manipulations.
(A) Feeding rate of L3 expressing npf-Gal4 >UAS-TrpA1 and genetic controls at 22 °C at CT13. (B–E) Sleep duration (B), bout number (C), bout length (D), and arousal threshold (E) in L3 expressing npf-Gal4 >UAS-TrpA1 and genetic controls at CT1 and CT13 at 22 °C (temperature controls). (F) Long-term aversive memory performance in L3 expressing npf-Gal4 >UAS-TrpA1 and genetic controls at 22 °C (temperature controls). (G–I) Naïve OCT, AM, and quinine preference in L3 expressing npf-Gal4 >UAS-TrpA1 and genetic controls at 30 °C. (A) n=18–20 larvae; (B-D) n=22–27 larvae; (E) n=120–205 sleep episodes, 18 larvae per genotype; (F) n=8 PIs (240 larvae) per genotype; (G-I) n=6 PREFs (180 larvae) per genotype. One-way ANOVAs followed by Sidak’s multiple comparisons tests (A), (E), and (F–I) Two-way ANOVAs followed by Sidak’s multiple comparison test (B–D). Source data in Figure 2—figure supplement 1—source data 1.
-
Figure 2—figure supplement 1—source data 1
Temperature control data for npf manipulations.
- https://cdn.elifesciences.org/articles/97256/elife-97256-fig2-figsupp1-data1-v1.xlsx
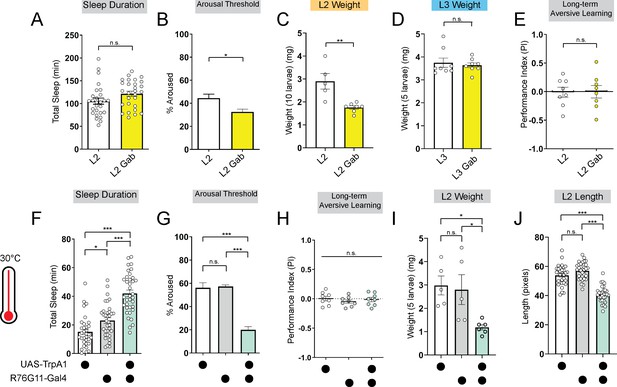
Deeper sleep in L2 is energetically disadvantageous.
(A, B) Sleep duration (A) and arousal threshold (B) of L2 control fed vehicle control (L2) or Gaboxadol (L2 Gab). (C, D) Total body weight of L2 (C) (in groups of 10) or L3 (D) (in groups of 5) fed vehicle control or Gaboxadol (Gab). (E) Long-term aversive memory performance in L2 fed vehicle control (L2) or Gaboxadol (L2 Gab). (F, G) Sleep duration (F) and arousal threshold (G) of L2 expressing R76G11-Gal4>UAS-TrpA1 and genetic controls at 30 °C. (H) Long-term aversive memory performance of L2 expressing R76G11-Gal4>UAS-TrpA1 and genetic controls at 30 °C. (I, J) Total body weight (I) and total body length (J) of L2 expressing R76G11-Gal4>UAS-TrpA1 and genetic controls at 30 °C. (A) n=28 larvae; (B) n=110–220 sleep episodes, 18 larvae per genotype; (C) n=5–7 groups (50–70 larvae); (D) n=8 groups (40 larvae); (E) n=8 PIs (240 larvae) per genotype; (F) n=33–36 larvae; (G) n=234–404 sleep episodes, 30–40 larvae per genotype; (H) n=8 PIs (240 larvae) per genotype; (I) n=5 groups (25 larvae); (J) n=31–32 larvae. Unpaired two-tailed Student’s t-tests (A–E) one-way ANOVAs followed by Sidak’s multiple comparisons tests (F–J). Source data in Figure 3—source data 1.
-
Figure 3—source data 1
Sleep, LTM, and developmental data for L2 manipulations.
- https://cdn.elifesciences.org/articles/97256/elife-97256-fig3-data1-v1.xlsx
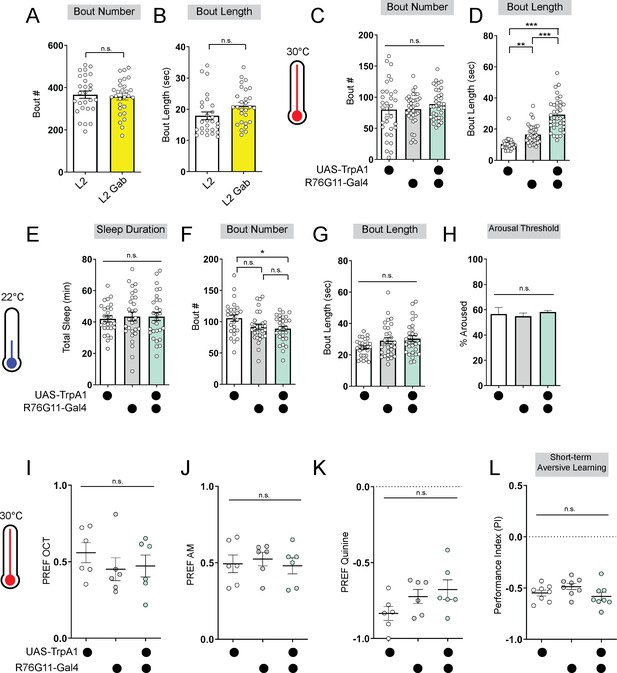
Baseline sleep and odor preferences are not disrupted by R76G11-Gal4 manipulations.
(A, B) Sleep bout number (A) and bout length (B) of L2 control fed vehicle control (L2) or Gaboxadol (L2 Gab). (C, D) Sleep bout number (C) and bout length (D) of L2 expressing R76G11-Gal4>UAS-TrpA1 and genetic controls at 30 °C. (E–H) Sleep duration (E), bout number (F), bout length (G), and arousal threshold (H) of L2 expressing R76G11-Gal4>UAS-TrpA1 and genetic controls at 22 °C (temperature controls). (I–K) Naïve OCT, AM, and quinine preference in L2 expressing R76G11-Gal4>UAS-TrpA1 and genetic controls at 30 °C. (L) Short-term aversive memory performance of L2 expressing R76G11-Gal4>UAS-TrpA1 and genetic controls at 30 °C. (A, B) n=28 larvae; (C-D) n=33–36 larvae; (E-G) n=27–29 larvae; (H) n=145–316 sleep episodes, 30–40 larvae per genotype; (I-K) n=6 PREFs (180 larvae) per genotype; (L) n=8 PIs (240 larvae) per genotype. Unpaired two-tailed Student’s t-tests (A–B) One-way ANOVAs followed by Sidak’s multiple comparisons tests (C–L). Source data in Figure 3—figure supplement 1—source data 1.
-
Figure 3—figure supplement 1—source data 1
Temperature control and naive preference data for L2 manipulations.
- https://cdn.elifesciences.org/articles/97256/elife-97256-fig3-figsupp1-data1-v1.xlsx
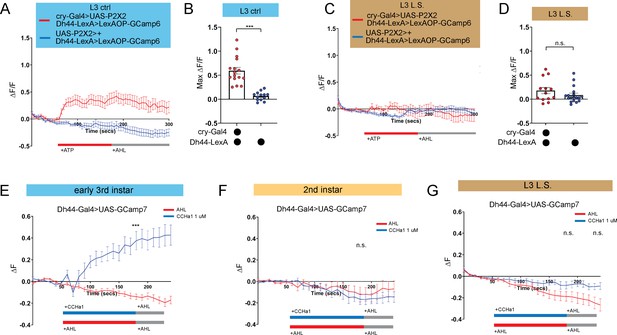
DN1a-Dh44 circuit formation is developmentally plastic.
(A, C) GCaMP6 signal in Dh44 neurons with activation of DN1a neurons in L3 controls (A) and L3 raised on L.S. food (C). Red bar indicates ATP application and gray bar indicates AHL application. (B, D) Maximum GCaMP change (ΔF/F) for individual cells in L3 controls (B) and L3 raised on L.S. food (D). (E–G) GCaMP7 signal in Dh44 neurons during bath application of 1 µM CCHa1 synthetic peptide in L3 controls (E), L2 controls (F) and L3 raised on L.S. food (G) brains. Red/blue bar indicates timing of CCHa1 (blue) or buffer (AHL, red) application and gray bar indicates timing of washout AHL application. (A-D) n=12–18 cells, 8–10 brains; (E-G) n=11–15 cells, 5–10 brains. Unpaired two-tailed Student’s t-tests (B and D) Mann-Whitney U test (E–G). Source data in Figure 4—source data 1.
-
Figure 4—source data 1
Individual cell fluorescence data for P2X2 and CCHa1 imaging.
- https://cdn.elifesciences.org/articles/97256/elife-97256-fig4-data1-v1.xlsx
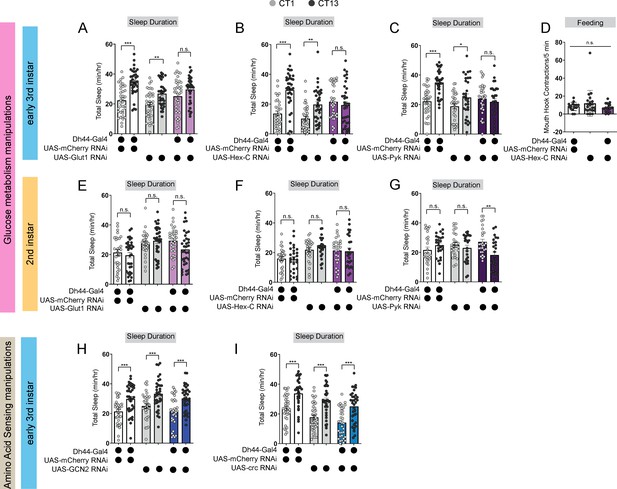
Dh44 neurons require glucose metabolic genes to regulate sleep-wake rhythms.
(A–C) Sleep duration in L3 expressing UAS-Glut1-RNAi (A), UAS-Hex-C-RNAi (B), and UAS-Pyk-RNAi (C) with Dh44-Gal4 and genetic controls at CT1 and CT13. (D) Feeding rate (# of mouth hook contractions per 5 min) of L3 expressing UAS-Hex-C-RNAi with Dh44-Gal4 and genetic controls at CT13. (E–G) Sleep duration in L2 expressing UAS-Glut1-RNAi (E), UAS-Hex-C-RNAi (F), and UAS-Pyk-RNAi (G) with Dh44-Gal4 and genetic controls at CT1 and CT13. (H, I) Sleep duration in L3 expressing UAS-GCN2-RNAi (H) and UAS-crc-RNAi (I) with Dh44-Gal4 and genetic controls at CT1 and CT13. (A-C) n=32–40 larvae; (D) n=20 larvae; (E-I) n=32–40 larvae. Two-way ANOVAs followed by Sidak’s multiple comparison test (A–C) and (E–I) One-way ANOVAs followed by Sidak’s multiple comparisons tests (D). Source data in Figure 5—source data 1.
-
Figure 5—source data 1
Total sleep duration measures for Dh44 metabolism manipulations in L2 and L3.
- https://cdn.elifesciences.org/articles/97256/elife-97256-fig5-data1-v1.xlsx
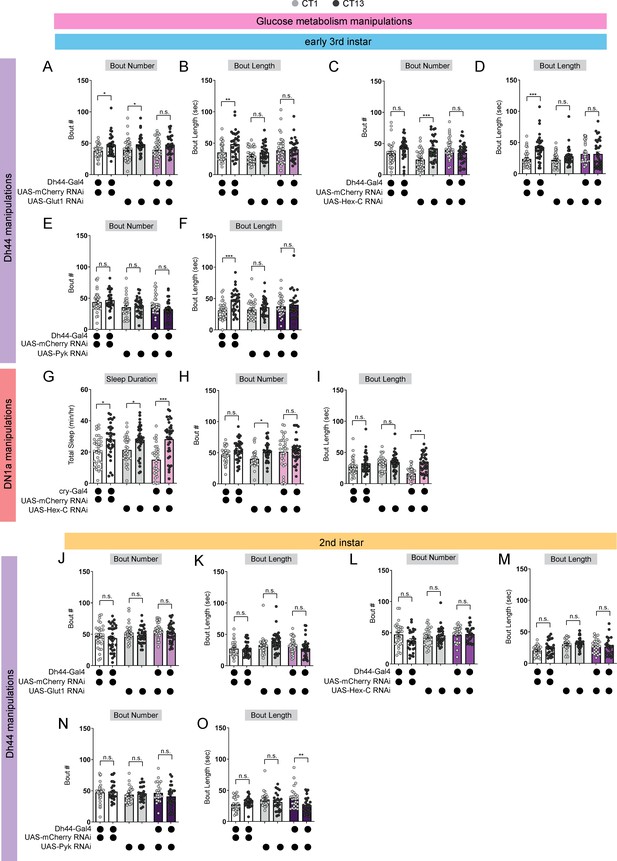
Glucose metabolic gene manipulations affect L3 sleep.
(A, B) Sleep bout number (A) and bout length (B) in L3 expressing UAS-Glut1-RNAi with Dh44-Gal4 and genetic controls at CT1 and CT13. (C, D) Sleep bout number (C) and bout length (D) in L3 expressing UAS-Hex-C-RNAi with Dh44-Gal4 and genetic controls at CT1 and CT13. (E, F) Sleep bout number (E) and bout length (F) in L3 expressing UAS-PyK-RNAi with Dh44-Gal4 and genetic controls as CT1 and CT13. (G–I) Sleep duration (G), sleep bout number (H), and bout length (I) in L3 expressing UAS-Hex-C-RNAi with cry-Gal4 and genetic controls at CT1 and CT13. (J, K) Sleep bout number (J) and bout length (K) in L2 expressing UAS-Glut1-RNAi with Dh44-Gal4 and genetic controls at CT1 and CT13. (L, M) Sleep bout number (L) and bout length (M) in L2 expressing UAS-Hex-C-RNAi with Dh44-Gal4 and genetic controls at CT1 and CT13. (N, O) Sleep bout number (N) and bout length (O) in L2 expressing UAS-PyK-RNAi with Dh44-Gal4 and genetic controls at CT1 and CT13. (A-O) n=32–40 larvae. Two-way ANOVAs followed by Sidak’s multiple comparison test (A–O). Source data in Figure 5—figure supplement 1—source data 1.
-
Figure 5—figure supplement 1—source data 1
Complete sleep data for Dh44 glucose metabolism manipulations.
- https://cdn.elifesciences.org/articles/97256/elife-97256-fig5-figsupp1-data1-v1.xlsx
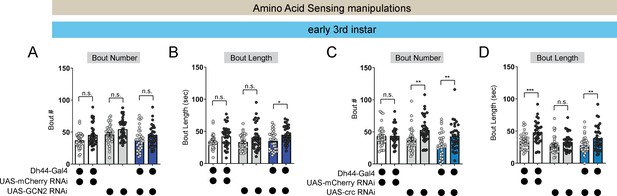
Amino acid sensing gene manipulations do not affect L3 sleep.
(A, B) Sleep bout number (A) and bout length (B) in L3 expressing UAS-GCN2-RNAi with Dh44-Gal4 and genetic controls at CT1 and CT13. (C, D) Sleep bout number (C) and bout length (D) in L3 expressing UAS-crc-RNAi with Dh44-Gal4 and genetic controls at CT1 and CT13. (A-D) n=32–40 larvae. Two-way ANOVAs followed by Sidak’s multiple comparison test (A–D). Source data in Figure 5—figure supplement 2—source data 1.
-
Figure 5—figure supplement 2—source data 1
Raw values for amino acid sensing manipulations in Dh44 neurons.
- https://cdn.elifesciences.org/articles/97256/elife-97256-fig5-figsupp2-data1-v1.xlsx
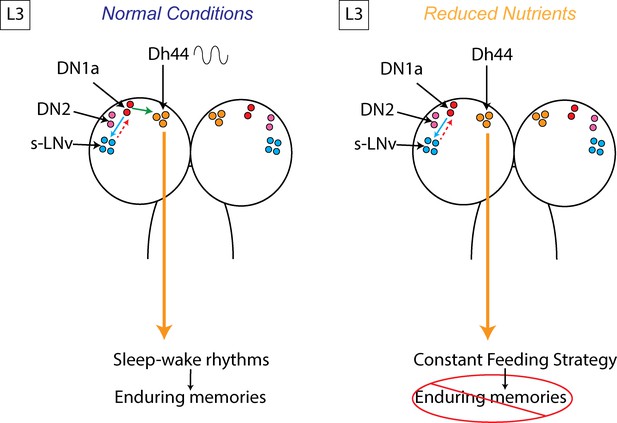
Model Figure.
Clock cells in the larval brain (s-LNv, DN2, and DN1a) communicate to coordinate circadian rhythms. In third instar larvae (L3), a new connection is formed between DN1as and Dh44 neurons, generating daily neural activity rhythms in Dh44 cells that drive sleep-wake patterns, deep sleep, and more enduring memories. In the setting of reduced nutrient availability, the functional connection between the clock (DN1a) and arousal output (Dh44) is not present, facilitating a more constant feeding strategy that benefits the animal under such conditions. However, without clock control of sleep at this stage, deep sleep is lost, as is the ability to exhibit long-term memory.
Additional files
-
MDAR checklist
- https://cdn.elifesciences.org/articles/97256/elife-97256-mdarchecklist1-v1.docx
-
Source code 1
Larval sleep analysis codes.
- https://cdn.elifesciences.org/articles/97256/elife-97256-code1-v1.zip